- 1Department of Chemistry, School of Science, University of Management and Technology, Lahore, Pakistan
- 2Department of Chemistry, School of Natural Sciences (SNS), National University of Science and Technology (NUST), Islamabad, Pakistan
- 3Chemistry and Chemical Engineering Guangdong Laboratory, Shantou, China
- 4Department of Chemistry, G. C. University Lahore, Lahore, Pakistan
- 5Department of Physics, College of Science, Princess Nourah Bint Abdulrahman University, Riyadh, Saudi Arabia
- 6Biology Department, College of Science, Princess Nourah Bint Abdulrahman University, Riyadh, Saudi Arabia
- 7Department of Chemistry, Turabah University College, Taif University, Taif, Saudi Arabia
- 8Department of Pharmaceutics and Pharmaceutical Technology, Taif University, Taif, Saudi Arabia
- 9Chemistry Department, Faculty of Science, King Khalid University, Abha, Saudi Arabia
- 10Biology Department, Faculty of Science, King Khalid University, Abha, Saudi Arabia
- 11Department of Semi Pilot Plant, Nuclear Materials Authority, New Cairo, Egypt
- 12Department of Pharmaceutical Sciences, College of Pharmacy, AlMaarefa University, Riyadh, Saudi Arabia
- 13Department of Applied Chemistry, Government College University Faisalabad, Faisalabad, Pakistan
The growth of carbon nanostructures from vegetable oils using a modified conventional approach is a simple and environmentally friendly technology with controllable features. The goal of this study is to develop a simple and environmentally friendly process for making carbon nanoparticles using commercially available, low-cost vegetable oils. The technique involves the controlled burning of “Mustard”, “Olive”, and “Linseed” oils using the traditional clay lamps and collecting the carbon soot on a ceramic plate. The prepared carbon nanoparticles were purified through sonication and subjected to characterization using powder X-ray diffraction, SEM, Fourier transformed infrared, Thermogravimetric and differential scanning calorimetric analyses. The average particle size of carbon nanoparticles as investigated by powder X-Ray Diffraction analyses was found to be 18, 24, and 57 nm for mustard, olive and linseed oils respectively. SEM analyses revealed the surface morphology of these carbon nanostructures as spherical particles. Thermogravimetric analysis (TGA) and Differential Scanning Calorimetry (DSC) providing knowledge about the thermal stability of these carbon nanoparticles. The synthesized carbon nanoparticles were screened for antibacterial activities against different species (e.g., Pseudomonas aeruginosa, Streptococcus haemolyticus, Proteus refrigere and Staphylococcus aureus) and fruitful results have been obtained.
Highlights
1) The construction of carbon nanomaterials from vegetable oils through the modified green method with controllable properties.
2) The technique involves the controlled burning of natural oils using traditional clay lamps and collecting the carbon soot on a ceramic plate.
3) Powder X-Ray Diffraction investigations revealed that the average particle size of carbon nanoparticles is in the range of 18–57 nm.
Introduction
In the current age, nanomaterials have found greater attention in the scientific community due to their wide applications in almost all current fields of technology such as chemical, manufacturing, medical, agricultural, and water purification (Iqbal, 2020; Gautam et al., 2022; Ma et al., 2022). The importance of such materials is due to their unique physical, electrical and mechanical properties (Iqbal et al., 2021; Zhu et al., 2021; Yang et al., 2022). They have been extensively used in manufacturing, fuel cells, molecular sieves, electric nano-conductors, Li-ion secondary batteries, hydrogen adsorbents and many more fields (Iqbal et al., 2020; Khan and Alaie, 2022). Carbonaceous materials, such as activated carbon, are widely used in water and wastewater treatment, chemical processing, and gas purification and separation because they are porous adsorption materials with abundant micropores (0–2 nm), mesopores (2–50 nm), and macropores (>50 nm) with high specific areas. (Sher et al., 2021; Mokhtari-Farsani et al., 2022; Zhang et al., 2022a).
Carbon nanotubes, fullerenes, and nanofibers are examples of carbon-based nanomaterials that have potential applications in biosensing, nanotechnology, and drug delivery (Shoaib et al., 2017; Liu et al., 2022; Rani et al., 2022). CNPs, a novel family of carbon-based nanomaterials with intriguing photoluminescence characteristics, have just been identified (Chattopadhyay et al., 2022; Nadeem et al., 2022). Nanodiamonds or materials generated from carbon nanotubes and graphite laser ablation are among the nanoparticles (Liu et al., 2007; Bahadur et al., 2021). Arc discharge, laser vaporization, and chemical vapor deposition are all recognized carbon nanomaterial manufacturing processes (Bakhshandeh et al., 2022; Liu et al., 2022). Besides this, the scientific world is always in search of far simpler and more economical methods of synthesis (Li D. et al., 2022). The different methods for the synthesis of carbon nanomaterial are broadly classified into two groups, called Physical and Chemical activation methods (Mei et al., 2022). Pyrolysis (or Carbonization) is followed by controlled oxidation to activate the carbon in the presence of an activating agent such as steam, carbon dioxide, or other gases. The pyrolysis method of organic compounds and oils is one of the growing methods of nano-material synthesis (Zhang et al., 2022b). Vegetable oils are an important natural raw material in the food sector (Bahadur et al., 2019; Chawla et al., 2022). They are complex mixtures whose primary components are triglycerols of saturated and unsaturated fatty acid units.
Their widespread availability, low cost, and ease of conversion into valuable chemicals and novel materials have piqued the public’s interest in their utilization. With carbon chains ranging from 6 to 20 carbon atoms, these fatty acids can be saturated or unsaturated. (Li H. et al., 2022).The simplicity is the attraction and continuous 6–8 h of pyrolysis is the investment of this experiment (Jayaprakasan et al., 2013). Carbon nanoparticles from three different seed oils (mustard, olive, and linseed oils) were prepared. The simple combustion method was found to be equally efficient as others for example chemical vapor deposition, electric arc discharge, and laser ablation methods, etc. This facile and cheaper method yielded the carbon particles in the nano range from the above-mentioned oils (Shenderova et al., 2002; Wang et al., 2002; Valcárcel et al., 2008; Lu et al., 2009). After the preparation of carbon nanoparticles, they were characterized by different techniques such as Thermogravimetric analysis (TGA), powder X-Ray Diffraction (XRD), and Differential Scanning Calorimetry (DSC), Scanning Electron Microscope (SEM), and Fourier Transform Infra-Red spectroscopy (FTIR) (Frackowiak and Béguin, 2002; Scida et al., 2011).
Experimental
Materials
Three different samples of seed oils (Mustard, Linseed and Olive oils) were purchased from an open shop and were used as such.
Preparation of Seed Oils Soot
Firstly, different seed oils including mustard, olive, linseed, and castor oils were placed in a chemical laboratory lamp having a combustible cylindrical wick or cotton. These lamps were left for 36 h for absorption of respective seed oils is carried out by cotton or wick. After lighting the lamp with a match and allowing it to burn, a china dish was placed above the flame of the lamp so that carbon soot should be collected above the lamp. After the collection of 5–10 g of carbon soot, the experiment was terminated.
Characterization of Carbon Nanoparticles
The carbon nanoparticles synthesized by combustion of seed oils (mustard, olive, linseed and castor) were analyzed by different techniques. Particle size determination of deposited black materials was confirmed by powder x-ray diffraction analysis (Biscoe and Warren, 1942; Jiang et al., 1999). Similarly, EDS, TGA, DSC, SEM and FTIR techniques were also applied to determine the surface morphology and thermal stability of the carbon Nanoparticles (Pang et al., 1993; Tang et al., 2004). Finally, biological applications of carbon nanoparticles were analyzed such as antimicrobial, carcinogenic, respiratory problems, skin exposure and other biomedical fields (Kang et al., 2008; Arias and Yang, 2009; Yang et al., 2010; Dong et al., 2012).
Results and Discussions
The burning of seed oils to form carbon nanoparticles is a thermal decomposition technique. In this technique, the formation of other substances is observed by the breakdown of seed oils (Mustard, Olive, Linseed, and Castor oil) flame. After the thermal decomposition technique, air-borne particles were analyzed to be extremely small and always show individual existence. The resulting carbon nanoparticles were finally tested for their biological activities such as antimicrobial, carcinogenic, respiratory problems (Lam et al., 2004; Warheit et al., 2004; Shvedova et al., 2005) and skin exposure (Sato et al., 2005; Murray et al., 2009).
The powder XRD results/spectra of carbon Nanoparticles (Kril and Birringer, 1998) (Figure 1) produced from mustard oil determine that two Bragg diffraction peaks are observed at near 2θ = 25.010°, 43.770°, and 45.237°. The XRD spectrum of carbon nanoparticles synthesized by olive oil determines that three Bragg diffraction peaks are observed at near 2θ = 24.620°, 45.088°, and 52.116°. The XRD analysis of carbon nanoparticles prepared with linseed oil showed that two Bragg diffraction peaks (Buhrke et al., 1998) are observed at near 2θ = 24.855° and 44.086° (Tables 1–3).
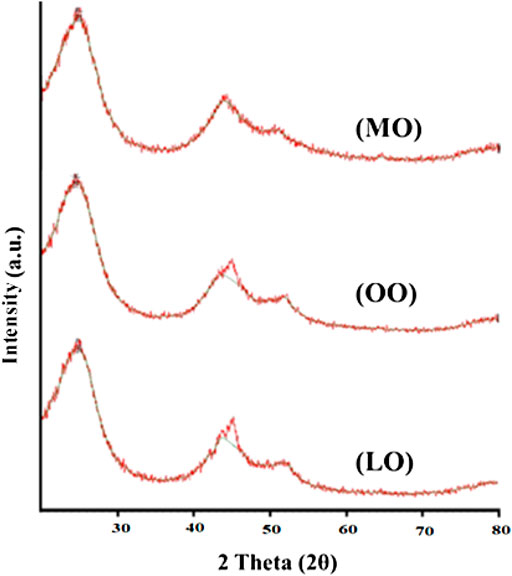
FIGURE 1. XRD spectra of carbon nanoparticles synthesized by Mustard oil (MO), Olive oil (OO) and Linseed oil (LO).
A scanning electron microscope (SEM) is used to determine the surface morphology of carbon nanoparticles, depending on the fact that SEM permits ideal visualization (Figure 2). SEM is a special kind of electron microscope in which the production of images of the sample occurs after scanning it with an electron gun (Mcmullan, 1995). After spreading the carbon nanoparticles samples on glue-covered aluminum stubs, the imaging of stub mounted samples were done on a scanning electron microscope (JSM-6490, JEOL, Japan) carried out at 20 KV. After obtaining the images of samples, surface morphology was determined at various magnifications from 550 to 25,000 times. Seed oils samples were observed by scanning electron microscope (SEM) at “Center for advance studies in physics” (CASP) in G.C. University Lahore. After careful analysis, images of seed oils samples (mustard, olive, linseed, and castor oil) were taken at different locations and different magnifications.
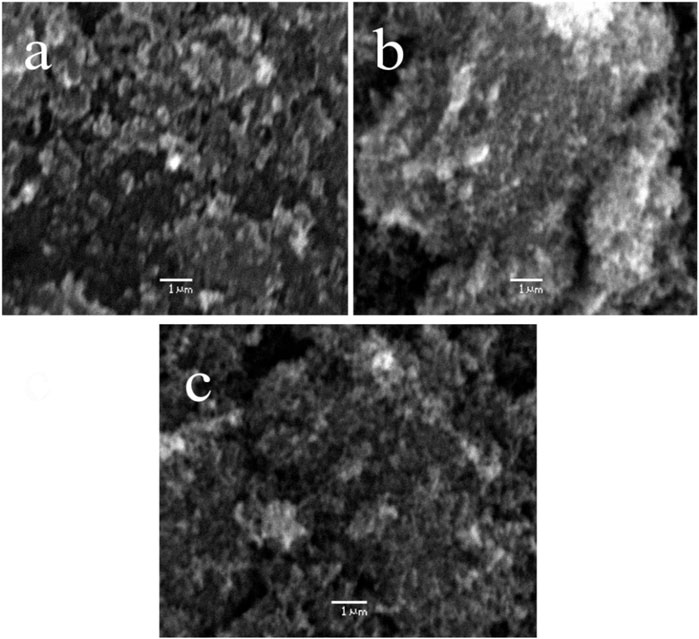
FIGURE 2. SEM micrographs of carbon nanoparticles synthesized from (A) mustard oil (B) olive oil and (C) linseed oil.
After SEM analyses of carbon nanoparticles, it was determined that the surface morphology of carbon nanoparticles synthesized by seed oils (mustard, olive, linseed and castor oil) was non-uniform (Mcmullan, 1953; Oatley et al., 1966). In the SEM micrograph, a few grains of carbon nanoparticles were indicated. These non-uniformed spherical carbon particles range from 8–77 nm but the majority of them have a particle size of 19 nm. Carbon nanoparticles produced by the combustion of seed oils are a mixture of a few nanoparticles and carbon in their elemental form. These SEM micrographs indicated that all carbon nanoparticles were magnified to 5500, 12,000, and 18,000 times. These soot particles manufactured from seed oils are tiny and due to their nanometric range, their antimicrobial activity is further enhanced (Smith and Oatley, 1955; Giri, 1957).
The thermal stability of carbon nanoparticles was determined by using the TGA technique in the temperature range of 0–500°C (Figure 3). Four different samples of carbon nanoparticles (Mustard, olive, Linseed, and Castor oil) were analyzed separately to determine their weight loss as a function of temperature (Coats and Redfern, 1963). After a brief TGA analysis of carbon nanoparticles, it was investigated that carbon nanoparticles synthesized by mustard oil show 60% loss in weight as the temperature is increased from 0–500°C and it is the highest weight loss observed in all seed oils samples as confirmed by its TGA spectra. Similarly, carbon nanoparticles synthesized by olive oil were tested for their thermal stability which revealed that these nanostructured materials indicate only 10% weight loss after enhancement of temperature from 0–500°C. Surprisingly, carbon nanoparticles produced by combustion of linseed oil after TGA analysis did not show the same trend as given by mustard oil. The minimum weight loss was observed in these particles which was only 4% even upon temperature variation of 0–500°C. Finally, carbon nanoparticles synthesized by combustion of castor oils showed the same trend as observed in the case of olive oil upon TGA analysis. These carbon nanoparticles also indicated 10% weight loss when the temperature is increased from 0–500°C. All these thermal stability facts are verified from their thermal gravimetric analytical spectra (Coats and Redfern, 1963).
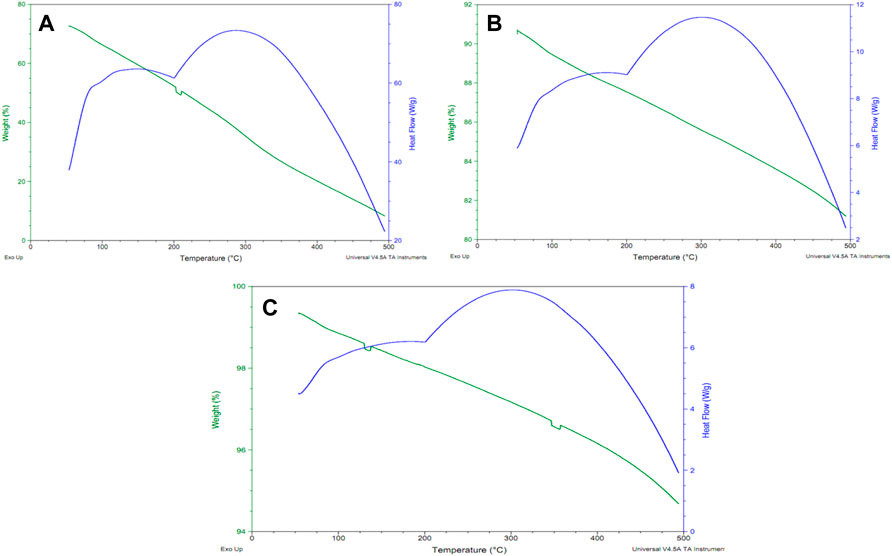
FIGURE 3. TGA and DSC spectra of carbon nanoparticles by (A) mustard oil, (B) olive oil and (C) linseed oil.
The chemical composition and structure identification of carbon nanoparticles was determined by FTIR analysis (Figure 4). The additional advantage of this technique is that detection of various functional groups is also investigated by this analytical tool. After FTIR analysis of mustard oil soot, it was indicated that we obtain a complicated mixture of various carbon nanoparticles, carbon in an elemental form, and some other species. Dry carbon nanoparticles after synthesis by mustard oil were fed to the FTIR instrument for the identification of functional groups. After obtaining the FTIR spectrum, it was observed that various functional groups of oxygen and hydrocarbon are present in it. In the mustard oil FTIR spectrum, the appearance of various peaks confirmed different functional groups [44]. The first peak at 3410 cm−1 (m) indicates O-H stretching and secondly a very slight peak at 1670 cm−1clearly justifies the C=C stretching of aromatic hydrocarbons. Similarly, peaks near 1465 cm−1. Similarly, several other peaks are observed in mustard oil spectra which further confirm the presence of other functional groups such as a peak near 1400 cm−1 indicating C-H bending in CH3 and 1118 cm−1 determine the identification of C-O stretching. During this technique, signals of C-O and O-H may appear due to some environmental moisture that contaminates the sample, and hence it was reported that synthesized carbon nanoparticles are a complex mixture of some percentage of hydrocarbons and little amount of oxygen (0.01%). Finally, it was determined that highly pure carbon nanoparticles are produced combustion of seed oils (Kiss-Eröss, 1976).
Conclusion
Synthesis of carbon nanoparticles was carried out by a very simple and cheaper method (combustion method) of different samples of seed oils including mustard oil, olive, linseed and castor oil. Scanning electron microscope (SEM) indicated that spherical carbon nanoparticles differ from each other in their particle size. The average particle size of carbon particles from mustard oil, olive oil and linseed oil was determined to be 18 nm, 24 and 57 nm respectively. The powder x-ray diffraction confirmed that these particles contained a small percentage of hexagonal graphite in combination with a high percentage of amorphous carbon nanoparticles. After completing the chemical characterization of these carbon nanoparticles with the help of XRD, SEM, TGA and FTIR analyses, their biological activities were tested like antimicrobial activity, carcinogenic, lungs exposure (respiratory problems) and skin exposure and produced excellent results. Hence, it is finally concluded that the synthesis of carbon nanoparticles by combustion of seed oils (Mustard, olive, linseed and castor oil) is not only the cheaper method but also provides an excellent platform for testing the biological activities of carbon nanoparticles.
Data Availability Statement
The original contributions presented in the study are included in the article/Supplementary Material, further inquiries can be directed to the corresponding author.
Author Contributions
SN: Conception, design of the study, writing-original draft preparation. MJ: Conception, design of the study, acquisition of data, interpret the data. SI: Interpret the data, performed major experimental works, writing-original draft preparation and editing. AM: Conception, design of the study, acquisition of data. QM: Visualization of data, reviewed the original manuscript and critical revision. NA: Interpret the data, and critical revision. MDA: Reviewed original manuscript, and critical revision. MTA: Visualization of data, and critical revision. HOA: Reviewed original manuscript, and critical revision. NSA: Visualization of data, EDX analysis, writing reviewing and editing. HAI: Conception, visualization of data, performed EDX analysis, acquisition of data. EBE: Reviewing of data and financial funding. MNA: Visualization of data, reviewed the original manuscript. SMH: Interpret the data, writing-original draft preparation. HR: Performed XRD analysis, reviewed the original manuscript and critical revision.
Conflict of Interest
The authors declare that the research was conducted in the absence of any commercial or financial relationships that could be construed as a potential conflict of interest.
Publisher’s Note
All claims expressed in this article are solely those of the authors and do not necessarily represent those of their affiliated organizations, or those of the publisher, the editors and the reviewers. Any product that may be evaluated in this article, or claim that may be made by its manufacturer, is not guaranteed or endorsed by the publisher.
Acknowledgments
The authors extend their appreciation to the Deanship of Scientific Research at King Khalid University for supporting this work through the research groups program under grant number R.G.P.2/107/42. HA would like to acknowledge Taif University Researchers Supporting Project number (TURSP-2020/67), Taif University, Taif, Saudi Arabia. The authors extend their appreciation to the Research Center at AlMaarefa University for funding this work.
References
Arias, L. R., and Yang, L. (2009). Inactivation of Bacterial Pathogens by Carbon Nanotubes in Suspensions. Langmuir 25, 3003–3012. doi:10.1021/la802769m
Bahadur, A., Hussain, W., Iqbal, S., Ullah, F., Shoaib, M., Liu, G., et al. (2021). A Morphology Controlled Surface Sulfurized CoMn2O4 Microspike Electrocatalyst for Water Splitting with Excellent OER Rate for Binder-free Electrocatalytic Oxygen Evolution. J. Mat. Chem. A 9, 12255–12264. doi:10.1039/d0ta09430g
Bahadur, A., Saeed, A., Shoaib, M., Iqbal, S., and Anwer, S. (2019). Modulating the Burst Drug Release Effect of Waterborne Polyurethane Matrix by Modifying with Polymethylmethacrylate. J. Appl. Polym. Sci. 136, 47253. doi:10.1002/app.47253
Bakhshandeh, B., Sorboni, S. G., Haghighi, D. M., Ahmadi, F., Dehghani, Z., and Badiei, A. (2022). New Analytical Methods Using Carbon-Based Nanomaterials for Detection of Salmonella Species as a Major Food Poisoning Organism in Water and Soil Resources. Chemosphere 287, 132243. doi:10.1016/j.chemosphere.2021.132243
Biscoe, J., and Warren, B. E. (1942). An X‐Ray Study of Carbon Black. J. Appl. Phys. 13, 364–371. doi:10.1063/1.1714879
Buhrke, V. E., Jenkins, R., and Smith, D. K. (1998). Practical Guide for the Preparation of Specimens for X-Ray Fluorescence and X-Ray Diffraction Analysis. Weinheim: Wiley-VCH.
Chattopadhyay, J., Pathak, T. S., and Pak, D. (2022). Heteroatom-Doped Metal-free Carbon Nanomaterials as Potential Electrocatalysts. Molecules 27, 670. doi:10.3390/molecules27030670
Chawla, S., Rai, P., Garain, T., Uday, S., and Hussain, C. M. (2022). Green Carbon Materials for the Analysis of Environmental Pollutants. Trends Environ. Anal. Chem. 33, e00156. doi:10.1016/j.teac.2022.e00156
Coats, A. W., and Redfern, J. P. (1963). Thermogravimetric Analysis. A Review. Analyst 88, 906–924. doi:10.1039/an9638800906
Dong, L., Henderson, A., and Field, C. (2012). Antimicrobial Activity of Single-Walled Carbon Nanotubes Suspended in Different Surfactants. J. Nanotechnol. 2012, 1–7. doi:10.1155/2012/928924
Frackowiak, E., and Béguin, F. (2002). Electrochemical Storage of Energy in Carbon Nanotubes and Nanostructured Carbons. Carbon 40, 1775–1787. doi:10.1016/s0008-6223(02)00045-3
Gautam, R. K., Singh, A. K., and Tiwari, I. (2022). Nanoscale Layered Double Hydroxide Modified Hybrid Nanomaterials for Wastewater Treatment: A Review. J. Mol. Liq. 350, 118505. doi:10.1016/j.molliq.2022.118505
Giri, C. C. (1957). The Construction of a Scanning Electron Microscope and its Application to the Study of Fibres. Biological Low-Voltage Scanning Electron Microscopy. PhD Dissertation. Cambridge: University of Cambridge.
Iqbal, S., Bahadur, A., Anwer, S., Ali, S., Irfan, R. M., Li, H., et al. (2020). Effect of Temperature and Reaction Time on the Morphology of L-Cysteine Surface Capped Chalcocite (Cu2S) Snowflakes Dendrites Nanoleaves and Photodegradation Study of Methyl Orange Dye under Visible Light. Colloids Surfaces A Physicochem. Eng. Aspects 601, 124984. doi:10.1016/j.colsurfa.2020.124984
Iqbal, S., Nadeem, S., Bano, R., Bahadur, A., Ahmad, Z., Javed, M., et al. (2021). Green Synthesis of Biodegradable Terpolymer Modified Starch Nanocomposite with Carbon Nanoparticles for Food Packaging Application. J. Appl. Polym. Sci. 138, 50604. doi:10.1002/app.50604
Iqbal, S. (2020). Spatial Charge Separation and Transfer in L-Cysteine Capped NiCoP/CdS Nano-Heterojunction Activated with Intimate Covalent Bonding for High-Quantum-Yield Photocatalytic Hydrogen Evolution. Appl. Catal. B Environ. 274, 119097. doi:10.1016/j.apcatb.2020.119097
Jayaprakasan, M. V. J., Viswanathan, K., and Pradyumnan, P. (2013). Nanocarbon Material from Edible Oils: Synthesis and Characterization. IOSR-JAP 3, 51–58. doi:10.9790/4961-0365158
Jiang, H. G., Rühle, M., and Lavernia, E. J. (1999). On the Applicability of the X-Ray Diffraction Line Profile Analysis in Extracting Grain Size and Microstrain in Nanocrystalline Materials. J. Mat. Res. 14, 549–559. doi:10.1557/jmr.1999.0079
Kang, S., Herzberg, M., Rodrigues, D. F., and Elimelech, M. (2008). Antibacterial Effects of Carbon Nanotubes: Size Does Matter!. Langmuir 24, 6409–6413. doi:10.1021/la800951v
Khan, S. H., and Alaie, S. A. (2022). “Green Nanomaterials for Environmental Applications,” in Green Nanomaterials for Industrial Applications. Editors U. Shanker, C. M. Hussain, and M. Rani (Elsevier), 365–396. doi:10.1016/b978-0-12-823296-5.00012-5
Kril, C. E., and Birringer, R. (1998). Estimating Grain-Size Distributions in Nanocrystalline Materials from X-Ray Diffraction Profile Analysis. Philos. Mag. A 77, 621–640. doi:10.1080/01418619808224072
Lam, C. W., James, J. T., Mccluskey, R., and Hunter, R. L. (2004). Pulmonary Toxicity of Single-Wall Carbon Nanotubes in Mice 7 and 90 Days after Intratracheal Instillation. Toxicol. Sci. 77, 126–134. doi:10.1093/toxsci/kfg243
Li, D., Yang, Z., Yang, J., Xu, X., Wang, X., Wang, J., et al. (2022a). Application of a Catechol-Polyamine Co-deposition Method for Synthesis of Heteroatom-Doped Carbon Nanomaterials. Chem. Eng. J. 429, 132363. doi:10.1016/j.cej.2021.132363
Li, H., Chen, L., Li, X., Sun, D., and Zhang, H. (2022b). Recent Progress on Asymmetric Carbon- and Silica-Based Nanomaterials: From Synthetic Strategies to Their Applications. Nano-Micro Lett. 14, 45. doi:10.1007/s40820-021-00789-y
Liu, H., Ye, T., and Mao, C. (2007). Fluorescent Carbon Nanoparticles Derived from Candle Soot. Angew. Chem. Int. Ed. 46, 6473–6475. doi:10.1002/anie.200701271
Liu, Z., Ling, Q., Cai, Y., Xu, L., Su, J., Yu, K., et al. (2022). Synthesis of Carbon-Based Nanomaterials and Their Application in Pollution Management. Nanoscale Adv. 4, 1246–1262. doi:10.1039/d1na00843a
Lu, J., Yang, J.-X., Wang, J., Lim, A., Wang, S., and Loh, K. P. (2009). One-Pot Synthesis of Fluorescent Carbon Nanoribbons, Nanoparticles, and Graphene by the Exfoliation of Graphite in Ionic Liquids. ACS Nano 3, 2367–2375. doi:10.1021/nn900546b
Ma, S., Jiang, C., Song, Q., Chen, J., Xiao, J., and Wei, Q. (2022). Tunable Electronic Structure and Properties of H-BN Nanomaterials under Elastic Strain. J. Electron. Mat. 51, 1663–1668. doi:10.1007/s11664-022-09433-w
Mcmullan, D. (1953). An Improved Scanning Electron Microscope for Opaque Specimens. Proc. IEE-Part II Power Eng. 100, 245–256. doi:10.1049/pi-2.1953.0095
Mei, Y., He, C., Zeng, W., Luo, Y., Liu, C., Yang, M., et al. (2022). Electrochemical Biosensors for Foodborne Pathogens Detection Based on Carbon Nanomaterials: Recent Advances and Challenges. Food Bioprocess Technol. 15, 498–513. doi:10.1007/s11947-022-02759-7
Mokhtari-Farsani, A., Hasany, M., Lynch, I., and Mehrali, M. (2022). Biodegradation of Carbon-Based Nanomaterials. Importance “Biomolecular Corona” Consid. 32, 2105649. doi:10.1002/adfm.202105649
Murray, A. R., Kisin, E., Leonard, S. S., Young, S. H., Kommineni, C., Kagan, V. E., et al. (2009). Oxidative Stress and Inflammatory Response in Dermal Toxicity of Single-Walled Carbon Nanotubes. Toxicology 257, 161–171. doi:10.1016/j.tox.2008.12.023
Nadeem, S., Javed, M., Iqbal, S., Alsaab, H. O., Awwad, N. S., Ibrahium, H. A., et al. (2022). Acrylic Acid-Functionalized Cellulose Diacrylate-Carbon Nanocomposite Thin Film: Preparation, Characterization, and Applications. JOM 74, 2113–2119. doi:10.1007/s11837-022-05231-1
Oatley, C. W., Nixon, W. C., and Pease, R. F. W. (1966). Scanning Electron Microscopy. Adv. Electron. Electron Phys. 21, 181–247. doi:10.1016/s0065-2539(08)61010-0
Pang, L. S. K., Saxby, J. D., and Chatfield, S. P. (1993). Thermogravimetric Analysis of Carbon Nanotubes and Nanoparticles. J. Phys. Chem. 97, 6941–6942. doi:10.1021/j100129a001
Rani, M., KeshuYadav, J., Yadav, J., Meenu, U., and Shanker, U. (2022). “Environmental, Legal, Health, and Safety Issues of Green Nanomaterials,” in Green Functionalized Nanomaterials for Environmental Applications. Editors U. Shanker, C. M. Hussain, and M. Rani (Elsevier), 567–594. doi:10.1016/b978-0-12-823137-1.00020-8
Sato, Y., Yokoyama, A., Shibata, K.-I., Akimoto, Y., Ogino, S.-I., Nodasaka, Y., et al. (2005). Influence of Length on Cytotoxicity of Multi-Walled Carbon Nanotubes against Human Acute Monocytic Leukemia Cell Line THP-1 In Vitro and Subcutaneous Tissue of Rats In Vivo. Mol. Biosyst. 1, 176–182. doi:10.1039/b502429c
Scida, K., Stege, P. W., Haby, G., Messina, G. A., and García, C. D. (2011). Recent Applications of Carbon-Based Nanomaterials in Analytical Chemistry: Critical Review. Anal. Chim. Acta 691, 6–17. doi:10.1016/j.aca.2011.02.025
Shenderova, O. A., Zhirnov, V. V., and Brenner, D. W. (2002). Carbon Nanostructures. Crit. Rev. Solid State Mater. Sci. 27, 227–356. doi:10.1080/10408430208500497
Sher, M. J., Shahid, S., Iqbal, S., Qamar, M. A., and BahadurQayyum, A. M. A. (2021). The Controlled Synthesis of G-C3N4/Cd-Doped ZnO Nanocomposites as Potential Photocatalysts for the Disinfection and Degradation of Organic Pollutants under Visible Light Irradiation. J. Rsc Adv. 11, 2025–2039. doi:10.1039/D0RA08573A
Shoaib, M., Bahadur, A., Rahman, M. S. U., Iqbal, S., Arshad, M. I., Tahir, M. A., et al. (2017). Sustained Drug Delivery of Doxorubicin as a Function of pH, Releasing Media, and NCO Contents in Polyurethane Urea Elastomers. J. Drug Deliv. Sci. Technol. 39, 277–282. doi:10.1016/j.jddst.2017.04.010
Shvedova, A. A., Kisin, E. R., Mercer, R., Murray, A. R., Johnson, V. J., Potapovich, A. I., et al. (2005). Unusual Inflammatory and Fibrogenic Pulmonary Responses to Single-Walled Carbon Nanotubes in Mice. Am. J. Physiol. Lung Cell Mol. Physiol. 289, L698–L708. doi:10.1152/ajplung.00084.2005
Smith, K. C. A., and Oatley, C. W. (1955). The Scanning Electron Microscope and its Fields of Application. Br. J. Appl. Phys. 6, 391–399. doi:10.1088/0508-3443/6/11/304
Tang, C., Qi, K., Wooley, K. L., Matyjaszewski, K., and Kowalewski, T. (2004). Well-Defined Carbon Nanoparticles Prepared from Water-Soluble Shell Cross-Linked Micelles that Contain Polyacrylonitrile Cores. Angew. Chem. 116, 2843–2847. doi:10.1002/ange.200353401
Valcárcel, M., Cárdenas, S., Simonet, B. M., Moliner-Martínez, Y., and Lucena, R. (2008). Carbon Nanostructures as Sorbent Materials in Analytical Processes. TrAC Trends Anal. Chem. 27, 34–43. doi:10.1016/j.trac.2007.10.012
Wang, Y., Serrano, S., and Santiago-Aviles, J. J. (2002). Conductivity Measurement of Electrospun PAN-Based Carbon Nanofiber. J. Mater. Sci. Lett. 21, 1055–1057. doi:10.1023/a:1016081212346
Warheit, D. B., Laurence, B. R., Reed, K. L., Roach, D. H., Reynolds, G. A., and Webb, T. R. (2004). Comparative Pulmonary Toxicity Assessment of Single-Wall Carbon Nanotubes in Rats. Toxicol. Sci. 77, 117–125. doi:10.1093/toxsci/kfg228
Yang, C., Mamouni, J., Tang, Y., and Yang, L. (2010). Antimicrobial Activity of Single-Walled Carbon Nanotubes: Length Effect. Langmuir 26, 16013–16019. doi:10.1021/la103110g
Yang, G., Kong, H., Chen, Y., Liu, B., Zhu, D., Guo, L., et al. (2022). Recent Advances in the Hybridization of Cellulose and Carbon Nanomaterials: Interactions, Structural Design, Functional Tailoring, and Applications. Carbohydr. Polym. 279, 118947. doi:10.1016/j.carbpol.2021.118947
Zhang, Y., Qi, G., Yao, L., Huang, L., Wang, J., and Gao, W. (2022a). Effects of Metal Nanoparticles and Other Preparative Materials in the Environment on Plants: From the Perspective of Improving Secondary Metabolites. J. Agric. Food Chem. 70, 916–933. doi:10.1021/acs.jafc.1c05152
Zhang, Y., Zhu, H., Zhang, R., Yu, L., Liu, Z., Shearing, P. R., et al. (2022b). Study of Tire Pyrolysis Oil Model Compound Structure on Carbon Nanomaterial Production. ACS Sustain. Chem. Eng. 10, 800–809. doi:10.1021/acssuschemeng.1c06033
Keywords: carbon nanoparticles, soot of natural oils, antibacterial activities, green method, catalyst
Citation: Nadeem S, Javed M, Iqbal S, Mohyuddin A, Mahmood Q, Abbas Q, Alfryyan N, Alqahtani MD, Alotaibi MT, Alsaab HO, Awwad NS, Ibrahium HA, Elkaeed EB, Ahmad MN, Haroon SM and Raza H (2022) Facile Synthesis of Catalyst Free Carbon Nanoparticles From the Soot of Natural Oils. Front. Mater. 9:874726. doi: 10.3389/fmats.2022.874726
Received: 12 February 2022; Accepted: 12 April 2022;
Published: 16 May 2022.
Edited by:
Munir Ahmad, King Saud University, Saudi ArabiaReviewed by:
Gang Tang, Anhui University of Technology, ChinaMuhammad Yaqub, Kumoh National Institute of Technology, South Korea
Copyright © 2022 Nadeem, Javed, Iqbal, Mohyuddin, Mahmood, Abbas, Alfryyan, Alqahtani, Alotaibi, Alsaab, Awwad, Ibrahium, Elkaeed, Ahmad, Haroon and Raza. This is an open-access article distributed under the terms of the Creative Commons Attribution License (CC BY). The use, distribution or reproduction in other forums is permitted, provided the original author(s) and the copyright owner(s) are credited and that the original publication in this journal is cited, in accordance with accepted academic practice. No use, distribution or reproduction is permitted which does not comply with these terms.
*Correspondence: Shahid Iqbal, c2hhaGlkaXFiYWwuY2hlbUBzbnMubnVzdC5lZHUucGs=