- 1Faculty of Chemical Engineering, Industrial University of Ho Chi Minh City, Ho Chi Minh, Vietnam
- 2Department of Inorganic Chemistry, Faculty of Science, Palacký University Olomouc, Olomouc, Czechia
- 3Department of Experimental Physics, Faculty of Science, Palacký University Olomouc, Olomouc, Czechia
- 4Regional Center of Advanced Technologies and Materials, Czech Advanced Technology and Research Institute, Palacky University Olomouc, Olomouc, Czechia
Synthesis of bi-functional covalent organic polymers in two distinctive morphologies has been accomplished by simply switching the solvent from DMF to DMSO when 1,3,5-tribenzenecarboxyldehyde and 2,5-diaminobenzene sulfonic acid were reacted via Schiff base condensation reaction to afford covalent organic polymers (COPs) encompassing flower (F-COPDMF)- and circular (C-COPDMSO)-type morphologies. Chemical and morphological natures of the synthesized COPs were compared by characterization using TEM, SEM, XRD, FT-IR, and XPS analysis techniques. Besides diverse morphology, both the polymeric materials were found to comprise similar chemical natures bearing protonic acid–SO3H and Lewis base–C=N functionalities. Subsequently, both the COPs were evaluated for the synthesis of hydroxymethylfurfural (HMF) by the dehydration of fructose to investigate their morphology-dependent catalytic activity.
Introduction
The synthesis of covalent organic polymers with diverse functionalities and morphology has always been a desirable pursuit in terms of their broad applicability (Li et al., 2017; Chen et al., 2021; Namsheer and Rout, 2021). Organic polymers comprise an important class of nanomaterials, which includes porous polymer networks (PPNs) (Yuan et al., 2011), polymers of intrinsic microporosity (PIMs) (McKeown, 2017), conjugated microporous polymers (CMPs) (Lee and Cooper, 2020), covalent organic frameworks (COFs) (Guan et al., 2020; Li et al., 2020), hyper-cross-linked polymers (HCPs) (Masoumi et al., 2021), crystalline triazine-based frameworks (CTFs) (Guo et al., 2021), porous organic frameworks (POFs) (Zhang et al., 2018), porous polymer frameworks (PPFs) (Zhu et al., 2013), polymeric organic networks (PONs) (Jeon et al., 2012), and porous aromatic frameworks (PAFs) (Yuan and Zhu, 2019). This area of research has witnessed a prodigious growth in terms of both synthetic diversity as well as applicability in the field of gas storage (Ahmed et al., 2018), energy storage (Magu et al., 2019), separation (Li et al., 2019a), sensors (Anik et al., 2019), and heterogeneous catalysis (Li et al., 2019b). Generally, these nanomaterials are synthesized via boronic acid condensation (Kubo et al., 2015), imine formation (Segura et al., 2016), Suzuki coupling (Zhou et al., 2019), and Friedel-crafts alkylation (Troschke et al., 2017). C-N linked organic polymers such as triazine-, imine-, and hydrazone-linked polymers are comparatively stable and do not decompose easily in the presence of moisture and thus are widely accepted, including the catalysis arena. Their physical properties, namely, morphology, crystalline/amorphous nature, and porous nature, depend on the reaction conditions such as catalyst, solvent system, temperature, and substrates. Additionally, the intrinsic geometrical features of the building blocks dictate the chemical nature and final topology of the materials. It has been well documented that the morphology and functionality of a nanomaterial significantly affect its catalytic activity and thus can be tuned as per the target applications (Zhuang et al., 2015). Moreover, morphological changes themselves have been found to be effective in various applications because of the morphology–activity correlation (Mondal et al., 2014; Ghorbanloo et al., 2017). Consequently, the synthesis of covalent organic polymers (COPs) with substituted back bone structure and nanoscale morphologies is an emerging field of research. However, it is challenging to acquire the morphology-controlled nanostructure due to the unrestricted kinetic of polymerization (Trewin and Cooper, 2010). Various synthesis methods have been developed involving template-assisted, external cross-linkers, and solvent knitting methods (Thomas et al., 2008; Zhao et al., 2013; Wang et al., 2017; Gao et al., 2019; Giri et al., 2022). Although a wide range of substrates have been used to design and synthesize newer types of COPs, most of these approaches require either an external cross-linker or a catalyst. Therefore, the development of a facile method to synthesize the bi-functional COPs with diverse morphology could be desirable for the organocatalytic applications (Gharpure et al., 2013; Khan et al., 2018; Lambat et al., 2019a; Lambat et al., 2019b; Khan et al., 2020; Chopra et al., 2021).
In this study, we have investigated the role of organic solvents in switching the morphology of organic polymers employing benzene-1,3,5-tricarboxaldehyde and 2,5-diaminobenzene sulfonic acid as the building blocks via the Schiff condensation process without the deployment of any catalyst and external cross-linkers. The choice of the sulfonic acid functional groups substituted substrate as one of the building blocks was intentional, to afford acidic COPs for targeting their applications in biomass upgradation. Carbohydrates represent 75% of the annual renewable biomass for the synthesis of various value-added chemicals of tremendous importance (Kumar et al., 2014; Verma et al., 2016; Verma et al., 2017a; Verma et al., 2017b; Tadele et al., 2017; Den et al., 2018) where HMF has been listed as one of the valuable biomass-derived products, and thus, its production involving fructose is of enormous interest (Sayed et al., 2020); it serves as an active intermediate for the preparation of value-added chemicals including dimethyl furan and levulinic acid (Aljammal et al., 2019). Thus, the ensued COPs bearing the Brønsted acidic sites were accordingly utilized as acid catalysts for the HMF production by fructose dehydration (Baig et al., 2016).
Experimental
Synthesis of Covalent Organic Polymers (F-COPDMF and C-COPDMSO)
For COP synthesis, 0.2 mmol of benzene-1,3,5-tricarboxaldehyde and 0.2 mmol of 2,5-diaminobenzene sulfonic acid were taken in 20 ml of dimethylformamide and 20 ml dimethylsulfoxide, and the solutions were sonicated for 5 min at room temperature. The resulting homogeneous wine-colored solutions were flushed with nitrogen and heated at 130°C for 24 h. After the reaction, solid materials were separated via centrifuge and washed with dehydrated solvents until the supernatant appeared colorless and then dried under vacuum at 60°C to yield brown-colored materials, represented here as F-COPDMF and C-COPDMSO. The other organic polymer, Fe-COPDMF, was prepared following the same procedure, except using an additional 0.2 mmol of iron chloride salt while maintaining the same molar ratio of the reacting components, benzene-1,3,5-tricarboxaldehyde and 2,5-diaminobenzene sulfonic acid.
Dehydration of Fructose to HMF
The catalytic activity of the prepared COPs was evaluated in the synthesis of HMF via the dehydration of fructose. For the synthesis of HMF, a 10-ml pressure tube (Sigma Aldrich) was charged with 0.3 mmol of fructose and 20 mg of the COFs (F-COPDMF or C-COPDMSO) as catalyst and 2 ml DMSO as solvent. The pressure tube was transferred into a preheated oil bath at 130°C, and then the stirring continued for 3 h. After the completion of the reaction, the catalyst was separated by centrifugation. The yield of HMF was calculated to be 83 and 76% for F-COPDMF and C-COPDMSO, respectively, and determined by using the UV-visible spectroscopy technique as described in the literature (Zhang et al., 2013).
Results and Discussions
One of the widely used strategies for the synthesis of COPs involves the condensation among the aromatic amines and aromatic aldehydes to afford the comparably stable imine-based polymeric network structures. In this case, COP materials with two different morphologies were successfully synthesized by taking equimolar amounts of benzene-1,3,5-tricarboxaldehyde and 2,5-diaminobenzenesulfonic acid in two different solvents. The application of DMF and DMSO solvents resulted in the nano flower and circular disk type morphology, respectively, under the same reaction conditions. The morphology transformation could be related to the polarity and chemical nature of the solvents. It was observed that 2,5-diaminobenzenesulfonic acid was not fully dissolved in most of the organic solvents because of the presence of sulfonic acid and amino functional groups and thus behaved as zwitterion. However, one of the imperative conditions for the facile growth of organic polymers is to have a better solubility of the substrates in the solvent system. Therefore, different solvent systems have been reported for the synthesis of assorted organic polymers. DMF and DMSO are nitrogen- and sulfur-containing highly polar organic solvents, which can provide the better solubility for a range of substrates. Moreover, the presence of substituted groups in the substrates evidently affects the nature of the polymerization and, thus, the physical and chemical properties of the ensuing polymeric material (Kim et al., 2001; Gao et al., 2020). Bulky groups are prone to destabilizing the framework structure due to the steric hindrance and may lead to the crosslinking of the substrates. In this case, the sulfonic acid functional group is a bulky substituted group and could be responsible for strained intermediate formation due to the lack of keto-enol tautomerization, thus facilitating the crosslinking polymerization (Peng et al., 2016). Moreover, different chemical interactions between solvents and substrates might be playing a vital role in stabilizing the intermediates, resulting in two different morphologies (Gao et al., 2018). There could be two possible different growth patterns in the respective solvents as shown in Figure 1. Therefore, we can conclude that the polar and chemical natures of the solvents are the factors, which has not only resulted in the reaction to ensue but also provided diverse morphologies.
The morphological identification of both materials was confirmed by using SEM and TEM analytical techniques, and the images are depicted in Figure 2. In case of C-COPDMSO, the TEM images clearly showed the presence of agglomerated and stacked circular disks with the size in the range of 100–200 nm. However, TEM images F-COPDMF indicated the presence of nanoflowers, with the sizes varying from 150 to 250 nm. A sheer dissimilarity between both the organic polymer structures is evident and thus confirmed the distinct effect of the solvent. Moreover, the morphology of the C-COPDMSO was found to be like that of the Cu2S bonded sulfonated organic polymers (Kumar et al., 2020). Interestingly, these results prompted us to envision that not only the solvent but also the presence of metal can play a vibrant role in determining the final morphology of the materials. Adhering to this thought, we synthesized the Fe-COPDMF material using iron chloride salt to recognize any possible effect on the morphology under the same reaction conditions. The synthesized material was characterized by the SEM and TEM analysis and shown in Supplementary Figure S1. In the SEM images, a mixed type of morphology containing disk as well as spherical domains in the range of the 200–500 nm were observed. In case of TEM images, Fe-COFDMF showed a high degree of resemblance in their bulk structures. It was difficult to find the individual domain due to the high agglomeration, and the morphology was found to be of the distorted spherical type. This study provided clear evidence that not only the solvent but also the type of metals can significantly affect the morphology of the resulting materials, as is evident in previous reports (Gao et al., 2018; Giri et al., 2022); further studies relating to the effect of metal are presently under progress in our group. In order to stick to the metal-free morphology transformation, we further study the chemical nature of the F-COPDMF or C-COPDMSO using the XPS analysis.
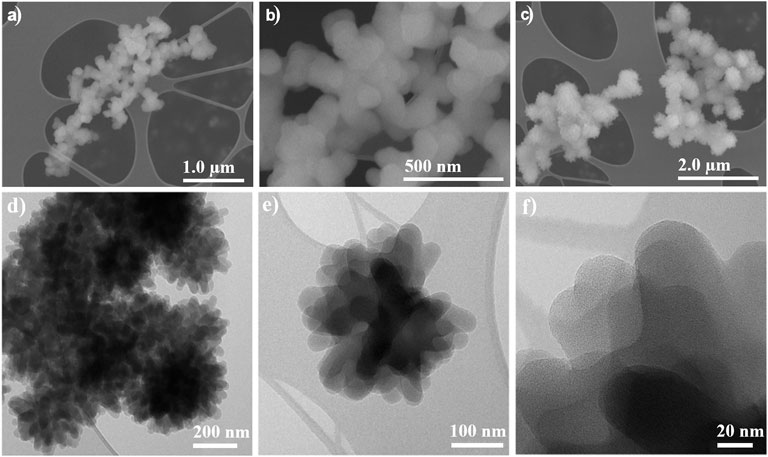
FIGURE 2. (A,B) SEM images of C-COPDMSO; (C) SEM image; and (D–F) TEM images of the F-COPDMF with different size scales.
X-ray photoluminescence spectroscopy (XPS) is a widely deployed surface technique to study the elemental composition and the probable bond connectivity. Subsequently, both the materials (F-COPDMF and C-COPDMSO) were subjected to the XPS analysis to provide the survey scan and high resolution XPS spectra of individual elements present in the different samples as shown in Figures 3A–D and Supplementary Figures S2B,C. The survey scan spectra of both the samples were similar in terms of presence of elements and thus confirmed the availability of C, O, N, and S atoms in both the samples. However, elemental compositions of both the materials were found to be different (Supplementary Figure S2B). The elemental composition was calculated to be C = 70.7; N = 10; O = 16.5; S = 2.8 at% for F-COFDMF and C = 76.4; N = 6.3; O = 15.3; and S = 2 at% for C-COFDMSO. In case of F-COFDMF, the atomic percent of S is found to be higher than that in C-COFDMSO, indicating the presence of higher sulfur-containing groups. Notably, F-COFDMF was synthesized using sulfur-free organic solvent, DMF, thus excluding the possibility of the role of solvent used in doping of the materials. High-resolution deconvoluted spectra of the respective elements recognized different bonding patterns. The peaks at 167, 284, 400, and 532 eV can be correlated with S2p, C1s, N1s, and O1s, respectively (Supplementary Figure S2A). In both the samples, the peak for C1s further splits into three peaks positioning at 284.7, 285.6, and 288.7 eV and thus can be correlated with the C–C/C=C, C=N/C-S linkages and oxidized carbon, respectively, as depicted in Supplementary Figures S2C,D. These peaks are presumably stemming from the aromatic regions and imine bond creation because of polymeric structure formation, while these peaks can be further corroborated from the high-resolution S2p and N1s XPS spectra. The presence of uncondensed -NH2 groups can be identified with the help of N1s spectra. The peak at 400.6 eV in N1s spectra reveals the presence of uncondensed–NH2 groups. In the case of C-COPDMSO, the intensity was higher than that of F-COPDMF, indicating the higher number of uncondensed amino groups. Moreover, lower intensity of the peak at 401.6 eV related to the C-N bonds, further affirming the lack of imine bond formation. The peak responsible for C=N-C linkage positioned at 398.8 eV was present in both the spectra (Figures 3A,B). The peaks at 167.6 eV for the S2p spectra of both the samples are the characteristic peaks ascribable to the availability of sulfonic acid groups on the surface of the polymeric materials. Furthermore, the peak position of deconvoluted S2p XPS spectra showed two peaks at 167.4 and 168.5 eV corresponding to the S2p3/2 and S2p1/2, which further authenticates the existence of the sulfonic acid group (Figures 3C,D). Notably, no other sulfur-containing functional groups such as thiol were observed in both the materials. The bonding pattern of the respective elements in each material was found to be similar, with a very small change in the peak positions. All these results authenticate the successful formation of COPs.
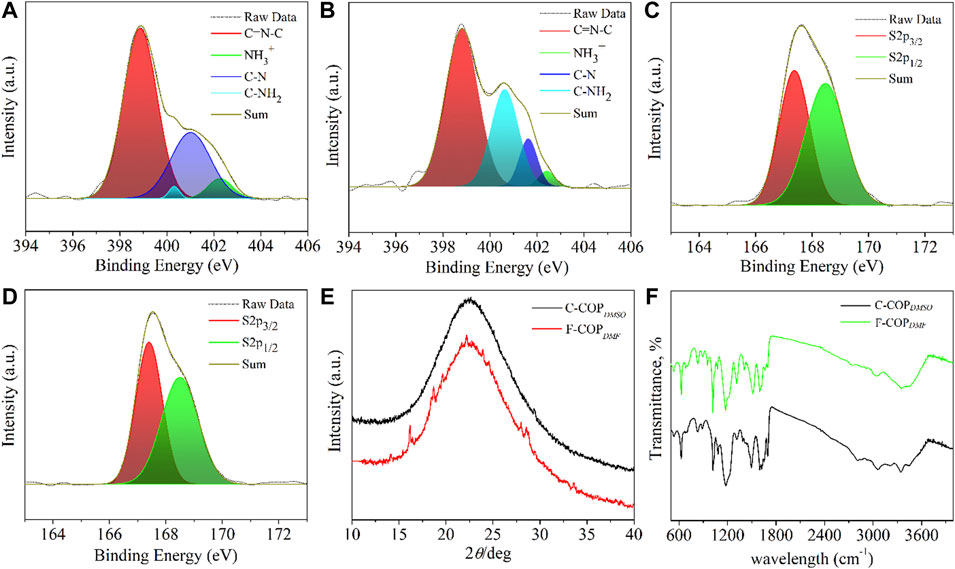
FIGURE 3. (A) N1s of F-COPDMF; (B) N1s of C-COPDMSO; (C) S2p of F-COPDMF; (D) S2p of C-COPDMSO; (E) XRD spectra; and (F) FT-IR spectra.
X-ray powder diffraction was utilized to determine the amorphous/crystalline nature of both the COPs (F-COPDMF and C-COPDMSO). A broad amorphous halo centered at 23° 2θ presented in both cases is indicative of the amorphous nature of the materials (Figure 3E). (Murthy and Minor, 1990; Yildirim and Derkus, 2020) These results appear to support the hypothesis that the size of surface-exposed functional moieties affects the crystallinity of the polymeric materials because of steric hindrance that causes angle strain between the substrates. Moreover, the low-intensity diffraction peaks observed in the diffraction pattern of F-COPDMF could be attributed to the generation of micro-crystallites in the material.
The FT-IR spectra of F-COPDMF or C-COPDMSO were scanned in the range of 400–4000 cm−1 and compared to witness any possible changes in the form of functional groups attributable to the DMF- and DMSO-assisted solvothermal condensation (Figure 3F). The characteristic stretching vibrations for the C=N functional groups at 1629 cm−1 appeared, thus affirming the successful condensation between benzene-1,3,5-tricarboxaldehyde and 2,5-diaminobenzenesulfonic acid. However, the intensity was diminished more for F-COPDMF in the region of 3200–3600 cm−1, which is primarily responsible for the availability of uncondensed -NH2 groups, indicating a higher extent of condensation than C-COPDMSO. Furthermore, symmetric and asymmetric vibration modes corresponding to the sulfonic groups at 1019, 1078, and 1218 cm−1 indicate that the sulfonic groups are well intact on their surface. The other common peaks could be associated to various stretching and bending vibrations of functional groups in the materials. XPS and FTIR techniques confirmed the presence of sulfonic acid functional groups on the surface of the polymeric material without showing any possible reduction of sulfonic acid into thiol groups during the synthesis process and thus come out to be a potential candidate as an acid catalyst. Moreover, the nitrogen adsorption-desorption isotherms were recorded to determine the surface area. F-COPDMF and C-COPDMSO presented type-II curves with Brunauer–Emmett–Teller (BET) surface areas of 50.03 and 48.89 m2/g, respectively (Supplementary Figure S3).
Subsequently, the catalytic activity of the prepared organic polymers (F-COPDMF or C-COPDMSO) was assessed and compared relating to the HMF formation via the dehydration of fructose in terms of yields (Scheme 1). The experiments were carried out in a 10.0-ml glass tube encompassing the fructose and catalyst in DMSO. The reaction was continued for 3 h under stirring at 130°C. After completion of the reaction, the glass tube was cooled in an ice bath, and the catalyst was separated by filtration. The resulting reaction mixture was analyzed using the UV-Vis spectroscopy technique by following the procedure documented elsewhere (Zhang et al., 2013).
The maximum yield of HMF under the optimized reaction conditions using F-COPDMF or C-COPDMSO was found to be 83 and 76%, respectively. Although, F-COPDMF showed better catalytic activity in terms of HMF yield, we cannot conclude that it is because of only morphological change. It could be due to the higher percentage of sulfonic acid groups. Therefore, at this stage, we can only determine that the DMF helps to retain higher percentage of sulfur in the possible form of sulfonic acid groups that could in turn effect the higher activity of the resulting material.
Conclusion
We have successfully synthesized two organic polymers (F-COPDMF or C-COPDMSO) with two distinct morphologies using a similar set of substrates but different solvent systems. Based on the experimental results, the solvent system not only dictated the final morphology outcome but also helped to retain the higher concentration of sulfur in the form of sulfonic acid groups on the surface of the material. Moreover, higher condensation is observed while using the DMF as solvent. In addition, the role of metal in determining the morphology was evaluated, and the ensued materials were evaluated for the synthesis of HMF; higher activity of the catalysts might be due to the presence of acidic groups. In case of HMF synthesis, dehydration of fructose to HMF is catalyzed by Bronsted acidic groups. This interesting protocol certainly opens the door to further material development by deploying a single solvent for synthesis and morphological variation of the material. Results also suggest that the product yields attained are almost similar in both the materials and have no morphological effect on the catalytic activity.
Data Availability Statement
The original contributions presented in the study are included in the article/Supplementary Material; further inquiries can be directed to the corresponding authors.
Author Contributions
SK and XC conceptualized the work and wrote the manuscript. IN analyzed and interpreted XRD data. JK performed the BET analysis. RV checked and edited the manuscript. All authors contributed to the article and agreed on the submitted version.
Conflict of Interest
The authors declare that the research was conducted in the absence of any commercial or financial relationships that could be construed as a potential conflict of interest.
Publisher’s Note
All claims expressed in this article are solely those of the authors and do not necessarily represent those of their affiliated organizations, or those of the publisher, the editors, and the reviewers. Any product that may be evaluated in this article, or claim that may be made by its manufacturer, is not guaranteed or endorsed by the publisher.
Acknowledgments
XC acknowledges the financial support from the Industrial University of Ho Chi Minh City, Vietnam. SK and IN gratefully acknowledge the financial support from institutional sources of the Department of Inorganic Chemistry, Palacký University Olomouc, Czech Republic. IN would like to thank O. F. Fellner for assistance with measurement of X-ray powder diffraction. The authors thank J. Stráská and Eirini Ioannou for SEM/TEM analysis and M. Petr for HR-XPS measurements.
Supplementary Material
The Supplementary Material for this article can be found online at: https://www.frontiersin.org/articles/10.3389/fmats.2022.889679/full#supplementary-material
References
Ahmed, D. S., El-Hiti, G. A., Yousif, E., Ali, A. A., and Hameed, A. S. (2018). Design and Synthesis of Porous Polymeric Materials and Their Applications in Gas Capture and Storage: a Review. J. Polym. Res. 25, 1–21. doi:10.1007/s10965-018-1474-x
Aljammal, N., Jabbour, C., Thybaut, J. W., Demeestere, K., Verpoort, F., and Heynderickx, P. M. (2019). Metal-organic Frameworks as Catalysts for Sugar Conversion into Platform Chemicals: State-Of-The-Art and Prospects. Coord. Chem. Rev. 401, 213064. doi:10.1016/j.ccr.2019.213064
Anik, Ü., Timur, S., and Dursun, Z. (2019). Metal Organic Frameworks in Electrochemical and Optical Sensing Platforms: a Review. Microchim. Acta 186, 1–15. doi:10.1007/s00604-019-3321-0
Baig, R., Verma, S., Nadagouda, M. N., and Varma, R. S. (2016). Room Temperature Synthesis of Biodiesel Using Sulfonated Graphitic Carbon Nitride. Sci. Rep. 6, 1–6. doi:10.1038/srep39387
Chen, W., Chen, P., Zhang, G., Xing, G., Feng, Y., Yang, Y.-W., et al. (2021). Macrocycle-derived Hierarchical Porous Organic Polymers: Synthesis and Applications. Chem. Soc. Rev. 50 (20), 11684–11714. doi:10.1039/d1cs00545f
Chopra, P. K. P. G., Lambat, T. L., Mahmood, S. H., Chaudhary, R. G., and Banerjee, S. (2021). Sulfamic Acid as Versatile Green Catalyst Used for Synthetic Organic Chemistry: A Comprehensive Update. ChemistrySelect 6, 6867–6889. doi:10.1002/slct.202101635
Den, W., Sharma, V. K., Lee, M., Nadadur, G., and Varma, R. S. (2018). Lignocellulosic Biomass Transformations via Greener Oxidative Pretreatment Processes: Access to Energy and Value-Added Chemicals. Front. Chem. 6, 141. doi:10.3389/fchem.2018.00141
Gao, Q., Li, X., Ning, G.-H., Xu, H.-S., Liu, C., Tian, B., et al. (2018). Covalent Organic Framework with Frustrated Bonding Network for Enhanced Carbon Dioxide Storage. Chem. Mat. 30, 1762–1768. doi:10.1021/acs.chemmater.8b00117
Gao, T. N., Wang, T., Wu, W., Liu, Y., Huo, Q., Qiao, Z. A., et al. (2019). Solvent‐Induced Self‐Assembly Strategy to Synthesize Well‐Defined Hierarchically Porous Polymers. Adv. Mat. 31, 1806254. doi:10.1002/adma.201806254
Gao, X., Shu, C., Zhang, C., Ma, W., Ren, S.-B., Wang, F., et al. (2020). Substituent Effect of Conjugated Microporous Polymers on the Photocatalytic Hydrogen Evolution Activity. J. Mat. Chem. A 8, 2404–2411. doi:10.1039/c9ta13212k
Gharpure, M., Choudhary, R., Ingle, V., and Juneja, H. (2013). Synthesis of New Series of 3-Hydroxy/acetoxy-2-Phenyl-4h-Chromen-4-Ones and Their Biological Importance. J. Chem. Sci. 125, 575–582. doi:10.1007/s12039-013-0420-z
Ghorbanloo, M., Safarifard, V., and Morsali, A. (2017). Heterogeneous Catalysis with a Coordination Modulation Synthesized MOF: Morphology-dependent Catalytic Activity. New J. Chem. 41, 3957–3965. doi:10.1039/c6nj04065a
Giri, A., Biswas, S., Hussain, M. W., Dutta, T. K., and Patra, A. (2022). Nanostructured Hypercrosslinked Porous Organic Polymers: Morphological Evolution and Rapid Separation of Polar Organic Micropollutants. ACS Appl. Mater. Interfaces 14 (5), 7369–7381. doi:10.1021/acsami.1c24393
Guan, X., Chen, F., Fang, Q., and Qiu, S. (2020). Design and Applications of Three Dimensional Covalent Organic Frameworks. Chem. Soc. Rev. 49, 1357–1384. doi:10.1039/c9cs00911f
Guo, L., Wang, X., Zhan, Z., Zhao, Y., Chen, L., Liu, T., et al. (2021). Crystallization of Covalent Triazine Frameworks via a Heterogeneous Nucleation Approach for Efficient Photocatalytic Applications. Chem. Mat. 33, 1994–2003. doi:10.1021/acs.chemmater.0c03716
Jeon, H. J., Choi, J. H., Lee, Y., Choi, K. M., Park, J. H., and Kang, J. K. (2012). Highly Selective CO2-Capturing Polymeric Organic Network Structures. Adv. Energy Mat. 2, 225–228. doi:10.1002/aenm.201100648
Khan, M., Hayat, A., Baburao Mane, S. K., Li, T., Shaishta, N., Alei, D., et al. (2020). Functionalized Nano Diamond Composites for Photocatalytic Hydrogen Evolution and Effective Pollutant Degradation. Int. J. Hydrogen Energy 45, 29070–29081. doi:10.1016/j.ijhydene.2020.07.274
Khan, M., Tiehu, L., Zhao, T. K., Khurram, A. A., Khan, I., Ullah, A., et al. (2018). Comparative Study of the Ball Milling and Acid Treatment of Functionalized Nanodiamond Composites. Int. J. Refract. Metals Hard Mater. 73, 46–52. doi:10.1016/j.ijrmhm.2018.01.015
Kim, H.-J., Lee, J.-K., Kim, J.-B., Park, E. S., Park, S.-J., Yoo, D. Y., et al. (2001). Substituent Effects on Microstructure and Polymerization of Polyalkylsilsesquioxanes. J. Am. Chem. Soc. 123, 12121–12122. doi:10.1021/ja0168099
Kubo, Y., Nishiyabu, R., and James, T. D. (2015). Hierarchical Supramolecules and Organization Using Boronic Acid Building Blocks. Chem. Commun. 51, 2005–2020. doi:10.1039/c4cc07712a
Kumar, S., Gawande, M. B., Medřík, I., Petr, M., Tomanec, O., Kupka, V., et al. (2020). Mechanochemical Synthesis of Cu2S Bonded 2D-Sulfonated Organic Polymers: Continuous Production of Dimethyl Carbonate (DMC) via Preheating of Reactants. Green Chem. 22, 5619–5627. doi:10.1039/d0gc01030h
Kumar, S., Kumar, J., Kaul, S., and Jain, S. L. (2014). Carbon Dioxide Promoted Hydrolysis of Xylose to Furfural Using 1,1,3,3-Tetramethyl Guanidinium Hydrogen Sulfate: A Remarkable Enhancement in Reaction Rate. Ind. Eng. Chem. Res. 53, 15571–15575. doi:10.1021/ie502614z
Lambat, T. L., Abdala, A. A., Mahmood, S., Ledade, P. V., Chaudhary, R. G., and Banerjee, S. (2019). Sulfamic Acid Promoted One-Pot Multicomponent Reaction: a Facile Synthesis of 4-Oxo-Tetrahydroindoles under Ball Milling Conditions. RSC Adv. 9, 39735–39742. doi:10.1039/c9ra08478a
Lambat, T. L., Chaudhary, R. G., Abdala, A. A., Mishra, R. K., H. Mahmood, S., and Banerjee, S. (2019). Mesoporous PbO Nanoparticle-Catalyzed Synthesis of Arylbenzodioxy Xanthenedione Scaffolds under Solvent-free Conditions in a Ball Mill. RSC Adv. 9, 31683–31690. doi:10.1039/c9ra05903b
Lee, J.-S. M., and Cooper, A. I. (2020). Advances in Conjugated Microporous Polymers. Chem. Rev. 120, 2171–2214. doi:10.1021/acs.chemrev.9b00399
Li, D., Xu, H.-Q., Jiao, L., and Jiang, H.-L. (2019). Metal-organic Frameworks for Catalysis: State of the Art, Challenges, and Opportunities. EnergyChem 1, 100005. doi:10.1016/j.enchem.2019.100005
Li, J., Zhou, X., Wang, J., and Li, X. (2019). Two-Dimensional Covalent Organic Frameworks (COFs) for Membrane Separation: a Mini Review. Ind. Eng. Chem. Res. 58, 15394–15406. doi:10.1021/acs.iecr.9b02708
Li, Q., Razzaque, S., Jin, S., and Tan, B. (2017). Morphology Design of Microporous Organic Polymers and Their Potential Applications: an Overview. Sci. China Chem. 60, 1056–1066. doi:10.1007/s11426-017-9089-3
Li, Y., Chen, W., Xing, G., Jiang, D., and Chen, L. (2020). New Synthetic Strategies toward Covalent Organic Frameworks. Chem. Soc. Rev. 49, 2852–2868. doi:10.1039/d0cs00199f
Magu, T., Agobi, A., Hitler, L., and Dass, P. (2019). A Review on Conducting Polymers-Based Composites for Energy Storage Application. J. Chem. Rev. 1, 19–34. doi:10.33945/SAMI/JCR.2019.1.1934
Masoumi, H., Ghaemi, A., and Gilani, H. G. (2021). Evaluation of Hyper-Cross-Linked Polymers Performances in the Removal of Hazardous Heavy Metal Ions: A Review. Sep. Purif. Technol. 260, 118221. doi:10.1016/j.seppur.2020.118221
McKeown, N. B. (2017). The Synthesis of Polymers of Intrinsic Microporosity (PIMs). Sci. China Chem. 60, 1023–1032. doi:10.1007/s11426-017-9058-x
Mondal, J., Borah, P., Sreejith, S., Nguyen, K. T., Han, X., Ma, X., et al. (2014). Morphology-Tuned Exceptional Catalytic Activity of Porous-Polymer-Supported Mn3O4in Aerobic sp3C-H Bond Oxidation of Aromatic Hydrocarbons and Alcohols. ChemCatChem 6, 3518–3529. doi:10.1002/cctc.201402512
Murthy, N. S., and Minor, H. (1990). General Procedure for Evaluating Amorphous Scattering and Crystallinity from X-Ray Diffraction Scans of Semicrystalline Polymers. Polymer 31, 996–1002. doi:10.1016/0032-3861(90)90243-r
Namsheer, K., and Rout, C. S. (2021). Conducting Polymers: a Comprehensive Review on Recent Advances in Synthesis, Properties and Applications. RSC Adv. 11, 5659–5697. doi:10.1039/d0ra07800j
Peng, Y., Xu, G., Hu, Z., Cheng, Y., Chi, C., Yuan, D., et al. (2016). Mechanoassisted Synthesis of Sulfonated Covalent Organic Frameworks with High Intrinsic Proton Conductivity. ACS Appl. Mat. Interfaces 8, 18505–18512. doi:10.1021/acsami.6b06189
Sayed, M., Warlin, N., Hulteberg, C., Munslow, I., Lundmark, S., Pajalic, O., et al. (2020). 5-Hydroxymethylfurfural from Fructose: an Efficient Continuous Process in a Water-Dimethyl Carbonate Biphasic System with High Yield Product Recovery. Green Chem. 22, 5402–5413. doi:10.1039/d0gc01422b
Segura, J. L., Mancheño, M. J., and Zamora, F. (2016). Covalent Organic Frameworks Based on Schiff-Base Chemistry: Synthesis, Properties and Potential Applications. Chem. Soc. Rev. 45, 5635–5671. doi:10.1039/c5cs00878f
Tadele, K., Verma, S., Gonzalez, M. A., and Varma, R. S. (2017). A Sustainable Approach to Empower the Bio-Based Future: Upgrading of Biomass via Process Intensification. Green Chem. 19, 1624–1627. doi:10.1039/c6gc03568j
Thomas, A., Goettmann, F., and Antonietti, M. (2008). Hard Templates for Soft Materials: Creating Nanostructured Organic Materials. Chem. Mat. 20, 738–755. doi:10.1021/cm702126j
Trewin, A., and Cooper, A. I. (2010). Porous Organic Polymers: Distinction from Disorder? Angew. Chem. Int. Ed. 49, 1533–1535. doi:10.1002/anie.200906827
Troschke, E., Grätz, S., Lübken, T., and Borchardt, L. (2017). Mechanochemical Friedel-Crafts Alkylation-A Sustainable Pathway towards Porous Organic Polymers. Angew. Chem. Int. Ed. 56, 6859–6863. doi:10.1002/anie.201702303
Verma, S., Baig, R. B. N., Nadagouda, M. N., Len, C., and Varma, R. S. (2017). Sustainable Pathway to Furanics from Biomass via Heterogeneous Organo-Catalysis. Green Chem. 19, 164–168. doi:10.1039/c6gc02551j
Verma, S., Baig, R. B. N., Nadagouda, M. N., and Varma, R. S. (2016). Sustainable Strategy Utilizing Biomass: Visible-Light-Mediated Synthesis of γ-Valerolactone. ChemCatChem 8, 690–693. doi:10.1002/cctc.201501352
Verma, S., Nadagouda, M. N., and Varma, R. S. (2017). Fixation of Carbon Dioxide into Dimethyl Carbonate over Titanium-Based Zeolitic Thiophene-Benzimidazolate Framework. Sci. Rep. 7, 655. doi:10.1038/s41598-017-00736-1
Wang, S., Zhang, C., Shu, Y., Jiang, S., Xia, Q., Chen, L., et al. (2017). Sci. Adv. 3, e1602610. doi:10.1126/sciadv.1602610
Yildirim, O., and Derkus, B. (2020). Triazine-based 2D Covalent Organic Frameworks Improve the Electrochemical Performance of Enzymatic Biosensors. J. Mater Sci. 55, 3034–3044. doi:10.1007/s10853-019-04254-5
Yuan, D., Lu, W., Zhao, D., and Zhou, H.-C. (2011). Highly Stable Porous Polymer Networks with Exceptionally High Gas-Uptake Capacities. Adv. Mat. 23, 3723–3725. doi:10.1002/adma.201101759
Yuan, Y., and Zhu, G. (2019). Porous Aromatic Frameworks as a Platform for Multifunctional Applications. ACS Cent. Sci. 5, 409–418. doi:10.1021/acscentsci.9b00047
Zhang, J., Li, J., Tang, Y., and Xue, G. (2013). Rapid Method for the Determination of 5-Hydroxymethylfurfural and Levulinic Acid Using a Double-Wavelength UV Spectroscopy. Sci. world J. 2013, 506329. doi:10.1155/2013/506329
Zhang, S., Yang, Q., Wang, C., Luo, X., Kim, J., Wang, Z., et al. (2018). Porous Organic Frameworks: Advanced Materials in Analytical Chemistry. Adv. Sci. 5, 1801116. doi:10.1002/advs.201801116
Zhao, J., Niu, W., Zhang, L., Cai, H., Han, M., Yuan, Y., et al. (2013). A Template-free and Surfactant-free Method for High-Yield Synthesis of Highly Monodisperse 3-Aminophenol-Formaldehyde Resin and Carbon Nano/Microspheres. Macromolecules 46, 140–145. doi:10.1021/ma302119t
Zhou, D., Tan, X., Wu, H., Tian, L., and Li, M. (2019). Synthesis of C−C Bonded Two-Dimensional Conjugated Covalent Organic Framework Films by Suzuki Polymerization on a Liquid-Liquid Interface. Angew. Chem. 131, 1390–1395. doi:10.1002/ange.201811399
Zhu, Y., Long, H., and Zhang, W. (2013). Imine-Linked Porous Polymer Frameworks with High Small Gas (H2, CO2, CH4, C2H2) Uptake and CO2/N2 Selectivity. Chem. Mat. 25, 1630–1635. doi:10.1021/cm400019f
Keywords: covalent organic polymer, HMF, morphology-dependent activity, biomass upgradation, morphological transformations
Citation: Cao XT, Kumar S, Nemec I, Kopp J and Varma RS (2022) Solvent-Directed Morphological Transformation in Covalent Organic Polymers. Front. Mater. 9:889679. doi: 10.3389/fmats.2022.889679
Received: 04 March 2022; Accepted: 04 May 2022;
Published: 28 June 2022.
Edited by:
Muhammad Idrees, Shenzhen University, ChinaReviewed by:
Ratiram Gomaji Chaudhary, Seth Kesarimal Porwal College, IndiaShengyu Dai, Anhui University, China
Muhammad Khan, Northwestern Polytechnical University, China
Copyright © 2022 Cao, Kumar, Nemec, Kopp and Varma. This is an open-access article distributed under the terms of the Creative Commons Attribution License (CC BY). The use, distribution or reproduction in other forums is permitted, provided the original author(s) and the copyright owner(s) are credited and that the original publication in this journal is cited, in accordance with accepted academic practice. No use, distribution or reproduction is permitted which does not comply with these terms.
*Correspondence: Xuan Thang Cao, caoxuanthang@iuh.edu.vn; Subodh Kumar, subodh.kumar@upol.cz