- 1Materials Genome Institute, Shanghai University, Shanghai, China
- 2China Automotive Engineering Research Institute Co., Ltd, Chongqing, China
- 3Department of Physics, Hong Kong University of Science and Technology, Hong Kong, Hong Kong SAR, China
Thermochromic glass possesses great potential for reducing the energy demand and providing indoor comfort in buildings. Suitable atomization temperature change points have a great influence on the application of thermochromic glass. Based on energy consumption simulations and theoretical calculations by introducing solar radiation in a transparent envelope, this paper investigates the atomization temperature change point of thermochromic glass in hot summer and cold winter zones in Chongqing, showing that the suitable temperature change point of the thermochromic glass is 39°C with air conditioning and 42.9°C without air conditioning. Furthermore, the results of simulations and theoretical calculations are applied to a test model, revealing that thermochromic glass with the temperature change point of 42.9°C compared with Low-E glass can reduce the temperature of a sunlight room by up to 5°C in the summer and displays a certain thermal insulation effect in winter.
Introduction
Urban building energy consumption is the total energy consumption of buildings in the city, including Heating, Ventilation, and Air Conditioning energy consumption (Sadineni et al., 2011; Nguyen et al., 2014) lighting energy consumption, the electrical energy consumption of sockets, etc., which can account for the total energy consumption 30–40% of the whole society (Li et al., 2011; Nguyen et al., 2014; Zhu et al., 2020) (that is, the sum of all energy consumption of industry, agriculture, and residents, etc.). The energy performance of a building depends on the building envelopes, especially windows, which frequently let in or let out energy and are responsible for 20–40% of wasted energy in a building (Hee et al., 2015; Konis et al., 2016). The energy saving potentials of windows are important determinants in building energy saving, and window glazing selection is one of the crucial issues in designing windows. Low-emissivity (Low-E) glass has been widely used in new buildings in western developed countries and regions and has been promoted in China. Low-E coatings are spectrum selective films designed to allow visible light to pass through and block infrared and ultraviolet wavelengths that normally generate heat. Due to its high infrared reflectance, this kind of glass has been greatly developed. Over the past 2 decades, great progress has been made to explore their different attributes (Cai et al., 2013). There are two kinds of typical low radiation coatings (Ruffolo and La Russa, 2019): tin oxide based hard coatings and silver based soft coatings, which have high infrared reflectance and low transmittance. However, the visible light transmittance of the hard coating can be improved by using the antireflection properties of silica.
Existing Low-E glass still has some limitations (Pierucci et al., 2018; Abundiz-Cisneros et al., 2020), including that its optical properties cannot be changed with people’s wishes or environmental conditions, and that it is difficult to achieve the best energy-saving effect in hot summer and cold winter areas. The comfort and energy-saving effects also have certain limitations. People hope to develop a kind of glass with intelligent adjustment ability (Cai et al., 2018), which can automatically adjust the optical properties according to the environmental temperature, sunlight intensity, and other conditions: when the indoor temperature is low, it could let the sunlight enter the room to heighten the indoor temperature; when the room temperature is high, it can block all or part of the sunlight and play a heat-resistant role (Gao et al., 2012). The glass with this adjustment ability is called “intelligent or smart glass”. Smart windows were first proposed by Lambert of the United States. It has the advantages of energy saving, heat insulation, heat preservation, and decoration (Jin et al., 2019; Zhang et al., 2019). It has important application values in automobiles, building doors and windows, display equipment, and other fields. Specifically, smart window is an intelligent device composed of transparent substrate (such as glass and a dimming material layer) (Javad and Navid, 2019). Under certain conditions (Calas et al., 2006) (such as temperature, electric field, gas, and light), the dimming material will undergo chemical or physical changes, change the transmission and reflection characteristics of sunlight in a specific band (Charaya et al., 2019; Kim and Yang, 2020), then adjust the intensity of light entering the room, reduce the energy consumption of cooling and heating, and achieve the purpose of energy saving. This kind of intelligent glass overcomes the shortcoming that traditional solar control glass and Low-E glass cannot adjust dynamically according to need. It is a more promising energy-saving window material (Allen et al., 2017).
Thermochromic glass (Wang and Narayan, 2021; Mlyuka et al., 2009) is a curtain wall shading integrated glass formed by the tight combination of nanogel thermochromic film, VO2-based coating film, and tempered glass (Gong et al., 2009; Yang et al., 2020; Muramoto et al., 2020). The nanogel thermochromic film is a three-dimensional network polymer, which is colorless, odorless, and non-toxic (Zhou et al., 2016). When the ambient temperature is higher than the temperature change point, the thermochromic film changes to dimming and plays the role of shading and heat insulation. When the temperature is lower, transparency is restored (Figure 1), thereby achieving penetration of natural light and solar radiations. According to the characteristics of thermochromic glass, the temperature change point can be set between 16 and 50°C (Supplementary Figure S1, Supplementary Figure S2). If the temperature change point is set too high, there may be a situation where the glass cannot be atomized under any working conditions, then the thermochromic glass completely loses its characteristics of reducing the solar heat gain of the building and cannot reduce the cooling load from air conditioning. If the temperature change point is set too low, there may be frequent dimming of the glass, when the outdoor temperature is low, meaning the glass will be fogged by the Sun, which is not conducive to introducing more sunlight into the room in winter. Based on this, choosing an appropriate temperature change point has great influence on the application of thermochromic glass. The temperature change point of glass fogging needs to be determined according to the application environment (Cannavale et al., 2010).
Based on an energy consumption simulation and theoretical calculations, this paper aimed to obtain the most suitable temperature change point of this kind of thermotropic glass and combines the theoretical calculation results with results from a test house in Chongqing. The test results are in good agreement with the theoretical calculations. This result is of great significance for the wide application of thermotropic glass.
Methodology
We used Ladybug (Kharvari, 2020) and energy plus (Iqbal et al., 2018; Kim et al., 2019) to build the model. Solar radiation is an important factor affecting the indoor thermal environment and energy consumption (Hasan et al., 2008; Negendahl and Nielsen, 2015). At the same time, the absorption of solar radiation by the building envelope will also increase the exterior surface temperature of the building envelope (Li et al., 2022). The external surface temperature of the glass is expressed by the temperature value, which indicates the thermal effect of outdoor air temperature, solar radiation, and atmospheric long wave radiation on the outer surface of the glass (Wu et al., 2019).
According to the heat balance relation of the building surface (Figure 2), we can deduce the calculation formula of the temperature of the glass outer surface. The expression is expressed as follows:
where
where I is the solar radiation intensity, k is the heat transfer coefficient of glass,
Among the equation,
From this simple expression, we can see that there is a simple linear relationship between the glass outdoor surface temperature, the outdoor air temperature, and the amount of solar radiation.
According to the characteristics of the thermochromic glass, the temperature change point can be approximately regarded as the outer surface temperature of the glass, which is determined by the outdoor temperature and solar radiation intensity. To fully realize the energy-saving effect of the product, two conditions should be met at the same time: higher outdoor temperature and larger solar radiation. Because when the outdoor temperature is high, but the solar radiation is very low, the energy saving effect of glass atomization is not obvious, and reduces the permeability; when the outdoor temperature is low and the solar radiation is large, it is most likely to be in winter, and the glass should not be atomized at this time. Transform the formula appropriately:
When the difference between the temperature of the glass outer surface and the temperature of the outdoor air is larger, the energy-saving effect brought by the glass atomization is better, and it can block more solar radiation (McCormick and Suehrcke, 2018) from entering the room. Therefore, we can conclude that the appropriate temperature change point should meet the high outdoor temperature, sufficient solar radiation, and large temperature difference between the outer surface of the glass and the air temperature (Xu et al., 2012).
The thermotropic glass used in this article was fabricated by Chongqing Hewei Technology Co., Ltd. The thermochromic properties of thermotropic glass were monitored on a Lambda 1,050 spectrophotometer equipped with a heating unit in the wavelength range of 300–2,500 nm. The temperature of the sunlit room was measured by an online temperature tester.
Results and Discussion
Building Performance Evaluation and Simulation Analysis
As mentioned above, when the temperature difference between the outside surface of the thermochromic glass and outdoor temperature is larger, the glass is atomized at this time, which can effectively reduce the increase in heat caused by solar radiation, and the effect of atomization is better. When the outer surface temperature of the glass is lower than that of outdoor temperature, the temperature rise caused by solar radiation is smaller. Currently, the effect of glass atomization is poor and even has an adverse effect in winter. Therefore, we calculated the outside surface and outdoor temperature of the glass in distinctive climatic regions of the city with typical climatic characteristics of hot summer and cold winter, and determine the temperature change points suitable for different regions by searching for their internal change rules.
In this study, the energy consumption simulation software EnergyPlus was used to simulate the 8,760 h energy consumption of the standard model, and the 8,760 h glass surface temperature was obtained. At the same time, the annual climate parameters of Chongqing used in the calculation were read to know the out-of-doors air temperature at the corresponding time. The calculated value of 8,760 h glass surface temperature and the outdoor air temperature value of the whole year can be obtained (Figure 3A). According to the data of 8,760 h of glass outer surface temperature and outdoor air temperature obtained by energy consumption simulation software, it is impossible to find relevant laws to determine the temperature change point before processing the data.
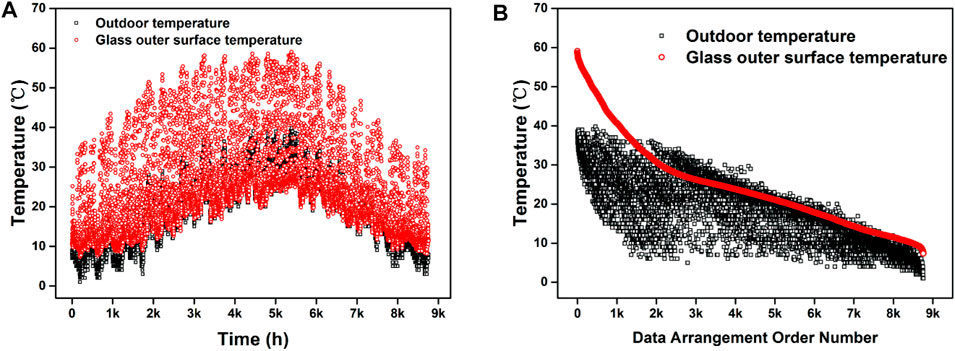
FIGURE 3. The raw data of the outdoor air temperature and the outer surface temperature of the glass for 8,760 h (A) and the data sorted by the descending order of the glass surface temperature (B).
After sorting the temperature of the glass outer surface from high to low, the temperature distribution shows a certain rule, as shown in Figure 3B. In the front part of the graph, there is a large gap between the temperature of the outer surface of the glass and the air temperature. This section meets the three conditions for selecting the temperature change point, that is, the temperature is high enough, the solar radiation is strong, and the temperature of the outer surface of the glass is higher than the air temperature. When the glass is in this time period, the atomization can exert the greatest energy saving effect. In the middle and later part of the graph, the difference between the outdoor air temperature and the outer surface temperature of the glass gradually decreases, and the change trend of the outdoor air temperature and the outer surface temperature of the glass tends to be the same. This time period is not suitable for atomization during this time period because of lower solar radiation. By performing regression processing on the temperature curve of the outer surface of the glass, an obvious boundary area between the front section and the middle and rear sections can be found. The temperature of the outer surface of the glass corresponding to this area should be the selected suitable temperature change point.
Considering that during the use of glass, the orientation of the glass and whether the indoor air conditioner is used may also affect the selection of its reasonable temperature change point, we designed two simulation conditions in an air-conditioned environment and a natural ventilation environment. Among them, the influence of glass orientation is also studied under air-conditioning environment.
In the simulation, to unify the building calculation model of each climate zone and minimize the impact of building form, we select the standard calibration building model in ASHRAE 140–2014 classic method of test for the evaluation of building energy analysis computer programs as the standard model of simulation. The model is a square box with a size of 6 m × 8 m × 2.7 m. In the air-conditioning environment, we designed four location conditions of east, west, south, and skylight. In addition, the location conditions of skylights are designed in a naturally ventilated environment. The method of setting the exterior windows in the model involved the exterior walls of the study orientation being set as exterior windows. That is, if the east exterior windows are studied, all exterior walls in the east orientation are set as exterior windows, and other orientations are set as walls, and the roof has no skylights. The principles of other orientations are the same.
According to the data processing method and temperature change point determination method mentioned above. The linear regression formula of the outer surface temperature of the skylight in Figure 4A is:
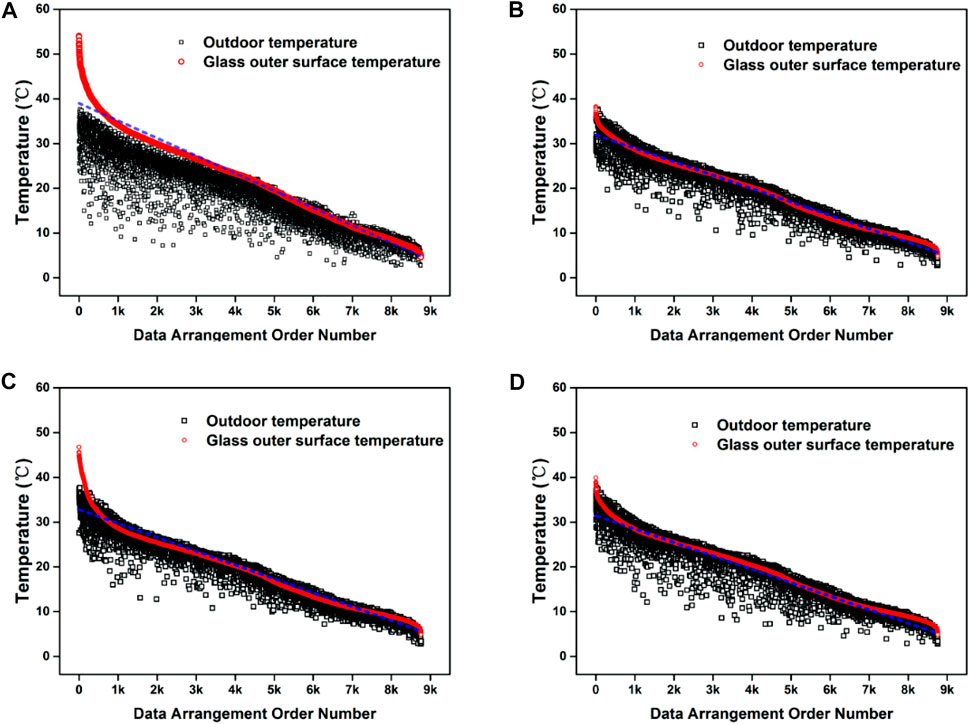
FIGURE 4. Temperature distribution of skylight (A), east (B), west (C), and south windows (D) outer surface and outdoor air temperature in Chongqing area. The blue dotted line is the linear regression curve of the outer surface temperature of the skylight.
The temperature distribution of skylight with large space outer surface and outdoor air temperature in Chongqing area is shown in Figure 5. The outer surface temperature of the skylight with large space is linearly regressed, and the regression formula is
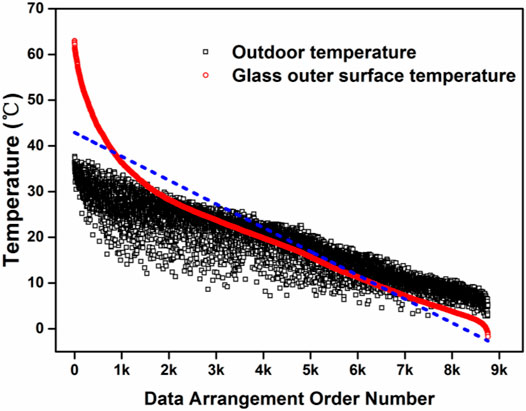
FIGURE 5. Temperature distribution of skylight outer surface and outdoor air temperature in Chongqing area in natural ventilation condition.
Sunlight Room Performance Test
To further verify the practicability and reliability of our simulation results, we tested the temperature changes in the test house in a natural ventilation environment. As shown in Figure 6, we designed two Sun rooms with a size of 3 m × 3 m×2.7 m for testing. The Sun room-1 on the left in Figure 6 is installed with ordinary Low-E glass, and the thermochromic glass is installed in the Sun room-2 on the right. Based on theoretical calculation, the thermochromic glass with a temperature change point of 42.9°C is tested without air conditioning.
We collected data on temperature changes in the two rooms over 3 days of summer and winter, as shown in Figure 7. Due to the high intensity of solar radiation in summer, the thermochromic glass on the roof of room-2 sunlight house began to atomize from 11:00 a.m. and gradually reached the full atomization state, thereby isolating solar radiation and slowing the increase in indoor temperature. According to the data analysis and as shown in Figure 7A, compared with room-1, the indoor temperature of room-2 drops by up to 5.07°C. Moreover, over a 72-h period, the thermochromic glass can reduce the indoor temperature of room-2 by an average of 1.35°C, and over the period from 8 o’clock to 17 o’clock, the thermochromic glass can reduce the indoor temperature by an average of 3.06°C. In winter, as shown in Figure 7B, the solar radiance intensity is weak, the thermochromic glass on the roof of room-2 sunlight room does not reach the temperature change point, and the indoor air temperature of the two sunlight rooms is the same. However, after the Sun sets, the indoor air temperature of the sunlight room with the thermochromic glass is higher than that of the ordinary glass. This test proves that the temperature change point obtained by the method in this study is suitable for various conditions in the actual use of thermochromic glass. Furthermore, it was proven that the thermochromic glass with a suitable temperature change point, especially in summer, has a significant effect of hindering solar radiation.
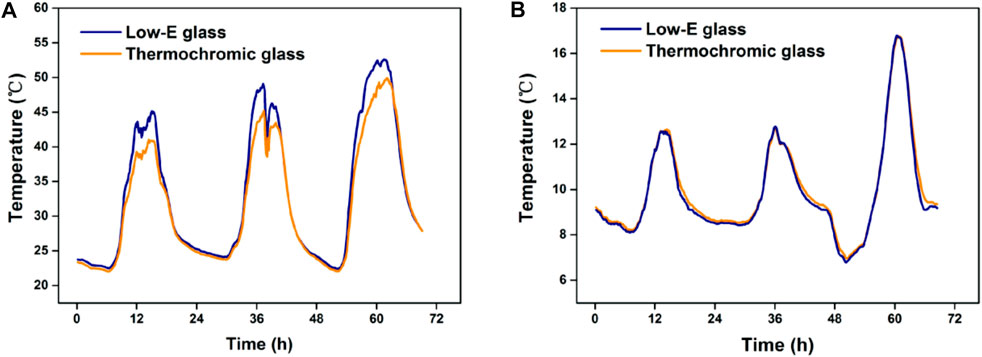
FIGURE 7. Comparison of temperature between thermal dimming glass and Low-E glass sunlight room in summer (A) and winter (B).
Conclusion
This paper investigates the atomization temperature change point of thermochromic glass in hot summer and cold winter zones based on energy consumption simulations and theoretical calculations. Under the climatic conditions of the summer hot and winter cold areas in Chongqing, with the premise of landscape permeability, based on the heat balance relationship of the transparent building envelope and the temperature atomization point of the thermochromic glass were simulated in the presence or absence of air conditioning. The thermal effects of outdoor air temperature, solar radiation, and atmospheric long-wave radiation on the external surface of the glass were considered in the energy simulation and theoretical calculation. Systematic investigations demonstrate that the suitable temperature change point of thermochromic glass under air-conditioned conditions is 39°C, while the value is 42.9°C under non-air-conditioned conditions. This simulation result is exactly consistent with the application environment. In comparison to Low-E glass, thermochromic glass with a temperature change point of 42.9°C can reduce the temperature of the sunlit room by up to 5.07°C, showing a significant contribution to energy consumption.
Data Availability Statement
The raw data supporting the conclusion of this article will be made available by the authors, without undue reservation.
Author Contributions
ZK and LY have contributed equally to this work and share first authorship.
Funding
This work was supported in part by the Project of Hetao Shenzhen-Hong Kong Science and Technology Innovation Cooperation Zone (HZQB-KCZYB-2020083).
Conflict of Interest
ZB was employed by the company China Automotive Engineering Research Institute Co., Ltd.
The remaining authors declare that the research was conducted in the absence of any commercial or financial relationships that could be construed as a potential conflict of interest.
Publisher’s Note
All claims expressed in this article are solely those of the authors and do not necessarily represent those of their affiliated organizations, or those of the publisher, the editors, and the reviewers. Any product that may be evaluated in this article, or claim that may be made by its manufacturer, is not guaranteed or endorsed by the publisher.
Supplementary Material
The Supplementary Material for this article can be found online at: https://www.frontiersin.org/articles/10.3389/fmats.2022.844469/full#supplementary-material
References
Abundiz-Cisneros, N., Sanginés, R., Rodríguez-López, R., Peralta-Arriola, M., Cruz, J., and Machorro, R. (2020). Novel Low-E Filter for Architectural Glass Pane. Energ. Buildings. 206, 109558. doi:10.1016/j.enbuild.2019.109558
Allen, K., Connelly, K., Rutherford, P., and Wu, Y. (2017). Smart Windows-Dynamic Control of Building Energy Performance. Energ. Buildings. 139, 535–546. doi:10.1016/j.enbuild.2016.12.093
Cai, B., Yang, M., Liu, J., Li, J., and Liu, B. (2018). Atomistic Simulation Study of Favored Compositions of Ni-Nb-Al Metallic Glasses. Sci. China Technol. Sc. 61, 1829–1838. doi:10.1007/s11431-018-9342-3
Cai, M., Guo, R., Zhou, F., and Liu, W. (2013). Lubricating a Bright Future: Lubrication Contribution to Energy Saving and Low Carbon Emission. Sci. China Technol. Sc. 56, 2888–2913. doi:10.1007/s11431-013-5403-2
Calas, G., Majérus, O., Galoisy, L., and Cormier, L. (2006). Determination of the thermal Expansion of Cr3+ Sites in Glasses. Appl. Phys. Lett. 88, 121918. doi:10.1063/1.2189111
Cannavale, A., Fiorito, F., Manca, M., Tortorici, G., Cingolani, R., and Gigli, G. (2010). Multifunctional Bioinspired Sol-Gel Coatings for Architectural Glasses. Build Environ. 45, 1233–1243. doi:10.1016/j.buildenv.2009.11.010
Charaya, H., La, T. G., Rieger, J., and Chung, H. J. (2019). Thermochromic and Piezocapacitive Flexible Sensor Array by Combining Composite Elastomer Dielectrics and Transparent Ionic Hydrogel Electrodes. Adv. Mater. Technol. 4, 1900327. doi:10.1002/admt.201900327
Gao, Y., Luo, H., Zhang, Z., Kang, L., Chen, Z., Du, J., et al. (2012). Nanoceramic VO2 Thermochromic Smart Glass: A Review on Progress in Solution Processing. Nano Energy 1, 221–246. doi:10.1016/j.nanoen.2011.12.002
Gong, X., Li, J., Chen, S., and Wen, W. (2009). Copolymer Solution-Based “Smart Window”. Appl. Phys. Lett. 95, 251907. doi:10.1063/1.3276289
Hasan, A., Vuolle, M., and Sirén, K. (2008). Minimisation of Life Cycle Cost of a Detached House Using Combined Simulation and Optimisation. Build Environ. 43, 2022–2034. doi:10.1016/j.buildenv.2007.12.003
Hee, W. J., Alghoul, M. A., Bakhtyar, B., Elayeb, O., Shameri, M. A., and Alrubaih, M. S. (2015). The Role of Window Glazing on Daylighting and Energy Saving in Buildings. Renew. Sust. Energ. Rev. 42, 323–343. doi:10.1016/j.rser.2014.09.020
Iqbal, M. I., Himmler, R., and Gheewala, S. H. (2018). Environmental Impacts Reduction Potential through a PV Based Transition from Typical to Energy Plus Houses in Thailand: A Life Cycle Perspective. Sci. China Technol. Sc. 37, 307–322. doi:10.1016/j.scs.2017.11.028
Javad, K., and Navid, G. (2019). Thermal comfort Investigation of Stratified Indoor Environment in Displacement Ventilation: Climate-Adaptive Building with Smart Windows. Sustain. Cities Soc. 46, 101354. doi:10.1016/j.scs.2018.11.029
Jin, J., Zhang, D., Qin, X., Imran, H. I. A., Yang, Y., Huang, Y., et al. (2019). Remarkable Improved Solar Transmission of VO2 Smart Windows by Partial Controlled Oxygen Plasma Bombarding. Energ. Buildings. 202, 109345. doi:10.1016/j.enbuild.2019.109345
Kharvari, F. (2020). An Empirical Validation of Daylighting Tools: Assessing Radiance Parameters and Simulation Settings in Ladybug and Honeybee against Field Measurements. Sol. Energ. 207, 1021–1036. doi:10.1016/j.solener.2020.07.054
Kim, H.-N., and Yang, S. (2020). Responsive Smart Windows from Nanoparticle-Polymer Composites. Adv. Funct. Mater. 30, 1902597. doi:10.1002/adfm.201902597
Kim, M. H., Kim, J. K., Lee, K. H., Baek, N. C., Park, D. Y., and Jeong, J. W. (2019). Performance Investigation of an Independent Dedicated Outdoor Air System for Energy-Plus Houses. Appl. Therm. Eng. 146, 306–317. doi:10.1016/j.applthermaleng.2018.09.131
Konis, K., Gamas, A., and Kensek, K. (2016). Passive Performance and Building Form: An Optimization Framework for Early-Stage Design Support. Sol. Energ. 125, 161–179. doi:10.1016/j.solener.2015.12.020
Li, W., Luo, X., Yang, P., Wang, Q., Zeng, M., and Markides, C. N. (2022). Solar-thermal Energy Conversion Prediction of Building Envelope Using Thermochemical Sorbent Based on Established Reaction Kinetics. Energ. Convers. Manage. 252, 115117. doi:10.1016/j.enconman.2021.115117
Li, Z., Luo, D., Shi, W., Li, Z., and Liang, X. (2011). Field Measurement of Wind-Induced Stress on Glass Facade of a Coastal High-Rise Building. Sci. China Technol. Sci. 54, 2587–2596. doi:10.1007/s11431-011-4512-z
McCormick, P. G., and Suehrcke, H. (2018). The Effect of Intermittent Solar Radiation on the Performance of PV Systems. Sol. Energ. 171, 667–674. doi:10.1016/j.solener.2018.06.043
Mlyuka, N. R., Niklasson, G. A., and Granqvist, C. G. (2009). Mg Doping of Thermochromic VO2 Films Enhances the Optical Transmittance and Decreases the Metal-Insulator Transition Temperature. Appl. Phys. Lett. 95, 171909. doi:10.1063/1.3229949
Muramoto, K., Takahashi, Y., Terakado, N., Yamazaki, Y., Suzuki, S., and Fujiwara, T. (2020). Fabrication of VO2-Dispersed Glass in B2O3-P2o5-V2o5 System and its Thermal Property. Front. Mater. 7, 00005. doi:10.3389/fmats.2020.00005
Negendahl, K., and Nielsen, T. R. (2015). Building Energy Optimization in the Early Design Stages: A Simplified Method. Energ. Buildings. 105, 88–99. doi:10.1016/j.enbuild.2015.06.087
Nguyen, A.-T., Reiter, S., and Rigo, P. (2014). A Review on Simulation-Based Optimization Methods Applied to Building Performance Analysis. Appl. Energ. 113, 1043–1058. doi:10.1016/j.apenergy.2013.08.061
Pierucci, A., Cannavale, A., Martellotta, F., and Fiorito, F. (2018). Smart Windows for Carbon Neutral Buildings: A Life Cycle Approach. Energ. Buildings. 165, 160–171. doi:10.1016/j.enbuild.2018.01.021
Ruffolo, S. A., and La Russa, M. F. (2019). Nanostructured Coatings for Stone Protection: An Overview. Front. Mater. 6, 00147. doi:10.3389/fmats.2019.00147
Sadineni, S. B., Madala, S., and Boehm, R. F. (2011). Passive Building Energy Savings: A Review of Building Envelope Components. Renew. Sustain. Energ. Rev. 15, 3617–3631. doi:10.1016/j.rser.2011.07.014
Wang, X., and Narayan, S. (2021). Thermochromic Materials for Smart Windows: A State-Of-Art Review. Front. Energ. Res. 9, 800382. doi:10.3389/fenrg.2021.800382
Wu, Y., Liu, H., Li, B., Kosonen, R., Deyu, K., Zhou, S., et al. (2019). Thermal Adaptation of the Elderly during Summer in a Hot Humid Area: Psychological, Behavioral, and Physiological Responses. Energ. Buildings. 203, 109450. doi:10.1016/j.enbuild.2019.109450
Xu, X., Wu, X., Zhao, C., Wang, J., and Ge, X. (2012). Simulation and Improvement of Energy Consumption on Intelligent Glasses in Typical Cities of China. Sci. China Technol. Sc. 55, 1999–2005. doi:10.1007/s11431-012-4854-1
Yang, Z., Yang, Q., Yang, L., Dai, B., Xia, F., Wang, P., et al. (2020). Effect of Thickness on Infrared Optical Property of VO2 Film Deposited by Magnetron Sputtering. Sci. China Technol. Sc. 63, 1591–1598. doi:10.1007/s11431-020-1656-5
Zhang, Y., Tso, C. Y., Iñigo, J. S., Liu, S., Miyazaki, H., Chao, C. Y. H., et al. (2019). Perovskite Thermochromic Smart Window: Advanced Optical Properties and Low Transition Temperature. Appl. Energ. 254, 113690. doi:10.1016/j.apenergy.2019.113690
Zhou, Y., Layani, M., Boey, F. Y. C., Sokolov, I., Magdassi, S., and Long, Y. (2016). Electro-Thermochromic Devices Composed of Self-Assembled Transparent Electrodes and Hydrogels. Adv. Mater. Technol. 1, 1600069. doi:10.1002/admt.201600069
Keywords: energy consumption simulation, thermochromic glass, temperature change point, smart window, low-e glass
Citation: Kai Z, YuDai L, BaoRong Z, Quan S, JinBo W and WeiJia W (2022) Modeling, Simulation and Testing of Atomization Temperature Change Point of Thermochromic Glass for Building Energy Saving. Front. Mater. 9:844469. doi: 10.3389/fmats.2022.844469
Received: 28 December 2021; Accepted: 21 February 2022;
Published: 08 April 2022.
Edited by:
Weihua Li, University of Wollongong, AustraliaReviewed by:
Tongfei Tian, University of the Sunshine Coast, AustraliaShuaishuai Sun, University of Science and Technology of China, China
Copyright © 2022 Kai, YuDai, BaoRong, Quan, JinBo and WeiJia. This is an open-access article distributed under the terms of the Creative Commons Attribution License (CC BY). The use, distribution or reproduction in other forums is permitted, provided the original author(s) and the copyright owner(s) are credited and that the original publication in this journal is cited, in accordance with accepted academic practice. No use, distribution or reproduction is permitted which does not comply with these terms.
*Correspondence: Shi Quan, quanshi@t.shu.edu.cn; Wen WeiJia, phwen@ust.hk