- 1School of Civil Engineering, North China University of Technology, Beijing, China
- 2Research Institute of Highway Ministry of Transport, Beijing, China
- 3School of Civil Engineering, Chongqing University, Chongqing, China
- 4Central Research Institute of Building and Construction Co., Ltd., MCC Group, Beijing, China
To provide references for the use of fabricated thin-walled cold-formed steel tubular lightweight concrete columns, the axial compression tests were conducted on single-limb and double-limb specimens. The results were compared with hollow specimens to analyze the effects of the cross-sectional size of the members, wall thickness, and steel ratio of the sections on the axial load-bearing capacity of the specimens. The test results show the following: 1) under the axial load, the typical damage of the specimens is local buckling of the steel tube and local crushing of the concrete. 2) The ultimate bearing capacity of the double-limb specimen is the highest. For the specimen with the sectional height-width ratio of 1.43, the ultimate bearing capacity of the double-limb specimen is 275.71% higher than that of the hollow steel tubular column and 241.49% that of single-limb specimens. 3) Based on the material superposition strength theory, the calculating method of the axial load-bearing capacity of thin-walled cold-formed steel tubular lightweight concrete columns was proposed and verified by comparing with the test results, which provides the design guidance for such members.
1 Introduction
Compared with the traditional reinforced concrete structure, the fabricated thin-walled cold-formed steel tubular lightweight concrete columns have the advantages of high bearing capacity, ready construction, and good economic efficiency (Cai, 2003). The steel tube and concrete work together in the fabricated thin-walled cold-formed steel tubular lightweight concrete columns, which can effectively delay the buckling of the steel tube wall, enhance the ductility and load-bearing capacity of the members, and make the structures have good seismic performance. Therefore, the fabricated thin-walled cold-formed steel tubular lightweight concrete columns have been widely used in building and bridge engineering (Wright, 1993).
At present, the mechanical properties of thin-walled steel tube concrete columns have been studied to some extent. Ge and Usami (1992) conducted horizontal low cyclic load tests on thin-walled square steel tube concrete columns and hollow steel tubes to investigate the ultimate bearing capacity and the deformation capacity of the thin-walled steel tube concrete structure. Mursi and Uy (2004) conducted experimental and numerical simulation studies on high-strength thin-walled steel tube concrete columns to investigate the main factors affecting the overall instability and local buckling of such members. Varma et al. (2004) analyzed the effects of axial compression ratio and width-to-thickness ratio on the hysteretic properties of high-strength concrete columns with square steel tubes, and the results showed that the horizontal bearing capacity of the specimens decreased with the increase of axial compression ratio. Skalomenos et al. (2016) conducted horizontal load tests on circular thin-walled steel tube concrete columns and found that the yield strength of the steel had a significant effect on the ductility and horizontal bearing capacity of the specimens. Zhao et al. (2008) found that the steel tube had an obvious constraining effect on the core concrete and discussed the calculating conditions for the bearing capacity of concrete-filled cold-formed steel tubular columns by the axial compression formula of concrete-filled ordinary steel tubular columns. Li (2017) conducted the axial compression experiment of high-strength concrete-filled cold-formed steel tube columns and obtained the calculation formula of the bearing capacity. Islam et al. (2021) studied the effect of concrete strength, the width-to-thickness ratio, and the height-to-width ratio of the section on the bearing capacity and ductility of the cold-formed concrete-filled steel tubular columns. Zhang et al. (2005) analyzed the effect of concrete types on the load-bearing capacity of lightweight concrete steel tubular columns and found that the higher the concrete strength, the higher the load-bearing capacity of steel tube lightweight concrete columns. Chen (2007) found that the reinforcement ratio could improve the flexural performance of light aggregate concrete steel tubes. Li and Liu (2001) investigated the type of cross section on the constraint performance of the tube on the infilled concrete and found the round tube had the best performance.
The current research mostly focuses on steel tube concrete and thin-walled steel tube concrete structure members, while the research on the fabricated thin-walled cold-formed steel tubular lightweight concrete columns is not yet perfect, and the existing code lacks the relevant provisions for the design and calculation of such members. Therefore, the axial load test was carried out for the fabricated thin-walled cold-formed steel tubular lightweight concrete columns. The main factors affecting the bearing capacity of the members were analyzed, and the calculation method for the bearing capacity of the members was proposed, which would provide support for the application of such members.
2 Design of Axial Compressive Load Test
2.1 Specimen Design and Fabrication
According to the Chinese Technical Code GB50936 (Zha et al., 2014), the axial compressive load test of single-limb and double-limb specimens was designed and compared with the hollow specimens. The dimensions of the specimen are shown in Figure 1. The material characterization tests were performed in order to obtain the real mechanical properties of steel and concrete concerned in this work. The specimens were made of Q390 weathering steel with the yield stress fy 390 MPa and the ultimate stress fu 490 MPa, produced into C-shape by the plate bender. Then, the steel plate was connected to the C-shape steel with an ST4.8 tapping screw to form a closed tube. The coarse and fine aggregates in the lightweight concrete were grade 900 spherical shale ceramic granules and expanded perlite granules, respectively, which were mixed with reference to the Chinese Technical Standard JGJ/T 12 (Ding et al., 2019). The concrete strength fcu with 16.7 MPa was consistent with the C35 grade, which met the minimum concrete strength requirement of C30 in the Chinese Technical Code GB50936 (Zha et al., 2014). The steel ratio is the percentage of the steel area to the concrete area. The design parameters of the specimen are shown in Table 1.
2.2 Test Loading Scheme and Measurement Point Arrangement
The test was carried out on the electro-hydraulic servo testing machine. A pre-load 5 kN was imposed on the top of the specimen to eliminate tiny gaps between specimens and the loading machine firstly. After that, the displacement control model was used, and the loading rate was 0.5 mm/s during formal loading. In order to measure the axial compression deformation of the specimen and the deflection in the middle of the specimen, axial and transverse displacement gauges were arranged at the top and 1/2 height of the specimen, respectively. The test loading and displacement gauges were arranged as shown in Figure 2A. In order to ensure the uniform force at the end of the specimens and restrain the horizontal displacement, two customized head plates were installed at both ends, as shown in Figure 2B. The test was terminated when the load was reduced to 85% of the ultimate load or the specimen was seriously damaged. At the same time, strain gauges were arranged on the outer surface of the cold-formed thin-walled steels tube. The measurement points are shown in Figure 3.
3 Analysis of Test Results
3.1 Specimen Damage Phenomenon
Under the axial load, both the steel tube and the concrete of the specimens were subjected to axial pressure. As the load increases, the lateral deformation of the concrete increases continuously. Once the lateral deformation of the concrete exceeds that of the steel tube, the steel tube will constrain the core concrete, which will increase the transverse stress of the steel tube and the radial pressure of the concrete.
When the load was close to the ultimate bearing capacity of the single-limb specimen, the sealing steel plate buckled between the tapping screws at the upper end of the specimen. After reaching the ultimate load, the bearing capacity of the specimen dropped rapidly, the C-shape steel also bulged outward rapidly, and the test was terminated. In the bulging part of the specimen after being opened, it could be found that the concrete in the section was crushed, but the concrete on the center was not damaged. The rivets were not detached in the test. The damage phenomenon is shown in Figure 4.
For the double-limb column specimen, a small deformation of the sealing steel plate appeared first at the beginning of loading. When reaching the ultimate bearing capacity of the specimen, the bulging damage occurred at the top of the specimen. The steel plate was buckled outward and the rivets were pulled out. The damage of the specimen is shown in Figure 5.
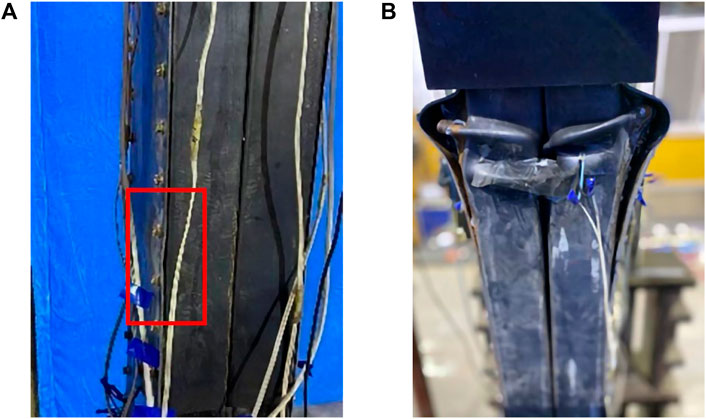
FIGURE 5. (A) Local deformation of specimen steel plate of SR50-2.2. (B) Specimen buckling of SR50-2.2.
The hollow specimen in the early loading stage had no obvious damage until close to the ultimate bearing capacity; the upper of the steel tube near the end caused rapid inward buckling. The test was terminated, and the rivets were not pulled out during the loading. The damage of the specimen is shown in Figure 6.
3.2 Load-Strain Curves of Specimens
Figure 7 shows the load-strain curves in the middle of specimens. At the beginning of loading, the specimens were uniformly compressed, and the strains increased simultaneously in the same direction with the load. As the load increases, local buckling appeared on the specimens before the ultimate damage of the specimens. At the same time, the bearing capacity of the double-limb specimen was greater than that of the single-limb specimen, which was greater than that of the hollow tubular specimen.
3.3 Load and Deformation Relationship of Specimens
Figure 8 shows the load-displacement curves of the specimens. It can be found that the ultimate bearing capacity of the double-limb specimen is about two times that of the single-limb specimen due to the restraint of the steel tube on the concrete and the inhibition of concrete on the buckling effect of the steel section. The specimen cross section size has a greater influence on the bearing capacity of the same limb column. However, the greater the wall thickness, the higher the bearing capacity of the specimen. The bearing capacity of the hollow specimen is influenced by the wall thickness of the steel tube. When the wall thickness of the steel tube is larger, the local buckling damage of the specimen appears relatively late, and thus the bearing capacity continues to grow.
Meanwhile, the specimens were divided into four groups according to the steel ratio. The ductility coefficient
where ε85% is the average vertical strain when the load drops to 85% of the ultimate load-bearing capacity of the specimen and εu is the average vertical strain when the load reaches the ultimate load-bearing capacity of the specimen. If the load did not drop to 85% of the peak load, the final failure point was defined as the symmetry point, which was reflected to 85% of the peak load in the rising part of the load-displacement curve (Lue et al., 2007).
The ductility coefficients of the specimens are shown in Figure 9. It can be found that the filled concrete greatly improves the ultimate bearing capacity of the specimen. For the specimens with the sectional height-width ratio of 1.43, the ultimate bearing capacity of the single-limb column specimen is 114.17% higher than that of the hollow steel tube column, and the ultimate bearing capacity of the double-limb column specimen is 275.71% higher than that of the hollow steel tube column. The steel ratio has little effect on the ductility coefficient of the specimens with the same limb.
4 Bearing Capacity Calculation Method of Fabricated Cold-Formed Thin-Walled Steel Tubular Concrete Columns
The existing specification lacks provisions for the design of fabricated thin-walled cold-formed steel tubular lightweight concrete columns. Therefore, according to the comprehensive comparison of Japanese Design Code (AIJ, 1997), Chinese Design Code GJB4142-2000 (Wei et al., 2000), engineering construction standard of Fujian province DBJB-51-2003 (Tao et al., 2003), CECS159-2004 (Shen et al., 2004), and Chinese concrete-filled steel tubular structure code GB50936-2014 (Zha et al., 2014) on the calculation method of the bearing capacity of steel tube concrete under compression, the ultimate bearing capacities of the specimens in the test were compared with the design value of the specifications. The results are shown in Table 2. It can be found that the ultimate bearing capacities of the specimens were 2.55∼4.26 times the design value of the concrete-filled steel tube (CFST) members. Compared with CFST, the cold-formed steel specimens have the advantages of lightweight, fewer welds, and convenient construction.
Based on the superimposed strength theory of steel tube concrete, the formula for calculating the design value of the cross-sectional bearing capacity is proposed when the fabricated thin-walled cold-formed steel tubular lightweight concrete columns are axially compressed as follows:
where kc is the concrete strength correction factor, related to the casing hoop steel coefficient ξ. fy and fc are the design values of steel and concrete strengths, respectively. Ac and As are the core concrete and steel tube cross-sectional areas. αsc is the steel ratio which is the percentage of the steel area to the concrete area.
The regression analysis of kc and ξ (shown in Figure 10) was performed according to the test results to obtain the expression of the concrete strength reduction coefficient kc, which is shown in Eq. 4, where the correlation coefficient
Therefore, the compressive bearing capacity of the fabricated thin-walled cold-formed steel tubular lightweight concrete columns can be calculated as follows:
The comparing results of the bearing capacity Nu obtained based on Eq. 5 with the test results Nexp are shown in Table 3. It can be found that the error Nu/Nexp is around 1, which indicates the calculation method is fitted.
5 Conclusion
The main factors affecting the bearing capacity and ductility performance of the combined columns were investigated through the axial compression load test on 12 fabricated thin-walled cold-formed steel tubular lightweight concrete columns. Based on the existing steel tubular concrete column design code, the load-bearing capacity calculation formula adapted to the cold-formed thin-walled steel-lightweight concrete column was proposed. The main conclusions are as follows:
(1) In the loading process, all specimens did not experience out-of-plane instability during the test. The local bucking is the main damage mode. The ultimate bearing capacity of the double-limb specimen is significantly higher than that of the single-limb specimen and hollow steel tube.
(2) For the specimen with the height-to-width ratio of 1.43, the ultimate bearing capacity of the single-limb specimen is 114.17% higher than that of the hollow steel tube column, and the ultimate bearing capacity of the double-limb column is 275.71% higher than that of hollow steel tube column. For the specimens with the same limb, the steel ratio has a large influence on the bearing capacity and little influence on the ductility coefficient.
(3) Based on the superimposed strength theory, the load-bearing capacity of the fabricated thin-walled cold-formed steel tubular lightweight concrete columns is proposed, which guides the design of such structure members.
Data Availability Statement
The raw data supporting the conclusion of this article will be made available by the authors without undue reservation.
Author Contributions
YL contributed to the conception and design of the study. KZ organized the database. XL performed the statistical analysis. YY wrote the first draft of the manuscript. CG wrote sections of the manuscript. All authors contributed to manuscript revision and read and approved the submitted version.
Conflict of Interest
Author XL was employed by the company Central Research Institute of Building and Construction Co., Ltd.
The remaining authors declare that the research was conducted in the absence of any commercial or financial relationships that could be construed as a potential conflict of interest.
Publisher’s Note
All claims expressed in this article are solely those of the authors and do not necessarily represent those of their affiliated organizations, or those of the publisher, the editors and the reviewers. Any product that may be evaluated in this article, or claim that may be made by its manufacturer, is not guaranteed or endorsed by the publisher.
References
AIJ (1997). Recommendations for Design and Construction of concrete Filled Steel Tubular structuresAIJ: 1997. Tokyo: Architectural Institute of Japan.
Chen, J. S. (2007). Experimental Study and Theoretical Analysis of Flexural Performance of Lightweight Aggregate concrete Filled Steel Tubes. Nanjing: Hehai University. [dissertation/master’s thesis]. doi:10.7666/d.y1031016
Ding, W., Zhu, A. P., and Wang, J. (2019).Technical Standard for Application of Lightweight Aggregate concrete Ministry of Construction of the People's Republic of China. JGJ/T 12-2019 (in Chinese).
Ge, H., and Usami, T. (1992). Strength of Concrete‐Filled Thin‐Walled Steel Box Columns: Experiment. J. Struct. Eng. 118, 3036–3054. doi:10.1061/(asce)0733-9445(1992)118:11(3036)
Islam, M. M., Ali, R. B., Begum, M., and Rahman, M. S. (2021). Experimental Study of Square Concrete-Filled Welded Cold-Formed Steel Columns under Concentric Loading. Arab J. Sci. Eng. 46, 4225–4237. doi:10.1007/s13369-020-04797-9
Li, D. (2017). Study on the Mechanical Properties of concrete Filled High Strength Cold-Formed Steel Tubular Columns. Jingzhou City, Hubei Province (China): Yangtze University, 24–60. [dissertation/master’s thesis]
Li, G. C., and Liu, Z. Y. (2001). Self-stressing Steel Tube Lightweight Aggregate concrete Structure. Shenyang: North eastern University Press.
Lue, D. M., Liu, J.-L., and Yen, T. (2007). Experimental Study on Rectangular CFT Columns with High-Strength concrete. J. Constructional Steel Res. 63 (1), 37–44. doi:10.1016/j.jcsr.2006.03.007
Mursi, M., and Uy, B. (2004). Strength of Slender concrete Filled High Strength Steel Box Columns. J. Constructional Steel Res. 60 (12), 1825–1848. doi:10.1016/j.jcsr.2004.05.002
Shen, Z. Y., Lv, X. L., He, B. K., Zhang, S. M., Cheng, Y. Y., Cheng, G. J., et al. (2004). Technical Regulations for Rectangular Steel Tube concrete Structures]. Shanghai City (China): China association of engineering construction standards. CECS159-2004(in Chinese).
Skalomenos, K. A., Hayashi, K., and Nishi, R. (2016). Experimental Behavior of concrete-filled Steel Tube Columns Using Ultra High-Strength Steel. J. Struct. Eng. J. 45 (2), 285–288. doi:10.1061/(ASCE)ST.1943-541X.0001513
Tao, Z., Chen, H., Yu, Q., Wang, Z. B., Lu, W. H., Lu, H., et al. (2003). Technical Specification for Steel Tube concrete Structure. Fuzhou City, Fujian Province (China): Department of housing construction of Fujian province. DBJB-51-2003 (in Chinese).
Varma, A. H., Ricles, J. M., Sause, R., and Lu, L.-W. (2004). Seismic Behavior and Design of High-Strength Square Concrete-Filled Steel Tube Beam Columns. J. Struct. Eng. 130 (2), 169–179. doi:10.1016/S0143-974X(01)00099-210.1061/(asce)0733-9445(2004)130:2(169)
Wei, Z. B., Han, L. H., Liu, X. L., Zhang, S. Y., Chen, H. F., Tao, Z., et al. (2000). Wartime Military Port Emergency Repair Early-Strength Combined Structure Technical Regulations, GJB4142. Beijing City (China): The General Logistics Department of the Chinese People's Liberation Army
Yang, J. (2012). Research on the Axial Compression Performance of Steel Tube-Recycled concrete Short Columns. Quanzhou City, Fujian Province (China): Huaqiao University. [dissertation/master’s thesis]. [Quanzhou(350000)]. doi:10.7666/d.D259861
Zha, X. X., Xiao, C. Z., Zhong, S. T., Cai, S. H., Fan, X. Q., Wang, Q., et al. (2014). Technical Code for concrete-filled Steel Tube Structure. Beijing City (China): Ministry of housing and urban rural development of the People’s Republic of China. GB50936- 2014 (in Chinese).
Zhang, Y. C., Wang, Q. P., and Mao, X. Y. (2005). Experimental Study on the Axial Compression Performance of Thin-Walled concrete-filled Steel Tube Short Columns. Building Struct. 35 (1), 22
Keywords: thin-walled steel tube, lightweight concrete, composite section, axial compression performance, Experiment
Citation: Li Y, Yan Y, Meng X, Zhang K, Li X and Gao C (2022) Experimental Study on Axial Compression Performance of Thin-Walled Steel Tube Lightweight Concrete Columns With Composite Section. Front. Mater. 9:858935. doi: 10.3389/fmats.2022.858935
Received: 20 January 2022; Accepted: 18 February 2022;
Published: 15 March 2022.
Edited by:
Fangying Wang, University of Nottingham, United KingdomReviewed by:
An He, South China University of Technology, ChinaYong Zhu, Harbin Institute of Technology, China
Luciano De Lima, Rio de Janeiro State University, Brazil
Copyright © 2022 Li, Yan, Meng, Zhang, Li and Gao. This is an open-access article distributed under the terms of the Creative Commons Attribution License (CC BY). The use, distribution or reproduction in other forums is permitted, provided the original author(s) and the copyright owner(s) are credited and that the original publication in this journal is cited, in accordance with accepted academic practice. No use, distribution or reproduction is permitted which does not comply with these terms.
*Correspondence: Yue Li, liyue@ncut.edu.cn; Kechao Zhang, kechao.zhang@ctvic.cn