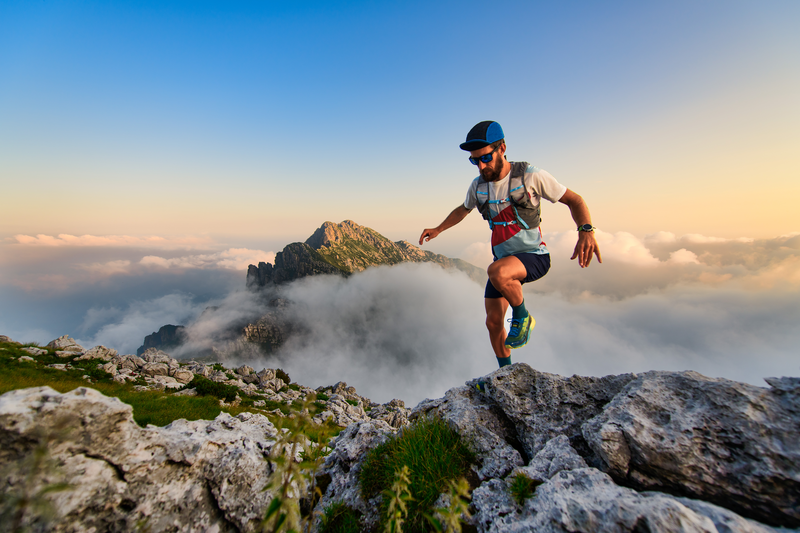
94% of researchers rate our articles as excellent or good
Learn more about the work of our research integrity team to safeguard the quality of each article we publish.
Find out more
REVIEW article
Front. Mater. , 27 April 2022
Sec. Polymeric and Composite Materials
Volume 9 - 2022 | https://doi.org/10.3389/fmats.2022.855727
The development of green, eco-friendly and sustainable packaging materials is a major challenge in the field of nanoscience and nanotechnology. Advancements in the designing biopolymeric nanocomposites for packaging applications have been established by considering features like biodegradability, environmental friendliness, mechanical properties, cost, safety, etc. Other properties such as thickness, weight, transparency, practicability, recyclability, antimicrobial, porosity, leaching effect, light protective capability, etc. need to be considered during the fabrication of next-generation packing materials for multifaceted applications. Of these, the ultraviolet (UV) protective properties of the designed nanocomposites for sustainable packaging applications are extremely important. The UV protective properties of the packaging materials can be modulated by adding a minute amount of biocompatible nanomaterials with maximum absorption properties in the UV region (200–400 nm). UV light absorption is very important to avoid loss or changes in intrinsic chemical, physical, and biological properties/content of foods, pharmaceuticals, beverages, and so forth during storage and transportation. In the current review, we highlight the principle of UV light protection, the selection of nanomaterials, and the design of nanocomposite films, including the properties and applications of biopolymeric nanocomposite materials for UV protective sustainable packaging. Lastly, the current challenges and prospects of sustainable UV protective packaging are also discussed to provide insights into the future of next-generation packaging technologies.
On the Earth, Sunlight is scattered in specific infrared, visible, and ultraviolet (UV) light rays. In addition, the massive depletion of the ozone layer in the atmosphere over the past decades by various human activities has led to an extensive increase in UV radiation (Zhao et al., 2018). The concern of ultraviolet radiation directly affect human health, both positively and negatively. Even ultraviolet radiation can be harmful to humans and cause skin aging and cancer, erythema, DNA damage, and cataracts (Su and Chen, 2018). Ultraviolet radiation is harmful due to discoloration, yellowing, and deterioration of the mechanical properties of organic materials including polymers and wood, etc. (Gijsman et al., 1999; Xiao et al., 2015; Zhou and Tang, 2017). In addition, UV absorption by dairy food products can significantly alter the flavors, color, and taste along with the loss of nutrients (Mestdagh et al., 2005). For chlorophyll synthesis in plants, red and blue lights play a significant role, while UV light from sunlight cannot be utilized directly (Poudel et al., 2008), this could be applied to UV radiation protection, with great potential in the field of human healthcare, food industries, and agriculture. UV protecting film is required by ordinary people, scientists, and industries (Yang et al., 2020; Sirerol et al., 2015). Due to a continuous increase in demand in human healthcare and biological systems, high efficient UV blocking materials have attracted considerable research attention (Gause and Chauhan, 2015; Qu et al., 2014; Wu et al., 2013; Feng et al., 2017). UV light protection film is composed of ultraviolet radiation blocking inhibitors or light protection components and a polymer matrix, where the UV light radiation protecting component acts as a key role player (You et al., 2016). However, the conventional organic light conversion components viz. triphenyl acrylonitrile (Qi et al., 2016) and perylene diimides (Yu Y. et al., 2018) are toxic and unstable under light (Wu X. et al., 2019). Due to the lack of efficiency and applicability of organic components, various types of inorganic fillers in the form of oxides such as SiO2, TiO2, CeO2, ZnO, Fe2O3, minerals (e.g. CaCO3, clays), semiconductors (e.g. CdS, PbS, CdTe, CdSe), metals and metal alloys (e.g. Ag, Cu, Au, Fe, Ge) are presently intermixed into the polymer matrices (Li et al., 2010). Inorganic fillers and ZnO nanoparticles have shown remarkable optical properties along with chemical stability, low cost, and environmentally friendly nature (Look, 2001). However, the blended effect of both organic and inorganic molecules can motivate a brand new pathway for novel materials that makes a specialty of their desired properties together with the right dispersion and high content material in nanocomposites at the side of low cost and large scale nanoparticle preparation but the organic−inorganic nanocomposite improvement is still beneath development levels (Balazs et al., 2006; Mackay et al., 2006). It has been reported that the poor dispersion of nanoparticles (NPs) with matrix is due to particle aggregation, which can lead to reduced performance and composites fragility (Huang et al., 2018). Due to the low affinity of nanoparticles with the matrix, the intrinsic properties can also be degraded. Despite this, the amalgamation of NPs into the polymer matrix without any aggregation is still a challenging task (Loste et al., 2019). It is important to obtain optically clear polymer-based functional nanocomposites either by nanoparticle dispersion or monodispersity for a variety of applications such as flexible displays, thin films, transistors (Yang et al., 2016), solar cells (Kim et al., 2015), light emitting diodes (Saurakhiya et al., 2014; Bae et al., 2016), and sensible windows (Bae et al., 2016), etc. Transparency in film formation during the introduction of particles into a polymer matrix is the main physical property, which can be better achieved by using nanoparticles.
As per the environmental accumulation of plastics in the surrounding areas and water bodies, waste management, and public concerns, synthetic plastic packaging materials need to be replaced by new environmentally sustainable packaging materials. Biopolymers are an alternative source of petroleum-based synthetic polymers such as polypropylene (PP), Polyethylene (PE), polyethylene terephthalate (PET), and polyvinyl chloride (PVC), etc. Generally, polymers are classified into synthetic, semi-synthetic, and natural groups. Figure 1 shows the types of polymers with examples. Indeed, biopolymers have significant advantages in overcoming the environmental problems caused by synthetic polymers.
Biopolymers are polymeric biomolecules that are made from natural sources such as plant materials, animal products, and microbial products and chemically synthesized from natural sources (Basumatary et al., 2020). Figure 2 shows the different sources of biopolymers with their examples.
Due to the non-toxicity of biopolymers, low cost and increasing interest in environmental compatibility, their properties and diversity are further expanding and have become a focus in developing wider industrial applications. Figure 3 shows the biodegradability and sources of polymers and biopolymers (Helanto et al., 2019; Philp et al., 2013; Puls et al., 2011). The inculsion of nanomaterials in biopolymers plays an important role in the improvement of their physical properties such as mechanical strength, barrier properties, thermal properties and applications properties widely based on applications (Sharma et al., 2018; Xiong et al., 2018). Nanocomposite materials based on organic and inorganic nano-fillers have been extensively studied to improve bio-polymer based films properties in various applications. The solution casting film preparation technique is the simplest method for nanocomposite-based film preparation as compared to extrusion and layer-by-layer techniques. Yang et al. (2020) have developed a sustainable light conversion nanocomposite film by incorporating carbon dots (CDs) as light conversion regents and nano reinforcements as a matrix in carboxymethyl xylan. It was reported from the result that the addition of carbon dots not only enhances the mechanical properties of film but also improves the optical properties, tensile strength and elastic modulus of the film. Goudarzi et al. (2017) studied the effect of different nano-TiO2 content on eco-friendly starch film for investigation of various properties such as physical, thermal, mechanical, UV transmittance and water-vapor permeability. It has been reported that the Young’s modulus and tensile strength were reduced with increase in TiO2 loading while elongation at break was increased. It was also observed that thermal properties including glass transition temperature and melting point were increased and decreased respectively by increasing TiO2 loading.
FIGURE 3. Biodegradability and sources of polymers and biopolymers (Reconstructed from. Helanto et al. (2019)).
Lignin is a phenolic bio-polymer obtained from pulp and paper industries as a by-product and also from bio-refining operations (Sadeghifar and Ragauskas, 2020). The use of lignin with other synthetic UV blockers shows a synergic effects and potentially improved final UV blocking capacity in comparison to using either only a synthetic UV blocker or lignin. Lignin has been used in the development of high performances sunscreen products to significantly enhance the UV absorbance range. In recent years, the use of lignin in various fields such as green chemistry, polymer and material science, chemical engineering, and biochemistry has shown excellent prospects (Sadeghifar and Ragauskas, 2020). In order to achieve better synergetic effects, lignin could be used in the form of nanocomposites and nanohybrids. Lignin-based transparent flexible film using gum, 2-hydroxyethyl cellulose (HEC), and softwood kraft lignin composite has been reported to have food packaging and biomedical applications (Rukmanikrishnan et al., 2020). The addition of lignin also improved the UV protection, and hydrophobic and mechanical properties of the film. In addition, chitin nanofibers are used for the growth and development of lignin nanoparticles (Lizundia et al., 2021). Along with the antioxidant properties of lignin, a well-known antibiotic property of chitosan has been used in recent developments in food packaging and medical science.
The current review studies the various applications of biopolymer nanocomposites for sustainable UV protective packaging along with studies of current challenges and the prospects of sustainable UV protective packaging in recent years.
As the ozone layer is being depleted by environmental pollution, the amount of UV rays that directly hit the earth’s surface is increasing. In addition, the UV rays generated by the Sun are harmful to living organisms and also adversely affect various types of non-living materials (Patil et al., 2021). When packaging materials are exposed to UV light for a long period of time, the original properties become altered and the intrinsic components of the packaging materials deteriorate, which weakens their original strength and functionality. The UV rays that have such an adverse effect are largely divided into ultraviolet A (UV-A), ultraviolet B (UV-B), and ultraviolet C (UV-C) (Figure 4). Of these, UV-A, which accounts for the largest proportion of the incident sunlight, penetrates glass at a wavelength of 320–400 nm and accounts for 95% of the radiation that reaches human skin (Singh et al., 2020; Patil et al., 2021). The radiation wavelength of UV-B is 280–320 nm, and it causes a degradation in the internal components of packaging material and decreases performance, thus shortening its shelf life. Fortunately, the majority of UV-C are absorbed by O2 molecules, and therefore packaging materials are primarily damaged by UV-A and UV-B rays. Therefore, packaging materials need to be blended with UV protective materials and their functionality must be protected and extended for a long period of time (Tang et al., 2012).
FIGURE 4. Ultraviolet (UV-A, UV-B, and UV-C) radiations and their position in the electromagnetic spectrum.
There are numerous organic as well as inorganic materials and hybrids that have been used for UV protective applications in various fields. In nanoscale size, these materials have different properties compared to bulk and can efficiently work for UV protection even at a low concentration (Ariane et al., 2020; Roy et al., 2020). Nanomaterials (organic/inorganic/hybrid) have an maximum absorption in the UV region (200–400 nm) and are capable to absorb UV light efficiently. The following Table 1 lists the types of materials that can be used in UV protection (Blanchard and Blanchet, 2011).
TABLE 1. Organic and inorganic materials used in UV protective applications. Reconstructed from Blanchard and Blanchet (2011).
Biopolymeric materials like alginate, chitosan, gelatin, starch, cellulose, etc. are derived from natural sources with good biocompatibility and biodegradability (Tang et al., 2012; Motelica et al., 2020). The pristine biopolymeric films/coating materials used for packaging may or may not be capable of absorbing a range of UV light, which also depends on the intrinsic chemical components of the polymers. To enhance the UV protection of the desired biopolymeric films nanomaterials having absorption band in UV region are added during fabrication of films. The nanomaterials have an appropriate size, chemical inertness, efficacy, and are friendly to the environment, meaning they are used in sustainbale packaging applications. It is possible to manipulation the UV absorption properties according to the requirements to optimize the experimental conditions. By considering the overall requirements of packaging films, nanomaterials like ZnO, TiO2, CeO2, and carbon nanomaterials (carbon nanodots, graphene quantum dots, etc.) are most suitable and compatible for UV protective applications (Reinosa et al., 2016; Rhim and Kim, 2014; Singh et al., 2020). The most common protocol for designing biopolymeric nanocomposite films by solution casting method has depicted in Figure 5.
FIGURE 5. General protocol for designing biopolymeric nanocomposite films by solution casting method.
The mechanism of light absorption from the UV region depends on the type of biopolymers and nanomaterials used for the design of UV protective films/coatings. Most of the biopolymers contain a basic backbone of carbohydrate repeating units which include amino, hydroxy, alcohol, acid, ketone, and aldehyde as functional groups. A lack of aromatic structures results in a significantly lower possibility for π-π transition and hence absorption is difficult or very weak in the UV region. Thus, pristine biopolymeric-based films may allow all UV, Visible, and near IR radiation to pass during exposure (Figure 6A). In contrast, nanomaterials with absorption in the UV region and embedded in biopolymeric films block UV light and allow light to pass other radiations like Visible and near IR (Figure 6B). Many mechanistic possibilities might be happening during UV protection by biopolymeric nanocomposites and depends mainly on the spectroscopic characteristics and kinds of nanomaterials incorporated (Singh et al., 2020). Nanomaterials have fluorescence/photoluminescence behavior when excited by the wavelength of UV light, and may be able to emit in the visible region (Patil et al., 2020). Due to their characteristics, biopolymeric nanocomposites could therefore easily potentially provide sustainable UV protection, by converting UV radiations into visible (Patil et al., 2020). Furthermore, backscattering, reflection, and absorption phenomenon are common during the process of UV protection with the aid of UV absorbing nanomaterials. Furthermore, these mechanisms also depend on the size, shape, crystallinity, and color of incorporated nanomaterials as well as the surface properties of the resultant biopolymeric nanocomposite films.
FIGURE 6. Schematic showing UV protection mechanism of pristine biopolymer (A) and biopolymer nanocomposite when exposed to UV, Visible, and IR radiation (B).
UV protective mechanisms and the effectiveness of UV protective composite films also depend on the nature and concentration of UV absorbing nanomaterials used. Most of the pristine biopolymeric films transmit (T = 90%) all UV radiation (Figure 7A). When such pristine films are doped with a small amount of nanomaterials, they can absorb UV light from UV-C and a few of the UV-B (Rhim and Kim, 2014; Singh et al., 2020). As the concentration of nanomaterial increases, there is a possibility that it could absorb maximum light from regions of UV-A, UV-B, and UV-C. In addition, the use of two or more nanomaterials with an appropriate ratio during the casting of films with biopolymer as base material enables more complete absorption of the UV region (UV-A, UV-B, and UV-C) (Feng et al., 2017; Wang et al., 2017). The use of photoresponsive fluorescent/photoluminescent nanomaterials is capable of simultenous absorption UV region and emissions in the visible region (Figure 7B) (Patil et al., 2020; Hess et al., 2017). The characteristic features of the emission bands depend on the excitation wavelength of the nanomaterials.
FIGURE 7. (A) Graphs showing the UV-A, UV-B, and UV-C absorption capacity of pristine biopolymers and biopolymer nanocomposite with respect to % T and (B) spectral profile of UV absorption and simultaneous conversion of absorbed UV light into visible by biopolymer nanocomposite embedded with fluorescent nanomaterials.
The UV light is highly energetic, which can deteriorate living and non-living things and shorten their shelf life. Prolonged exposure to UV radiation can cause serious health problems for the eyes and skin diseases, and in severe cases causes skin cancer. The shelf life of vegetables and fruit may be affected by UV radiation (Patil et al., 2021). Therefore, to address this highly emerging problem, researchers are trying to develop newly designed sustainable materials capable of UV blocking as well as biodegradable packaging. The chemical and mechanical properties of nanomaterials along with UV blocking potential make them novel in a variety of applications. These functional organic and inorganic nanomaterials and their biopolymer nanocomposites are highly demanding and applicable in the field of UV protection and easily decomposable packaging materials. Nanomaterials like ZnO, TiO2, CeO2, SiO2, Cu NPs, CDs, plant extracts, and melamine (Roy et al., 2020; Li et al., 2018; Wang et al., 2019; Reinosa et al., 2016) (in situ generated silica reinforced polyvinyl alcohol liquefied chitin biodegradable films for food packaging. pdf), etc. are widely used in UV blocking applications.
Wei Wang and colleagues designed CeO2 NPs immobilized regenerated cellulose films (Wang et al., 2019). The as-prepared films showed excellent UV shielding properties. The optimized CeO2 concentration in the biopolymer film absorbs UV-A and UV-B and more than 75% transmittance was observed up to 550 nm. Along with UV blocking, the film revealed moderate thermal stability, high transmittance, and a certain degree of hydrophobicity. Bio-based UV protective films (UV-PF) of polylactic acid and diethyl ether extract of Phoebe zhennan were prepared by Pan et al. (2018). Adding 24 wt% extract showed complete absorption of UV-C and UV-B along with 90% absorption in the UV-A region. The UV-PF film showed more tensile strength than the bare PF film.
The research group of Hasan Sadeghifer prepared a semitransparent, flexible, and biodegradable UV protective film from biopolymers (Sadeghifar et al., 2017). Azide modified cellulose dissolved in dimethylacetamide/lithium chloride reacts with propargilated lignin to form a homogeneous and covalently bonded cellulose-lignin UV protective film. The film showed 100% protection of UV-B and 90% of UV-A. The film is stable up to 120°C and in UV radiation. Yibo Dai et al. fabricated a polyvinyl alcohol/metal-organic framework nanocomposite (PVA/HKUST-1) film by a simple solvent casting method (Dai et al., 2018). The film has enhanced mechanical, thermal and UV shielding properties, with 137 and 32% improved Young’s model and tensile strength than bare PVA film.
The oxidized nanocellulose (ONC) was extracted from bamboo fibers and carbon dots (CDs) and ZnO nanostructures were assembled into the film to prepare CDs-ONC-ZnO composite film (Feng et al., 2017). The designed film showed high visible light transparency, excellent thermal stability, and enhanced UV blocking properties. The use of CDs in addition to ZnO nanostructures enhanced the UV blocking capacity of the CDs-ONC-ZnO composite film. Our research group has successfully developed a highly transparent, flexible, and re-emissive PVA@WTR-CDs composite film for UV blocking and practically applied it to food packaging (Patil et al., 2020). The distinguishable results revealed that the fluorescent WTR-CDs embedded PVA films not only absorb UV radiation but that it also re-emit it in the visible region. The films have successfully blocked 100% of UV-C and UV-B as well 20–60% of light from the UV-A region. Another practical application of this film is its food packaging application. This film was used to enhance the shelf life of grapes.
The melanin nanoparticles (MNP) reinforced cellulose nanofiber (CNF) composite (CNF/MNP) UV shielding and the antioxidant film were prepared by Swaroop Roy’s research group (Roy et al., 2020). The MNPs were isolated from sepia ink and CNF was prepared from hardwood pulp. The naturally isolated MNPs and CNFs incorporated nanocomposite films that showed UV blocking, water vapor barrier properties along with strong antioxidant activities. UV protective applications of various biopolymer nanocomposites are summarised systematically and tabulated in Table 2.
Much progress has been made in recent years in the design, development and use of biopolymer-based nanocomposite materials or in the form of films or coatings for durable packaging. During the design of biopolymer nanocomposites, properties like surface structure, biodegradability, transparency, mechanical strength, antibacterial, toxicity, gas barrier crystallinity, etc., are used to consider its use and practical applications in various fields. However, there are still many technical issues that need to be addressed. In particular, further study of good UV protection by restoring the mechanical as well as optical transparency of the biopolymer nanocomposite is required. In most cases, to block the complete UV region (UV-A, UV-B, and UV-C; 200–400 nm) two or more nanomaterials need to be incorporated into the polymer matrix, which can affect the strength and transparency of composites. The fluorescence/photoluminescence of the UV protective materials with an excitation maximum in the UV region should be more focused to achieve the simultaneous emission of visible light during the absorption of UV radiation. This will enable next-generation smart packaging materials. Therefore, in the near future, there is an urgent need to develop a sustainable pathway for the synthesis of nanomaterials that will cover the entire range of UV at low concentrations. Furthermore, more specific methods of manufacturing biopolymer nanocomposites need to be developed to meet the basic requirements of packaging material with durable UV protection.
This review discusses the origin, classification, and types of various biopolymers used in packaging-related applications. A more detailed discussion of the various properties of biopolymers with subclasses is also given. The position of the UV region in the electromagnetic spectrum, their types, and their hazardous nature for various materials on earth have been mentioned in short. We also provide information on the types, characteristics, size, and applications of organic and inorganic UV protective materials/nanomaterials commercialized by various groups. The general methods for designing nanomaterial embedded biopolymeric nanocomposites are shown pictorially. The fundamental principle of the UV protective mechanism of pristine biopolymer and biopolymer nanocomposite with respect to UV absorption and emission in the visible region by fluorescent nanomaterials is also schematically presented. It also discusses various reports on the use of biopolymer nanocomposite for UV protective packaging applications along with a systematic tabulation. Finally, the current challenges and future prospects of nanocomposite-based sustainable packaging materials were discussed.
AG—Topic Selection, TOC Planning, Literature Study, Writing Draft, Formal Analysis, Suggestion, and Editing. AP—Literature search, Writing Manuscript Draft, Formal Analysis.
The authors declare that the research was conducted in the absence of any commercial or financial relationships that could be construed as a potential conflict of interest.
All claims expressed in this article are solely those of the authors and do not necessarily represent those of their affiliated organizations, or those of the publisher, the editors and the reviewers. Any product that may be evaluated in this article, or claim that may be made by its manufacturer, is not guaranteed or endorsed by the publisher.
The authors thank Uka Tarsadia University for supporting this work.
Ahmed, S., and Ikram, S. (2016). Chitosan and Gelatin Based Biodegradable Packaging Films with UV-Light protection. J. Photochem. Photobiol. B: Biol. 163, 115–124. doi:10.1016/j.jphotobiol.2016.08.023
Ariane, K., Tamayo, A., Chorfa, A., Rubio, F., and Rubio, J. (2020). Effect of P2O5 and Al2O3 on crystallization, structure, microstructure and properties of Li2O–MgO–Al2O3–SiO2–TiO2–ZrO2 glass ceramicsEfecto del P2O5 yAl2O3 en la cristalización, estructura, microestructura y propiedades de vitrocerámicos del sistema Li2O-MgO-Al2O3-SiO2-TiO2-ZrO2. Boletín de la Sociedad Española de Cerámica y Vidrio. doi:10.1016/j.bsecv.2020.08.004
Bae, J.-Y., Kim, H.-Y., Lim, Y.-W., Kim, Y.-H., and Bae, B.-S. (2016). Optically Recoverable, Deep Ultraviolet (UV) Stable and Transparent Sol-Gel Fluoro Siloxane Hybrid Material for a UV LED Encapsulant. RSC Adv. 6, 26826–26834. doi:10.1039/c6ra01346e
Balazs, A. C., Emrick, T., and Russell, T. P. (2006). Nanoparticle Polymer Composites: Where Two Small Worlds Meet. Science 314, 1107–1110. doi:10.1126/science.1130557
Basumatary, I. B., Mukherjee, A., Katiyar, V., and Kumar, S. (2020). Biopolymer-based Nanocomposite Films and Coatings: Recent Advances in Shelf-Life Improvement of Fruits and Vegetables. Crit. Rev. Food Sci. Nutr. 62, 1912–1935. doi:10.1080/10408398.2020.1848789
Blanchard, V., and Blanchet, P. (2011). Color Stability for wood Products during Use: Effects of Inorganic Nanoparticles. BioResources 6, 1219–1229. doi:10.15376/biores.6.2.1219-1229
Chen, J., Wu, A., Yang, M., Ge, Y., Pristijono, P., Li, J., et al. (2021). Characterization of Sodium Alginate-Based Films Incorporated with Thymol for Fresh-Cut Apple Packaging. Food Control 126, 108063. doi:10.1016/j.foodcont.2021.108063
Chouhan, S., Bajpai, A. K., Bajpai, J., Katare, R., and Dhoble, S. J. (2017). Mechanical and UV Absorption Behavior of Zinc Oxide Nanoparticles: Reinforced Poly(vinyl Alcohol-G-Acrylonitrile) Nanocomposite Films. Polym. Bull. 74, 4119–4141. doi:10.1007/s00289-017-1942-1
Dai, Y., Tang, Q., Zhang, Z., Yu, C., Li, H., Xu, L., et al. (2018). Enhanced Mechanical, thermal, and UV-Shielding Properties of Poly(vinyl Alcohol)/metal-Organic Framework Nanocomposites. RSC Adv. 8, 38681–38688. doi:10.1039/c8ra07143h
Feng, X., Zhao, Y., Jiang, Y., Miao, M., Cao, S., and Fang, J. (2017). Use of Carbon Dots to Enhance UV-Blocking of Transparent Nanocellulose Films. Carbohydr. Polym. 161, 253–260. doi:10.1016/j.carbpol.2017.01.030
Gause, S., and Chauhan, A. (2015). Incorporation of Ultraviolet (UV) Absorbing Nanoparticles in Contact Lenses for Class 1 UV Blocking. J. Mater. Chem. B 4, 327–339. doi:10.1039/c5tb01532d
Gijsman, P., Meijers, G., and Vitarelli, G. (1999). Comparison of the UV-Degradation Chemistry of Polypropylene, Polyethylene, Polyamide 6 and Polybutylene Terephthalate. Polym. Degrad. Stab. 65, 433–441. doi:10.1016/S0141-3910(99)00033-6
Goudarzi, V., Shahabi-Ghahfarrokhi, I., and Babaei-Ghazvini, A. (2017). Preparation of Ecofriendly UV-Protective Food Packaging Material by starch/TiO2 Bio-Nanocomposite: Characterization. Int. J. Biol. Macromolecules 95, 306–313. doi:10.1016/j.ijbiomac.2016.11.065
He, X., Luzi, F., Hao, X., Yang, W., Torre, L., Xiao, Z., et al. (2019). Thermal, Antioxidant and Swelling Behaviour of Transparent Polyvinyl (Alcohol) Films in Presence of Hydrophobic Citric Acid-Modified Lignin Nanoparticles. Int. J. Biol. Macromolecules 127, 665–676. doi:10.1016/j.ijbiomac.2019.01.202
Helanto, K., Matikainen, L., Talj, R., and Rojas, O. J. (2019). Bio-based Polymers for Sustainable Packaging and Biobarriers: A Critical Review. BioResources 14, 4902–4951. doi:10.15376/biores.14.2.Helanto
Hess, S. C., Permatasari, F. A., Fukazawa, H., Schneider, E. M., Balgis, R., Ogi, T., et al. (2017). Direct Synthesis of Carbon Quantum Dots in Aqueous Polymer Solution: One-Pot Reaction and Preparation of Transparent UV-Blocking Films. J. Mater. Chem. A. 5, 5187–5194. doi:10.1039/c7ta00397h
Huang, X.-J., Zeng, X.-F., Wang, J.-X., and Chen, J.-F. (2018). Transparent Dispersions of Monodispersed ZnO Nanoparticles with Ultrahigh Content and Stability for Polymer Nanocomposite Film with Excellent Optical Properties. Ind. Eng. Chem. Res. 57, 4253–4260. doi:10.1021/acs.iecr.7b04878
Kim, Y., Ryu, T. I., Ok, K.-H., Kwak, M.-G., Park, S., Park, N.-G., et al. (2015). Inverted Layer-By-Layer Fabrication of an Ultraflexible and Transparent Ag Nanowire/Conductive Polymer Composite Electrode for Use in High-Performance Organic Solar Cells. Adv. Funct. Mater. 25, 4580–4589. doi:10.1002/adfm.201501046
Kumar Barman, B., Nagao, T., and Nanda, K. K. (2020). Dual Roles of a Transparent Polymer Film Containing Dispersed N-Doped Carbon Dots: A High-Efficiency Blue Light Converter and UV Screen. Appl. Surf. Sci. 510, 145405. doi:10.1016/j.apsusc.2020.145405
Li, S., Meng Lin, M., Toprak, M. S., Kim, D. K., and Muhammed, M. (2010). Nanocomposites of Polymer and Inorganic Nanoparticles for Optical and Magnetic Applications. Nano Rev. 1, 5214. doi:10.3402/nano.v1i0.5214
Li, T., Li, B., Ji, Y., and Wang, L. (2018). Luminescent and UV-Shielding ZnO Quantum Dots/carboxymethylcellulose Sodium Nanocomposite Polymer Films. Polymers 10, 1112. doi:10.3390/polym10101112
Lizundia, E., Sipponen, M. H., Greca, L. G., Balakshin, M., Tardy, B. L., Rojas, O. J., et al. (2021). Multifunctional Lignin-Based Nanocomposites and Nanohybrids. Green. Chem. 23, 6698–6760. doi:10.1039/d1gc01684a
Look, D. C. (2001). Recent Advances in ZnO Materials and Devices. Mater. Sci. Eng. B 80, 383–387. doi:10.1016/S0921-5107(00)00604-8
Loste, J., Lopez-Cuesta, J.-M., Billon, L., Garay, H., and Save, M. (2019). Transparent Polymer Nanocomposites: An Overview on Their Synthesis and Advanced Properties. Prog. Polym. Sci. 89, 133–158. doi:10.1016/j.progpolymsci.2018.10.003
Mackay, M. E., Tuteja, A., Duxbury, P. M., Hawker, C. J., Van Horn, B., Guan, Z., et al. (2006). General Strategies for Nanoparticle Dispersion. Science 311, 1740–1743. doi:10.1126/science.1122225
Mestdagh, F., De Meulenaer, B., De Clippeleer, J., Devlieghere, F., and Huyghebaert, A. (2005). Protective Influence of Several Packaging Materials on Light Oxidation of Milk. J. Dairy Sci. 88, 499–510. doi:10.3168/jds.S0022-0302(05)72712-0
Motelica, L., Ficai, D., Ficai, A., Oprea, O. C., and Andronescu, E. (2020). Biodegradable Antimicrobial Food Packaging : Trends and Perspectives. Foods 9 (10), 1438. doi:10.3390/foods9101438
Pan, F., Chen, L., Jiang, Y., Xiong, L., Min, L., Xie, J., et al. (2018). Bio-based UV Protective Films Prepared with Polylactic Acid (PLA) and Phoebe Zhennan Extractives. Int. J. Biol. Macromolecules 119, 582–587. doi:10.1016/j.ijbiomac.2018.07.189
Parit, M., Saha, P., Davis, V. A., and Jiang, Z. (2018). Transparent and Homogenous Cellulose Nanocrystal/Lignin UV-Protection Films. ACS Omega 3, 10679–10691. doi:10.1021/acsomega.8b01345
Patil, A. S., Nille, O. S., Kolekar, G. B., Sohn, D., and Gore, A. H. (2021). Biopolymer @ Nanocomposites for Sustainable Packaging Applications. Singapore: Springer.
Patil, A. S., Waghmare, R. D., Pawar, S. P., Salunkhe, S. T., Kolekar, G. B., Sohn, D., et al. (2020). Photophysical Insights of Highly Transparent, Flexible and Re-emissive PVA @ WTR-CDs Composite Thin Films: A Next Generation Food Packaging Material for UV Blocking Applications. J. Photochem. Photobiol. A: Chem. 400, 112647. doi:10.1016/j.jphotochem.2020.112647
Philp, J. C., Bartsev, A., Ritchie, R. J., Baucher, M.-A., and Guy, K. (2013). Bioplastics Science from a Policy Vantage point. New Biotechnol. 30, 635–646. doi:10.1016/j.nbt.2012.11.021
Poudel, P. R., Kataoka, I., and Mochioka, R. (2008). Effect of Red- and Blue-Light-Emitting Diodes on Growth and Morphogenesis of Grapes. Plant Cel Tiss Organ. Cult 92, 147–153. doi:10.1007/s11240-007-9317-1
Puls, J., Wilson, S. A., and Hölter, D. (2011). Degradation of Cellulose Acetate-Based Materials: A Review. J. Polym. Environ. 19, 152–165. doi:10.1007/s10924-010-0258-0
Qi, Y., Wang, Y., Yu, Y., Liu, Z., Zhang, Y., Qi, Y., et al. (2016). Exploring Highly Efficient Light Conversion Agents for Agricultural Film Based on Aggregation Induced Emission Effects. J. Mater. Chem. C 4, 11291–11297. doi:10.1039/c6tc04215e
Qu, L., Tian, M., Hu, X., Wang, Y., Zhu, S., Guo, X., et al. (2014). Functionalization of Cotton Fabric at Low Graphene Nanoplate Content for Ultrastrong Ultraviolet Blocking. Carbon 80, 565–574. doi:10.1016/j.carbon.2014.08.097
Reinosa, J., Leret, P., Álvarez-Docio, C. M., Del Campo, A., and Fernández, J. F. (2016). Enhancement of UV Absorption Behavior in ZnO-TiO2 Composites. Boletín de la Sociedad Española de Cerámica y Vidrio 55, 55–62. doi:10.1016/j.bsecv.2016.01.004
Rhim, J.-W., and Kim, Y.-T. (2014). Biopolymer-Based Composite Packaging Materials with Nanoparticles. Innov. Food Packaging 2014, 413–442. doi:10.1016/B978-0-12-394601-0.00017-5
Roy, S., Kim, H. C., Kim, J. W., Zhai, L., Zhu, Q. Y., and Kim, J. (2020). Incorporation of Melanin Nanoparticles Improves UV-Shielding, Mechanical and Antioxidant Properties of Cellulose Nanofiber Based Nanocomposite Films. Mater. Today Commun. 24, 100984. doi:10.1016/j.mtcomm.2020.100984
Rukmanikrishnan, B., Ramalingam, S., Rajasekharan, S. K., Lee, J., and Lee, J. (2020). Binary and Ternary Sustainable Composites of Gellan Gum, Hydroxyethyl Cellulose and Lignin for Food Packaging Applications: Biocompatibility, Antioxidant Activity, UV and Water Barrier Properties. Int. J. Biol. Macromolecules 153, 55–62. doi:10.1016/j.ijbiomac.2020.03.016
Sadeghifar, H., and Ragauskas, A. (2020). Lignin as a UV Light Blocker-A Review. Polymers 12, 1134–1210. doi:10.3390/POLYM12051134
Sadeghifar, H., Venditti, R., Jur, J., Gorga, R. E., and Pawlak, J. J. (2017). Cellulose-Lignin Biodegradable and Flexible UV Protection Film. ACS Sust. Chem. Eng. 5, 625–631. doi:10.1021/acssuschemeng.6b02003
Saurakhiya, N., Sharma, S. K., Kumar, R., and Sharma, A. (2014). Templated Electrochemical Synthesis of polyaniline/ZnO Coaxial Nanowires with Enhanced Photoluminescence. Ind. Eng. Chem. Res. 53, 18884–18890. doi:10.1021/ie500989m
Segura González, E. A., Olmos, D., Lorente, M. Á., Vélaz, I., and González-Benito, J. (2018). Preparation and Characterization of Polymer Composite Materials Based on PLA/TiO2 for Antibacterial Packaging. Polymers 10, 1365. doi:10.3390/polym10121365
Sharma, B., Malik, P., and Jain, P. (2018). Biopolymer Reinforced Nanocomposites: A Comprehensive Review. Mater. Today Commun. 16, 353–363. doi:10.1016/j.mtcomm.2018.07.004
Shi, Y.-e., Zhuang, X., Cao, L., Gou, S., Xiong, Y., Lai, W.-F., et al. (2019). Copper-Nanocluster-Based Transparent Ultraviolet-Shielding Polymer Films. ChemNanoMat 5, 110–115. doi:10.1002/cnma.201800448
Singh, H. K., Kumar, S., Bamne, J., Taiwade, K., Singh, N., Chandel, V., et al. (2021). Analyzing the Synthesis of Various Inorganic Nanoparticles and Their Role in UV-Shielding. Mater. Today Proc. 46, 5607–5618. doi:10.1016/j.matpr.2020.09.499
Sirerol, J. A., Feddi, F., Mena, S., Rodriguez, M. L., Sirera, P., Aupí, M., et al. (2015). Topical Treatment with Pterostilbene, a Natural Phytoalexin, Effectively Protects Hairless Mice against UVB Radiation-Induced Skin Damage and Carcinogenesis. Free Radic. Biol. Med. 85, 1–11. doi:10.1016/j.freeradbiomed.2015.03.027
Su, X., and Chen, B. (2018). Transparent, UV-Proof and Mechanically strong montmorillonite/alginate/Ca2+ Nanocomposite Hydrogel Films with Solvent Sensitivity. Appl. Clay Sci. 165, 223–233. doi:10.1016/j.clay.2018.07.044
Subramani, N. K., Kasargod Nagaraj, S., Shivanna, S., and Siddaramaiah, H. (2016). Highly Flexible and Visibly Transparent Poly(vinyl alcohol)/Calcium Zincate Nanocomposite Films for UVA Shielding Applications as Assessed by Novel Ultraviolet Photon Induced Fluorescence Quenching. Macromolecules 49, 2791–2801. doi:10.1021/acs.macromol.5b02282
Tan, Y. M., Lim, S. H., Tay, B. Y., Lee, M. W., and Thian, E. S. (2015). Functional Chitosan-Based Grapefruit Seed Extract Composite Films for Applications in Food Packaging Technology. Mater. Res. Bull. 69, 142–146. doi:10.1016/j.materresbull.2014.11.041
Tang, X. Z., Kumar, P., Alavi, S., and Sandeep, K. P. (2012). Recent Advances in Biopolymers and Biopolymer-Based Nanocomposites for Food Packaging Materials. Crit. Rev. Food Sci. Nutr. 52, 426–442. doi:10.1080/10408398.2010.500508
Wang, W., Zhang, B., Jiang, S., Bai, H., and Zhang, S. (2019). Use of CeO2 Nanoparticles to Enhance UV-Shielding of Transparent Regenerated Cellulose Films. Polymers 11, 458. doi:10.3390/polym11030458
Wang, Y., Li, T., Ma, P., Bai, H., Xie, Y., Chen, M., et al. (2016). Simultaneous Enhancements of UV-Shielding Properties and Photostability of Poly(vinyl Alcohol) via Incorporation of Sepia Eumelanin. ACS Sust. Chem. Eng. 4, 2252–2258. doi:10.1021/acssuschemeng.5b01734
Wang, Y., Su, J., Li, T., Ma, P., Bai, H., Xie, Y., et al. (2017). A Novel UV-Shielding and Transparent Polymer Film: When Bioinspired Dopamine-Melanin Hollow Nanoparticles Join Polymers. ACS Appl. Mater. Inter. 9, 36281–36289. doi:10.1021/acsami.7b08763
Wu, T., Barker, M., Arafeh, K. M., Boyer, J.-C., Carling, C.-J., and Branda, N. R. (2013). A UV-Blocking Polymer Shell Prevents One-Photon Photoreactions while Allowing Multi-Photon Processes in Encapsulated Upconverting Nanoparticles. Angew. Chem. Int. Ed. 52, 11106–11109. doi:10.1002/anie.201305253
Wu, W., Liu, T., Deng, X., Sun, Q., Cao, X., Feng, Y., et al. (2019a). Ecofriendly UV-Protective Films Based on Poly(propylene Carbonate) Biocomposites Filled with TiO2 Decorated Lignin. Int. J. Biol. Macromolecules 126, 1030–1036. doi:10.1016/j.ijbiomac.2018.12.273
Wu, X., Zhang, Y. n., Zhan, S., Li, J., Nie, G., Hu, S., et al. (2019b). Tracing of Dye Molecules in Living Plants through NaGdF4:Yb3+,Er3+ Fluorescent Nanoprobes. J. Rare Earths 37, 237–241. doi:10.1016/j.jre.2018.08.007
Xiao, X., Liu, X., Chen, F., Fang, D., Zhang, C., Xia, L., et al. (2015). Highly Anti-UV Properties of Silk Fiber with Uniform and Conformal Nanoscale TiO2 Coatings via Atomic Layer Deposition. ACS Appl. Mater. Inter. 7, 21326–21333. doi:10.1021/acsami.5b05868
Xing, Q., Buono, P., Ruch, D., Dubois, P., Wu, L., and Wang, W.-J. (2019). Biodegradable UV-Blocking Films through Core-Shell Lignin-Melanin Nanoparticles in Poly(butylene Adipate-Co-Terephthalate). ACS Sust. Chem. Eng. 7, 4147–4157. doi:10.1021/acssuschemeng.8b05755
Xiong, R., Grant, A. M., Ma, R., Zhang, S., and Tsukruk, V. V. (2018). Naturally-derived Biopolymer Nanocomposites: Interfacial Design, Properties and Emerging Applications. Mater. Sci. Eng. R: Rep. 125, 1–41. doi:10.1016/j.mser.2018.01.002
Yang, H. Y., Park, H.-W., Kim, S. J., Hong, J.-M., Kim, T. W., Kim, D. H., et al. (2016). Intense Pulsed Light Induced Crystallization of a Liquid-Crystalline Polymer Semiconductor for Efficient Production of Flexible Thin-Film Transistors. Phys. Chem. Chem. Phys. 18, 4627–4634. doi:10.1039/c5cp06989k
Yang, Y., Zhao, Y., Hu, Y., Peng, X., and Zhong, L. (2020). Xylan-Derived Light Conversion Nanocomposite Film. Polymers 12 (8), 1779. doi:10.3390/polym12081779
You, Y., Zhang, H., Liu, Y., and Lei, B. (2016). Transparent Sunlight Conversion Film Based on Carboxymethyl Cellulose and Carbon Dots. Carbohydr. Polym. 151, 245–250. doi:10.1016/j.carbpol.2016.05.063
Yu, Y., Wang, Y., Liu, W., Jia, X., Ma, L., Ren, L., et al. (2018a). Exploration of Highly Efficient Light Conversion Agents for Agricultural Film Based on the bay-substituted Perylene Diimides Derivatives. Dyes Pigm. 159, 483–490. doi:10.1016/j.dyepig.2018.07.015
Yu, Z., Li, B., Chu, J., and Zhang, P. (2018b). Silica In Situ Enhanced PVA/chitosan Biodegradable Films for Food Packages. Carbohydr. Polym. 184, 214–220. doi:10.1016/j.carbpol.2017.12.043
Zhao, Z., Mao, A., Gao, W., and Bai, H. (2018). A Facile In Situ Method to Fabricate Transparent, Flexible Polyvinyl alcohol/ZnO Film for UV-Shielding. Composites Commun. 10, 157–162. doi:10.1016/j.coco.2018.09.009
Keywords: biopolymer, nanocomposite, sustainable, UV protective, packaging, films
Citation: Gore AH and Prajapat AL (2022) Biopolymer Nanocomposites for Sustainable UV Protective Packaging. Front. Mater. 9:855727. doi: 10.3389/fmats.2022.855727
Received: 15 January 2022; Accepted: 28 March 2022;
Published: 27 April 2022.
Edited by:
Xing Zhou, Xi’an University of Technology, ChinaReviewed by:
Hossein Yousefi, Gorgan University of Agricultural Sciences and Natural Resources, IranCopyright © 2022 Gore and Prajapat. This is an open-access article distributed under the terms of the Creative Commons Attribution License (CC BY). The use, distribution or reproduction in other forums is permitted, provided the original author(s) and the copyright owner(s) are credited and that the original publication in this journal is cited, in accordance with accepted academic practice. No use, distribution or reproduction is permitted which does not comply with these terms.
*Correspondence: Anil H. Gore, YW5pbGFuYWNoZW1AZ21haWwuY29t
Disclaimer: All claims expressed in this article are solely those of the authors and do not necessarily represent those of their affiliated organizations, or those of the publisher, the editors and the reviewers. Any product that may be evaluated in this article or claim that may be made by its manufacturer is not guaranteed or endorsed by the publisher.
Research integrity at Frontiers
Learn more about the work of our research integrity team to safeguard the quality of each article we publish.