- National Center for Physics, Quaid-i-Azam University Campus, Islamabad, Pakistan
This review deals with the progress in the field of polymer/fullerene nanocomposites particularly for the energy storage applications. Fullerene is a unique zero dimensional nanocarbon nanomaterial. Fullerene proposes several unique structural, optical, electrical, thermal, mechanical and other superior physical features to the polymeric nanocomposites. Consequently, the high performance polymer/fullerene nanocomposites result from the amalgamation of the unique characteristics of fullerene with the functional polymers. Here, the advancements in the polymer/fullerene nanocomposites regarding their processing and properties, especially the electrical conductivity, charge storage capacities, charge density, power density, charge-discharge, and cyclic performance have been discussed. Moreover, the future and challenging prospects have been summarized anticipating the progress in the field of polymer/fullerene-based energy storage technology.
1 Introduction
Fullerene is a unique allotropic form of nanocarbon (Thota et al., 2010). Like other nanocarbons, fullerene molecules have been reinforced in the polymers. Inclusion of fullerene in polymers has been known to enhance the facile processing and characteristics (Kim et al., 2017; Kanbur and Tayfun, 2019). Consequently, the polymer/fullerene nanomaterials have gained interest in the material science (Ravi et al., 2007). Fullerene nanofillers in polymers have amended the structural (Djahnit et al., 2019), morphology/crystallinity (Mohamadi and Sharifi-Sanjani, 2018), electrical conductivity (Yoshino et al., 1999), mechanical robustness (Zhao et al., 2021), and heat constancy (Djahnit et al., 2019) properties. Subsequently, polymer/fullerene nanomaterials have promising applications in the supercapacitor (Shen et al., 2021), solar cell (Armin et al., 2018), and biomedical (Moon et al., 2010) fields. Here, the poor fullerene miscibility in polymers may hinder the nanocomposite features (Prylutskyy et al., 2014). For the purpose, the fullerene molecules have been modified and introduced in polymers to developed the physical or chemical interactions. Recently, energy storage devices have adopted the use of polymer/fullerene nanocomposite (Issar and Arora, 2021; Shrestha et al., 2021). The capacitance properties and application in energy storage device have gained recent research interest (Lawes et al., 2015).
This review article presents progress in the arena of polymer and fullerene based nanocomposites. Inclusion of fullerene molecules in polymers has been employed to progress the electrical conductivity of the nanocomposites. Moreover, the specific capacitance, power density, charge density, charge-discharge, recyclability, and durability properties of the polymer/fullerene nanocomposites have been explored. However, novel polymer/fullerene nanocomposites have several challenges, which need to be overcome to attain high performance. In this regard, the mostly conducting polymers have been doped with fullerenes to attain the desired properties for the supercapacitors.
2 Fullerene
Fullerene is a symmetrical form of nanocarbon (Jehoulet et al., 1992). Fullerene is a zero dimensional molecule with sp2 hybrid carbon atoms (Chang, 2006). Fullerene is made up of polygones, i.e., pentagons and hexagons (Figure 1).
The discovery of fullerene is dated back to 1985 (Montellano López et al., 2011). Fullerene usually has inherent electrical, optical, magnetic, mechanical, thermal, and biomedical features (Withers et al., 1997; Akasaka et al., 2001; Giacalone and Martín, 2006). Fullerene molecules have been synthesized through the plasma method, arc discharge procedure, microwave synthesis, and chemical routes (Withers et al., 1997; Akasaka et al., 2001; Mojica et al., 2013). The methodological applications of fullerene have been perceived in solar cells (Chae et al., 2011), sensors (Modi et al., 2003), drug delivery (Gallo et al., 2007), etc. The solubility properties of fullerene molecules in various solvents have been focused (Nierengarten, 2004; Dmitruk, 2010). The chemical modification of C60 has found to improve the solubility of these molecules in water, chloroform, xylene, and organic solvents (Giacalone and Martín, 2006). Sometimes, the solubilizing agents or polymers such as poly(vinylpyrrolidone) have been used to improve the solubility of fullerene (Behera and Ram, 2016; Behera and Ram, 2017).
3 Fullerene Reinforced Polymeric Nanocomposites
Fullerene C60, C70, and higher fullerene analogues have been employed as reinforcement to form the polymeric nanocomposites (Geckeler and Samal, 1999). The polymer/fullerene nanocomposites have been premeditated for the optical, mechanical, thermal, charge transport, and energy storage characteristics (Kausar, 2017; Etxebarria et al., 2015; Harris, 2020). The dispersion and solubility of the fullerene molecules in polymers remain as major challenges during the nanocomposite formation (Mackay et al., 2006). Lack of solubility may lead to fullerene aggregation and mass formation. The agglomeration problem may lead to the decrease in the properties of the polymer/fullerene nanocomposites. In this way, the large-scale processing of the polymer/fullerene materials is quite challenge (Bartelt et al., 2014). The modification or functionalization of fullerenes have been focused (Song et al., 2018). Thus, the better physical or covalent contacts between the polymer and fullerene nanoparticles have revealed high performance nanocomposites. The polymer/fullerene nanocomposites have been researched for the improved electrical, magnetic, thermal, and mechanical features (Kausar, 2021). The thermoplastic and thermosetting polymers, such as epoxies, polystyrene, polyethylene, poly(methyl methacrylate), poly(ethylene glycol), and block copolymers, have been filled with the fullerenes. Then, the conducting polymers have been reinforced with the fullerene molecules (Bergbreiter et al., 1984). Among conducting polymers, polythiophene (PTh), polyaniline (PANI), polypyrrole (PPy), poly(3,4‐ethylenedioxythiophene), etc. and derived polymers have been connected with the fullerene molecules to develop the nanocomposites (Umeda, 2005). Few conducting polymers covalently linked with the fullerene molecules are given in Figure 2 (Martín, 2006).
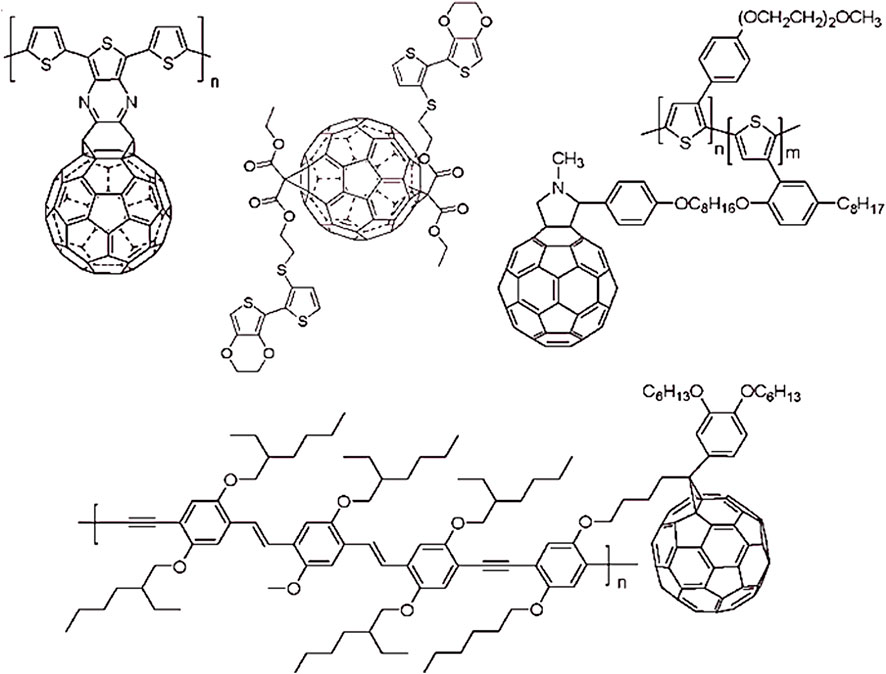
FIGURE 2. Some polymers with covalently linked fullerenes (Martín, 2006). Reproduced with permission from RSC.
The thermal stability properties of the polymer/C60 nanocomposite have been explored (Table 1) (Pereira et al., 2015). The addition of C60 contents improved the decomposition temperature of the nanocomposites (Alekseeva et al., 2018).
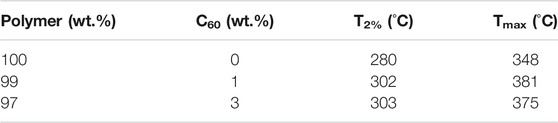
TABLE 1. Thermo-oxidative stability of polymer and corresponding fullerene nanocomposites (Pereira et al., 2015).
4 Electrical Conductivity of Polymer/Fullerene Nanocomposites
The polymer/fullerene nanocomposites have been considered for the supercapacitors (Schon et al., 2014), photovoltaics (Campoy-Quiles et al., 2008), electronics (McCamey et al., 2008), and other significant technical relevance. Tumbleston et al. (2014) produced the poly(3,4‐ethylenedioxythiophene):polystyrene sulfonate acid and fullerene C60 based nanocomposite. The conjugated polymers behave as electron donor, whereas the fullerene molecules act as electron acceptors. Huang et al. (2011) formed poly(3-hexylthiophene) (P3HT) and fullerene C60 derived nanocomposites. Here again, the electron conduction was observed due to the electron donating polymer and electron accepting C60. Chirvase et al. (2004) designed the P3HT and (Mohamadi and Sharifi-Sanjani, 2018Mohamadi and Sharifi-Sanjani, 2018)-phenyl-C61 butyric acid methyl ester (PCBM) based poly(3,4‐ethylenedioxythiophene) (Mohamadi and Sharifi-Sanjani, 2018):-phenyl-C61 butyric acid methyl ester (P3HT:PCBM) nanocomposites. The P3HT:PCBM and fullerene based nanocomposites were explored for the conducting properties. The short circuit current density of 7.8 mAcm−2 was observed. Zabihi et al. (Zabihi et al., 2016) also formed the P3HT:PCBM nanocomposites. The P3HT:PCBM and C60 based nanocomposites had high electrical conductivity, charge mobility, and current density properties. Table 2 shows the conductivity properties of the P3HT and related nanocomposite. The carrier mobility and carrier density of the nanocomposite were found superior, relative to the neat P3HT. The notable augmentation in the electrical properties of the nanocomposite was due to the matrix-nanofiller interactions. Cheng et al. (Cheng et al., 2017) inspected PANI and polydivinylbenzene (PDVB) based PANI/PDVB nanocomposites with fullerene. Figure 3 shows the enhancement in the electrical conductivity with the frequency. The electrical conductivity of the PANI/PDVB nanocomposite was enhanced from the 9 × 10−10 to 63.7 Sm-1. In this way, various conjugated polymers and their blended combinations have been used with fullerenes to promote the electron conduction characteristics.
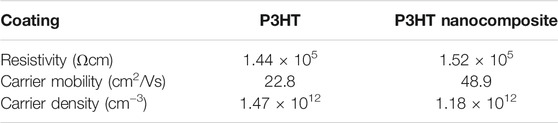
TABLE 2. Resistivity, Hall mobility, and carrier density of P3HT and nanocomposite thin film (Zabihi et al., 2016). P3HT = poly(3-hexylthiophene). Reproduced with permission from Elsevier.
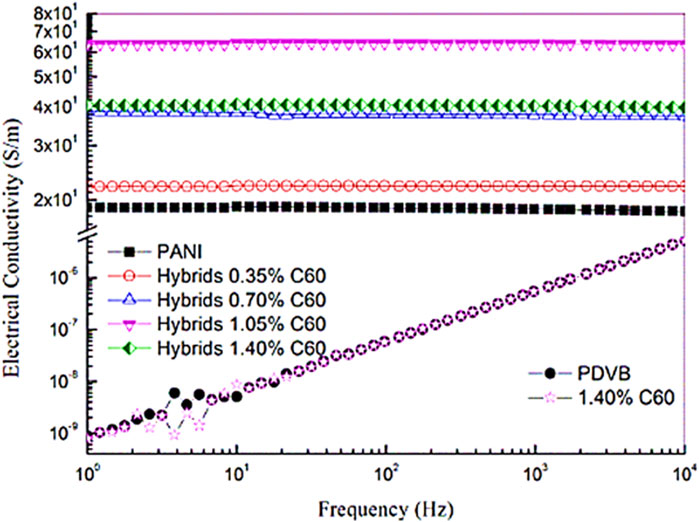
FIGURE 3. Electrical conductivity of nanocomposite with different amount of C60 (Cheng et al., 2017). PANI, polyaniline; PDVB, polydivinylbenzene. Reproduced with permission Elsevier.
Polyazomethine is a conjugated polymer (Sobarzo et al., 2021). Bronnikov et al. (2017) reported polyazomethine/fullerene nanocomposites. Fullerene was loaded in 0.25–2.5 wt.% contents. The DC electrical conductivity of the polyazomethine/fullerene nanocomposites was augmented with the cumulative nanofiller content, as well as the rising temperature. The effect was observed due to the homogeneous dispersion of the fullerene molecules in the polyazomethine and the formation of percolation complex (Bronnikov et al., 2016). Ltaief (2004) amalgamated poly[2-methoxy-5-(2′-ethyl)hexoxy-1,4-phenylenevinylene] with fullerene. The aromatic stacking interactions between the polymer and fullerene has upsurges the electron conduction properties. The 40 vol.% fullerene developed conductive pathways and percolation threshold.
Mostly, conjugated polymers have generated better conducting trails with the fullerene molecules.
5 Polymer/Fullerene Nanocomposites for Energy Storage Applications
Supercapacitors have been considered as capable energy storage devices for advanced electronic devices (Naoi et al., 2013; Raza et al., 2018). Among energy storage devices, the supercapacitors possess high specific capacitance, power density, and charge-discharge performance (Simon and Gogotsi, 2010a). The major types of the supercapacitors are portrayed in Figure 4.
Supercapacitors have been mainly categorized as the pseudocapacitors having electrochemical charge storage, double-layer capacitors with electrostatic charge storage, and hybrid capacitors containing electrochemical/electrostatic charge storage. Among energy storage devices, supercapacitors have wide ranging energy density and power density performances (Figure 5) (Libich et al., 2018). The supercapacitor performances have been found in between the capacitors and batteries/fuel cells (Conway, 1999; Hu et al., 2006; Pothu, 2020).
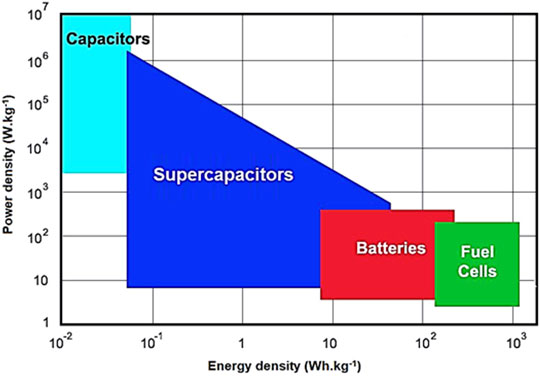
FIGURE 5. Ragone chart of power density as a function of energy density for numerous energy devices (Libich et al., 2018). Reproduced with permission Elsevier.
Energy storage devices have been the current demand of the modern electronics and vehicle industry (Winter and Brodd, 2004; Simon and Gogotsi, 2010b; Larcher and Tarascon, 2015). The pseudocapacitors or electrochemical supercapacitors have been deliberated as talented energy storage devices (Mousty and Leroux, 2012; Chen, 2017). Supercapacitors revealed inexpensiveness and high power stowage (Bao et al., 2011; Chen et al., 2011). These devices have been known for the high resilience, high energy density, and high power density, compared with the conservative storage devices (Ryu et al., 2004; Kim et al., 2005; Sen et al., 2013). Consequently, supercapacitors have caused revolution in the field of next-generation energy storage devices (Liu et al., 2010; Arico et al., 2011). Supercapacitors have been successfully applied in the electronics, robotics, etc. (Wang et al., 2007; Smith et al., 2008; Chen et al., 2010). The supercapacitors have found the use of conjugated polymers such as polyaniline, polypyrrole, polythiophene, and poly(3,4-ethylenedioxythiophene) (PEDOT), etc. (White and Slade, 2004; Snook et al., 2007; Liu et al., 2008). These conjugated polymers possess high electron conduction and specific capacitance (Patake et al., 2009; Li et al., 2010; Poizot and Dolhem, 2011). However, drawback of using conducting polymers in supercapacitors may be the low charge-discharge, stability, and reversibility. Therefore, the conjugated polymers have been filled with the nanoparticles such as graphene, graphite, and inorganic nanoparticles (Stenger-Smith et al., 2002; Jayalakshmi and Balasubramanian, 2008; Zhang et al., 2010).
Fullerenes have been explored for the supercapacitor devices due to the intrinsic high surface area and electrical conductivity properties (Wang et al., 2017; Benzigar et al., 2019). The polymeric nanocomposites of fullerenes have been applied in the supercapacitor designs (Pech et al., 2010; Lin et al., 2013). Wang et al. (2017) prepared polyaniline emeraldine base (PANI-EB) and polyaniline emeraldine base/fullerene C60 whisker (PANI-EB/FW) nanocomposite. The PANI-EB/FW was used in the supercapacitor electrode. Figure 6 shows the unique morphology of the PANI-EB/FW supercapacitor electrode. The unique fullerene nanowhisker with high surface area was observed. Figure 7 demonstrates the specific capacitance of PANI-EB/FW as 813 Fg−1, which was found considerably higher than the neat PANI-EB (248 Fg−1). Figure 8 displays the capacitance retention of 85.2% for the nanocomposite electrode, after 1,500 cycles. The excellent performance was due to the synergistic effects of the polymer and fullerene nanoparticles.
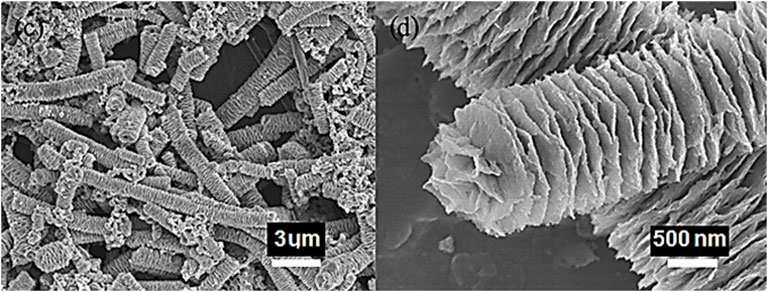
FIGURE 6. SEM images PANI-EB/FW (Wang et al., 2017). PANI-EB/FW = polyaniline emeraldine base/fullerene C60 whisker. Reproduced with permission from Elsevier.
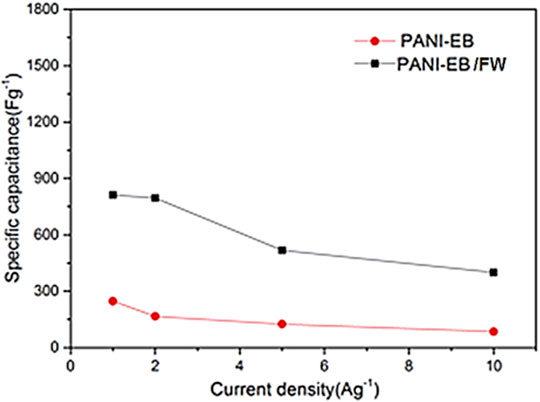
FIGURE 7. Specific capacitance of PANI-EB and PANI-EB/FW at different current densities of 1, 2, 5, 10 Ag−1, respectively. PANI-EB = polyaniline emeraldine base; PANI-EB/FW = polyaniline emeraldine base/fullerene C60 whisker (Wang et al., 2017). Reproduced with permission from Elsevier.
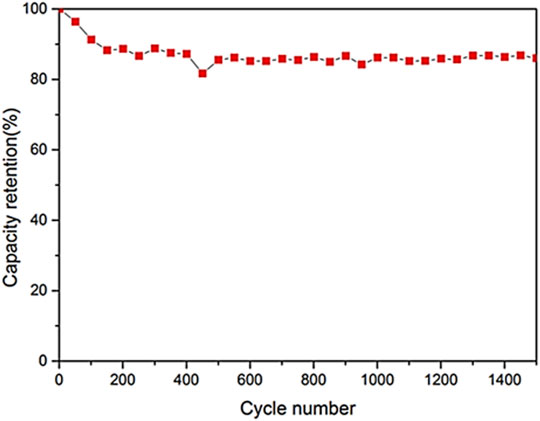
FIGURE 8. Cycling stability of PANI-EB/FW nanocomposite at 10 Ag-1 for 1,500 cycles (Wang et al., 2017). PANI-EB/FW = polyaniline emeraldine base/fullerene C60 whisker. Reproduced with permission from Elsevier.
Xiong et al. (2012) also designed PANI-EB and fullerene C60 based supercapacitor electrode. Figure 9 presents the synthesis course to the PANI-EB and C60 based nanocomposite. The C60 was linked with para-phenylenediamine (PPD) to form functional fullerene. Then, the PPD functional C60 was included to the in situ polymerization of aniline monomer. Figure 10 depicts the increased specific capacitance of 776 Fg−1, relative to the neat PANI-EB (492 Fg−1) over 50 cycles. The capacitance retention of the fullerene based nanocomposite and neat PANI-EB were observed as 96.5% and 94.9%, respectively, after 500 cycle. The C60 has electron retreating efficiency towards the conjugated polymer and so enhancing the conductivity and capacitance properties (Xiong et al., 2010; Dou et al., 2013). Ramadan et al. (2020) produced the polyaniline (PANI)/phenyl-C60-butyric acid methyl ester (PCBM) based PANI/PCBM nanocomposite. The PCBM of 2.5, 5 and 10 wt.% was added to the polyaniline. Figure 11 shows that the specific capacitance of the neat polyaniline was 1,110 Fg−1. The capacitance was considerably enhanced to 2,201 Fg−1 with wt.% nanofiller. The 96% capacitance retention was observed over 1,000 cycles. Such high values of specific capacitance were observed due to the better amalgamation of PANI and PCBM and high charge transport properties.
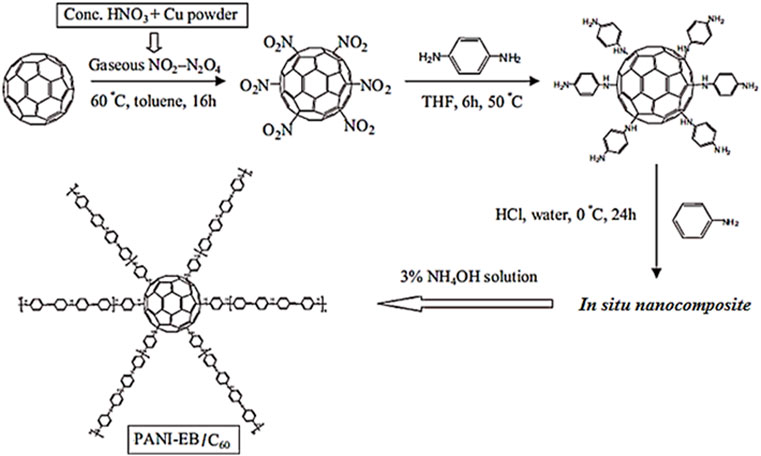
FIGURE 9. Synthesis route to PANI-EB/C60 hybrid (Xiong et al., 2012). PANI-EB/C60 = polyaniline emeraldine base/fullerene C60. Reproduced with permission from Elsevier.
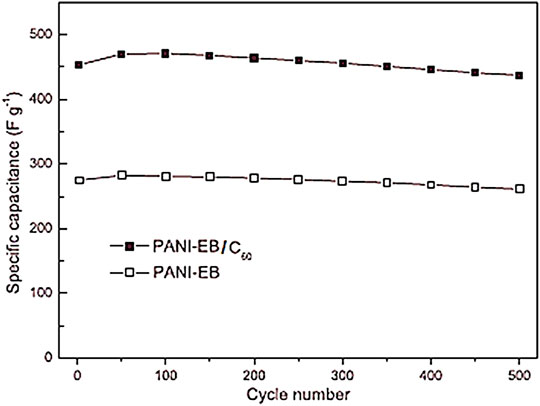
FIGURE 10. Specific capacitance against the cycle number for PANI-EB and PANI-EB/C60 (Xiong et al., 2012). PANI-EB = polyaniline emeraldine base; PANI-EB/C60 = polyaniline emeraldine base/fullerene C60. Reproduced with permission from Elsevier.
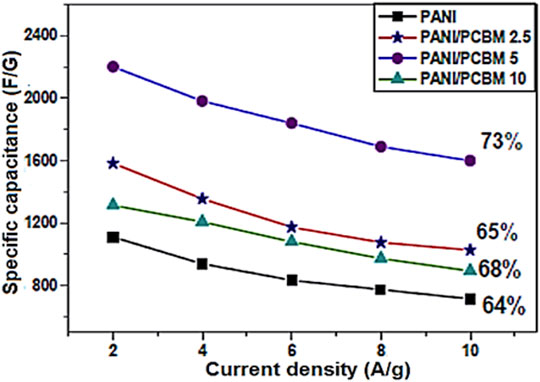
FIGURE 11. The specific capacitance vs. current density for PANI/PCBM nanocomposite (Ramadan et al., 2020). PANI/PCBM = polyaniline/phenyl-C60-butyric acid methyl ester. Reproduced with permission from Elsevier.
The poly(3-hexylthiophene) (P3HT) and PCBM derived P3HT:PCBM nanocomposites have also bene reported for the supercapacitors (Wang et al., 2012; Momodu et al., 2015). The current-voltage and capacitance-voltage techniques were used for the electrical conductivity and specific capacitance measurements (Amao, 2003). Such supercapacitors have been used for the optical/electronic devices. Hence, the approaching investigations must focus the better design combinations of the conjugated polymers and fullerenes with superior specific capacitance values (Peng et al., 2019).
6 Future and Challenging Attributes of Polymer/Fullerene Towards Energy Storage
Nowadays, the modern supercapacitor devices having superior resilience, capacitance, power density, and charge storage have been necessitated (Sen and De, 2010; Amoura et al., 2015; Senthilkumar, 2015). In this regard, novel design distinctions have been researched for the energy storage devices (Choudhary et al., 2020; Duran et al., 2020; Wang et al., 2020). Conducting polymers such as PANI, PTh, P3HT, PEDOT, etc. possess high electrical conductivity and capacitance to be applied in the supercapacitor electrodes (Wu, 2002; Jayalakshmi et al., 2007; Pandolfo, 2013). The supercapacitors having light weight, low cost, high capacitance, high power density, high constancy, recyclability, and charge-discharge recitals have been investigated (Villers et al., 2003; Cericola and Kötz, 2012; Abdelhamid et al., 2015). Use of fullerene molecules have further heightened the performance of the conjugated polymers in the supercapacitors. The polymer/fullerene nanocomposites with combinations of numerous polymers and fullerene molecules towards the structure, morphology, and energy storage properties have unveil the plethora of future opportunities. These devices have been pragmatic in electronics, digital devices, and electric vehicles. Nevertheless, the future supercapacitors with higher energy density than batteries have been desirable. The specific capacitance of the polymer/fullerene nanomaterials has been directly related to the electron conduction properties. Consequently, there is a lot of interest to augment the electrical conductivity of the nanocomposites. The fullerene aggregation may decrease the electron conductivity and capacitance characteristics. In this regard, the fullerenes demand apposite functionalization and dispersion to develop the percolation pathways in the polymers. Better interactions between polymer and fullerene nanofiller presented considerable development in the electrical conductivity and ensuing features for the high performance supercapacitors. Moreover, the future research may focus the use of phase change material in these energy storage materials (Yin et al., 2021). Especially the use of eco-friendly or bio-based materials may yield high performance fullerene based energy storage devices (Gong et al., 2021; Yin et al., 2022).
7 Summary
Hence, this review abridges the indispensable aspects of the polymer/fullerene nanocomposites for the energy storage devices. This article will be pioneering in the field of polymer/fullerene based supercapacitors. The polymer/fullerene nanomaterials have enriched electrical conductivity properties in addition to the thermal stability and mechanical strength features. Mostly, the conducting or conjugated polymers have been explored with fullerenes in the supercapacitor application. The dispersion properties of the fullerene nanoparticles in the polymeric matrices have enhanced the electron transport through the system. The superior electrical conductivity has in turn promoted the specific capacitance, and other desirable supercapacitor properties.
Author Contributions
AK: Writing of entire manuscript, outline, original draft, analysis, review, and editing.
Conflict of Interest
The author declares that the research was conducted in the absence of any commercial or financial relationships that could be construed as a potential conflict of interest.
Publisher’s Note
All claims expressed in this article are solely those of the authors and do not necessarily represent those of their affiliated organizations, or those of the publisher, the editors and the reviewers. Any product that may be evaluated in this article, or claim that may be made by its manufacturer, is not guaranteed or endorsed by the publisher.
References
Abdelhamid, M. E., O'Mullane, A. P., and Snook, G. A. (2015). Storing Energy in Plastics: a Review on Conducting Polymers & Their Role in Electrochemical Energy Storage. RSC Adv. 5 (15), 11611–11626. doi:10.1039/c4ra15947k
Akasaka, T., Wakahara, T., Nagase, S., Kobayashi, K., Waelchli, M., Yamamoto, K., et al. (2001). Structural Determination of the La@C82 Isomer. J. Phys. Chem. B 105 (15), 2971–2974. doi:10.1021/jp003930d
Alekseeva, O. V., Noskov, A. V., and Guseynov, S. S. (2018). Thermal Behaviour of Poly(methyl Methacrylate)/fullerene Composite Films. Philos. Mag. Lett. 98 (8), 330–340. doi:10.1080/09500839.2018.1548786
Amao, Y. (2003). Probes and Polymers for Optical Sensing of Oxygen. Microchimica Acta 143 (1), 1–12. doi:10.1007/s00604-003-0037-x
Amoura, D., Sánchez-Jiménez, M., Estrany, F., Makhloufi, L., and Alemán, C. (2015). Clay Incorporation at the Dielectric Layer of Multilayer Polymer Films for Electrochemical Activation. Eur. Polym. J. 69, 296–307. doi:10.1016/j.eurpolymj.2015.05.030
Arico, A. S., Bruce, P., Scrosati, B., Tarascon, J. M., and Van Schalkwijk, W. (2011). “Nanostructured Materials for Advanced Energy Conversion and Storage Devices,” in Materials for Sustainable Energy: A Collection of Peer-Reviewed Research and Review Articles from (Nature Publishing GroupWorld Scientific), 148–159.
Armin, A., Chen, Z., Jin, Y., Zhang, K., Huang, F., and Shoaee, S. (2018). A Shockley-type Polymer: Fullerene Solar Cell. Adv. Energ. Mater. 8 (7), 1701450. doi:10.1002/aenm.201701450
Bao, L., Zang, J., and Li, X. (2011). Flexible Zn2SnO4/MnO2 Core/Shell Nanocable−Carbon Microfiber Hybrid Composites for High-Performance Supercapacitor Electrodes. Nano Lett. 11 (3), 1215–1220. doi:10.1021/nl104205s
Bartelt, J. A., Douglas, J. D., Mateker, W. R., Labban, A. E., Tassone, C. J., Toney, M. F., et al. (2014). Controlling Solution-phase Polymer Aggregation with Molecular Weight and Solvent Additives to Optimize Polymer-Fullerene Bulk Heterojunction Solar Cells. Adv. Energ. Mater. 4 (9), 1301733. doi:10.1002/aenm.201301733
Behera, M., and Ram, S. (2017). Strongly Optical Absorptive Nanofluids and Rheology in Bonded Fullerene C60 via Poly(vinyl Pyrrolidone) Molecules in Water. Fullerenes, Nanotubes and Carbon Nanostructures 25 (3), 143–150. doi:10.1080/1536383x.2016.1271788
Behera, M., and Ram, S. (2016). Variation of Optical Properties, Rheology, and Microstructure in Fullerene/poly(vinyl Pyrrolidone) Nanofluids with Fullerene Content Inn-Butanol. Fullerenes, Nanotubes and Carbon Nanostructures 24 (2), 154–161. doi:10.1080/1536383x.2015.1130703
Benzigar, M. R., Joseph, S., Saianand, G., Gopalan, A.-I., Sarkar, S., Srinivasan, S., et al. (2019). Highly Ordered Iron Oxide-Mesoporous Fullerene Nanocomposites for Oxygen Reduction Reaction and Supercapacitor Applications. Microporous Mesoporous Mater. 285, 21–31. doi:10.1016/j.micromeso.2019.04.071
Bergbreiter, D. E., Chen, Z., and Hu, H. P. (1984). Entrapment of Functionalized Ethylene Oligomers in Polyethylene. Macromolecules 17 (10), 2111–2116. doi:10.1021/ma00140a041
Bronnikov, S., Podshivalov, A., Kostromin, S., Asandulesa, M., and Cozan, V. (2016). Dielectric Spectroscopy Investigation on Relaxation in Polyazomethine/fullerene C 60 Nanocomposites. Eur. Polym. J. 78, 213–225. doi:10.1016/j.eurpolymj.2016.03.035
Bronnikov, S., Podshivalov, A., Kostromin, S., Asandulesa, M., and Cozan, V. (2017). Electrical Conductivity of Polyazomethine/fullerene C60 Nanocomposites. Phys. Lett. A 381 (8), 796–800. doi:10.1016/j.physleta.2016.12.045
Campoy-Quiles, M., Ferenczi, T., Agostinelli, T., Etchegoin, P. G., Kim, Y., Anthopoulos, T. D., et al. (2008). Morphology Evolution via Self-Organization and Lateral and Vertical Diffusion in Polymer:fullerene Solar Cell Blends. Nat. Mater 7 (2), 158–164. doi:10.1038/nmat2102
Cericola, D., and Kötz, R. (2012). Hybridization of Rechargeable Batteries and Electrochemical Capacitors: Principles and Limits. Electrochimica Acta 72, 1–17. doi:10.1016/j.electacta.2012.03.151
Chae, S.-R., Therezien, M., Budarz, J. F., Wessel, L., Lin, S., Xiao, Y., et al. (2011). Comparison of the Photosensitivity and Bacterial Toxicity of Spherical and Tubular Fullerenes of Variable Aggregate Size. J. Nanopart Res. 13 (10), 5121–5127. doi:10.1007/s11051-011-0492-y
Chen, G. Z. (2017). Supercapacitor and Supercapattery as Emerging Electrochemical Energy Stores. Int. Mater. Rev. 62 (4), 173–202. doi:10.1080/09506608.2016.1240914
Chen, H.-W., Hsu, C.-Y., Chen, J.-G., Lee, K.-M., Wang, C.-C., Huang, K.-C., et al. (2010). Plastic Dye-Sensitized Photo-Supercapacitor Using Electrophoretic Deposition and Compression Methods. J. Power Sourc. 195 (18), 6225–6231. doi:10.1016/j.jpowsour.2010.01.009
Chen, Z., Augustyn, V., Wen, J., Zhang, Y., Shen, M., Dunn, B., et al. (2011). High-Performance Supercapacitors Based on Intertwined CNT/V2O5 Nanowire Nanocomposites. Adv. Mater. 23 (6), 791–795. doi:10.1002/adma.201003658
Cheng, X., Yokozeki, T., Yamamoto, M., Wang, H., Wu, L., Koyanagi, J., et al. (2017). The Decoupling Electrical and thermal Conductivity of Fullerene/polyaniline Hybrids Reinforced Polymer Composites. Composites Sci. Tech. 144, 160–168. doi:10.1016/j.compscitech.2017.03.030
Chirvase, D., Parisi, J., Hummelen, J. C., and Dyakonov, V. (2004). Influence of Nanomorphology on the Photovoltaic Action of Polymer-Fullerene Composites. Nanotechnology 15 (9), 1317–1323. doi:10.1088/0957-4484/15/9/035
Choudhary, R. B., Ansari, S., and Purty, B. (2020). Robust Electrochemical Performance of Polypyrrole (PPy) and Polyindole (PIn) Based Hybrid Electrode Materials for Supercapacitor Application: A Review. J. Energ. Storage 29, 101302. doi:10.1016/j.est.2020.101302
Conway, B. E. (1999). “Similarities and Differences between Supercapacitors and Batteries for Storing Electrical Energy,” in Electrochemical Supercapacitors (Springer), 11–31. doi:10.1007/978-1-4757-3058-6_2
Djahnit, L., Sened, N., El-Miloudi, K., Lopez-Manchado, M. A., and Haddaoui, N. (2019). Structural Characterization and thermal Degradation of Poly(methylmethacrylate)/zinc Oxide Nanocomposites. J. Macromolecular Sci. A 56 (3), 189–196. doi:10.1080/10601325.2018.1563494
Dmitruk, N. (2010). Effect of Chemical Modification of Thin C60 Fullerene Films on the Fundamental Absorption Edge. Semiconductor physicsquantum Electron. optoelectronics 13 (2), 180–185. doi:10.15407/spqeo13.02.180
Dou, F., Silva, C., and Zhang, X. (2013). The Role of Acceptor-Rich Domain in Optoelectronic Properties of Photovoltaic Diodes Based on Polymer Blends. Chem. Phys. Lett. 583, 92–96. doi:10.1016/j.cplett.2013.07.049
Duran, B., Ünver, İ. Ç., and Bereket, G. (2020). Investigation of Supporting Electrolyte Effect on Supercapacitor Properties of Poly(Carbazole) Films. J. Electrochem. Sci. Technol. 11 (1), 41–49. doi:10.33961/jecst.2019.00129
Etxebarria, I., Ajuria, J., and Pacios, R. (2015). Polymer: Fullerene Solar Cells: Materials, Processing Issues, and Cell Layouts to Reach Power Conversion Efficiency over 10%, a Review. J. Photon. Energ. 5 (1), 057214.
Gallo, M., Favila, A., and Glossman-Mitnik, D. (2007). DFT Studies of Functionalized Carbon Nanotubes and Fullerenes as Nanovectors for Drug Delivery of Antitubercular Compounds. Chem. Phys. Lett. 447 (1-3), 105–109. doi:10.1016/j.cplett.2007.08.098
Geckeler, K. E., and Samal, S. (1999). Syntheses and Properties of Macromolecular Fullerenes, a Review. Polym. Int. 48 (9), 743–757. doi:10.1002/(sici)1097-0126(199909)48:9<743::aid-pi246>3.0.co;2-4
Giacalone, F., and Martín, N. (2006). Fullerene Polymers: Synthesis and Properties. Chem. Rev. 106 (12), 5136–5190. doi:10.1021/cr068389h
Gong, S., Li, X., Sheng, M., Liu, S., Zheng, Y., Wu, H., et al. (2021). High thermal Conductivity and Mechanical Strength Phase Change Composite with Double Supporting Skeletons for Industrial Waste Heat Recovery. ACS Appl. Mater. Inter. 13 (39), 47174–47184. doi:10.1021/acsami.1c15670
Hu, C.-C., Chang, K.-H., Lin, M.-C., and Wu, Y.-T. (2006). Design and Tailoring of the Nanotubular Arrayed Architecture of Hydrous RuO2 for Next Generation Supercapacitors. Nano Lett. 6 (12), 2690–2695. doi:10.1021/nl061576a
Huang, D. M., Moule, A. J., and Faller, R. (2011). Characterization of Polymer–Fullerene Mixtures for Organic Photovoltaics by Systematically Coarse-Grained Molecular Simulations. Fluid Phase Equilibria 302 (1-2), 21–25. doi:10.1016/j.fluid.2010.07.025
Issar, U., and Arora, R. (2021). Functionalized Carbon Nanomaterials ( FCNMs ): A Green and Sustainable Vision. Environ. Appl. Carbon Nanomaterials‐Based Devices, 395–422. doi:10.1002/9783527830978.ch17
Jayalakshmi, M., and Balasubramanian, K. (2008). Simple Capacitors to Supercapacitors-An Overview. Int. J. Electrochem. Sci. 3 (11), 1196–1217.
Jayalakshmi, M., Rao, M. M., Venugopal, N., and Kim, K.-B. (2007). Hydrothermal Synthesis of SnO2-V2o5 Mixed Oxide and Electrochemical Screening of Carbon Nano-Tubes (CNT), V2O5, V2O5-CNT, and SnO2-V2o5-CNT Electrodes for Supercapacitor Applications. J. Power Sourc. 166 (2), 578–583. doi:10.1016/j.jpowsour.2006.11.025
Jehoulet, C., Obeng, Y. S., Kim, Y. T., Zhou, F., and Bard, A. J. (1992). Electrochemistry and Langmuir Trough Studies of Fullerene C60 and C70 Films. J. Am. Chem. Soc. 114 (11), 4237–4247. doi:10.1021/ja00037a030
Kanbur, Y., and Tayfun, U. (2019). Development of Multifunctional Polyurethane Elastomer Composites Containing Fullerene: Mechanical, Damping, thermal, and Flammability Behaviors. J. Elastomers Plastics 51 (3), 262–279. doi:10.1177/0095244318796616
Kausar, A. (2017). Advances in Polymer/fullerene Nanocomposite: a Review on Essential Features and Applications. Polymer-Plastics Tech. Eng. 56 (6), 594–605. doi:10.1080/03602559.2016.1233278
Kausar, A. (2021). Fullerene Nanofiller Reinforced Epoxy Nanocomposites-Developments, Progress and Challenges. Mater. Res. Innov. 25 (3), 175–185. doi:10.1080/14328917.2020.1748794
Kim, T., Choi, J., Kim, H. J., Lee, W., and Kim, B. J. (2017). Comparative Study of Thermal Stability, Morphology, and Performance of All-Polymer, Fullerene-Polymer, and Ternary Blend Solar Cells Based on the Same Polymer Donor. Macromolecules 50 (17), 6861–6871. doi:10.1021/acs.macromol.7b00834
Kim, Y.-T., Tadai, K., and Mitani, T. (2005). Highly Dispersed Ruthenium Oxide Nanoparticles on Carboxylated Carbon Nanotubes for Supercapacitor Electrode Materials. J. Mater. Chem. 15 (46), 4914–4921. doi:10.1039/b511869g
Larcher, D., and Tarascon, J.-M. (2015). Towards Greener and More Sustainable Batteries for Electrical Energy Storage. Nat. Chem 7 (1), 19–29. doi:10.1038/nchem.2085
Lawes, S., Riese, A., Sun, Q., Cheng, N., and Sun, X. (2015). Printing Nanostructured Carbon for Energy Storage and Conversion Applications. Carbon 92, 150–176. doi:10.1016/j.carbon.2015.04.008
Li, Y., Wang, B., Chen, H., and Feng, W. (2010). Improvement of the Electrochemical Properties via Poly(3,4-Ethylenedioxythiophene) Oriented Micro/nanorods. J. Power Sourc. 195 (9), 3025–3030. doi:10.1016/j.jpowsour.2009.11.074
Libich, J., Máca, J., Vondrák, J., Čech, O., and Sedlaříková, M. (2018). Supercapacitors: Properties and Applications. J. Energ. Storage 17, 224–227. doi:10.1016/j.est.2018.03.012
Lin, J., Zhang, C., Yan, Z., Zhu, Y., Peng, Z., Hauge, R. H., et al. (2013). 3-dimensional Graphene Carbon Nanotube Carpet-Based Microsupercapacitors with High Electrochemical Performance. Nano Lett. 13 (1), 72–78. doi:10.1021/nl3034976
Liu, C., Li, F., Ma, L.-P., and Cheng, H.-M. (2010). Advanced Materials for Energy Storage. Adv. Mater. 22 (8), E28–E62. doi:10.1002/adma.200903328
Liu, K., Hu, Z., Xue, R., Zhang, J., and Zhu, J. (2008). Electropolymerization of High Stable Poly(3,4-Ethylenedioxythiophene) in Ionic Liquids and its Potential Applications in Electrochemical Capacitor. J. Power Sourc. 179 (2), 858–862. doi:10.1016/j.jpowsour.2008.01.024
Ltaief, A. (2004). Electrical and Optical Properties of Thin Films Based on MEH-PPV/fullerene Blends. Synth. met. 147 (1-3), 261–266. doi:10.1016/j.synthmet.2004.09.018
Mackay, M. E., Tuteja, A., Duxbury, P. M., Hawker, C. J., Van Horn, B., Guan, Z., et al. (2006). General Strategies for Nanoparticle Dispersion. Science 311 (5768), 1740–1743. doi:10.1126/science.1122225
Martín, N. (2006). New Challenges in Fullerene Chemistry. Chem. Commun. 37 (20), 2093–2104. doi:10.1002/chin.200638221
McCamey, D. R., Seipel, H. A., Paik, S.-Y., Walter, M. J., Borys, N. J., Lupton, J. M., et al. (2008). Spin Rabi Flopping in the Photocurrent of a Polymer Light-Emitting Diode. Nat. Mater 7 (9), 723–728. doi:10.1038/nmat2252
Modi, A., Koratkar, N., Lass, E., Wei, B., and Ajayan, P. M. (2003). Miniaturized Gas Ionization Sensors Using Carbon Nanotubes. Nature 424 (6945), 171–174. doi:10.1038/nature01777
Mohamadi, S., and Sharifi-Sanjani, N. (2018). Effect of thermal Annealing on the Crystalline Structure of PVDF/PMMA-modified Graphene Nanocomposites. J. Macromolecular Sci. Part A 55 (11-12), 775–784. doi:10.1080/10601325.2018.1528848
Mojica, M., Alonso, J. A., and Méndez, F. (2013). Synthesis of Fullerenes. J. Phys. Org. Chem. 26 (7), 526–539. doi:10.1002/poc.3121
Momodu, D., Bello, A., Dangbegnon, J., Barzeger, F., Fabiane, M., and Manyala, N. (2015). P3HT:PCBM/nickel-aluminum Layered Double Hydroxide-Graphene Foam Composites for Supercapacitor Electrodes. J. Solid State. Electrochem. 19 (2), 445–452. doi:10.1007/s10008-014-2602-0
Montellano López, A., Mateo-Alonso, A., and Prato, M. (2011). Materials Chemistry of Fullerene C60derivatives. J. Mater. Chem. 21 (5), 1305–1318. doi:10.1039/c0jm02386h
Moon, J. S., Takacs, C. J., Cho, S., Coffin, R. C., Kim, H., Bazan, G. C., et al. (2010). Effect of Processing Additive on the Nanomorphology of a Bulk Heterojunction Material. Nano Lett. 10 (10), 4005–4008. doi:10.1021/nl101923m
Mousty, C., and Leroux, F. (2012). LDHs as Electrode Materials for Electrochemical Detection and Energy Storage: Supercapacitor, Battery and (Bio)-sensor. Nanotec 6 (3), 174–192. doi:10.2174/187221012803531556
Naoi, K., Naoi, W., Aoyagi, S., Miyamoto, J.-i., and Kamino, T. (2013). New Generation “Nanohybrid Supercapacitor”. Acc. Chem. Res. 46 (5), 1075–1083. doi:10.1021/ar200308h
Nierengarten, J.-F. o. (2004). Chemical Modification of C60 for Materials Science Applications. New J. Chem. 28 (10), 1177–1191. doi:10.1039/b402661f
Pandolfo, T. (2013). “General Properties of Electrochemical Capacitors,” in Francois Béguin and Elzbieta Fr Şackowiak.
Patake, V. D., Lokhande, C. D., and Joo, O. S. (2009). Electrodeposited Ruthenium Oxide Thin Films for Supercapacitor: Effect of Surface Treatments. Appl. Surf. Sci. 255 (7), 4192–4196. doi:10.1016/j.apsusc.2008.11.005
Pech, D., Brunet, M., Durou, H., Huang, P., Mochalin, V., Gogotsi, Y., et al. (2010). Ultrahigh-power Micrometre-Sized Supercapacitors Based on Onion-like Carbon. Nat. Nanotech 5 (9), 651–654. doi:10.1038/nnano.2010.162
Peng, Z., Hu, Y., Wang, J., Liu, S., Li, C., Jiang, Q., et al. (2019). Fullerene‐Based In Situ Doping of N and Fe into a 3D Cross‐Like Hierarchical Carbon Composite for High‐Performance Supercapacitors. Adv. Energ. Mater. 9 (11), 1802928. doi:10.1002/aenm.201802928
Pereira, P., Gaspar, H., Fernandes, L., and Bernardo, G. (2015). Impact of Fullerenes on the thermal Stability of Melt Processed Polystyrene and Poly(methyl Methacrylate) Composites. Polym. Test. 47, 130–136. doi:10.1016/j.polymertesting.2015.09.004
Poizot, P., and Dolhem, F. (2011). Clean Energy New deal for a Sustainable World: from non-CO2 Generating Energy Sources to Greener Electrochemical Storage Devices. Energy Environ. Sci. 4 (6), 2003–2019. doi:10.1039/c0ee00731e
Pothu, R. (2020). Graphene-Based Materials for Self-Healable Supercapacitors. Mater. Res. Foundations 64, 167–180.
Prylutskyy, Y. I., Petrenko, V. I., Ivankov, O. I., Kyzyma, O. A., Bulavin, L. A., Litsis, O. O., et al. (2014). On the Origin of C60 Fullerene Solubility in Aqueous Solution. Langmuir 30 (14), 3967–3970. doi:10.1021/la404976k
Ramadan, A., Anas, M., Ebrahim, S., Soliman, M., and Abou-Aly, A. I. (2020). Polyaniline/fullerene Derivative Nanocomposite for Highly Efficient Supercapacitor Electrode. Int. J. Hydrogen Energ. 45 (32), 16254–16265. doi:10.1016/j.ijhydene.2020.04.093
Ravi, P., Dai, S., Wang, C., and Tam, K. C. (2007). Fullerene Containing Polymers: a Review on Their Synthesis and Supramolecular Behavior in Solution. J. Nanosci Nanotechnol 7 (4-5), 1176–1196. doi:10.1166/jnn.2007.456
Raza, W., Ali, F., Raza, N., Luo, Y., Kim, K.-H., Yang, J., et al. (2018). Recent Advancements in Supercapacitor Technology. Nano Energy 52, 441–473. doi:10.1016/j.nanoen.2018.08.013
Ryu, K. S., et al. (2004). Poly (ethylenedioxythiophene)(PEDOT) as Polymer Electrode in Redox Supercapacitor. Electrochimica acta 50 (2-3), 843–847. doi:10.1016/j.electacta.2004.02.055
Schon, T. B., DiCarmine, P. M., and Seferos, D. S. (2014). Polyfullerene Electrodes for High Power Supercapacitors. Adv. Energ. Mater. 4 (7), 1301509. doi:10.1002/aenm.201301509
Sen, P., De, A., Chowdhury, A. D., Bandyopadhyay, S. K., Agnihotri, N., and Mukherjee, M. (2013). Conducting Polymer Based Manganese Dioxide Nanocomposite as Supercapacitor. Electrochimica Acta 108, 265–273. doi:10.1016/j.electacta.2013.07.013
Sen, P., and De, A. (2010). Electrochemical Performances of Poly(3,4-Ethylenedioxythiophene)-NiFe2O4 Nanocomposite as Electrode for Supercapacitor. Electrochimica Acta 55 (16), 4677–4684. doi:10.1016/j.electacta.2010.03.077
Senthilkumar, B. (2015). Synthesis and Characterization of Manganese Molybdate for Symmetric Capacitor Applications. Int. J. Electrochem. Sci. 10 (1), 185–193.
Shen, T., Chang, Z., Liu, X., Chen, Q., and Feng, L. (2021). Palladium Complex Composites Based on Fullerene Encapsulated in Porous Zinc Porphyrin Polymers. J. Macromolecular Sci. Part A 58, 1–8. doi:10.1080/10601325.2021.1964369
Shrestha, R. G., Shrestha, L. K., and Ariga, K. (2021). Carbon Nanoarchitectonics for Energy and Related Applications. C 7 (4), 73. doi:10.3390/c7040073
Simon, P., and Gogotsi, Y. (2010). “Materials for Electrochemical Capacitors,” in Nanoscience and Technology: A Collection of Reviews from Nature Journals (World Scientific), 320–329. doi:10.1142/9789814317665_0021
Simon, P., and Gogotsi, Y. (2010). Materials For Electrochemical Capacitors. Nanoscience and Technology: A Collection of Reviews from Nature Journals, 320–329.
Smith, S. C., Sen, P., and Kroposki, B. (2008). “Advancement of Energy Storage Devices and Applications in Electrical Power System,” in 2008 IEEE Power and Energy Society General Meeting-Conversion and Delivery of Electrical Energy in the 21st Century (IEEE). doi:10.1109/pes.2008.4596436
Snook, G. A., Peng, C., Fray, D. J., and Chen, G. Z. (2007). Achieving High Electrode Specific Capacitance with Materials of Low Mass Specific Capacitance: Potentiostatically Grown Thick Micro-nanoporous PEDOT Films. Electrochemistry Commun. 9 (1), 83–88. doi:10.1016/j.elecom.2006.08.037
Sobarzo, P. A., Mariman, A. P., Sánchez, C. O., Hauyon, R. A., Rodríguez-González, F. E., Medina, J., et al. (2021). Comparison between Poly(azomethine)s and Poly(p-Phenylvinylene)s Containing a Di-R-diphenylsilane (R = Methyl or Phenyl) Moiety. Optical, Electronic and thermal Properties. Eur. Polym. J. 159, 110714. doi:10.1016/j.eurpolymj.2021.110714
Song, S., Hill, R., Choi, K., Wojciechowski, K., Barlow, S., Leisen, J., et al. (2018). Surface Modified Fullerene Electron Transport Layers for Stable and Reproducible Flexible Perovskite Solar Cells. Nano Energy 49, 324–332. doi:10.1016/j.nanoen.2018.04.068
Stenger-Smith, J. D., Webber, C. K., Anderson, N., Chafin, A. P., Zong, K., and Reynolds, J. R. (2002). Poly(3,4-alkylenedioxythiophene)-Based Supercapacitors Using Ionic Liquids as Supporting Electrolytes. J. Electrochem. Soc. 149 (8), A973–A977. doi:10.1149/1.1485773
Thota, S., Jeon, S., Wang, M., and Chiang, L. Y. (2010). Synthesis of Cationic Dumbbell-Shaped Fullerene Nanostructures as Potential Photodynamic Sensitizers. J. Macromolecular Sci. Part A 47 (12), 1184–1190. doi:10.1080/10601325.2010.518838
Tumbleston, J. R., Yang, L., You, W., and Ade, H. (2014). Morphology Linked to Miscibility in Highly Amorphous Semi-conducting Polymer/fullerene Blends. Polymer 55 (19), 4884–4889. doi:10.1016/j.polymer.2014.07.051
Umeda, T. (2005). Improvement of Characteristics on Polymer Photovoltaic Cells Composed of Conducting Polymer-Fullerene Systems. Synth. met. 152 (1-3), 93–96. doi:10.1016/j.synthmet.2005.07.172
Villers, D., Jobin, D., Soucy, C., Cossement, D., Chahine, R., Breau, L., et al. (2003). The Influence of the Range of Electroactivity and Capacitance of Conducting Polymers on the Performance of Carbon Conducting Polymer Hybrid Supercapacitor. J. Electrochem. Soc. 150 (6), A747–A752. doi:10.1149/1.1571530
Wang, H., Yan, X., and Piao, G. (2017). A High-Performance Supercapacitor Based on Fullerene C 60 Whisker and Polyaniline Emeraldine Base Composite. Electrochimica acta 231, 264–271. doi:10.1016/j.electacta.2017.02.057
Wang, J., Xu, Y., Chen, X., and Du, X. (2007). Electrochemical Supercapacitor Electrode Material Based on Poly(3,4-Ethylenedioxythiophene)/polypyrrole Composite. J. Power Sourc. 163 (2), 1120–1125. doi:10.1016/j.jpowsour.2006.10.004
Wang, Y. Z., Wang, Q., Xie, H. Y., Ho, L. P., Tan, D. M. F., Diao, Y. Y., et al. (2012). Fabrication of Highly Ordered P3HT:PCBM Nanostructures and its Application as a Supercapacitive Electrode. Nanoscale 4 (12), 3725–3728. doi:10.1039/c2nr30486d
Wang, Z., Zhu, M., Pei, Z., Xue, Q., Li, H., Huang, Y., et al. (2020). Polymers for Supercapacitors: Boosting the Development of the Flexible and Wearable Energy Storage. Mater. Sci. Eng. R: Rep. 139, 100520. doi:10.1016/j.mser.2019.100520
White, A. M., and Slade, R. C. T. (2004). Electrochemically and Vapour Grown Electrode Coatings of Poly(3,4-Ethylenedioxythiophene) Doped with Heteropolyacids. Electrochimica acta 49 (6), 861–865. doi:10.1016/j.electacta.2003.10.003
Winter, M., and Brodd, R. J. (2004). What Are Batteries, Fuel Cells, and Supercapacitors? ACS Publications.
Withers, J. C., Loutfy, R. O., and Lowe, T. P. (1997). Fullerene Commercial Vision. Fullerene Sci. Tech. 5 (1), 1–31. doi:10.1080/15363839708011971
Wu, N.-L. (2002). Nanocrystalline Oxide Supercapacitors. Mater. Chem. Phys. 75 (1-3), 6–11. doi:10.1016/s0254-0584(02)00022-6
Xiong, S., Phua, S. L., Dunn, B. S., Ma, J., and Lu, X. (2010). Covalently Bonded Polyaniline−TiO2 Hybrids: A Facile Approach to Highly Stable Anodic Electrochromic Materials with Low Oxidation Potentials. Chem. Mater. 22 (1), 255–260. doi:10.1021/cm903058c
Xiong, S., Yang, F., Jiang, H., Ma, J., and Lu, X. (2012). Covalently Bonded Polyaniline/fullerene Hybrids with Coral-like Morphology for High-Performance Supercapacitor. Electrochimica acta 85, 235–242. doi:10.1016/j.electacta.2012.08.056
Yin, G.-Z., Díaz Palencia, J. L., and Wang, D.-Y. (2021). Fully Bio-Based Poly (Glycerol-Itaconic Acid) as Supporter for PEG Based Form Stable Phase Change Materials. Composites Commun. 27, 100893. doi:10.1016/j.coco.2021.100893
Yin, G.-Z., Yang, X.-M., Hobson, J., López, A. M., and Wang, D.-Y. (2022). Bio-based Poly (Glycerol-itaconic acid)/PEG/APP as Form Stable and Flame-Retardant Phase Change Materials. Composites Commun. 30, 101057. doi:10.1016/j.coco.2022.101057
Yoshino, K., Kajii, H., Araki, H., Sonoda, T., Take, H., and Lee, S. (1999). Electrical and Optical Properties of Conducting Polymer - Fullerene and Conducting Polymer - Carbon Nanotube Composites. Fullerene Sci. Technol. 7 (4), 695–711. doi:10.1080/10641229909351371
Zabihi, F., Chen, Q., Xie, Y., and Eslamian, M. (2016). Fabrication of Efficient Graphene-Doped Polymer/fullerene Bilayer Organic Solar Cells in Air Using Spin Coating Followed by Ultrasonic Vibration post Treatment. Superlattices and Microstructures 100, 1177–1192. doi:10.1016/j.spmi.2016.10.087
Zhang, K., Zhang, L. L., Zhao, X. S., and Wu, J. (2010). Graphene/polyaniline Nanofiber Composites as Supercapacitor Electrodes. Chem. Mater. 22 (4), 1392–1401. doi:10.1021/cm902876u
Zhao, H., Yu, Y., Han, C., Liu, Q., Liu, H., Zhou, G., et al. (2021). Improving the Stereocomplexation and Toughness of poly(L-Lactic acid)/poly(D-Lactic Acid) Blends via Melt Blending with Ethylene/methyl Acrylate/glycidyl Methacrylate Terpolymer. J. Macromolecular Sci. Part A 58 (6), 419–430. doi:10.1080/10601325.2021.1873071
Keywords: polymer, fullerene, nanocomposite, conductivity, supercapacitor
Citation: Kausar A (2022) Fullerene Reinforced Polymeric Nanocomposites for Energy Storage—Status and Prognoses. Front. Mater. 9:874169. doi: 10.3389/fmats.2022.874169
Received: 11 February 2022; Accepted: 23 February 2022;
Published: 09 March 2022.
Edited by:
Patricia Krawczak, Institut Mines-Télécom, FranceReviewed by:
José Luis Díaz Palencia, Universidad Francisco de Vitoria, SpainGuilherme Mariz De Oliveira Barra, Federal University of Santa Catarina, Brazil
Copyright © 2022 Kausar. This is an open-access article distributed under the terms of the Creative Commons Attribution License (CC BY). The use, distribution or reproduction in other forums is permitted, provided the original author(s) and the copyright owner(s) are credited and that the original publication in this journal is cited, in accordance with accepted academic practice. No use, distribution or reproduction is permitted which does not comply with these terms.
*Correspondence: Ayesha Kausar, dr.ayeshakausar@yahoo.com