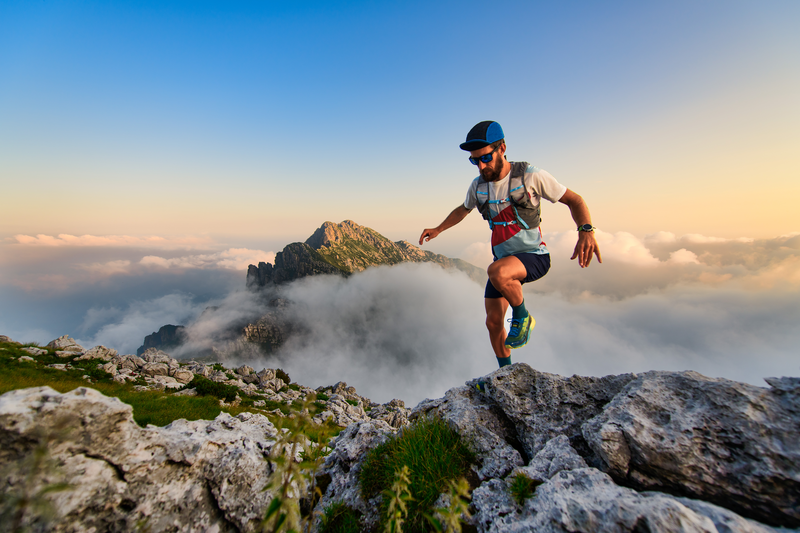
95% of researchers rate our articles as excellent or good
Learn more about the work of our research integrity team to safeguard the quality of each article we publish.
Find out more
ORIGINAL RESEARCH article
Front. Mater. , 29 March 2022
Sec. Polymeric and Composite Materials
Volume 9 - 2022 | https://doi.org/10.3389/fmats.2022.874735
This article is part of the Research Topic Metal-Organic Framework/Polymer Hybrid Composites: Synthesis Strategies, Characterization, and Applications for Energy and Environment View all 8 articles
Polyorthochloroaniline (POC) nanocomposites with montmorillonite (MMT) clay (POC/MMT) nanocomposites (POC/MMT clay NCs) are the most widespread and attractive among organic/inorganic nanocomposites due to their distinctive properties in addition to inexpensive, abundance, and attractive characteristics such as ion-exchange and great surface area properties and have ample applications. The preparation of polyorthochloroaniline nanocomposites with montmorillonite (POC/MMT) clay nanocomposite has been completed chemically with the help of oxidative polymerization of orthochloroaniline in the presence of montmorillonite (MMT) clay. POC will be admixed with montmorillonite (MMT) clay at various concentrations, by keeping the o-chloroaniline monomer in the reaction mixture constant. To investigate the influence of MMT clay on the characteristics of POC, the load percentage of MMT clay in the nanocomposites was adjusted. The characterization and properties have been determined by using different techniques. FTIR spectroscopy was used to investigate the interactions between POC and MMT. The complete homogeneous dispersion of MMT clay in the POC matrix was examined by scanning electron microscope (SEM) analysis that revealed the compactness. The thermal stability of polymer–clay nanocomposites was determined by thermogravimetric analysis (TGA). The results of DSC/TGA measurements revealed that the flame retardancy and thermal stability of POC/MMT clay nanocomposites were considerably improved.
1 The load percentage of MMT clay in the nanocomposites had changed the properties of polyorthochloroaniline.
2 The percentage of residue increased gradually by increasing the amount of MMT clay.
3 Because of the MMT nanofiller, adding MMT clay to the polymer improved thermal stability.
Nanocomposite technology provides a malleable forum for the investigation of novel nanomaterials with the intention of unique characteristics and different purposes for advanced applications and developments (Mishra and Militky, 2019). The progress and development in the science and technology field are at their peak. Human imagination and dreams frequently conduce to the new science and technological development. The outset of new technologies generally effectuates new demands. Clay minerals are ample (Chang et al., 2019), and said phyllosilicates belong to a family of inorganic layered nanomaterials (Mousa et al., 2018). Due to their complex and handy structures, they can be assembled again congruously by ion exchange that has a high economic value and a vast range of applications (Chang et al., 2019; Cankaya et al., 2020). MMT clay is a well-liked runner in polymer nanocomposites as a reinforcing nanofiller due to the presence of outstanding properties such as nontoxic, lightweight, inexpensive, large surface-to-volume ratio, economic competitiveness, lofty stiffness, and high aspect ratio (Xu et al., 2019). Conjugated polymer composites are intriguing because they have the ability to combine features that are difficult to achieve with individual components.
POC/MMT clay nanocomposites, among organic/inorganic nanocomposites, are the most widespread and attractive due to their distinctive properties in addition to inexpensive, abundance, and attractive characteristics such as ion exchange and great surface area properties and have ample applications (Linganathan and Samuel, 2014a). Montmorillonite fillers at nanoscale show signs of large surface-to-volume ratio, so the thermal, electrical, dielectric, optical, and thermal properties of polymers are modified significantly (Linganathan et al., 2014). When nanoscale fillers are used along with polymers, then the interaction is awfully strong among polymer chains and nanofiller surfaces due to expanding the surface area-to-volume ratio of nanofillers. As the polymer–clay particle interface area is improved, polymer chains can be forced to wrap firmly upon the nanofiller, and an interphase layer is created. This interphase layer is responsible for the novel and fabulous properties demonstrated by the polymer nanocomposites (Choi et al., 2015). The orthochloroaniline is a substituted derivative of aniline that has the chloro (–Cl) group substituted at the ortho position. Poly (o-chloroaniline) shows some unique and interesting properties. The POC polymer/MMT clay nanocomposite is novel and unique because superlative properties of MMT clay and polymer POC are combined to open new doors for researchers (Rubilar et al., 2013). In the literature, various polymer nanocomposites are reported in which poly (o-chloroaniline) has been used (Padmaja and Samuel, 2020). Some of them are POC/SiO2 (Gok and Sen, 2006), POC/red mud (RM) (Gok and Oguz, 2006), POC/CuS (Saeed et al., 2014), POC/MMT clay, POC/Na-bentonite (Linganathan and Samuel, 2014b), POC/Cu (Ahmad et al., 2017), and POC/Cr (Ahmad M. N. et al., 2018). The objective of this research work was to prepare nanocomposites with the help of chemical polymerization, characterized by different techniques, and study the properties of poly (o-chloroaniline)/MMT clay nanocomposites.
Montmorillonite (MMT) clay, 4,4 diaminodiphenylamine (DDPA), ethanol, ammonium persulfate (APS), orthochloroaniline, and distilled water were used in this study. All the chemicals were purchased with perfect analytical grades and utilized without further purifications.
The chemical oxidative polymerization method was applied to synthesize poly (o-chloroaniline). In this process, the orthochloroaniline monomer was polymerized. For this purpose, two solutions X and Y were prepared. Solution X was prepared by mixing 0.255 g of orthochloroaniline/2-chlroaniline (monomer) in 20 ml of 1 M HCl solution, and then 0.0255 g of DDPA was added into solution X. It was 10% of the weight of the monomer. After that, solution Y was formed by mixing 0.456 g of (NH4)2S2O8 (ammonium persulphate) into 20 ml of 1 M HCl. The ratio of (NH4)2S2O8 to o-chlroaniline monomer was the same (1:1). Solution X was put onto magnetic stirring in chilled (ice) water, and the temperature of chilled water was maintained; then, solution Y was added dropwise, and continuous agitation of the mixture X was performed for 3 h. With time, the solution was altered to bluish-green color, then agitating was stopped, and the solution remained without interruption for a certain period. At 4,000 arpm, the solution was centrifuged and rinsed with 1 M hydrochloric acid (HCl) after that by distilled H2O, land at the end by ethanol. The resultant amount was spread out in a Petri dish and kept in a microwave at 80–100°C overnight. Then, the dried sample was collected in a powder form (Figures 1, 2).
Nanocomposites of poly (o-chloroaniline)/MMT clay were synthesized according to a similar procedure as those implemented for poly (o-chloroaniline) polymerization. Montmorillonite (MMT) clay was added to the monomer according to the desired percentage such as 1, 3, 5, 7, and 10% at the time of polymerization. These percentages were made according to the weight of 2-chloroaniline monomer. The monomer (orthochloroaniline) was dissolved in 1 M HCl, then DDPA was added, and after that MMT clay was dissolved in this reaction mixture and sonicated for 15 min to get a homogeneous mixture. After this, the solution was mixed with the help of a magnetic stirrer constantly. Another solution was prepared by dissolving APS into 20 ml of 1 M HCl and then this solution was added into the first solution dropwise as an oxidant; then, the mixture was kept on magnetic stirring for almost 3 h. The temperature was upheld during the polymerization at 0–5°C. The bluish-green color formation has demonstrated the preparation of the reaction as the final product. By following the aforementioned process, all the nanocomposites were formed by changing (1, 3, 5, 7, and 10%) the amounts of filler MMT clay. At last, the mixture was centrifuged and rinsed with distilled water then ethanol. The resultant amounts were spread out in a Petri plate and put in a microwave at 80–100°C overnight. Then, the dried samples were collected in a powder form and characterized.
Characterization of the nanoparticles and nanocomposites was set up by using Fourier transform infrared (FTIR) spectroscopy, UV–visible spectroscopy, TGA, and scanning electron microscopy (SEM). A variety of analytical instruments were used to characterize the structure, properties, and morphology of POC and POC/MMT clay nanocomposites. Ultraviolet–visible spectra were found using a UV–visible spectrophotometer (Varian Cary 50) in the scanning range of 200–800 nm at the scanning rate of 400 nm/min. The spectrophotometer was set with UV Winlab software to record and evaluate the data. The baseline line correction was produced by distilled water in the form of a blank reference. The scanning electron microscopic images were found by using Hitachi S-4700 operating at 30 kV. FTIR spectra were reported using a FTIR spectrometer (Impact 400, Nicolet, Waltham, and MA) by the KBr pellet technique in the range of 400–4,000 cm−1. The thermogravimetric characterization of nanocomposites and the organoclay were performed on a SDT Q600 V20.9 Build 20. The samples have an average mass of 2 mg and were subjected to heating up to 700°C at a heating rate of 10°C/min under nitrogen atmosphere.
The formation of poly (o-chloroaniline) was carried out using a chemical-oxidative polymerization procedure. (NH4)2S2O8 behaved like an oxidant. After that, poly (o-chloroaniline)/MMT nanocomposites were amalgamated by varying the percentage of MMT clay (filler). The FTIR technique was used to analyze POC/MMT clay nanocomposites, and spectra were obtained. The FTIR technique was applied to investigate the polymer that exhibited the vibration manners in polymer and polymer-based nanocomposite samples (Ahmad MN. et al., 2018). All the functional groups have been known with the help of FTIR analysis (Kalotra and Mehta, 2020). The FTIR spectrum of polymer and polymer-based nanocomposites was reported, and the position of emission and absorption bands was notified. At 1,200 cm−1 and 1,500 cm−1, the most prominent bands of the benzene ring were shown. The presence of both these bands is a clear indication of the formation of polymer and polymer nanocomposites. In the pure spectrum of POC, the strongest bands at 1,581 cm−1 and 1,515 cm−1 indicate the existence of a quinonoid ring and benzenoid ring in the polymer. The peak observed above 3,076 cm−1 indicates the stretching vibrations of the N-H group, and the stretching vibrational peak of aromatic C-N was there at 1,305 cm−1.
The chloro group located at the ortho position on the phenyl ring in the polymer was specified by the signal at 748 cm−1, and two important peaks appeared at 918 cm−1 and 833 cm−1 in the spectrum due to out of plane bending vibrations of –CH in 1-, 2-, 4-trisubstituted aromatic rings (Ahmad M. N. et al., 2018). By the addition of the load percentage of MMT clay filler into the polymer POC, the characteristics bands appeared due to MMT clay exposed at 1,038 cm−1 by Si–O–Si relation and ∼800 cm−1 due to the vibrational stretching of Al4+ tetrahedra in clay–polymer nanocomposites. It proves the maintenance of the fundamental clay structure (Linganathan and Samuel 2014a). The broad absorption bands at 1,350–750 cm−1 have four Si-O stretching modes of MMT clay (Kazim et al., 2012). In MMT clay nanofiller in the polymer-based nanocomposites, the peaks at 2,350–2,250 cm−1 showed the coupling of MMT clay nanofiller with POC (Figure 3). The frequency of -NH stretching of poly (o-chloroaniline) is studied at 3,035 cm−1 (Linganathan and Samuel 2014b).
The –NH stretching frequency which appears at 3,076 cm−1 in pure POC is shifted to the low wavenumber in the spectra of composites which verified that there is contact between POC and nanofiller chains (Linganathan and Samuel 2014a). The value at 744 cm−1 was because of the –Cl group joined to the benzyl ring at the ortho position (Linganathan and Samuel 2014b). By the addition of 3% MMT clay filler into the polymer-based composite, the crystallinity of the polymer is enhanced. The spectrum showed that by adding 3% MMT clay nanofiller in the POC, the peak at 2,348 cm−1 showed the coupling of MMT clay nanofiller with POC. The characteristic point of phenyl C-N stretching mode appeared at 1,297 cm−1, and the spectrum values at 810 cm−1 and 743 cm−1 were for C-H out-of-plane bending vibrations of 1, 2, 4 trisubstituted aryl rings (Linganathan and Samuel 2014a). When 5% MMT clay nanofiller was added into the polymer, the bands were moved toward lower values and became broader and deeper (Ahmad MN. et al., 2018). FTIR spectra of 7% MMT clay added in poly (o-chloroaniline) prepared by the chemical-oxidation method, the strongest bands at 1,573 cm−1 and 1,494 cm−1 indicate the existence of the quinonoid ring and benzenoid ring in the poly (o-chloroaniline). The characteristic point of phenyl–CN stretching mode appeared at 1,294 cm−1 (Figure 4). By increasing the load percentage by 1% toward 10% increased the reactivity and enhanced the crystallinity of the polymer. The characteristic point of phenyl C-N stretching mode appeared at 1,297 cm−1 (Ahmad et al., 2017). The formation of POC/MMT clay nanocomposites was proved by the deviations and as well as illustrated the coordinate covalent bond configuration between nanofiller and polymer chains (Ahmad et al., 2017).
Ultraviolet–visible absorption of POC/MMT clay nanocomposites was put down in the spectral range of 200–800 nm on the UV spectrophotometer using unionized water in terms of reference solvent. Ultraviolet spectroscopy revealed that POC and nanocomposites of POC/MMT clay absorbed more than 90% of incident UV light (Ahmad et al., 2017). The UV–Vis spectra of poly (o-chloroaniline) and POC–MMT nanocomposites with various load percentages (0, 1, 3, 5, 7, and 10%) of MMT clay were reported. It was cleared from the pure polymer spectrum that the absorption peak at 230 nm demonstrated π–π* transition by the benzene ring and other 283 nm because of n–π* transition of the quinonoid ring. It was illustrated that they slowly enhance the intensities of the peaks by increasing the amount of nanofiller. It was showed that pure polymer (POC) demonstrated absorption at a lower wavelength than poly (o-chloroaniline)/MMT nanocomposites and showed redshift in poly (o-chloroaniline)-MMT clay nanocomposite, and the presence of MMT clay in POC was implanted. By increasing the mass of MMT clay, n–π* absorption bands become broad. This demonstrated some sort of physical interaction between polymer chains and nanofiller (Ahmad et al., 2017). The first absorption band of π–π* has appeared at 243–245 nm is given to the π–π* transition of the benzenoid ring based on earlier studies on POC–MMT clay nanocomposites (Ibrahim, 2017) because of the excitation of nitrogen in the benzenoid segments π–π* transition (Gok and Sen, 2006).
The graph demonstrated UV–vis spectra of 1% MMT clay added in poly (o-chloroaniline) formed by the chemical oxidation process; the band at 245 nm showed π–π* transition for the benzene ring, and it gradually shifted toward 293 nm in POC/MMT clay. The graph showed the UV–vis spectrum of 3% MMT into POC formed by chemical oxidation method; the band at 245 nm demonstrated π–π* transition for the benzene ring that shifted toward 285 and 339 nm range. The graph revealed 5% MMT-clay spectrum of polymer–clay nanocomposite by UV–vis analysis, and the same band at 245 nm showed π–π* transition due to the benzenoid molecule steadily transferred toward n–π* at 293 and 380 nm range. The Graph opened the mystery of POC/MMT clay nanocomposite which has 7% clay by using the UV–vis technique, and the high band showed at 245 nm (π–π* transition) turned toward n–π* at 293 and 332 nm range (Figure 5). An increase in the percentage of MMT clay into the POC polymer nanocomposite showed higher bands, and the intensities of these bands were also increased. The most prominent bands have been shown by UV–vis spectrum in 10% loading amount present in POC at 245 nm toward 293 nm and then slightly increased band at 385 nm (Ahmad M. N. et al., 2018).
FIGURE 5. UV–vis of (A) pure POC, (B) 1% POC/MMT, (C) 3% POC/MMT, (D) 5% POC/MMT, (E) 7% POC/MMT, and (F) 10% POC/MMT.
SEM surface analysis of pure POC and POC-MMT nanocomposites by changing the percentages of clay (5% and 10%) is shown in Figure 6. Poly (o-chloroaniline) exhibits a compact structure as it is evident with the help of the SEM image of POC. POC/MMT clay nanocomposites illustrate this compactness of polymer, but these have the appearance of grains in the image. The aggregation of POC/MMT nanocomposites becomes very clear by increasing the load % of MMT clay added to the polymer. The SEM-magnified micrograph image of pure POC and POC–MMT nanocomposites of 500 µm was used to clarify the compactness and composition of samples. The POC–MMT nanocomposite with 5% nanoclay exhibited uniform dispersion with POC (Ahmad MN. et al., 2018). The compatibility between the POC polymer and MMT clay nanofiller has an enormous impact on the properties of POC/MMT clay nanocomposites (Narayanasamy et al., 2021). By increasing the percentage load between 5–10%, more prominent and attached nanocomposites were observed to the surface of poly (o-chloroaniline) that was granular. The SEM micrographs revealed that MMT clay was entrenched so deeply into POC (polymer) (Ahmad M. N. et al., 2018).
The thermal stability of the polymers under investigation is checked using TGA/DSC (Abd El-Salam et al., 2020). Thermal stability is a vital characteristic in favor of which the morphology of the nanocomposites performs an imperative role (Xiao et al., 2005). Two different types of analysis were performed for determining the thermal stability of nanocomposites (Mishra and Militky, 2019). TGA and DSC are complementary thermal analysis methods that are frequently employed mutually to get the utmost information on the oxidation or combustion reactions (Sarathi, 1999). The thermal properties of pure POC and its nanocomposites (POC/MMT) are examined with the help of thermogravimetric analysis (Xiao et al., 2005). TGA (thermogravimetric analysis) is used to study the degradation of polymeric materials in many cases that observed the loss of weight of the product as a function of time, especially temperature. The thermal stability can be improved by the addition of nanofiller clay into the polymer matrix. It signifies a prospect to achieve considerable enhancement in the thermal stability by using only a little amount of MMT clay in the final product that is extremely elegant and important in marketable reasons because the products can be prepared cheaper, using easy process, and are lightweight (Mattausch, 2015). In comparison with the consequent pure POC, the results of DSC/TGA measurements revealed that the flame retardancy and thermal stability of POC/MMT clay nanocomposites were considerably improved because of the occurrence of dispersed MMT clay in the polymer matrix.
The thermogram of pure POC and POC/MMT nanocomposites revealed that the first weight loss occurs due to the removal of H2O molecules at 50°C and continuously up to 110°C. The next degradation temperature at 200 oC marked the loss of HCl (Kongkaew et al., 2018), and then the rapid mass loss occurs till 620°C. The decomposition of poly (o-chloroaniline) is continuous up to 700°C (Linganathan et al., 2014) (Figure 7). This thermogravimetric analysis indicates better thermal stability of the POC nanocomposites than that of pure poly (o-chloroaniline). It comes into view that the constancy and strength of the inserted polymer are due to not only its specific structure but also the steric factors that inhibit the thermal motion of the sections sandwiched between MMT clay layers (Nikolic et al., 2012). The enhanced thermal stability of nanocomposites can be clarified by the supremacy of the benzenoid structure. On the other hand, the low-grade thermal stability of pure poly (o-chloroaniline) is because of the existence of a quinonoid ring in its structure (Gupta et al., 2010). Poly (o-chloroaniline) is continuously decomposed up to 700°C, but in the case of POC/MMT clay nanocomposites, the complete decomposition has not taken place ever after 700°C. Some amount of char is seen in the decomposition of POC and POC/MMT nanocomposites. This indicates that the existence of -Cl has avoided the entire decomposition. By comparing poly (o-chloroaniline) and poly (o-chloroaniline)/MMT clay nanocomposites with different mass wt. demonstrates lower weight loss. Poly (o-chloroaniline) has 50% residue, but poly (o-chloroaniline)/MMT clay nanocomposites with 1 and 5% have 53 and 56% residues, respectively. In this present work, MMT clay nanofiller enhanced the thermal stability of POC nanocomposites. When the temperature is raised, the POC polymer turned to decompose state but adding the MMT clay into the polymer does not allow decomposition because of the high thermal stability of the MMT nanofiller. The percentage of residue increased gradually by increasing the amount of MMT clay (Linganathan et al., 2014).
In the present research work, poly (o-chloroaniline) was synthesized by the chemical oxidative polymerization of o-chloroaniline in the first step. In the second step, polymer–clay nanocomposites were prepared by a chemical oxidative polymerization process with the existence of MMT-clay. O-Chloroaniline was polymerized by using ammonium persulphate that behaves like oxidants, and distilled water was used as a solvent. Before starting the polymerization process, nanoclay was dispersed to the monomer and then the mixture was kept on the magnetic stirring to be constantly stirred for 3 h at low temperature, and POC/MMT nanocomposite samples were synthesized in the same way and changed the load percentage (1, 3, 5, 7, and 10%) of MMT clay into the POC polymer. MMT clay nanofiller in the polymer-based nanocomposites showed the peaks at 2,350–2,250 cm−1, thus showing the coupling of MMT clay nanofiller with POC. The graph opened the mystery of POC/MMT clay nanocomposite which has 7% clay using the UV–vis technique, and the high band showed at 245 nm (π-π* transition) turned toward n–π* at 293 and 332 nm range. By increasing the percentage load ranging between 5–10%, then more prominent and attached nanocomposites were observed to the surface of poly (o-chloroaniline) that was granular. When the temperature is raised, the POC polymer turned to decompose state but adding the MMT clay into the polymer does not allow decomposition because of the high thermal stability of the MMT nanofiller. The percentage of residue increased gradually by increasing the amount of MMT clay.
The original contributions presented in the study are included in the article/Supplementary Material, further inquiries can be directed to the corresponding author.
SI: Interpret the data, performed major experimental works, writing-original draft preparation and editing. SN: Conception, design of the study, writing-original draft preparation. MNA: Visualization of data, reviewed the original manuscript. MJ: Conception, design of the study, acquisition of data, interpret the data. HA: Reviewed original manuscript, and critical revision. NSA: Visualization of data, EDX analysis, writing reviewing and editing. HI: Conception, visualization of data, performed EDX analysis, acquisition of data. EE: Financial funding, reviewing of data. FA: Interpret the data, and critical revision. NA: Reviewed original manuscript, and critical revision. MIAlahmdi: Visualization of data, and critical revision. AB: Visualization of data, reviewed the original manuscript and critical revision. SH: Interpret the data, writing-original draft preparation. AM: Conception, design of the study, acquisition of data.
The authors declare that the research was conducted in the absence of any commercial or financial relationships that could be construed as a potential conflict of interest.
All claims expressed in this article are solely those of the authors and do not necessarily represent those of their affiliated organizations, or those of the publisher, the editors, and the reviewers. Any product that may be evaluated in this article, or claim that may be made by its manufacturer, is not guaranteed or endorsed by the publisher.
The authors extend their appreciation to the Deanship of Scientific Research at King Khalid University for supporting this work through research groups program under grant number R.G.P.2/107/42. The authors extend their appreciation to the Research Center at AlMaarefa University for funding this work under TUMA project agreement number (TUMA-2021-4).
Abd El-Salam, H. M., Abd El-Hafez, G. M., Askalany, H. G., and Fekry, A. M. (2020). A Creation of Poly (N-2-Hydroxyethylaniline-Co-2-Chloroaniline) for Corrosion Control of Mild Steel in Acidic Medium. J. Bio-and Tribo-Corrosion 6 (2), 1–14. doi:10.1007/s40735-020-00351-0
Ahmad, M. N., Rafique, F., Nawaz, F., Farooq, T., Anjum, M. N., Hussain, T., et al. (2018b). Synthesis of Antibacterial Poly(o-Chloroaniline)/chromium Hybrid Composites with Enhanced Electrical Conductivity. Chem. Cent. J. 12, 46–47. doi:10.1186/s13065-018-0416-3
Ahmad, M. N., Anjum, M. N., Nawaz, F., Iqbal, S., Saif, M. J., Hussain, T., et al. (2018a). Synthesis and Antibacterial Potential of Hybrid Nanocomposites Based on Polyorthochloroaniline/copper Nanofiller. Polym. Compos. 39 (12), 4524–4531. doi:10.1002/pc.24558
Cankaya, N. (2020). Preparation of Oxo Methacrylate-Containing Polymer/Clay Based Nanocomposites. Int. Transaction J. Eng. Manag. Appl. Sciences&Technologies 11 (7), 1–8. doi:10.14456/ITJEMAST.2020.128
Chang, P.-H., Li, Z., Jiang, W.-T., and Sarkar, B. (2019). “Clay Minerals for Pharmaceutical Wastewater Treatment,” in Modified Clay and Zeolite Nanocomposite Materials (Amsterdam: Elsevier), 167–196. doi:10.1016/b978-0-12-814617-0.00011-6
Choi, Y., and Chan, A. P. (2015). PROVEAN Web Server: A Tool To Predict The Functional Effect of Amino Acid Substitutions And Indels. Bioinformatics 31, 2745–2747.
Gök, A., and Oğuz, İ. (2006). Structural and thermal Characterization of Poly(2-Chloroaniline)/red Mud Nanocomposite Materials. J. Appl. Polym. Sci. 99 (5), 2101–2108. doi:10.1002/app.22740
Gök, A., and Şen, S. (2006). Preparation and Characterization of Poly(2-chloroaniline)/SiO2 Nanocomposite via Oxidative Polymerization: Comparative UV-Vis Studies into Different Solvents of Poly(2-Chloroaniline) and Poly(2-chloroaniline)/SiO2. J. Appl. Polym. Sci. 102 (1), 935–943. doi:10.1002/app.24266
Gupta, A. S., van der Meer, M. A., Touretzky, D. S., and Redish, A. D. J. N. (2010). Hippocampal Replay is Not A Simple Function of Experience. 65, 695–705.
Ibrahim, K. A. (2017). Synthesis and Characterization of Polyaniline and Poly(aniline-Co-O-Nitroaniline) Using Vibrational Spectroscopy. Arabian J. Chem. 10, S2668–S2674. doi:10.1016/j.arabjc.2013.10.010
Kalotra, S., and Mehta, R. (2020). Synthesis of Polyaniline/clay Nanocomposites by In Situ Polymerization and its Application for the Removal of Acid Green 25 Dye from Wastewater. Polym. Bull. 78, 2439–2463. doi:10.1007/s00289-020-03222-3
Kazim, S., Ahmad, S., Pfleger, J., Plestil, J., and Joshi, Y. M. (2012). Polyaniline-sodium Montmorillonite clay Nanocomposites: Effect of clay Concentration on thermal, Structural, and Electrical Properties. J. Mater. Sci. 47 (1), 420–428. doi:10.1007/s10853-011-5815-y
Kongkaew, W., Sangwan, W., Prissanaroon-Ouajai, W., and Sirivat, A. (2018). Synthesis and Characterization of Poly(2-Chloroaniline) by Chemical Oxidative Polymerization. Chem. Pap. 72 (4), 1007–1020. doi:10.1007/s11696-017-0343-0
Linganathan, P., and Samuel, J. M. (2014a). Effect of Dodecyl Benzene Sulphonic Acid on the Electrical Conductivity Behavior of Poly (2-chloroaniline) and Poly (2-chloroaniline)/Silk Blends. Am. J. Polym. Sci. 4 (4), 107–116. doi:10.5923/j.ajps.20140404.02
Linganathan, P., and Samuel, J. M. (2014b). Synthesis Characterization and Electrical Conductivity of Poly (2-chloroaniline)/MMT and Poly (2-chloroaniline)/Na-Bentonite Nanocomposites in the Presence of Surfactants. Int. J. Sci. Techn. Res. 3, 69–75.
Linganathan, P., Sundararajan, J., and Samuel, J. M. (2014). Synthesis, Characterization, and Photoconductivity Studies on Poly(2-Chloroaniline) and Poly(2-chloroaniline)/CuO Nanocomposites. J. Composites 2014, 1–9. doi:10.1155/2014/838975
Mattausch, H. (2015). “Properties and Applications of Nanoclay Composites,” in Polymer Nanoclay Composites (Singapore: William Andrew Publishing), 127–155. doi:10.1016/b978-0-323-29962-6.00005-4
Mishra, R., and Militky, J. (2019). “Nanocomposites,” in Nanotechnology in Textiles, 263–310. doi:10.1016/b978-0-08-102609-0.00006-7
Mousa, M., Evans, N. D., Oreffo, R. O. C., and Dawson, J. I. (2018). Clay Nanoparticles for Regenerative Medicine and Biomaterial Design: a Review of clay Bioactivity. Biomaterials 159, 204–214. doi:10.1016/j.biomaterials.2017.12.024
Narayanasamy, K., Sekar, S. S., R, R., R, S. K., Roy, D., and Dinakaran, K. (2021). Synthesis and Characterization of Ag/Au-MnO2 Nanostructure Embedded Polyvinylidine Difluoride High K Nanocomposites. Int. J. Polym. Anal. Characterization 26 (1), 37–46. doi:10.1080/1023666x.2020.1840864
Nikolic, L., Ristic, I., Stojiljkovic, S., Vukovic, Z., Stojiljkovic, D., Nikolic, V., et al. (2012). The Influence of Montmorillonite Modification on the Properties of Composite Material Based on Poly(methacrylic Acid). J. Compos. Mater. 46 (8), 921–928. doi:10.1177/0021998311412987
Padmaja, S., and Samuel, J. M. (2020). Chemically Copolymerized Poly (2-Chloroaniline-Co-2-Ethylaniline) as an Anodic Material in Li-Ion Batteries. Polym. Bull. 78, 1–18. doi:10.1007/s00289-020-03478-9
Rubilar, G. (2013). Imágenes de Alteridad. Reflexiones y aportes para el trabajo social en contextos de pobreza y exclusión.
Saeed, J. A., Mamta, R., and Rani, S. K. (2014). Preparation and Characterization of Nanocomposite between Poly(aniline-Co-M-Chloroaniline)-Copper Sulfide Nanoparticles. Physica B 443, 107–113. doi:10.1016/j.physb.2014.02.025
Sarathi, P. S. (1999). In-situ Combustion Handbook-Pprinciples and Practices. Tulsa, OK US: National Petroleum Technology Office. No. DOE/PC/91008-0374.
Xiao, J., Hu, Y., Wang, Z., Tang, Y., Chen, Z., and Fan, W. (2005). Preparation and Characterization of Poly(butylene Terephthalate) Nanocomposites from Thermally Stable Organic-Modified Montmorillonite. Eur. Polym. J. 41 (5), 1030–1035. doi:10.1016/j.eurpolymj.2004.11.025
Keywords: montmorillonite clay, polyorthochloroaniline, nanocomposites, nanofiller, thermal stability, flame retardancy
Citation: Iqbal S, Nadeem S, Ahmad MN, Javed M, Alsaab HO, Awwad NS, Ibrahium HA, Elkaeed EB, Alshammari FH, Alwadai N, Alahmdi MI, Bahadur A, Hassan Su and Mohyuddin A (2022) Fabrication of Poly(o-Chloroaniline) to MMT Clay as Potential Flame-Resistant Material. Front. Mater. 9:874735. doi: 10.3389/fmats.2022.874735
Received: 12 February 2022; Accepted: 01 March 2022;
Published: 29 March 2022.
Edited by:
Saima Batool, Shenzhen University, ChinaReviewed by:
Shoaib Anwer, Khalifa University, United Arab EmiratesCopyright © 2022 Iqbal, Nadeem, Ahmad, Javed, Alsaab, Awwad, Ibrahium, Elkaeed, Alshammari, Alwadai, Alahmdi, Bahadur, Hassan and Mohyuddin. This is an open-access article distributed under the terms of the Creative Commons Attribution License (CC BY). The use, distribution or reproduction in other forums is permitted, provided the original author(s) and the copyright owner(s) are credited and that the original publication in this journal is cited, in accordance with accepted academic practice. No use, distribution or reproduction is permitted which does not comply with these terms.
*Correspondence: Shahid Iqbal, c2hhaGlkZ2NzMTBAeWFob28uY29t
†These authors have contributed equally to this work
Disclaimer: All claims expressed in this article are solely those of the authors and do not necessarily represent those of their affiliated organizations, or those of the publisher, the editors and the reviewers. Any product that may be evaluated in this article or claim that may be made by its manufacturer is not guaranteed or endorsed by the publisher.
Research integrity at Frontiers
Learn more about the work of our research integrity team to safeguard the quality of each article we publish.