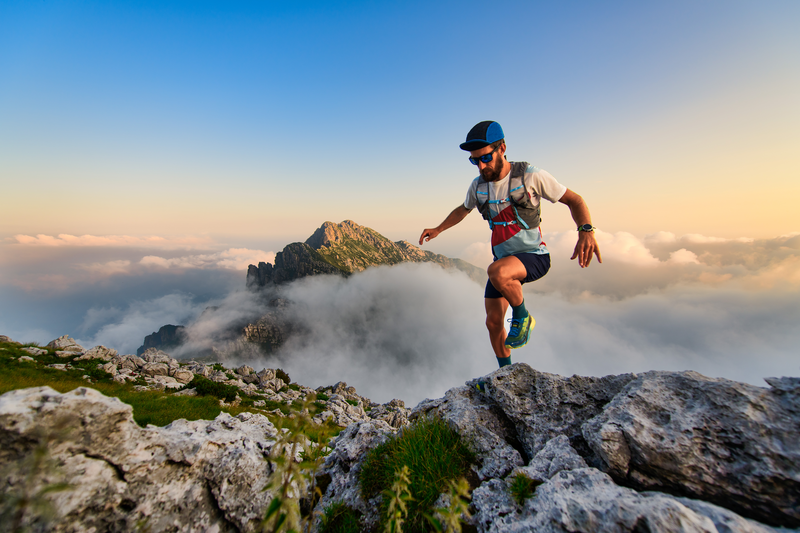
95% of researchers rate our articles as excellent or good
Learn more about the work of our research integrity team to safeguard the quality of each article we publish.
Find out more
MINI REVIEW article
Front. Mater. , 08 April 2022
Sec. Biomaterials
Volume 9 - 2022 | https://doi.org/10.3389/fmats.2022.878176
The development of drug-eluting bioactive coatings for orthopedic implants has gained increased interest in recent years with an intent to reduce postoperative complications and improve tissue regeneration at the implant interface. Due to the remarkable benefits of natural polyphenolic components, such as antioxidant, antimicrobial, anti-inflammatory, anti-cancer and bioactive activity, and their ubiquitous availability in nature, they are promising candidates for incorporation into bioactive coatings of advanced medical devices in future clinical applications. However, further research is needed to address all challenges. This review aims to highlight the prosperity of natural compounds widely available in nature loaded in implantable devices, summarize the “state of the art” in this field, identify the challenges, and accordingly suggest the optimal preparation methods and characterization.
Despite evident advances in the development of implantable orthopaedic devices and usually favourable surgical outcomes, revision surgeries are still occasionally required due to infection, inadequate stability, and aseptic loosening (Levent et al., 2021). Current implants are generally made of biocompatible metallic materials (medical grade stainless steel, titanium, titanium alloys, etc.), which have adequate mechanical stability and corrosion resistance but insufficient biological response necessary for tissue growth around the implant (Hench and Jones, 2005). A viable approach to improve osteointegration of implants is by preparation of drug-eluting bioactive coatings for implants with osteoinductive, osteoconductive (Song et al., 2021a), biocompatible, antimicrobial, and anti-inflammatory effects (Bagherifard, 2017). Thus far, mostly synthetic bioactive substances such as growth factors (Yu et al., 2022), osteoclast inhibitors (Bjelić and Finšgar, 2022), antibiotics (Li et al., 2022), and anti-inflammatory drugs (Gherasim et al., 2021) have been at the forefront for this purpose. However, their main disadvantages are associated with their possible incompatibility and high cost. For example, commercially available growth factors are fairly unstable and are produced using recombinant technology, making them extremely expensive. Therefore, the focus has been on preparing simple, inexpensive, and, most importantly, effective bioactive coatings (Córdoba et al., 2015).
Natural compounds such as polyphenols (anthocyanidins, catechins, flavanones, flavones, flavonols, isoflavones, hydroxybenzoic acids, hydroxycinnamic acids, lignans, and tannins) are of great interest in pharmaceutical, nutraceutical, and medical fields due to their antioxidant, antimicrobial, anti-inflammatory, bioactive, and anti-cancer effects (Fang and Bhandari, 2010). With their diverse benefits, abundance, and resulting affordability, they can be employed in a wide range of pharmaceutical and biomedical applications by incorporating them into suitable formulations that promote controlled release (Lewandowska et al., 2013; Agrawal, 2015; de Araújo et al., 2021). Thus far, numerous attempts have been made to use polyphenols for oral drug delivery, successfully improving the oxidative stability, light insensitivity, and bioavailability of poorly water-soluble bioactive compounds (Wang et al., 2018; Ahmadi et al., 2019). In combination with biocompatible, porous, and biodegradable polymers, they show promise for wound dressing due to the breathability, ability to absorb excess exudate, and antibacterial activity of the biofilms obtained (Fras Zemljič et al., 2020; Maver et al., 2020). In addition, polyphenols have been shown to have selective toxicity toward cancer cells and a protective role on healthy cells. Therefore, they are great candidates for grafting onto ferrimagnetic materials to stimulate anti-cancer activity and hyperthermia simultaneously (Córdoba et al., 2015). It was previously suggested that polyphenols grafted onto bioactive glasses are effective as bone substitutes in cancer treatment (Cazzola et al., 2017). While many studies (Fraga et al., 2010; Gorzynik-Debicka et al., 2018; Enaru et al., 2021) have confirmed the benefits of bioactive substances from natural sources, references regarding their incorporation into bioactive coatings for orthopedic implants are limited. The objective of this review is to highlight the advantages and challenges of bioactive substances isolated from plants or microorganisms in implantable devices and to summarize the current research data in this field. The masucript will also focus on the preparation of such coatings by suggesting deposition techniques, encapsulation methods to maintain the efficacy of the otherwise unstable bioactive substances, and characterization required to evaluate the properties of the coatings obtained, allowing their improvements to achieve optimal bioactive coatings in the future.
In the development of coating for the implant, synthetic drugs, polyphenols alone, or polyphenol-rich extracts from natural sources are either immobilized on the substrate (implant) or deposited in combination with other materials on the substrate using well establised deposition techniques. Several studies confirm that incorporating polyphenols meets the requirements for use in bioactive implant coatings, such as osteoinductivity, osteoconductivity, biocompatibility, corrosion inhibition, and antibacterial and anti-inflammatory activity, and, more importantly, contributes to their improvement. The bioactive coatings for implants containing natural compounds developed to date, the benefits obtained, and related references are listed in Table 1.
TABLE 1. Summary of natural compounds included in bioactive coatings for implants, matrix/substrate used, and benefits reported in the literature.
Various coating deposition techniques have been developed to ensure uniformity, desired thickness, sufficient load-bearing mechanical properties, controlled release, and high adhesion to the implant (Asri et al., 2016; Ehlert et al., 2011). The simplest yet very effective are dip coating (Babu et al., 2004; Banerjee and Bose, 2019), drop casting (Shukla and Bhathena, 2015), and layer-by-layer deposition techniques (Yang et al., 2019). However, over the years, immense progress has been made by implementing electrophoretic deposition (Erakovic et al., 2014), electrospinning (Ji et al., 2014), plasma spraying (Sarkar and Bose, 2020), physical vapor deposition (Aktug et al., 2019), chemical vapor deposition (Youn et al., 2019), sol-gel (Omar et al., 2020), and biomimetic deposition, the latter being especially prosperous by encountering heterogeneous nucleation and crystal growth of the coating with bone-like properties (Koju et al., 2017). Nevertheless, not all are suitable for the deposition of fairly sensitive natural bioactive compounds, as they would not withstand the working conditions (e.g., high processing temperatures). It was shown previously that titania nanotubes (Figure 1)prepared by anodization of pure Ti or its alloys are very promising. They combine the ability to integrate bioactive compounds in their hollow structure and allow bioactivity enhancement by cell attachment to nanotopographic surface properties (Mohan et al., 2016).
FIGURE 1. SEM images of titania nanotubes prepared by anodization of Ti substrate: (A,B), top view (C) cross-sectional view. Reprinted with permission from (Tong et al., 2021).
The importance of 3D printing should also be emphasized because it can be used for the production of tailorable bioactive coatings and can in the future easily enable the fabrication of personalized implant coatings by varying the coating material as well as the type and dosage of bioactive compounds to be released from the matrix (Li et al., 2019; Maver et al., 2021).
Despite the many advantageous properties of bioactive compounds of natural origin, they often lack stability and solubility in body fluids (Parisi et al., 2014; Wildman et al., 2016; Lu et al., 2016). To ensure their maximum efficacy, various encapsulation methods have been established over the years to 1) protect the active compounds from undesirable environmental factors such as light, temperature, moisture, oxygen, etc., thereby reducing their reactivity and spoilage, 2) to allow controlled release of the compounds, 3) to mask the taste and odor (e. g. in food industry), and 4) to achieve the desired dosage and dispersion of the compounds in the matrix (Sonawane et al., 2020). The recently developed encapsulation methods are already explained in detail by several references (Fang and Bhandari, 2010; Munin and Edwards-Lévy, 2011; Castro-Rosas et al., 2017) and are generally divided into physical, physiochemical, or chemical methods as shown in Table 2, with the main principle of incorporating the core material (bioactive compound) into the wall material in reservoir or matrix manner to preserve its biological, chemical, and physical properties (Munin and Edwards-Lévy, 2011; Sonawane et al., 2020).
TABLE 2. Classification and a short description of encapsulation methods used for active compounds (Munin and Edwards-Lévy, 2011).
To the best of the authors’ knowledge, very few, if any, studies have been found on the encapsulation of natural compounds by the methods mentioned above for use in coatings for orthopedic implants (Cazzola et al., 2018; Gamna and Spriano, 2021; Riccucci et al., 2021; Riccucci et al., 2022). Nevertheless, they are promising for future research as they have been extensively studied for use in the food industry. Therefore, a more in-depth evaluation of some of the methods is presented below. Spray drying is one of the most frequently used encapsulation techniques due to its simplicity, low operating cost, adequate yield, uniform, spherical particle size, and high stability of the obtained capsules (Mahdavi et al., 2014). Its disadvantage lies mainly in the application of high processing temperatures, which can lead to the degradation of thermally sensitive compounds (Belščak-Cvitanović et al., 2011; Sun-Waterhouse et al., 2013; González et al., 2019; Chaumun et al., 2020; Goëlo et al., 2020). However, an example of the aromatic evergreen tree Laurus nobilis L., rich in phenols, which has been alongside gallic acid (GA) encapsulated by the spray drying method in different polymer matrices, allowed yields ranging from 73 to 99%, while in vitro drug release testing simulated body fluids (SBF) allowed controlled release (Chaumun et al., 2020).
Furthermore, encapsulation methods using supercritical fluids (SCFs) have been established as green technologies. They are a promising alternative to conventional methods because they do not require the use of harmful organic solvents and have suitable operating parameters, especially when using supercritical CO2 (SC-CO2) with the critical point of 31°C and 73.8 bar, offer the possibility of dissolving both polar and nonpolar bioactive compounds, produce practically no waste, and have high encapsulation efficiency. Depending on the role of SC-CO2, which can act as a solvent, solute, or anti-solvent, three encapsulation methods were developed, namely Rapid Expansion of Supercritical Solutions (RESS), Particles from Gas Saturated Solutions (PGSS™), and Supercritical Anti Solvent (SAS), respectively (Weidner et al., 2004; Munin and Edwards-Lévy, 2011; Kravanja et al., 2018; Klettenhammer et al., 2020). In a study by Gonçalves et al. (Gonçalves et al., 2016), PGSS™ was used to encapsulate epigallocatechin gallate (EGCG) to preserve its chemical stability using modified n-octenyl succinate anhydride starch, soybean lecithin, and barley-β-glucan as polymer carriers. The obtained products showed no cytotoxicity, improved storage stability, and maintenance of antioxidant activity with all polymer carriers at an encapsulation efficiency of about 80%. Furthermore, β-glucan and lecithin facilitated the intracellular activity of EGCG, and lecithin as a carrier promoted a more sustained EGCG release in SBF (Gonçalves et al., 2016).
Among physiochemical methods, there are several emulsion-based encapsulation variants (Munin and Edwards-Lévy, 2011; Markočič et al., 2012; Lu et al., 2016). Poly (lactic acid) (PLA) nanoparticles containing a polyphenol aureusidin with high antioxidant activity were prepared by an emulsification-solvent evaporation technique in which PLA and aureusidin were dissolved in acetone and injected into an aqueous solution of polyvinyl alcohol (PVA). The encapsulation efficiency of the final product ranged from 68 to 98% at a drug loading of 60% (Roussaki et al., 2014). In addition, methods based on ionic interactions have gained increasing interest over the years due to their simplicity. Ionotropic gelation is typically used to prepare sodium alginate beads by mixing the bioactive compound/drug solution and sodium alginate, which is then dripped into a solution of divalent ions (e.g., Ca2+) using a syringe. Upon the contact, ionic crosslinking occurs between the carboxylate groups of the guluronate groups (G-blocks) of the alginate backbone and the divalent ions, creating a hydrogel network (Burdick et al., 2005; Giri et al., 2016). A study was conducted for the preparation of alginate microspheres for the encapsulation of blueberry residues. After dissolving sodium alginate and blueberry residues in ultrapure water, they were dripped into a ZnCl2 solution, which aided in microencapsulation with a resulting encapsulation efficiency of up to 100%. However, phenolic dissolution measurements showed an almost immediate burst release that reached a plateau within the first 10 min (Bittencourt et al., 2018). Such high-diffusion rates through the porous alginate structure indicate certain limitations in its use for drug delivery, hence various fillers were added to improve the structure. In a study by Bušić et al., natural fillers such as whey proteins, cocoa powder, and carob powder were added to the alginate for encapsulation of polyphenols from dandelion (Taraxacum officinale L.). The fillers enabled higher retention of antioxidant capacity, high encapsulation yield, and prolonged release of polyphenols in simulated gastric fluids (SGF) and simulated intestinal fluids (SIF) (Bušić et al., 2018).
Another commonly used method of microencapsulation is molecular inclusion, which generally refers to cyclodextrins formed by enzymatic modification of starch. β-cyclodextrin and its derivatives are most commonly used as they are inexpensive, are not inclined to cause irritation, and are easy to prepare (Singh et al., 2019). Polyphenols from pomegranate fruit (Diamanti et al., 2017), tea (Song et al., 2021b), cornelian cherry (Cornus mas L.) (Popović et al., 2021), St. John’s wort (Hypericum perforatum) (Kalogeropoulos et al., 2010), olive leaf (Mourtzinos et al., 2007), etc. have been successfully encapsulated in β-cyclodextrins. Moreover, a study of olive leaf encapsulation, it was demonstrated that the aqueous solubility of polyphenolic content increased by more than 150% (Mourtzinos et al., 2007).
As mentioned above, many encapsulation methods have successfully preserved the stability of natural bioactive compounds while allowing their controlled release and ensuring high encapsulation yields. The choice of method depends on the active compound and encapsulation material, their properties, application, and cost. A detailed analysis of the obtained capsules is essential to determine their usefulness for the application or necessary improvements in the future.
Further characterization of the obtained implant coatings containing natural bioactive compounds can be divided into three important segments: 1) characterization for qualitative and/or quantitative analysis of the chemical composition, interactions, and morphology data, 2) in vitro release testing required for the optimization of controlled release formulations to achieve desired release kinetics of bioactive compounds, and 3) cell culture characterization. As mentioned above, the first segment includes chemical composition determination techniques such as secondary ion mass spectrometry (SIMS), X-ray photoelectron spectroscopy (XPS), or Fourier transform infrared spectroscopy (FTIR). Combining these with techniques for determining morphology, topography, and other surface-specific features, such as scanning electron microscopy (SEM), transmission electron microscopy (TEM), atomic force microscopy (AFM), 3D tomography, quartz crystal microbalance (QCM), adhesion and contact angles (CA) measurements, enables a comprehensive analysis of bioactive coatings and a better understanding of the correlation between the physiochemical properties of the coatings and the results of bioactive compound release and bioactivity. Considering the known antioxidant activity of natural extracts or isolated bioactive compounds, several conventional spectrophotometric methods can be employed to test antioxidativity, namely the 1,1′-diphenyl-2-picrylhydrazyl (DPPH) free radical scavenging assay, determination of total phenolic content using the Folin-Ciocalteu reagent, total flavonoid content, proanthocyanidins, etc. (Sultana et al., 2009). However, chemiluminescent probes, electrochemical sensors, spectroscopic, fluorescent-dependent, spectrophotometric, and chromatographic methods have been applied to detect reactive oxygen species (ROS) associated with oxidative stress generated at the cellular level in vivo and in vitro systems (Prasad et al., 2019). In vitro release testings of bioactive compounds are typically performed using one of the seven types of USP dissolution apparatuses or their variations (e.g., Franz diffusion cells) and provide as a result a cumulative percentage of released active compound in SBF detected by UV-Vis, high performance liquid chromatography (HPLC) or enzyme-linked immunosorbent assay (ELISA) over a selected period of time, which can be evaluated using known kinetic models (Kravanja and Finšgar, 2021). Depending on the type of bioactive compound and the prepared coating system, studies have reported successful controlled release of natural bioactive compounds has been reported in studies, lasting between 6 and 100 days (Ji et al., 2014; Shukla and Bhathena, 2015; Mohan et al., 2016; Banerjee and Bose, 2019; Sarkar and Bose, 2020). Lastly, cell culture characterization is useful for evaluating antimicrobial activity against selected microorganisms causing postoperative infections (Shukla and Bhathena, 2015), bioactivity (e.g., fluorescent microscopy to determine osteogenic differentiation of mesenchymal stem cells or osteoblast adhesion to the coatings) (Rožanc et al., 2021), and biocompatibility of the prepared coatings by testing cytotoxicity on healthy cells (e.g., WST-1 assay, MTT assay, etc.) (Felice et al., 2013; Sarkar and Bose, 2020).
Polyphenols are a promising alternative to synthetic drugs for reducing postoperative complications by incorporating them into bioactive coatings for orthopedic device implantation. This is due to their remarkable biological activity and abundant occurrence in natural sources from which they can be easily isolated using various extraction methods. However, before they can be used in clinical practice, several challenges must be addressed.
For example, polyphenols are inherently subjected to light and heat sensitivity, oxidation reactions, and poor solubility in body fluids. Therefore, the selected application requires careful consideration of the coating deposition technique, which should not operate under processing conditions that are hazardous to the bioactive compounds. To preserve their efficacy, encapsulation of bioactive compounds is a particularly convenient solution that simultaneously contributes to their localized controlled release from the prepared formulations by modifying their pharmacokinetics. While cellular viability data are already available for singular bioactive compounds, further studies surrounding the cytotoxicity of complex systems of multiple bioactive compounds in polyphenol-rich extracts are needed before they can be implemented in coatings. On this basis, appropriate characterization of the developed coatings is crucial as it can evaluate the relationship between interaction, morphology, release kinetics, and bioactivity.
Ongoing research has shown that applying natural bioactive compounds in coatings that stimulate osteogenesis in vitro and bone formation in vivo has been an initial success. Profound studies are still required to consider current limitations and to fabricate optimized medical implants that exhibit long-term osteointegration and prevent postoperative infection and inflammation.
MK, MF, and KK conceived and designed the review. KK has written and edited most of the manuscript. MM, ŽK and MF reviewed the manuscript. ŽK and MF are responsible for the financial part of the projects listed in fundings. All authors accepted the final version of the manuscript.
This activity was supported by the Slovenian Research Agency (ARRS) within the frame of program P2-0046 (Separation Processes and Production Design), project No. J2-1725 (Smart materials for bioapplications), project No. J2-3037 (Bionanotechnology as a tool for stabilization and applications of bioactive substances from natural sources), project No. J1-2470 (Biofunctionalization of 3D-Printed Metal Alloys as a Newly Emerging Strategy to Diminish Undesired Effects of Orthopedic Implants) and young researcher ARRS fellowship contract of KK.
The authors declare that the research was conducted in the absence of any commercial or financial relationships that could be construed as a potential conflict of interest.
All claims expressed in this article are solely those of the authors and do not necessarily represent those of their affiliated organizations, or those of the publisher, the editors and the reviewers. Any product that may be evaluated in this article, or claim that may be made by its manufacturer, is not guaranteed or endorsed by the publisher.
The authors would like to acknowledge Slovenian Research Agency (ARRS) for financing research and current and previous coworkers in research group.
Agrawal, M. (2015). Natural Polyphenols Based New Therapeutic Avenues for Advanced Biomedical Applications. Drug Metab. Rev. 47 (4), 420–430. doi:10.3109/03602532.2015.1102933
Aguilar, L., Lee, J., Park, C., and Kim, C. (2019). Biomedical Grade Stainless Steel Coating of Polycaffeic Acid via Combined Oxidative and Ultraviolet Light-Assisted Polymerization Process for Bioactive Implant Application. Polymers 11 (4), 584. doi:10.3390/polym11040584
Ahmadi, Z., Mohammadinejad, R., and Ashrafizadeh, M. (2019). Drug Delivery Systems for Resveratrol, a Non-flavonoid Polyphenol: Emerging Evidence in Last Decades. J. Drug Deliv. Sci. Tech. 51, 591–604. doi:10.1016/j.jddst.2019.03.017
Aktug, S. L., Durdu, S., Aktas, S., Yalcin, E., and Usta, M. (2019). Surface and In Vitro Properties of Ag-Deposited Antibacterial and Bioactive Coatings on AZ31 Mg alloy. Surf. Coat. Tech. 375, 46–53. doi:10.1016/j.surfcoat.2019.07.013
Asri, R. I. M., Harun, W. S. W., Hassan, M. A., Ghani, S. A. C., and Buyong, Z. (2016). A Review of Hydroxyapatite-Based Coating Techniques: Sol-Gel and Electrochemical Depositions on Biocompatible Metals. J. Mech. Behav. Biomed. Mater. 57, 95–108. doi:10.1016/j.jmbbm.2015.11.031
Babu, N. R., Manwatkar, S., Rao, K. P., and Kumar, T. S. (2004). Bioactive Coatings on 316L Stainless Steel Implants. Trends Biomater. Artif. Organs 17 (2), 43–47.
Bagherifard, S. (2017). Mediating Bone Regeneration by Means of Drug Eluting Implants: From Passive to Smart Strategies. Mater. Sci. Eng. C 71, 1241–1252. doi:10.1016/j.msec.2016.11.011
Banerjee, D., and Bose, S. (2019). Effects of Aloe Vera Gel Extract in Doped Hydroxyapatite-Coated Titanium Implants on In Vivo and In Vitro Biological Properties. ACS Appl. Bio Mater. 2 (8), 3194–3202. doi:10.1021/acsabm.9b00077
Belščak-Cvitanović, A., Stojanović, R., Manojlović, V., Komes, D., Cindrić, I. J., Nedović, V., et al. (2011). Encapsulation of Polyphenolic Antioxidants from Medicinal Plant Extracts in Alginate–Chitosan System Enhanced with Ascorbic Acid by Electrostatic Extrusion. Food Res. Int. 44 (4), 1094–1101.
Bittencourt, L. L. d. A., Silva, K. A., de Sousa, V. P., Fontes-Sant’Ana, G. C., and Rocha-Leão, M. H. (2018). Blueberry Residue Encapsulation by Ionotropic Gelation. Plant Foods Hum. Nutr. 73 (4), 278–286. doi:10.1007/s11130-018-0685-y
Bjelić, D., and Finšgar, M. (2022). Bioactive Coatings with Anti-osteoclast Therapeutic Agents for Bone Implants: Enhanced Compliance and Prolonged Implant Life. Pharmacol. Res. 176, 106060. doi:10.1016/j.phrs.2022.106060
Burdick, J. A., and Stevens, M. M. (2005). “11 - Biomedical Hydrogels,” in Biomaterials, Artificial Organs and Tissue Engineering. Editors L. L. Hench, and J. R. Jones (Woodhead Publishing), 107–115.
Bušić, A., Belščak-Cvitanović, A., Vojvodić Cebin, A., Karlović, S., Kovač, V., Špoljarić, I., et al. (2018). Structuring New Alginate Network Aimed for Delivery of Dandelion (Taraxacum officinale L.) Polyphenols Using Ionic Gelation and New Filler Materials. Food Res. Int. 111, 244–255. doi:10.1016/j.foodres.2018.05.034
Castro-Rosas, J., Ferreira-Grosso, C. R., Gómez-Aldapa, C. A., Rangel-Vargas, E., Rodríguez-Marín, M. L., Guzmán-Ortiz, F. A., et al. (2017). Recent Advances in Microencapsulation of Natural Sources of Antimicrobial Compounds Used in Food - A Review. Food Res. Int. 102, 575–587. doi:10.1016/j.foodres.2017.09.054
Cazzola, M., Ferraris, S., Boschetto, F., Rondinella, A., Marin, E., Zhu, W., et al. (2018). Green Tea Polyphenols Coupled with a Bioactive Titanium Alloy Surface: In Vitro Characterization of Osteoinductive Behavior through a KUSA A1 Cell Study. Ijms 19 (8), 2255. doi:10.3390/ijms19082255
Cazzola, M., Vernè, E., Cochis, A., Sorrentino, R., Azzimonti, B., Prenesti, E., et al. (2017). Bioactive Glasses Functionalized with Polyphenols: In Vitro Interactions with Healthy and Cancerous Osteoblast Cells. J. Mater. Sci. 52 (15), 9211–9223. doi:10.1007/s10853-017-0872-5
Chaumun, M., Goëlo, V., Ribeiro, A. M., Rocha, F., and Estevinho, B. N. (2020). In Vitro evaluation of Microparticles with Laurus Nobilis L. Extract Prepared by spray-drying for Application in Food and Pharmaceutical Products. Food Bioproducts Process. 122, 124–135. doi:10.1016/j.fbp.2020.04.011
Chen, S., Zhao, S., Chen, M., Zhang, X., Zhang, J., Li, X., et al. (2019). The Anticorrosion Mechanism of Phenolic Conversion Coating Applied on Magnesium Implants. Appl. Surf. Sci. 463, 953–967. doi:10.1016/j.apsusc.2018.08.261
Córdoba, A., Manzanaro-Moreno, N., Colom, C., Rønold, H. J., Lyngstadaas, S. P., Monjo, M., et al. (2018). Quercitrin Nanocoated Implant Surfaces Reduce Osteoclast Activity In Vitro and In Vivo. Int. J. Mol. Sci. 19 (11), 3319.
Córdoba, A., Satué, M., Gómez‐Florit, M., Hierro‐Oliva, M., Petzold, C., Lyngstadaas, S. P., et al. (2015). Flavonoid‐modified Surfaces: Multifunctional Bioactive Biomaterials with Osteopromotive, Anti‐inflammatory, and Anti‐fibrotic Potential. Adv. Healthc. Mater. 4 (4), 540–549.
de Araújo, F. F., de Paulo Farias, D., Neri-Numa, I. A., and Pastore, G. M. (2021). Polyphenols and Their Applications: An Approach in Food Chemistry and Innovation Potential. Food Chem. 338, 127535.
Diamanti, A. C., Igoumenidis, P. E., Mourtzinos, I., Yannakopoulou, K., and Karathanos, V. T. (2017). Green Extraction of Polyphenols from Whole Pomegranate Fruit Using Cyclodextrins. Food Chem. 214, 61–66. doi:10.1016/j.foodchem.2016.07.072
Ehlert, N., Badar, M., Christel, A., Lohmeier, S. J., Luessenhop, T., Stieve, M., et al. (2011). Mesoporous Silica Coatings for Controlled Release of the Antibiotic Ciprofloxacin from Implants. J. Mater. Chem. 21 (3), 752–760. doi:10.1039/c0jm01487g
Enaru, B., Socaci, S., Farcas, A., Socaciu, C., Danciu, C., Stanila, A., et al. (2021). Novel Delivery Systems of Polyphenols and Their Potential Health Benefits. Pharmaceuticals 14 (10), 946. doi:10.3390/ph14100946
Erakovic, S., Jankovic, A., Tsui, G., Tang, C.-Y., Miskovic-Stankovic, V., and Stevanovic, T. (2014). Novel Bioactive Antimicrobial Lignin Containing Coatings on Titanium Obtained by Electrophoretic Deposition. Ijms 15 (7), 12294–12322. doi:10.3390/ijms150712294
Fang, Z., and Bhandari, B. (2010). Encapsulation of Polyphenols - a Review. Trends Food Sci. Tech. 21 (10), 510–523. doi:10.1016/j.tifs.2010.08.003
Felice, F., Zambito, Y., Belardinelli, E., D'Onofrio, C., Fabiano, A., Balbarini, A., et al. (2013). Delivery of Natural Polyphenols by Polymeric Nanoparticles Improves the Resistance of Endothelial Progenitor Cells to Oxidative Stress. Eur. J. Pharm. Sci. 50 (3), 393–399. doi:10.1016/j.ejps.2013.08.008
Fraga, C. G., Galleano, M., Verstraeten, S. V., and Oteiza, P. I. (2010). Basic Biochemical Mechanisms behind the Health Benefits of Polyphenols. Mol. Aspects Med. 31 (6), 435–445. doi:10.1016/j.mam.2010.09.006
Fras Zemljič, L., Maver, U., Kraševac Glaser, T., Bren, U., Knez Hrnčič, M., Petek, G., et al. (2020). Electrospun Composite Nanofibrous Materials Based on (Poly)-Phenol-Polysaccharide Formulations for Potential Wound Treatment. Materials 13 (11), 2631.
Gamna, F., and Spriano, S. (2021). Vitamin E: A Review of its Application and Methods of Detection when Combined with Implant Biomaterials. Materials 14 (13), 3691. doi:10.3390/ma14133691
Gherasim, O., Grumezescu, A. M., Grumezescu, V., Negut, I., Dumitrescu, M. F., Stan, M. S., et al. (2021). Bioactive Coatings Based on Hydroxyapatite, Kanamycin, and Growth Factor for Biofilm Modulation. Antibiotics 10 (2), 160. doi:10.3390/antibiotics10020160
Giri, T. K. (2016). “20 - Alginate Containing Nanoarchitectonics for Improved Cancer Therapy,” in Nanoarchitectonics for Smart Delivery and Drug Targeting. Editors A. M. Holban, and A. M. Grumezescu (William Andrew Publishing), 565–588.
Goëlo, V., Chaumun, M., Gonçalves, A., Estevinho, B. N., and Rocha, F. (2020). Polysaccharide-based Delivery Systems for Curcumin and Turmeric Powder Encapsulation Using a spray-drying Process. Powder Tech. 370, 137–146.
Gomez-Florit, M., Pacha-Olivenza, M. A., Fernández-Calderón, M. C., Córdoba, A., González-Martín, M. L., Monjo, M., et al. (2016). Quercitrin-nanocoated Titanium Surfaces Favour Gingival Cells against Oral Bacteria. Sci. Rep. 6 (1), 22444. doi:10.1038/srep22444
González, E., Gómez-Caravaca, A. M., Giménez, B., Cebrián, R., Maqueda, M., Martínez-Férez, A., et al. (2019). Evolution of the Phenolic Compounds Profile of Olive Leaf Extract Encapsulated by spray-drying during In Vitro Gastrointestinal Digestion. Food Chem. 279, 40–48.
Gonçalves, V., Poejo, J., Matias, A., Rodríguez-Rojo, S., Cocero, M., and Duarte, C. (2016). Using Different Natural Origin Carriers for Development of Epigallocatechin Gallate (EGCG) Solid Formulations with Improved Antioxidant Activity by PGSS-Drying. RSC Adv. 6 (72), 67599–67609.
Gorzynik-Debicka, M., Przychodzen, P., Cappello, F., Kuban-Jankowska, A., Marino Gammazza, A., Knap, N., et al. (2018). Potential Health Benefits of Olive Oil and Plant Polyphenols. Ijms 19 (3), 686. doi:10.3390/ijms19030686
Gurzawska, K., Svava, R., Yihua, Y., Haugshøj, K. B., Dirscherl, K., Levery, S. B., et al. (2014). Osteoblastic Response to Pectin Nanocoating on Titanium Surfaces. Mater. Sci. Eng. C 43, 117–125. doi:10.1016/j.msec.2014.06.028
Ji, Y., Wang, L., Watts, D. C., Qiu, H., You, T., Deng, F., et al. (2014). Controlled-release Naringin Nanoscaffold for Osteoporotic Bone Healing. Dental Mater. 30 (11), 1263–1273. doi:10.1016/j.dental.2014.08.381
Kalogeropoulos, N., Yannakopoulou, K., Gioxari, A., Chiou, A., and Makris, D. P. (2010). Polyphenol Characterization and Encapsulation in β-cyclodextrin of a Flavonoid-Rich Hypericum perforatum (St John's Wort) Extract. LWT - Food Sci. Tech. 43 (6), 882–889. doi:10.1016/j.lwt.2010.01.016
Klettenhammer, S., Ferrentino, G., Morozova, K., and Scampicchio, M. (2020). Novel Technologies Based on Supercritical Fluids for the Encapsulation of Food Grade Bioactive Compounds. Foods 9 (10), 1395. doi:10.3390/foods9101395
Koju, N., Sikder, P., Ren, Y., Zhou, H., and Bhaduri, S. B. (2017). Biomimetic Coating Technology for Orthopedic Implants. Curr. Opin. Chem. Eng. 15, 49–55. doi:10.1016/j.coche.2016.11.005
Kravanja, G., Knez, Ž., Kotnik, P., Ljubec, B., and Knez Hrnčič, M. (2018). Formulation of Nimodipine, Fenofibrate, and O-Vanillin with Brij S100 and PEG 4000 Using the PGSS Process. J. Supercrit. Fluids 135, 245–253. doi:10.1016/j.supflu.2018.01.021
Kravanja, K. A., and Finšgar, M. (2021). Analytical Techniques for the Characterization of Bioactive Coatings for Orthopaedic Implants. Biomedicines 9 (12), 1936. doi:10.3390/biomedicines9121936
Levent, A., Suero, E. M., Gehrke, T., Bakhtiari, I. G., and Citak, M. (2021). Risk Factors for Aseptic Loosening in Complex Revision Total Knee Arthroplasty Using Rotating Hinge Implants. Int. Orthopaedics (Sicot) 45 (1), 125–132. doi:10.1007/s00264-020-04878-2
Lewandowska, U., Szewczyk, K., Hrabec, E., Janecka, A., and Gorlach, S. (2013). Overview of Metabolism and Bioavailability Enhancement of Polyphenols. J. Agric. Food Chem. 61 (50), 12183–12199. doi:10.1021/jf404439b
Li, L., Li, Y., Yang, L., Yu, F., Zhang, K., Jin, J., et al. (2019). Polydopamine Coating Promotes Early Osteogenesis in 3D Printing Porous Ti6Al4V Scaffolds. Ann. Transl Med. 7 (11), 240. doi:10.21037/atm.2019.04.79
Li, X., Shi, H., Cui, Y., Pan, K., Wei, W., and Liu, X. (2020). Dextran-caffeic Acid/tetraaniline Composite Coatings for Simultaneous Improvement of Cytocompatibility and Corrosion Resistance of Magnesium alloy. Prog. Org. Coat. 149, 105928. doi:10.1016/j.porgcoat.2020.105928
Li, Y., Wang, S., Li, S., and Fei, J. (2022). Clindamycin-loaded Titanium Prevents Implant-Related Infection through Blocking Biofilm Formation. J. Biomater. Appl. 36 (7), 1231–1242. doi:10.1177/08853282211051183
Lu, W., Kelly, A. L., and Miao, S. (2016). Emulsion-based Encapsulation and Delivery Systems for Polyphenols. Trends Food Sci. Tech. 47, 1–9. doi:10.1016/j.tifs.2015.10.015
Mahdavi, S. A., Jafari, S. M., Ghorbani, M., and Assadpoor, E. (2014). Spray-drying Microencapsulation of Anthocyanins by Natural Biopolymers: A Review. Drying Technol. 32 (5), 509–518. doi:10.1080/07373937.2013.839562
Markočič, E., Škerget, M., and Knez, Ž. (2012). Supercritical Fluid Technology as Sustainable Approach to Processing Polylactic Acid for Biomedical Applications, International Conference on Bio-Based Polymers and Composites. Hungary: Lake Balaton.
Maver, T., Kurečič, M., Pivec, T., Maver, U., Gradišnik, L., Gašparič, P., et al. (2020). Needleless Electrospun Carboxymethyl Cellulose/polyethylene Oxide Mats with Medicinal Plant Extracts for Advanced Wound Care Applications. Cellulose 27 (8), 4487–4508. doi:10.1007/s10570-020-03079-9
Maver, T., Mastnak, T., Mihelič, M., Maver, U., and Finšgar, M. (2021). Clindamycin-Based 3D-Printed and Electrospun Coatings for Treatment of Implant-Related Infections. Materials 14 (6), 1464. doi:10.3390/ma14061464
Mohan, L., Anandan, C., and Rajendran, N. (2016). Drug Release Characteristics of Quercetin-Loaded TiO 2 Nanotubes Coated with Chitosan. Int. J. Biol. Macromolecules 93, 1633–1638. doi:10.1016/j.ijbiomac.2016.04.034
Mourtzinos, I., Salta, F., Yannakopoulou, K., Chiou, A., and Karathanos, V. T. (2007). Encapsulation of Olive Leaf Extract in β-Cyclodextrin. J. Agric. Food Chem. 55 (20), 8088–8094. doi:10.1021/jf0709698
Munin, A., and Edwards-Lévy, F. (2011). Encapsulation of Natural Polyphenolic Compounds; a Review. Pharmaceutics 3 (4), 793–829. doi:10.3390/pharmaceutics3040793
Omar, S. A., Ballarre, J., Castro, Y., Martinez Campos, E., Schreiner, W., Durán, A., et al. (2020). 58S and 68S Sol-Gel Glass-like Bioactive Coatings for Enhancing the Implant Performance of AZ91D Magnesium alloy. Surf. Coat. Tech. 400, 126224. doi:10.1016/j.surfcoat.2020.126224
Parisi, O. I., Puoci, F., Restuccia, D., Farina, G., Iemma, F., and Picci, N. (2014). “Polyphenols and Their Formulations,” in Polyphenols in Human Health and Disease. Editors R. R. Watson, V. R. Preedy, and S. Zibadi (San Diego: Academic Press), 29–45. doi:10.1016/b978-0-12-398456-2.00004-9
Popović, B. M., Blagojević, B., Latković, D., Četojević-Simin, D., Kucharska, A. Z., Parisi, F., et al. (2021). A One Step Enhanced Extraction and Encapsulation System of Cornelian Cherry (Cornus Mas L.) Polyphenols and Iridoids with β-cyclodextrin. LWT 141, 110884.
Prasad, A., Pospíšil, P., and Tada, M. (2019). Editorial: Reactive Oxygen Species (ROS) Detection Methods in Biological System. Front. Physiol. 10, 1316. doi:10.3389/fphys.2019.01316
Riccucci, G., Cazzola, M., Ferraris, S., Gobbo, V. A., Guaita, M., and Spriano, S. (2021). Surface Functionalization of Ti6Al4V with an Extract of Polyphenols from Red Grape Pomace. Mater. Des. 206, 109776. doi:10.1016/j.matdes.2021.109776
Riccucci, G., Cazzola, M., Ferraris, S., Gobbo, V. A., Miola, M., Bosso, A., et al. (2022). Surface Functionalization of Bioactive Glasses and Hydroxyapatite with Polyphenols from Organic Red Grape Pomace. J. Am. Ceram. Soc. 105 (3), 1697–1710. doi:10.1111/jace.17849
Roussaki, M., Gaitanarou, A., Diamanti, P. C., Vouyiouka, S., Papaspyrides, C., Kefalas, P., et al. (2014). Encapsulation of the Natural Antioxidant Aureusidin in Biodegradable PLA Nanoparticles. Polym. Degrad. Stab. 108, 182–187. doi:10.1016/j.polymdegradstab.2014.08.004
Rožanc, J., Žižek, M., Milojević, M., Maver, U., and Finšgar, M. (2021). Dexamethasone-Loaded Bioactive Coatings on Medical Grade Stainless Steel Promote Osteointegration. Pharmaceutics 13 (4), 568. doi:10.3390/pharmaceutics13040568
Sarkar, N., and Bose, S. (2020). Controlled Delivery of Curcumin and Vitamin K2 from Hydroxyapatite-Coated Titanium Implant for Enhanced In Vitro Chemoprevention, Osteogenesis, and In Vivo Osseointegration. ACS Appl. Mater. Inter. 12 (12), 13644–13656. doi:10.1021/acsami.9b22474
Shukla, V., and Bhathena, Z. (2015). Sustained Release of a Purified Tannin Component of Terminalia Chebula from a Titanium Implant Surface Prevents Biofilm Formation by Staphylococcus aureus. Appl. Biochem. Biotechnol. 175 (7), 3542–3556. doi:10.1007/s12010-015-1525-2
Singh, N., and Sahu, O. (2019). “4 - Sustainable Cyclodextrin in Textile Applications,” in The Impact and Prospects of Green Chemistry for Textile Technology. Editors I. Shahid ul, and B. S. Butola (Woodhead Publishing), 83–105.
Sonawane, S., Bhanvase, B. A., and Sivakumar, M. (2020). Encapsulation of Active Molecules and Their Delivery System. Elsevier.
Song, J., Feng, H., Wu, M., Chen, L., Xia, W., and Zhang, W. (2021). Development of a Bioactive Chitosan HPMC-Based Membrane with tea Polyphenols Encapsulated in β-cyclodextrin as an Effective Enhancement. Mater. Today Commun. 27, 102324. doi:10.1016/j.mtcomm.2021.102324
Song, Q., Zhu, M., and Mao, Y. (2021). Chemical Vapor Deposited Polyelectrolyte Coatings with Osteoconductive and Osteoinductive Activities. Surf. Coat. Tech. 423, 127522. doi:10.1016/j.surfcoat.2021.127522
Sultana, B., Anwar, F., and Ashraf, M. (2009). Effect of Extraction Solvent/Technique on the Antioxidant Activity of Selected Medicinal Plant Extracts. Molecules 14 (6), 2167–2180. doi:10.3390/molecules14062167
Sun-Waterhouse, D., Wadhwa, S. S., and Waterhouse, G. I. N. (2013). Spray-drying Microencapsulation of Polyphenol Bioactives: A Comparative Study Using Different Natural Fibre Polymers as Encapsulants. Food Bioproc. Technol 6 (9), 2376–2388. doi:10.1007/s11947-012-0946-y
Tong, S., Sun, X., Wu, A., Guo, S., and Zhang, H. (2021). Improved Biocompatibility of TiO2 Nanotubes via Co-precipitation Loading with Hydroxyapatite and Gentamicin. Coatings 11 (10), 1191. doi:10.3390/coatings11101191
Wang, T., Wu, C., Fan, G., Li, T., Gong, H., and Cao, F. (2018). Ginkgo Biloba Extracts-Loaded Starch Nano-Spheres: Preparation, Characterization, and In Vitro Release Kinetics. Int. J. Biol. Macromolecules 106, 148–157. doi:10.1016/j.ijbiomac.2017.08.012
Weidner, E., Knez, Ž., and Novak, Z. (2004). Process for Preparing Particles or Powders : JP3510262 (B2). Zurich: EPO–0322.
Wildman, R. E., Wildman, R., and Wallace, T. C. (2016). Handbook of Nutraceuticals and Functional Foods. CRC Press.
Yang, S., Wang, Y., Luo, S., Shan, C., Geng, Y., Zhang, T., et al. (2019). Building Polyphenol and Gelatin Films as Implant Coating, Evaluating from In Vitro and In Vivo Performances. Colloids Surf. B: Biointerfaces 181, 549–560. doi:10.1016/j.colsurfb.2019.05.058
Youn, Y. H., Lee, S. J., Choi, G. R., Lee, H. R., Lee, D., Heo, D. N., et al. (2019). Simple and Facile Preparation of Recombinant Human Bone Morphogenetic Protein-2 Immobilized Titanium Implant via Initiated Chemical Vapor Deposition Technique to Promote Osteogenesis for Bone Tissue Engineering Application. Mater. Sci. Eng. C 100, 949–958. doi:10.1016/j.msec.2019.03.048
Keywords: polyphenols, bioactive coatings, orthopedic implants, deposition techniques, encapsulation, characterization
Citation: Kravanja KA, Finšgar M, Knez Ž and Knez Marevci M (2022) Evaluation of Natural Extracts as Promising Components of Bioactive Coatings for Orthopedic Implants. Front. Mater. 9:878176. doi: 10.3389/fmats.2022.878176
Received: 17 February 2022; Accepted: 08 March 2022;
Published: 08 April 2022.
Edited by:
Changchun Zhou, Sichuan University, ChinaReviewed by:
Silvia Spriano, Politecnico di Torino, ItalyCopyright © 2022 Kravanja, Finšgar, Knez and Knez Marevci. This is an open-access article distributed under the terms of the Creative Commons Attribution License (CC BY). The use, distribution or reproduction in other forums is permitted, provided the original author(s) and the copyright owner(s) are credited and that the original publication in this journal is cited, in accordance with accepted academic practice. No use, distribution or reproduction is permitted which does not comply with these terms.
*Correspondence: Maša Knez Marevci, bWFzYS5rbmV6QHVtLnNp
Disclaimer: All claims expressed in this article are solely those of the authors and do not necessarily represent those of their affiliated organizations, or those of the publisher, the editors and the reviewers. Any product that may be evaluated in this article or claim that may be made by its manufacturer is not guaranteed or endorsed by the publisher.
Research integrity at Frontiers
Learn more about the work of our research integrity team to safeguard the quality of each article we publish.