- School of Mechanical Engineering, North University of China, Taiyuan, China
A composite modifier made from waste ethylene-vinyl acetate copolymer (WEVA) and graphene oxide (GO) is used to modify asphalt used to pave the roadway. At the same time, the effect of GO on the related properties of modified composite asphalt is investigated in terms of softening point, penetration, ductility, as well as distribution. The preparation of the composite modifier can reduce the modification’s complexity and enhance the modifier’s dispersion in the asphalt system in the storage phase. By increasing WEVA and GO contents, the softening point of modified composite asphalt increases, and both the penetration and ductility decrease. It is proved that the high-temperature performance of composite asphalt modified by WEVA and GO is better than that of modified asphalt with WEVA. The distribution characteristics of modified asphalt on a fluorescence microscope show that by adding more GO, we get a more even and smaller “bee structure” asphalt system. It indicates that GO can promote dispersion and stop the aggregation of WEVA. The FTIR spectrum results show that the composite modification by adding WEVA and GO is a physical modification, indicating GO can physically prevent the aggregation of the polymers in the storage phase. We present a schematic of the effect on GO and WEVA in asphalt modification to show the improvement in the distribution of the asphalt system from GO. In all, this study provides an idea for the preparation of modified composite asphalt and the application of nano-materials.
Introduction
Waste packaging polymers result in serious environmental pollution and resource wastage, gradually attracting close attention from all walks of life (Saleem et al., 2018). As a polymer modifier applied to asphalt modification, waste ethylene-vinyl acetate copolymer (WEVA) can not only improve the shortcomings of asphalt, such as easy flow in high temperatures and easy cracking in low temperatures, but also promote the recycling of waste packaging polymers (Yu et al., 2017; Yan et al., 2020; Yao et al., 2020). The performance of modified asphalt is a comprehensive embodiment of both modifier performance and asphalt performance. The modifier and modification process have essential impacts on the performance of modified asphalt (Yu et al., 2018). The dispersion of modifiers in the modified asphalt system directly affects the compatibility and storage stability of the modified asphalt (Sun et al., 2021; Zhang et al., 2021). The poor compatibility between the polymer modifier and the asphalt is the reason for the poor storage stability of the modified asphalt system, which is due to the differences in density, molecular weight, polarity, and solubility of the polymer and base asphalt (Guo et al., 2020). The asphalt modified by a single polymer has some defects, such as uneven dispersion of polymers, unstable storage, and easy aging. So, composite modification is usually adopted to improve the performance of bare asphalt (Bala et al., 2020; Xin et al., 2020; Wu et al., 2021).
A high-quality composite modifier of polymer and nanoparticles is necessary for the high-grade paved asphalt to meet the requirements (Jin et al.,2021; Liao et al., 2021; Wu et al., 2021). As nanoparticles, graphene oxide (GO) with unique surface effects, quantum size effects, volume effects, and quantum tunneling effects has excellent abilities to enhance the composite modification (Duan et al., 2019; Zhu et al., 2019; Adnan et al., 2020; Singh et al., 2020; Wang et al., 2020; Fakhri and shahryari, 2021). The decrease in the size of the “bee structure” in the graphene-modified asphalt was primarily related to the factors contributing to the formation of the “bee structures,” which indicated graphene could improve the dispersibility of the modified asphalt (Li et al., 2021). The GO content of 1% can improve the thermal stability of 90A, and the GO can slightly improve the anti-fatigue and anti-cracking performance of the asphalt binders utilized (Li et al., 2018). Through the rutting test, low-temperature bending test, and freeze-thaw splitting test of the asphalt mixture, it was found that the GO–rubber asphalt mixture achieved good road performance (Adnan et al., 2020). The modified asphalt was improved due to the polymer modifier and GO’s dispersibility, and then the formation of the small “bee structures.” The addition of GO can significantly enhance the physical properties of asphalt.
In this subject, the self-prepared graphene oxide (GO) emulsion and the packaging waste ethylene-vinyl acetate copolymer (WEVA) were used as a composite modifier to modify asphalt, which made up the defects of a single modifier or multiple polymer composite modifier. Subsequently, a physical performance analysis and dispersion discussion would clarify the reason for the improvement of modified asphalt and the effects of GO on polymer dispersion. Basic material rules, phase transformation theory, and diffusion theory were introduced to form the schematic to interpret the impact of GO on WEVA in the asphalt system. The research provided an idea for modifying high-performance asphalt and for the application and study of nano-materials in modified asphalt.
Materials and Experiments
Materials
In this experiment, we chose ordinary industrial asphalt 90 produced by Xi’an Petrochemical Factory, and its main properties are shown in Table 1. Recycled waste toys whose main content was ethylene-vinyl acetate copolymer (WEVA) and their box lining were selected as a modifier. They were cleaned and crushed, then granulated by a twin-screw extruder. The granulation process was as follows: mixing in a double-roller, crushing at high speed, then extruding via a twin-screw extruder, grinding, and at last drying. Finally, the WEVA particles were prepared.
The self-prepared GO emulsion in our laboratory was used in this experiment. Sulfuric acid and potassium permanganate were used to oxidize flake graphite. After that, the mixture was cleaned several times and then centrifuged. Finally, the GO emulsion was prepared.
Modification
Based on our research on asphalt composite modification and the preparation of composite modifier (Fang et al., 2015; Zhang et al., 2016), WEVA concentration was varied by 2, 4, and 6 wt%, while GO concentration was varied by 0, 0.02, 0.04, 0.06, 0.08, and 0.1 wt% in the experiment.
If the GO emulsion were directly mixed with the molten raw asphalt over 100°C in modification, the volatilization of moisture in the GO emulsion would lead to the splashing of modified asphalt. In this case, there would be a security risk caused, and it would also not be suitable for the control of the asphalt modification.
The Improvement of This Experiment Is as Follows:
The Preparation of the Composite Modifier
The prepared WEVA particles and the corresponding GO emulsion were mixed with the right amount of xylene in a glass container. At 90 5°C, the mixture was melted and dispersed in an oil bath. WEVA particles could be dissolved in xylene to form a viscous mixture, while GO emulsion was insoluble in xylene. However, GO could be dispersed better in the formation mixed by WEVA particles and xylene under the stirring and moisture volatilization in GO emulsion. And then, the mixture of WEVA and GO was flocculated, filtrated, and dried.
The Preparation of Modified Asphalt
The base asphalt was heated to 170°C until it was completely melted. After that, WEVA and GO prepared modifiers were added to the melted asphalt at 170°C in a reaction kettle. We maintained the mixture temperature. The mixture was stirred for 30 min with a glass stirring rod and then sheared by a shearing machine at a high speed of 4,000 r/min for 80 min. The mixture’s temperature was reduced to 130°C and left undisturbed for 50 min to fully swell. The mixture was sheared again at a high speed of 3,800 r/min for 80 min until the particles of modifiers dispersed uniformly in the asphalt mixture.
Performance Test of the Modified Asphalts
The penetration degree, softening point, and ductility of modified asphalt were measured according to GB/T0604-2000, GB/T0606-2000, and GB/T0605-1993, respectively. The penetration degree of modified asphalt was measured with a GS-IV type automatic asphalt penetrometer (China). The softening point of modified asphalt was tested with a SLR-C type digital softening point tester (China). The ductility was tested with a STYD-3 type digital ductility testing machine (China). In addition, a CX40-RFL32J fluorescent microscope, produced by OLYMPUS, was employed to view the microstructure of the modified asphalt surface distribution. HD-2700 of TEM was used to conduct the tests. FT-IR spectra (Shimazu FTIR-8400S) were used, with a scanning range of 400–5000 cm−1, frequency: 20-Hz meas.
Results and Discussions
Properties of Modified Asphalt
In Figure 1, the horizontal axis represented GO concentration in three types of modified asphalt with 2, 4, and 6% WEVA. Figure 1A shows the softening points of the asphalt composite modified by WEVA and GO. With the same content of WEVA, the softening point increased along with the increase of GO content in three types of modified asphalt. As it could be seen, modified by adding GO, the softening points changed from 52.3 to 56°C in 2%WEVA asphalt, 58.7–64°C in 4% WEVA asphalt, and 62.3–66°C in 6% WEVA asphalt, increasing, respectively, by 3.7, 5.3, and 4.7°C. The changes in the softening point of modified asphalt caused by WEVA were more significant than those caused by GO, indicating that both GO and WEVA could increase the softening point of modified asphalt. Still, the improved high-temperature performance of modified asphalt was mainly determined by WEVA and could be further enhanced by GO.
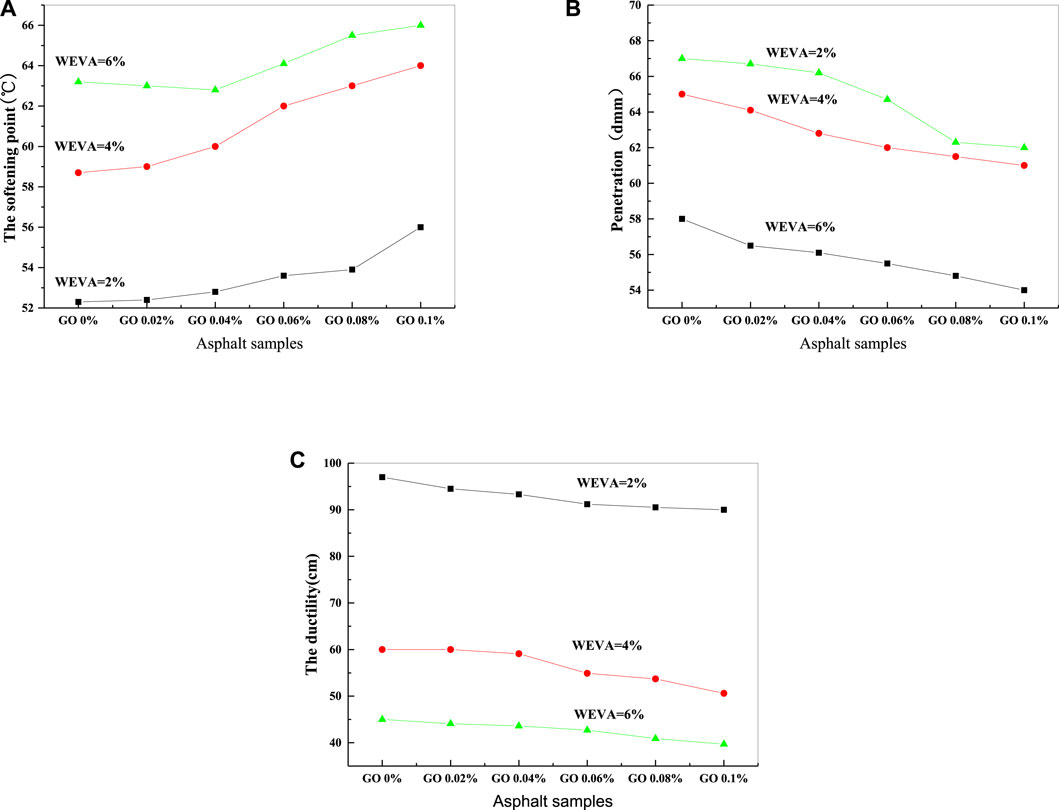
FIGURE 1. Properties of three types of modified asphalt: (A) softening point, (B) penetration, (C) ductility.
The penetrations of three asphalts were modified by 2, 4, and 6% WEVA, respectively, and GO with different contents is shown in Figure 1B. It was shown that the penetration of three kinds of modified asphalt decreased continuously with GO and WEVA. With the same range of GO, for example, at the point “GO 0%,” the decrease in penetration of “WEVA = 6%” modified asphalt was more prominent than that of “WEVA = 2%” and “WEVA = 4%” modified asphalt. The penetration was reduced from 67 to 62 dmm with a decreasing range of 5 dmm in “WEVA = 2%” modified asphalt. The penetration of “WEVA = 4%” modified asphalt dropped from 65 to 61d mm, with a decreasing degree of 4 dmm. The penetration of “WEVA = 6%” modified asphalt fell from 57 to 54 dmm with a decreasing range of 3 dmm. The penetrations of three modified asphalts decreased continuously with the increase of GO, while the decreasing amplitudes were getting smaller along with the increasing WEVA content. It indicated that the change in the penetration of the modified asphalt was mainly affected by the content of WEVA, and GO had an enhanced effect on penetration.
In Figure 1C, the curves of “WEVA = 2%,” “WEVA = 4%,” and “WEVA = 6%” showed the change in ductility of three types of asphalt modified by WEVA and GO. As seen from Figure 1C, when the content of GO was the same, the ductility of the composite modified asphalt decreased as the content of WEVA increased. While with the same range of WEVA, the ductility of the composite modified asphalt gradually reduced with the increase of the content of GO. The ductility decreased from 97 to 90 cm with a decreasing amplitude of 9 cm in “WEVA = 2%” modified asphalt. The ductility of “WEVA = 4%” modified asphalt dropped from 60 to 50.6 cm with a decreasing amplitude of 9.4 cm, and it decreased from 45 to 39.7 cm with a decreasing amplitude of 5 cm in “WEVA = 6%” modified asphalt. By contrast with the decreasing range of ductility for “WEVA = 2%” modified asphalt, it had sharp drops in “WEVA = 4%” and “WEVA = 6%” modified asphalt. The ductility of the asphalt modified by adding WEVA with a content of 2% was 97 cm at “GO 0%.” The decrement was about 30 cm lower than that of the raw asphalt. Throughout the ductility of three kinds of composite modified asphalt, we found that it decreased by nearly 30 cm with each 2% content addition of WEVA. However, the ductility of the composite asphalt modified by WEVA and GO was lower than that of the asphalt modified by WEVA. With the same WEVA content, the ductility of composite modified asphalt decreased within a small range in pace with the increased content of GO. It indicated that the reduced ductility of modified asphalt was mainly affected by the addition of WEVA.
Distribution Characteristics of Modifier
Figure 2 shows the distribution characteristics of modified composite asphalt. Throughout the distribution characteristics of the modified composite asphalt, the asphalt modified by 4% WEVA and GO had serious aggregation of WEVA particles in contrast to that of the modified asphalt with 2% WEVA and GO. It indicated that the aggregation of WEVA particles got more serious along with the increasing content of WEVA. In “a” to “d” or “e” to “h,” under the condition of maintaining the same range of WEVA, the dispersion of WEVA particles was getting more evenly and smaller in the modified composite asphalt system along with the increasing content of GO. It indicated that GO was able to promote the distribution of WEVA in asphalt by stopping the aggregation of WEVA, which enhanced the compatibility of the modified asphalt. The smaller the WEVA particles’ size was, the better the modification was (Huang et al., 2021). It also confirmed that GO could enhance the distribution of the swelled WEVA particles in the modified asphalt multiphase system and then form a spatial network structure, which played a decisive role in distributing WEVA in modified asphalt (Hu et al., 2021; Yu et al., 2021). The dispersion of asphalt modified by 0.8% GO and WEVA is better than that of modified asphalt with other Go content, and the cost of asphalt modification does not increase for the little content of GO.
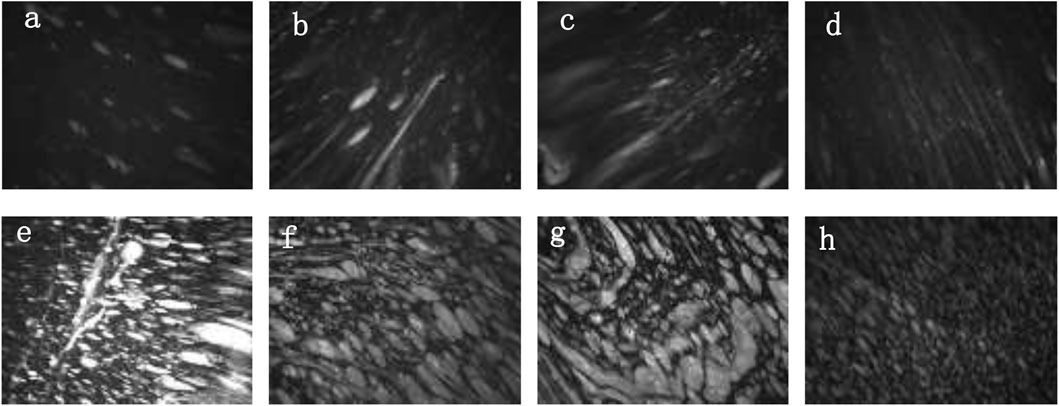
FIGURE 2. Fluorescence microscope of composite asphalt modified by GO and WEVA (A–D) is the asphalt modified by 2% WEVA and GO with, respectively, 0.02, 0.04, 0.06, and 0.08%. (E–H) Modified asphalt including 6% WEVA and GO content, respectively, as 0.02, 0.04, 0.06, 0.08%.
FTIR Analysis
Infrared spectra of the raw asphalt, the asphalt modified by WEVA, and the composite modified asphalt with WEVA and GO are shown in Figure 3. For the modified asphalt and the base asphalt, there was no change in the peaks within 3,000–2,754 cm−1(A). The typical stretching vibration of saturated hydrocarbons and their derivative functional groups of base asphalt are familiar, which means no new substances were created in the modified asphalt. Carbonyl C=O stretching vibration of EVA is observed at 1735 cm−1(B), and the subsequent peaks at 1,600 and 1,450 cm−1(C) are the stretching vibrations of conjugated C=C and C=O in asphalt. The weak absorption peak at 1,242 cm−1 (D) is the C-H bending vibration of an aromatic component. Characteristic peaks within 2,400–1,500 cm−1 are mainly unsaturated functional groups’ stretching vibrations, which are the features of aromatics in modified asphalt. The aromatic characteristic peaks significantly weakened in modified asphalt, while the vibration intensity varied in modified asphalt and stabilized at last. We can conclude that the modifier and asphaltene are striving for the aromatics to form their own stable colloidal structure. The characteristic aromatic peaks of modified composite asphalt weakened significantly as GO increased.. It indicated that GO could enhance the dispersion of EVA and then get more aromatics to form a stable colloidal structure and reach a more stable equilibrium state in the composite modified asphalt system.
Interaction of GO and WEVA
GO was a two-dimensional and lamellar nanometer carbon material with a high specific surface area and large functional groups (Lin et al., 2019; Sali et al., 2019; Hu et al., 2021). There were many polar functional groups on the two-dimensional surface of GO, such as hydroxyl, carbonyl, carboxyl, and epoxy functional groups (Joshi et al., 2021; Li et al., 2022). The TEM of GO in asphalt modified by GO with a content of 0.1% is shown in Figure 4. We can find that GO was aggregated and distributed at the nano-stage without physical changes, including no swelling in the modified asphalt system. It ultimately confirmed why there is no significant improvement in the physical properties of GO-modified asphalt.
We get better physical properties in GO/WEVA-modified asphalt than in WEVA-modified asphalt. In graphene-modified asphalt, the size of the “bee structure” became smaller (Li et al., 2021). In the GO/WEVA-modified composite asphalt, we found that the WEVA particles were dispersed in a micron size. The WEVA particles suspended in the modified asphalt system became smaller and more even, along with the increased content of GO in Figure 2. As there is no improvement in GO-modified asphalt, the progress of composite asphalt modified by GO and WEVA comes from the effect of GO on WEVA particles (Li et al., 2022). Based on the mentioned changes in physical properties of modified asphalt, the respective characteristics of modifier, and their effect on the modification, we conducted the schematic (Figure 5) to clarify the interaction of GO and WEVA in the asphalt modification.
As shown in Figure 5, due to the immense surface and high free energy on surfaces, GO could stop the aggregation of WEVA particles strongly in the storage phase. In modified composite asphalt, the adsorption and deposition of GO nanoparticles on the surfaces of WEVA particles decreased the free energy on the surfaces of GO nanoparticles, suppressed the aggregation of WEVA particles, improved interface binding capacity between the WEVA particles and asphalt, and enhanced the dispersion and swelling of WEVA particles in modified asphalt. GO has stable chemistry and polarity. Asphalt is an organic mixture of hydrocarbons and non-hydrocarbon derivatives. They are incompatible materials as their solubility parameters are being different. However, GO molecules can form hydrogen bonds with asphalt molecules. They can also form aromatic ring stacks with aromatic and resin molecules; these non-bond interactions were the key to strengthening the adsorption between the GO molecules and asphalt (Zeng et al., 2020). Therefore, GO could enhance the formation of the stable dispersion in the modified composite asphalt system, which was good at improving the asphalt modification.
Conclusion
1) The performance of modified composite asphalt is mainly influenced by the addition of WEVA. In contrast, GO can suppress the aggregation of WEVA particles in the modified asphalt system by the adsorption and deposition of GO nanoparticles on the surface of WEVA particles.
2) In the modified composite asphalt system, it is better to control the content of GO by about 0.08%. The cost of the asphalt modification does not become more for the tiny scope of GO. The excessive amounts of GO could not significantly improve the comprehensive performance of modified asphalt.
3) Based on FTIR analysis, no new substance is produced in asphalt modified by GO and WEVA. The modification is physical blending, and it belongs to physical action.
4) The non-bond interactions among GO, WEVA, and asphalt are the key to enhancing the formation of the smaller “bee structures” of the asphalt system and the comprehensive performance of modified asphalt.
Data Availability Statement
The original contributions presented in the study are included in the article/supplementary materials; further inquiries can be directed to the corresponding author.
Author Contributions
MZ conducted the experiments. CL, HW, and BC collected and analyzed the data. MZ and CL drafted and revised the manuscript. CL, MZ, and JW conceived and designed the experiments. The final manuscript was read and approved by all authors. All authors approved the submitted version.
Conflict of Interest
The authors declare that the research was conducted in the absence of any commercial or financial relationships that could be construed as a potential conflict of interest.
Publisher’s Note
All claims expressed in this article are solely those of the authors and do not necessarily represent those of their affiliated organizations, or those of the publisher, the editors, and the reviewers. Any product that may be evaluated in this article, or claim that may be made by its manufacturer, is not guaranteed or endorsed by the publisher.
References
Adnan, A. M., Luo, X., Lü, C., Wang, J., and Huang, Z. (2020a). Improving Mechanics Behavior of Hot Mix Asphalt Using Graphene-Oxide. Constr. Build. Mater. 254, 119261. doi:10.1016/j.conbuildmat.2020.119261
Adnan, A. M., Luo, X., Lü, C., Wang, J., and Huang, Z. (2020b). Physical Properties of Graphene-Oxide Modified Asphalt and Performance Analysis of its Mixtures Using Response Surface Methodology. Int. J. Pavement Eng. 21 (9), 1–15. doi:10.1080/10298436.2020.1804061
Bala, N., Napiah, M., and Kamaruddin, I. (2020). Nanosilica Composite Asphalt Mixtures Performance-Based Design and Optimisation Using Response Surface Methodology. Int. J. Pavement Eng. 21 (1), 29–40. doi:10.1080/10298436.2018.1435881
Duan, S., Li, J., Muhammad, Y., Su, Z., Meng, F., Yang, H., et al. (2019). Synthesis and Evaluation of High‐temperature Properties of Butylated Graphene Oxide Composite Incorporated SBS (C4H9 ‐GO/SBS)‐modified Asphalt. J. Appl. Polym. Sci. 136, 48231. doi:10.1002/app.48231
Fakhri, M., and shahryari, E. (2021). The Effects of Nano Zinc Oxide (ZnO) and Nano Reduced Graphene Oxide (RGO) on Moisture Susceptibility Property of Stone mastic Asphalt (SMA). Case Stud. Constr. Mater. 15, e00655. doi:10.1016/j.cscm.2021.e00655
Fang, C., Zhang, Y., Yu, R., Liu, X., Hu, J., and Zhang, M. (2015). Effect of Organic Montmorillonite on the Hot Storage Stability of Asphalt Modified by Waste Packaging Polyethylene. J. Vinyl Addit Technol. 21 (2), 89–93. doi:10.1002/vnl.21376
Guo, F., Zhang, J., Pei, J., Ma, W., Hu, Z., and Guan, Y. (2020). Evaluation of the Compatibility between Rubber and Asphalt Based on Molecular Dynamics Simulation. J. Front. Struct. Civil Eng. 14 (2), 435–445. doi:10.1007/s11709-019-0603-x
Hu, K., Yu, C., Yang, Q., Chen, Y., Chen, G., and Ma, R. (2021). Multi-scale Enhancement Mechanisms of Graphene Oxide on Styrene-Butadiene-Styrene Modified Asphalt: An Exploration from Molecular Dynamics Simulations. Mater. Des. 208, 109901. doi:10.1016/j.matdes.2021.109901
Huang, J., Liu, Y., Subhan, S., Quan, X., Zhong, H., Li, J., et al. (2021). Fast Deposition of Fe3+ Chelated Tannic Acid Network via Salt Induction over Graphene Oxide Based SBS Modified Asphalt. Constr. Build. Mater. 307, 125009. doi:10.1016/j.conbuildmat.2021.125009
Jin, J., Gao, Y., Wu, Y., Liu, S., Liu, R., Wei, H., et al. (2021). Rheological and Adhesion Properties of Nano-Organic Palygorskite and Linear SBS on the Composite Modified Asphalt. Powder Technol. 377, 212–221. doi:10.1016/j.powtec.2020.08.080
Joshi, D. J., Koduru, J. R., Malek, N. I., Hussain, C. M., and Kailasa, S. K. (2021). Surface Modifications and Analytical Applications of Graphene Oxide: A Review. Trac Trends Anal. Chem. 144, 116448. doi:10.1016/j.trac.2021.116448
Li, S., Xu, W., Zhang, F., Wu, H., and Zhao, P. (2022). Effect of Graphene Oxide on the Low-Temperature Crack Resistance of Polyurethane-SBS-Modified Asphalt and Asphalt Mixtures. Polymers 14 (3), 453. doi:10.3390/polym14030453
Li, X., Wang, Y., Wu, Y., Wang, H., Wang, Q., Zhu, X., et al. (2021). Effect of Graphene on Modified Asphalt Microstructures Based on Atomic Force Microscopy. Materials 14, 3677. doi:10.3390/ma14133677
Li, Y., Wu, S., and Amirkhanian, S. (2018). Investigation of the Graphene Oxide and Asphalt Interaction and its Effect on Asphalt Pavement Performance. Constr. Build. Mater. 165, 572–584. doi:10.1016/j.conbuildmat.2018.01.068
Liao, M., Liu, Z., Gao, Y., Liu, L., and Xiang, S. (2021). Study on UV Aging Resistance of Nano-TiO2/Montmorillonite/Styrene-Butadiene Rubber Composite Modified Asphalt Based on Rheological and Microscopic Properties. Constr. Build. Mater. 301, 124108. doi:10.1016/j.conbuildmat.2021.124108
Lin, M., Wang, Z. L., Yang, P. W., and Li, P. (2019). Micro- Structure and Rheological Properties of Graphene Oxide Rubber Asphalt. J. Nanotechnol Rev. 8, 227–235. doi:10.1515/ntrev-2019-0021
Saleem, J., Adil Riaz, M., and Gordon, M. (2018). Oil Sorbents from Plastic Wastes and Polymers: A Review. J. Hazard. Mater. 341, 424–437. doi:10.1016/j.jhazmat.2017.07.072
Sali, S., Mackey, H. R., and Abdala, A. A. (2019). Effect of Graphene Oxide Synthesis Method on Properties and Performance of Polysulfone-Graphene Oxide Mixed Matrix Membranes. Nanomaterials 9, 769. doi:10.3390/nano9050769
Singh, D., Kuity, A., Girimath, S., Suchismita, A., and Showkat, B. (2020). Investigation of Chemical, Microstructural, and Rheological Perspective of Asphalt Binder Modified with Graphene Oxide. J. Mater. Civ. Eng. 32 (11), 04020323. doi:10.1061/(ASCE)MT.1943-5533.0003385
Sun, X., Yuan, J., Liu, Z., Qin, X., and Yin, Y. (2021). Evaluation and Characterization on the Segregation and Dispersion of Anti-UV Aging Modifying Agent in Asphalt Binder. Constr. Build. Mater. 289, 123204. doi:10.1016/j.conbuildmat.2021.123204
Wang, R., Qi, Z., Li, R., and Yue, J. (2020). Investigation of the Effect of Aging on the Thermodynamic Parameters and the Intrinsic Healing Capability of Graphene Oxide Modified Asphalt Binders. Constr. Build. Mater. 230, 116984. doi:10.1016/j.conbuildmat.2019.116984
Wu, C., Li, L., Wang, W., and Gu, Z. (2021). Experimental Characterization of Viscoelastic Behaviors of Nano-TiO2/CaCO3 Modified Asphalt and Asphalt Mixture. Nanomaterials 11 (1), 106. doi:10.3390/nano11010106
Xin, X., Yao, Z., Shi, J., Liang, M., Jiang, H., Zhang, J., et al. (2020). Rheological Properties, Microstructure and Aging Resistance of Asphalt Modified with CNTs/PE Composites. Constr. Build. Mater. 262, 120100. doi:10.1016/j.conbuildmat.2020.120100
Yan, K., Chen, J., You, L., and Tian, S. (2020). Characteristics of Compound Asphalt Modified by Waste Tire Rubber (WTR) and Ethylene Vinyl Acetate (EVA): Conventional, Rheological, and Microstructural Properties. J. Clean. Prod. 258, 120732. doi:10.1016/j.jclepro.2020.120732
Yao, B., Chen, W., Li, C., Yang, F., Sun, G., Wang, G., et al. (2020). Polar Asphaltenes Facilitate the Flow Improving Performance of Polyethylene-Vinyl Acetateflow Improving Performance of Polyethylene-Vinyl Acetate. Fuel Process. Technol. 207, 106481. doi:10.1016/j.fuproc.2020.106481
Yu, R., Liu, X., Zhang, M., Zhu, X., and Fang, C. (2017). Dynamic Stability of Ethylene-Vinyl Acetate Copolymer/crumb Rubber Modified Asphalt. Constr. Build. Mater. 156, 284–292. doi:10.1016/j.conbuildmat.2017.08.182
Yu, R., Wang, Q., Wang, W., Xiao, Y., Wang, Z., Zhou, X., et al. (2021). Polyurethane/graphene Oxide Nanocomposite and its Modified Asphalt Binder: Preparation, Properties and Molecular Dynamics Simulation. Mater. Des. 209, 109994. doi:10.1016/j.matdes.2021.109994
Yu, R., Zhu, X., Zhou, X., Kou, Y., Zhang, M., and Fang, C. (2018). Rheological Properties and Storage Stability of Asphalt Modified with Nanoscale Polyurethane Emulsion. Pet. Sci. Technol. 36 (1), 85–90. doi:10.1080/10916466.2017.1405028
Zeng, Q., Liu, Y., Liu, Q., Liu, P., He, Y., and Zeng, Y. (2020). Preparation and Modification Mechanism Analysis of Graphene Oxide Modified Asphalts. Constr. Build. Mater. 238, 117706. doi:10.1016/j.conbuildmat.2019.117706
Zhang, H., Duan, H., Zhu, C., Chen, Z., and Luo, H. (2021). Mini-Review on the Application of Nanomaterials in Improving Anti-aging Properties of Asphalt. Energy Fuels 35, 11017–11036. doi:10.1021/acs.energyfuels.1c01035
Zhang, M.-r., Fang, C.-q., Zhou, S.-s., Cheng, Y.-l., and Hu, J.-b. (2016). Modification of Asphalt by Dispersing Waste Polyethylene and Carbon Fibers in it. New Carbon Mater. 31 (4), 424–430. doi:10.1016/S1872-5805(16)60022-7
Keywords: asphalt, graphene oxide, performance, dispersibility, WEVA
Citation: Zhang M, Lian C, Wang J, Wang H and Cheng B (2022) Modification of Asphalt Modified by Packaging Waste EVA and Graphene Oxide. Front. Mater. 9:833593. doi: 10.3389/fmats.2022.833593
Received: 11 December 2021; Accepted: 07 February 2022;
Published: 12 April 2022.
Edited by:
Ahmed A. Abdala, Texas A&M University at Qatar, QatarCopyright © 2022 Zhang, Lian, Wang, Wang and Cheng. This is an open-access article distributed under the terms of the Creative Commons Attribution License (CC BY). The use, distribution or reproduction in other forums is permitted, provided the original author(s) and the copyright owner(s) are credited and that the original publication in this journal is cited, in accordance with accepted academic practice. No use, distribution or reproduction is permitted which does not comply with these terms.
*Correspondence: Junyuan Wang, d2p5QG51Yy5lZHUuY24=