- 1Braunschweig Pavement Engineering Centre (ISBS), Technische Universität Braunschweig, Braunschweig, Germany
- 2Anton Paar Germany GmbH, Ostfildern-Scharnhausen, Germany
A novel Three-Point Bending Beam Test (3-PBT) using a Dynamic Shear Rheometer (DSR) with linear drive motor is introduced for testing asphalt binder at low temperature conditions. This 3-PBT in DSR can be used as surrogate for Bending Beam Rheometer (BBR) tests. Advantages in comparison to BBR are that (i) 3-PBT works without harmful cooling medium ethanol, (ii) the amount of asphalt binder for testing is significantly lower (using material equivalent of 10% of BBR tests), (iii) a silicone rubber mold is used for specimen preparation instead of standard aluminum mold for BBR tests, which makes handling easier, and (iv) the loading protocol is more practical without applying contact load manually. The novel 3-PBT in DSR is presented with respect to preparation, installation, and conditioning of specimens, as well as to the loading protocol. Tests were conducted at temperatures of −10, −16, and −20°C. For the purpose of comparison to BBR tests, and of identifying most suitable testing conditions from alternative setups, a test series was run including 9 different asphalt binders. As to BBR tests, two different cooling media were used, i.e., ethanol and air. Finally, all test results obtained from DSR and BBR tests were analyzed in view of precision and correlation. DSR results correlated well with BBR results, while precision of 3-PBT in DSR was found to be satisfying.
Introduction
Thermal cracking is known as a major mode of deterioration in poorly designed asphalt pavements (Arand, 1983; Arand, 1998; Bouldin et al., 2000; Wistuba et al., 2009; Wistuba, 2019). The specific asphalt mixture composition such as geometric characteristics of aggregates and surface state of the aggregate as well as the choice of the asphalt binder type play an important role in the occurrence and progression of cracks (Isacsson and Zeng, 1998; Pszczola et al., 2018; Li et al., 2019; Sun et al., 2020; Wei et al., 2021). The resistance of asphalt binder to low-temperature is usually addressed via Bending Beam Rheometer (BBR) tests (AASTHO T313-12, 2012; EN 14771, 2012). However, there are some drawbacks reported with BBR testing and new test instruments might be able to replace the rather simple BBR.
Background
Flexural Creep Stiffness Measured in BBR
In BBR tests, the low-temperature flexural creep stiffness and the relaxation properties of the asphalt binder are addressed. For this purpose, an asphalt binder prismatic beam (125 mm in length, 6.25 mm in height, and 12.5 mm in width) is horizontally placed in a cold fluid bath, and is loaded by applying a constant load of 980 mN to the midpoint of the beam. From the measured deflection of the specimen, the flexural creep stiffness is calculated from the actual load and the actual specimen dimensions. Typically, the flexural creep stiffness S at a loading time of 60 s, and the corresponding m-value, which represents the absolute value of the slope of stiffness versus time curve on a double logarithmic scale, are derived at various constant test temperatures in the low temperature range. The characteristic temperatures T (S = 300) and T (m = 0.3) are determined by interpolation, or extrapolation respectively, and serve as key parameters to evaluate the asphalt binder’s resistance to low temperature cracking.
The BBR asphalt binder beam is manufactured using a specific metal mold as defined in AASHTO T313-12 or EN 14771. After demolding, the specimen is conditioned at the test temperature in the fluid bath for about 1 hour using suitable bath fluids like ethanol, methanol or glycol–methanol mixtures (AASTHO T313-12, 2012; EN 14771, 2012). BBR has developed as the primary testing tool for measuring low-temperature characteristics of asphalt binders (mostly long-term aged prior to BBR testing). However, various authors have reported significant drawbacks of BBR testing related to specimen preparation and testing conditions, i.e.,
• Conventional BBR test requires an amount of asphalt binder of 15 g per beam specimen. As usually, three test replicates and three test temperatures are considered, there is need for 135 g of testing material, which requires considerable effort for providing the suitable amount of asphalt binder. In particular in the case of field studies, the amount of available asphalt binder extracted from field cores is significantly constrained (Lu et al., 2017; Wang et al., 2019b).
• Preparation of specimen beams is elaborate, time consuming, and the manual work quality significantly influences accuracy of test results (EN 14771, 2012). In particular, trimming of the bulge and removing from the mold may lead to small but influential deficiencies in specimen shape. Additionally, binders of high viscosity provoke partial breaking or flaking of the specimen, while binders of low viscosity will result in slightly twisted specimen shape.
• Considering the fluid bath medium, ethanol is usually used in Europe (EN 14771, 2012). However, it was reported that ethanol potentially changes chemical and physical binder properties through inhomogeneous diffusion into the asphalt binder and consequently influences the test results (Marasteanu et al., 2018). Moreover, ethanol production is controlled by REACH registrations, as it is toxic, volatile, and has a low flash point (Iman, 2005). REACH is a chemical management regulation that requires the registration, evaluation, approval and restriction of specific chemicals manufactured or imported in the EU. Therefore, various authors studied alternative cooling media, and running BBR tests in air was also investigated, also because the specific heat capacity of air (Cp = 1.012, J g−1 K−1 at room temperature) is smaller than the one of ethanol (Cp = 2.44 J g−1 K−1) (Wilhelm, 2010) and thus the target temperature in air is reached in relatively short time (Cannone Falchetto et al., 2012; Riccardi et al., 2017; Cannone Falchetto et al., 2018; Marasteanu et al., 2018; Moon et al., 2018; Wang et al., 2019a).
• Depending on the specific type of the BBR instrument, tempering the liquid bath takes relatively long (total 60 min, including a stabilization time of 20 min to achieve ± 0.2°C), and moreover, it can be challenging to ensure a homogenous temperature distribution within the bath (EN 14771, 2012; Riccardi et al., 2017).
• During storage of the BBR specimen at constant low temperature, physical hardening was observed (Lu and Isacsson, 2000; Baglieri et al., 2012; Santagata et al., 2016; Wang et al., 2019b; Santosh and Kim, 2019; Zeng et al., 2020; Xu et al., 2021). The specific conditions of storage (temperature and conditioning time) may have a significant effect on BBR test results. Note that physical hardening can also occur prior to DSR testing as observed by Farrar et al. (2015) or Laukkanen et al. (2018b).
• BBR load is usually applied automatically through the instrument system using pressurized air and a pressure valve (Cannon, 2019). Because there is no electrical drive to exactly adjust the position of the loading shaft on the specimen, manual operation is necessary (EN 14771, 2012), and the subsequent pre-defined testing routine is rather complex, consisting of contact loading (between 25 and 45 mN), impulse loading (980 ± 50 mN), contact loading (between 25 and 45 mN), and test loading (between 930 and 1,030 mN). This pre-defined testing routine also allows rather broad tolerances to apply the loads.
• It has been reported that silicone grease was applied to a steel mold to easily separate the mold and specimen, and silicone oil had no effect on the BBR test results (Al-Qadi et al., 2008). Furthermore, some authors identified aluminum an inferior material for specimen molds, and proposed silicone rubber molds instead because of their easier handling and of less consumption of laboratory work compared to aluminum molds (Mamlouk and Zaniewski, 2006; D'Angelo, 2012). Even though the beams produced with silicone molds are slightly thinner, identical test results were found (Dongré et al., 2001). Today, silicone molds are also available from BBR manufacturers, even though they are not represented in the respective standards (EN 14771, 2012; AASTHO T313-19, 2019).
• Having to do the conventional BBR test, involves purchasing, maintenance and calibration costs of an instrument only capable of this specific test. This adds to the cost as well as requires more space in the laboratory, all for just one test.
Low Temperature Properties Measured in DSR
In recent years, the Dynamic Shear Rheometer (DSR) has been established for rheological characterization of asphalt binders in the full temperature range (Radenberg and Gehrke, 2016; Gehrke, 2017; Wistuba et al., 2019; Büchner and Wistuba, 2020; Büchner and Wistuba, 2021; Kim et al., 2021; Radenberg and Staschkiewicz, 2021; Büchner and Wistuba, 2022b). For identifying rheological properties in the low-temperature range, the DSR is usually equipped with a 4 mm plate-plate test setup. With this setup the linear viscoelastic properties can accurately be analyzed using the oscillation mode, as previously demonstrated by various authors (Sui et al., 2011; Lu et al., 2017; Riccardi et al., 2017; Laukkanen et al., 2018a; Büchner et al., 2019; Wang et al., 2019b; Grazulyte et al., 2019; Hajj et al., 2019; Büchner et al., 2020). Alternatively, the relaxation properties, which significantly governs the asphalt mixture low-temperature cracking resistance, can be determined by applying a relaxation test using the quasi-static mode of the DSR (Büchner, 2021; Büchner and Wistuba, 2022a).
Until now DSR test results cannot directly be compared with BBR test results, as the standard DSR setup only allows for measuring shear properties but not bending properties (flexural creep stiffness). However, many authors previously identified correlations between BBR and DSR by converting shear properties to bending properties and time domain to frequency domain using different rheological models (Sui et al., 2011; Farrar et al., 2015; Lu et al., 2017; Riccardi et al., 2017; Wang et al., 2019a; Wang et al., 2019b; Hajj et al., 2019; Zeng et al., 2020). Other authors obtained empirical correlations with linear functions between the viscoelastic values from DSR and the flexural creep values from BBR (Lu et al., 2017; Oshone, 2018). Overall, it can be stated that rheological material properties at low-temperatures can theoretically either be obtained via bending tests in the BBR or via shear test in the DSR with identical significance. However, the acceptance of the 4 mm geometry in the asphalt community is still limited.
In special DSR instruments equipped with a linear drive, a bending beam setup can be installed for running a bending test similar to BBR test, and for directly measuring flexural creep stiffness. Such novel approach using DSR to test beams of asphalt binders under bending load allow for measuring flexural creep stiffness at low-temperature, while eliminating some drawbacks of BBR testing at the same time. Significant advantages of Three-Point Bending Beam Test in DSR in comparison to BBR are that (i) harmful cooling medium ethanol is not used, (ii) the amount of asphalt binder for testing is significantly lower, (iii) there is no need to apply any contact load manually, the effect of which on the test specimen is unknown and (iv) the time to reach equilibrium temperature in the sample and in the tempering cell and thus the total test time can be drastically reduced.
Objectives of This Study
A Three-Point Bending Beam Test (3-PBT) using a Dynamic Shear Rheometer (DSR) with linear drive is introduced for testing asphalt binder at low temperature conditions. The objectives of this study are
• to develop a 3-PBT in DSR suitable to serve as a surrogate for Bending Beam Rheometer (BBR) test,
• to identify suitable specimen dimensions, a procedure to prepare asphalt binder beams for 3-PBT in the DSR, and to carefully analyze the precision of the specimen dimensions,
• to identify suitable duration for temperature conditioning in the DSR testing chamber, and to determine the suitable temperature range for 3-PBT,
• to transfer the BBR loading scheme to the DSR system,
• to analyze repeatability of the novel 3-PBT,
• and to validate the novel test procedure by comparing test results from BBR and DSR considering a variety of different materials and test temperatures, and considering the effect of the cooling medium.
Methodology and Testing
Flexural creep stiffness in three-point-bending is measured of various asphalt binders. All tests are conducted using BBR as well as DSR, at test temperatures −10, −16, and −20°C. As a result, the measured flexural creep stiffnesses obtained from BBR and from DSR are comparatively analyzed, and the suitability of the novel 3-PBT in the DSR is validated.
BBR tests are conducted based on conventional BBR instrument and standard test protocol using ethanol as cooling medium (BBRethanol). In addition, a BBR instrument is used that allows for running the standard test protocol in air instead of ethanol as the cooling medium (BBRair).
DSR tests are conducted based on a modern DSR equipped with a linear drive motor and a test setup especially developed for Three-point Bending Tests (3-PBT). As the bearing distance for 3-PBT in the DSR is limited to 40 mm compared to 102 mm in the BBR, there is need to identify suitable DSR specimen dimensions and a new specimen conditioning protocol, as well as a new test protocol by adopting the applied load to the DSR test geometry.
Materials
In this study, nine different penetration graded asphalt binders are used (Table 1), i.e., three plain binders, and four polymer modified binders, all representing common asphalt binder products of the European market.
All binder samples are subjected to BBR tests in ethanol, BBR tests in air, and DSR tests in air, always considering a number of 3 test replicates (Table 2). Due to material quantity restrictions, for the BBR tests conducted in air, the materials 50/70 (R + P) and 25/55-55 (R + P) are tested only at the lowest temperature of −20°C as this is the worst-case scenario. Additionally, as good correlation is shown between the BBRethanol and BBRair results (cp. Precision of BBR Tests and DSR Tests), extra tests would be redundant, the materials 70/100, 40/100-65, 45/80-50 are not tested at −16°C in the DSR also due to material restrictions and availability of laboratory equipment.
Conventional BBR Tests
For conventional BBR tests using ethanol as cooling medium (BBRethanol), the instrument Cannon TE-BBR is used. Standard tests are run (in ethanol fluid bath) according to AASTHO T313-19 (2019), EN 14771 (2012).
Standard metal molds are used to prepare the prismatic specimens with standard dimensions of 125 mm × 6.35 mm × 12.5 mm, and prior to testing, the specimens are conditioned to the test temperature (tolerance of ±0.2°C) in the fluid bath for 60 min.
According to the standard test protocol, testing consists of four stages. First, a contact load of 35 ± 10 mN is manually adjusted and applied for a short time to the asphalt binder beam to ensure contact of the specimen with the support and the load frame. Then, the software automatically applies the test load of 980 ± 50 mN for one second before returning to the contact load and holding it for 20 s. Finally, the test load of 980 mN is applied for 240 s and the resulting beam deflection is measured with a data recording interval of one second. In the end, the load returns to the contact load (35 ± 10 mN).
BBR Tests in Air
For running BBR tests in cooling medium air (BBRair), the instrument Cannon TE-BBR Pro™ is used. The procedures for specimen preparation, installation and conditioning, as well as the test protocol exactly followed standard BBR test (cp. Section 3.2). Note that a fan is installed inside the chamber to ensure homogeneous temperature distribution.
Bending Tests in DSR
Instrument and Test Setup
For DSR tests, the instrument MCR 702 MultiDrive rheometer from manufacturer Anton Paar (Graz, Austria) is used (Figure 1). The instrument is equipped with a linear drive motor and a three-point bending measuring system with a free length of 40 mm (TPB40). This measuring system is designed for bending tests. For specimen conditioning, the DSR is equipped with a convection oven device CTD 180, which covers a temperature range from −20 to 180°C. This convection oven can be used for temperature control with inert gas (e.g., N2) or directly with the air. For the experiments presented here, air is used to control the temperature. The TPB40 measuring system contains a Pt100 close to the sample location. The temperature system is calibrated using an external calibration system consisting of a digital thermometer and a temperature sensor placed in the sample itself. Under these conditions, the accuracy of the CTD 180 in combination with the TPB40 is the equivalent of a Pt100 class A. For a temperature of −20°C this corresponds to a minimum accuracy of ± 0.19°C. For temperatures of −16°C and −10°C, the accuracy corresponds to ± 0.18°C and ± 0.17°C, respectively.
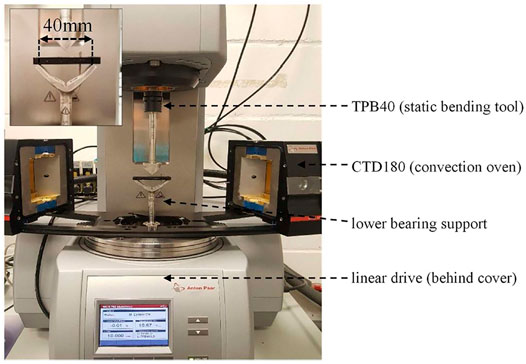
FIGURE 1. DSR instrument of the type MCR 702 MultiDrive Rheometer (Anton Paar) used for Three-Point Bending Tests.
The geometry of the new specimen is carefully selected so as not to generate disturbances in the bending test that could arise from the new size. The free length of the TPB40 measuring system in the DSR (
In the case of BBR tests, the dimensions of the specimen are usually assumed to be identical to the dimensions of the predetermined mold size. For the case of the DSR tests performed in this study, the width and height of the specimen are actually measured. In particular, the mean value of 8 individual measurements is determined. Measured widths ranged from 4.9 to 5.2 mm, and coefficient of variation was below 2%, measured heights ranged from 2.4 to 3.2 mm, and coefficient of variation was below 5%.
The test load is adjusted to the new specimen geometry to be such that the maximum stress generated during the DSR test is the same as in the BBR tests (cp. Eq. 1).
The resulting maximum stress for a rectangular specimen under a load in a three-point bending setup is given by the formula below (Grellmann and Seidler, 2013) (cp. Eq. 2),
where,
The free length,
Specimen Preparation and Loading Scheme
Figure 2 illustrates the manufacturing process of the specimens. The specimen is fabricated using a heat-resistant two-component silicone mold. First, the asphalt binder is slowly poured into the silicone mold, while taking care that no air bubbles are included (Figure 2A). After 15 min, the excess material is trimmed with a hot spatula (Figure 2B). Afterwards, the specimen is cooled to a temperature of −18°C for a duration of 3 min, so that it can easily be demolded from the silicone mold (Figure 2C). For unmolding the silicone mold including the specimen is held upside down. By slightly bending the silicon mold on both sides the specimen in loosened and can be placed on a flat surface without any deformation (Figure 2D). Silicone has excellent self-lubricating properties so that the binder specimen can be demolded without using a separating agent. This also reduces manufacturing time if compared to BBR tests using metal molds.
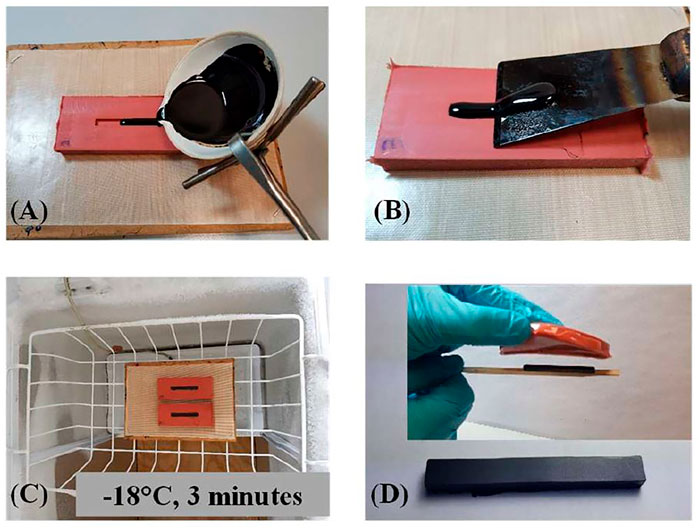
FIGURE 2. Specimen preparation: (A) Pouring into silicone mold, (B) Trimming of specimen using a heated spatula, (C) Conditioning at −18°C for 3 min, (D) demolding of specimen.
Conditioning of specimen is carried out in two stages, lasting 1 h in total (as for BBR tests): For the first 30 min, the unmolded specimen is stored in a separate conditioning chamber on top of a flat silicon plate (Figure 3A). For the remaining 30 min, the specimen is aligned to the bearing supports and to the central upper load geometry (lifted up), and it is then conditioned by the convection oven of the DSR instrument (Figure 3B).
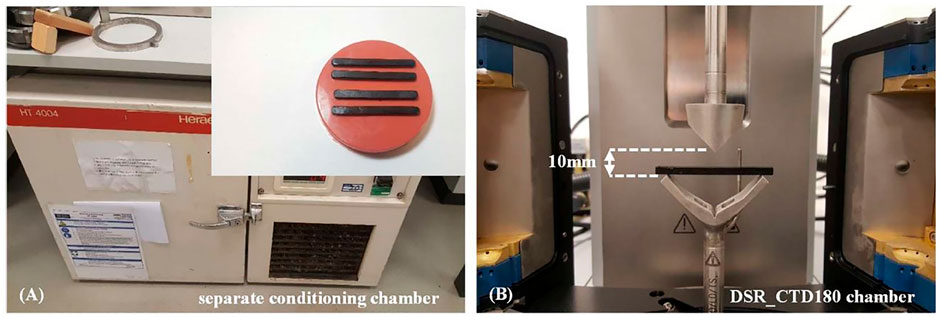
FIGURE 3. (A) Silicone specimen molds placed in conditioning chamber, (B) specimen placed on bearings.
After 30 min, the upper measuring system is lowered with a speed of 600 µm until it lightly touches the specimen surface. A sufficiently small force within the viscoelastic limit of the sample was used for the first contact between the measuring system and the specimen. This ensures that the first contact between the upper measuring system and the specimen does not alter the subsequent measurement. Hence, unlike to standard BBR tests using pneumatic pressure, there is no need to manually control the contact load.
Then, the load of 220 mN is applied to the specimen for 240 s. Measurement points are obtained every 0.25 s. At the end of the test, the load is immediately removed. Figure 4 visualizes the loading scheme in comparison to the one of conventional BBR test.
Calculation of Flexural Creep Stiffness
Flexural creep stiffness is considered a key parameter for evaluating the binders’ resistance to low-temperature cracking. Based on beam theory, the flexural creep stiffness can be calculated from Eq. 4 (see for instance Grellmann and Seidler, 2013).
where, S(t) [MPa] is the flexural creep stiffness at time t, F [N] is test load, L0 (mm) is the distance between the bearings, b (mm) is width of specimen, h [mm] is thickness of specimen, δ(t) (mm) is the deflection of the specimen at time t.
Flexural creep stiffness is measured after t = 8, 15, 30, 60, 120, and 240 s. A quadratic polynomial regression function is used to fit these data (cp. Eq. 5).
where, Sc(t) [MPa] is calculated flexural creep stiffness at loading time t [s], and A, B and C are regression coefficients. From the regression line represented by Eq. 5, the m-value can be derived, representing the slope at an arbitrary time t in the time-flexural creep stiffness diagram (when using logarithmic scales), reading (Eq. 6)
In this study, flexural creep stiffness S60 at 60 s was determined from Eq. 5, and m60 from Eq. 6 respectively, for every individual test specimen. From three test replicates the mean values for S60 and m60 are calculated. The mean values derived for different temperatures are shown in Figure 5. With increase in temperature the flexural creep stiffness S60 decreases, while m60 increases. Further, the characteristic temperatures corresponding to S = 300 MPa and to m = 0.3 are determined from data interpolation or extrapolation respectively, designated as T (S = 300) and T (m = 0.3) (Figure 5). Note that extrapolation was necessary for soft binders having temperatures T (S = 300) below −20°C (such as 70/100, 45/80-50, and 40/100-65), because the minimum test temperature of the DSR instrument used in this study was limited to −20°C.
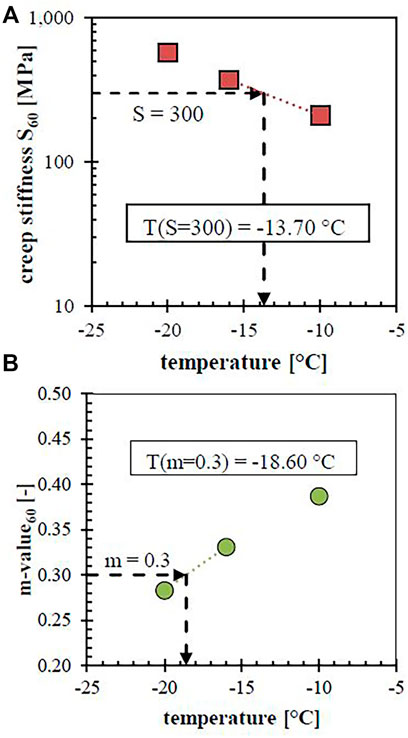
FIGURE 5. Example for determining characteristic temperatures of asphalt binder 10/40-65: (A) T (S = 300), and (B) T (m = 0.3).
Precision of BBR Tests and DSR Tests
Precision of any test method is addressed by calculating the mean value and the coefficient of variation from a number of three test replicates for any binder sample. The coefficient of variation is a measure of the relative variability of test result with respect to the mean value, and hence, it is useful for comparing data series with different mean values. It refers to the ratio of the standard deviation to the mean value. A large coefficient means that the standard deviation of the data range is rather large compared to the mean value.
In Figure 6 the coefficient of variation (CV) is depicted for always three replicates of the flexural creep stiffness measured at −20°C with BBR (considering both cooling media ethanol and air) and with DSR.
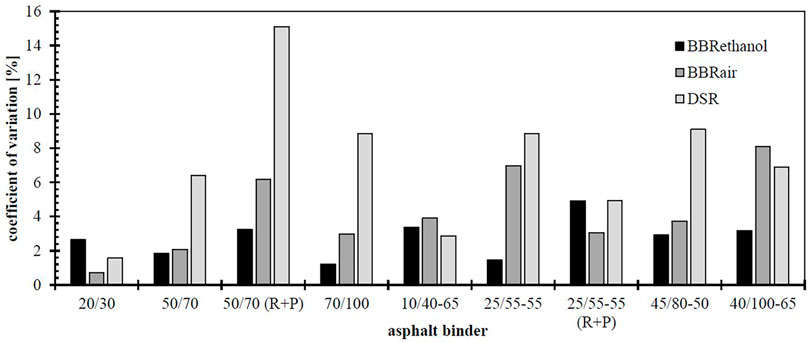
FIGURE 6. Comparison of coefficient of variation of flexural creep stiffness at −20°C of BBRethanol, BBRair, and DSR.
From these results it can be stated, that for DSR tests the coefficient of variation is less than 10%, except for binder 50/70 (R + P), while for BBR tests it is even less than 8%.
Test Results and Evaluation
Flexural creep stiffness and corresponding m-value are obtained from bending tests using BBR and ethanol as cooling medium (BBRethanol), BBR and air as cooling medium (BBRair), and DSR. While the results from BBRethanol and BBRair were comparatively analyzed to determine the effect of the cooling medium on the test results, the results of BBRethanol and DSR were comparatively analyzed to evaluate the novel three-point bending test in DSR.
Correlating the Results Obtained From BBRethanol Tests and BBRair Tests
Figure 7 shows mean flexural creep stiffnesses (S60) of all investigated binders and temperatures as obtained from BBRethanol and BBRair tests. Colors are used to differentiate binders, while different shapes are used to indicate different test temperatures. The absolute deviation of individual test results from the mean value are also displayed.
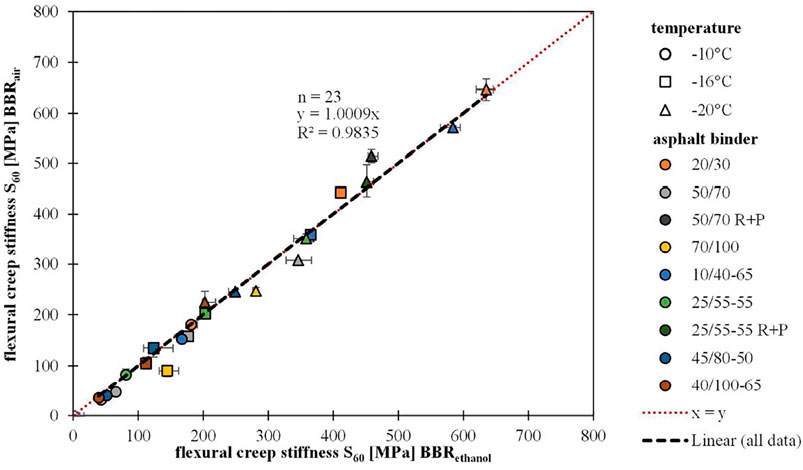
FIGURE 7. Correlating flexural creep stiffnesses (S60) obtained from BBRethanol tests and BBRair tests.
Flexural creep stiffness always increases with decreasing temperature. A linear trend line is drawn with the intercept value set to zero. All data (n = 23) are almost evenly distributed along the linear regression line regardless of the asphalt binder type, aging state, and test temperature. It can also be observed that the deviations of the individual test results from the mean values are quite small and within the precision of the test method. Data obtained from BBRethanol and BBRair are linked via a coefficient of determination of 0.98.
It is noteworthy that in comparison with the results of previous study (Riccardi et al., 2017), which presumably applied AASTHO T313-12 (2012) to evaluate the effects of the cooling medium on the low PG value of the asphalt binder, BBRethanol and BBRair tests almost result in same stiffnesses S60. If testing conditions and derivation of results is carried out in a proper manner, there is hardly any difference between BBR tests run according to EN 14771 (2012) either in ethanol or in air.
In analogy, Figure 8 shows mean m-values (m60) of all investigated binders and temperatures as obtained from BBRethanol and BBRair tests. Value m60 decreases with decreasing temperature, which is explained by the fact that relaxation capability decreases with decreasing temperature.
Again, a linear regression line is drawn, and all data (n = 23) are found to be almost evenly distributed along this line regardless of the asphalt binder type, aging state, and test temperature. Data obtained from BBRethanol and BBRair are linked via a coefficient of determination of 0.94. However, at a temperature of −10°C the m-values60 of binders 50/70, 70/100, and 25/55-55 shows rather large deviations between BBRethanol and BBRair tests, and from the mean values obtained from BBR tests in air.
It is stated, that the obtained BBR results are very similar independently of the cooling medium (ethanol or air), both in terms of flexural creep stiffness and m-value. In summary it is concluded that the obtained values are accurate and correct as they are similarly obtained with two different rheometers, and that the choice of cooling medium, ethanol or air, does not influence the test result.
Correlating Results Obtained From BBR Tests and DSR Tests
Flexural Creep Stiffness and Characteristic Temperature T(S = 300)
Figure 9 shows mean flexural creep stiffnesses (S60) of all investigated binders and temperatures as obtained from standard BBR tests (BBRethanol) and DSR tests. Colors, shapes, and deviations from mean values are displayed in analogy to Figure 7, and again the data are found along the linear regression line.
Deviations of the individual test results from the mean value are quite similar. Data obtained from BBR and DSR are linked via a coefficient of determination of 0.96. It is concluded that both test methods deliver similar results.
Stiffnesses determined at a temperature of −20°C are illustrated in the bar chart in Figure 10, which demonstrates the similarity of test results obtained from BBR tests and DSR tests.
Temperatures T (S = 300) are calculated from the stiffness values obtained from BBR tests and DSR tests at different temperatures (Figure 11). It is found that data obtained from BBR and DSR are linked via a coefficient of determination of 0.94.
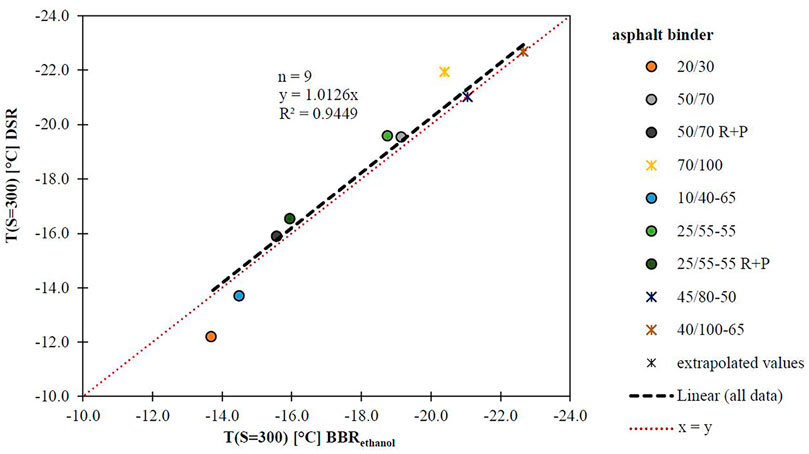
FIGURE 11. Correlating characteristic temperatures T (S = 300) obtained from BBR tests and DSR tests.
m-Value and Characteristic Temperature T(m = 0.3)
Figure 12 shows mean m-values (m-value60) of all investigated binders and temperatures as obtained from standard BBR tests (BBRethanol) and DSR tests.
Deviations of the individual test results from the mean value are quite similar, and DSR data are slightly higher. However, data obtained from BBR and DSR are linked via a coefficient of determination of 0.90.
Note that the difference of BBR and DSR results is not consistent for a specific material. E.g., for asphalt binder 20/30 tested at −16°C the m-value from DSR is approximately 14% above the m-value from BBR, while at −10°C the m-value from DSR is approximately 3% below the m-valued from BBR. At the same time, for asphalt binder 25/55-55 RTFOT + PAV the m-value from DSR is between 6 and 24% above the m-value from BBR. It could be that the stress applied to the specimen (despite being equivalent in both BBR and DSR systems) is slightly above the viscoelastic limit for some of the materials. This would induce a non-linearity in the system. In that case, the fit used in Eq. 5 and its derivative with time as shown in Eq. 6 for the evaluation of the m-value could lose its pragmatic sense as a universal material function. In such a non-linear scenario, the parameters are strongly affected by test apparatus or chosen test conditions. This type of non-linearity actually makes interpretation and equivalence of results difficult when different devices come into play.
Temperatures T (m = 0.3) are calculated from the m-values obtained from BBR tests and DSR tests at different temperatures (Figure 13). It is found that data obtained from BBR and DSR are linked via a coefficient of determination of 0.55, indicating an inferior quality of correlation. This is because the characteristic temperature T (m = 0.3) is a very sensitive parameter. Although m-values obtained from DSR and BBR are rather similar (cp. Figure 12), the results for temperatures T (m = 0.3) are significantly different. For softer binders 70/100, 50/70, 45/80-50, 40/100-65, 25/55-55 the difference between DSR and BBR is smaller compared to the results for harder (and aged) binders 20/30, 10/40-65, 50/70 (R + P), and 25/55-55 (R + P), tested at −16°C and −20°C. Additionally, the measurements were made at only three temperatures values (−10, −16, and −20°C) and the T (m = 0.3) is linearly interpolated (or extrapolated) from the data obtained at these discrete temperature values. A linear interpolation as suggested in (AASTHO T313-12, 2012; EN 14771, 2012) could lead to a strong oversimplification. Note that, a non-linear dependence with temperature of viscoelastic parameters such as creep compliance or flexural creep stiffnesses is expected.
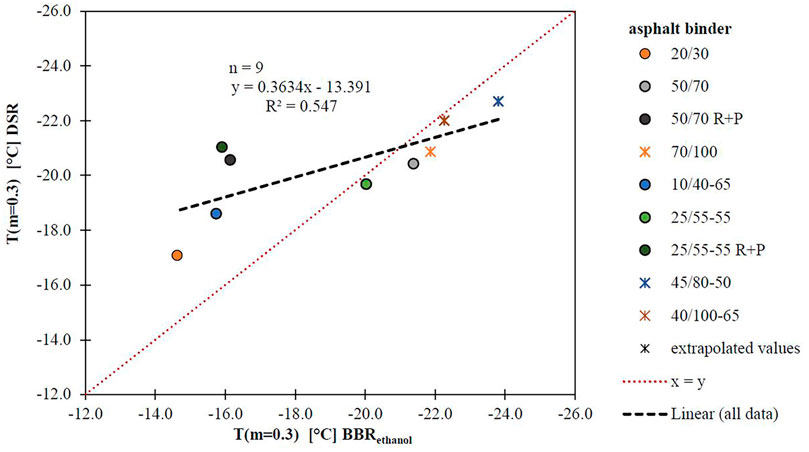
FIGURE 13. Correlating characteristic temperatures T (m = 0.3) obtained from BBR tests and DSR tests.
From the results of T (S = 300) and T (m = 0.3) the parameter ΔTc was calculated by simple subtraction. The ΔTc-values are significantly affected by the very sensitive parameter T (m = 0.3) and therefore no sound correlation could be identified between DSR and BBR tests.
Summary and Conclusions
In this study, a novel Three-Point Bending Beam Test (3-PBT) using a Dynamic Shear Rheometer (DSR) with linear drive motor is presented for testing asphalt binder at low temperature conditions. It can be used as a surrogate for Bending Beam Rheometer (BBR) tests. Significant advantages in comparison to BBR are that
(i) 3-PBT works without harmful cooling medium ethanol, but in air,
(ii) the specimen geometry and the thermal chamber are considerably smaller. Therefore, reaching thermal equilibrium not only in the specimen but also in the measuring cell requires less time, Note that the DSR can save up to 30 min compared to BBR to achieve the required thermal equilibrium.
(iii) the amount of asphalt binder for specimen fabrication (with dimensions of 50 mm³ × 5 mm³ × 3 mm³) is around 1.2 g and therefore significantly lower, using a material equivalent of 10% of BBR tests only,
(iv) a silicone rubber mold is used for specimen preparation instead of standard aluminum mold for BBR tests, which makes handling easier and saves time,
(v) the loading protocol is more practical without any need to apply contact load manually, the effect of which on the test specimen is unknown,
(vi) one and the same DSR instrument can be used for the determination of characteristic rheological binder properties in shear mode and in bending mode. This can save costs of purchasing and maintenance of a separate instrument and save space in the laboratory.
Depending on the accessories and the manufacturer of each device, the price of a DSR including an axial system for 3-PBT can range from two to three times the price of a BBR. Initial costs are therefore slightly higher than BBR. The aforementioned advantages, however, may still show DSR as an alternative to BBR. The temperature control system used in this study (CTD 180) is based on a convection oven in combination with a Peltier and therefore achieves a minimum temperature of −20°C. To reach lower temperatures it would be necessary to use another type of furnace which can be directly coupled to liquid nitrogen (minimum temperature of −160°C) or to an external chiller (e.g., a gas cooling unit, capable of cooling the air inside the cell down to −90°C). This kind of system would be necessary if the BBR is to be fully replaced.
For the purpose of comparison to BBR tests, and of identifying most suitable testing conditions from alternative setups, a test series was run including 9 different asphalt binders ranging from soft to hard (aged) types, and tests were conducted at temperatures of −10, −16, and −20°C.
As to BBR tests, two different instruments were used for the purpose of identifying any influence of the cooling medium, i.e., ethanol and air.
From DSR and BBR tests, the flexural creep stiffness after 60 s (S60) and the corresponding m-value after 60 s (m-value60) were calculated for each test temperature. In addition, the characteristic temperatures T (S = 300) and T (m = 0.3) were determined. All test results obtained from DSR and BBR tests were analyzed in view of correlation and precision. From this analysis, the following conclusions were drawn:
• The cooling medium (ethanol or air) did not affect BBR test results. The degree of determination between BBR in ethanol and BBR in air was in the range of 0.94–0.98. The deviation of individual results measured in air was found to be slightly higher compared to results measured in ethanol.
• Flexural creep stiffness obtained in DSR tests was almost identical to the one obtained in BBR tests. Based on a number of 24 different test results considering three replicates, the slope of the linear regression line was 0.97, and the degree of determination was 0.96. Further, the characteristic temperature T (S = 300) was found to be similar for DSR and BBR tests, with a degree of determination of 0.94. However, especially for hard binders the value obtained from DSR tests was slightly smaller compared to the one obtained from BBR tests.
• In terms of the m-value, slight differences were identified between DSR and BBR tests, the degree of determination was found to be 0.90. Regarding the characteristic temperature T (m = 0.3) no significant correlation was identified between DSR and BBR tests. The temperature T (m = 0.3) parameter appear to be very sensitive to the type of instrument used.
• Based on the coefficient of variation of three replicates the repeatability of different instruments was evaluated. For conventional BBR tests (in ethanol), a coefficient of variation in the range of 1–5% was identified, while for measuring in air it ranged from 1 to 8%. As concerned DSR tests, the coefficient of variation was higher than for BBR tests for almost all materials, demonstrating slightly lower repeatability. However, as DSR results correlated well with BBR results, precision of 3-PBT was found to be satisfying.
It is assumed that the repeatability of the 3PBT in the DSR could be improved after more routine testing, as this was just a preliminary study to demonstrate applicability of the novel method.
Data Availability Statement
The original contributions presented in the study are included in the article/Supplementary material, further inquiries can be directed to the corresponding author.
Author Contributions
YK: Methodology, Investigation, Data curation, Formal analysis, Writing—original draft. JB: Research Concept and Supervision, Writing—original draft. MW: Funding acquisition, Resources, Writing—significant editing of the original draft. JA: Instrument and Software Support, Methodology, Writing—review and editing. MR: Writing—review and editing. MS: Funding acquisition, Resources, Writing—review.
Conflict of Interest
Author JRA, MR and MS are employed by Anton Paar Germany GmbH.
The remaining authors declare that the research was conducted in the absence of any commercial or financial relationships that could be construed as a potential conflict of interest.
Publisher’s Note
All claims expressed in this article are solely those of the authors and do not necessarily represent those of their affiliated organizations, or those of the publisher, the editors and the reviewers. Any product that may be evaluated in this article, or claim that may be made by its manufacturer, is not guaranteed or endorsed by the publisher.
Acknowledgments
Gerhard & Karin Matthäi Foundation is acknowledged for financial support of research work of the first author. We acknowledge support by the Open Access Publication Funds of Technische Universität Braunschweig.
References
AASTHO T313-12 (2012). Standard Method of Test for Determining the Flexural Creep Stiffness of Asphalt Binder Using the Bending Beam Rheometer (BBR). Washington, D.C. American Association of State Highway and Transportation Officials.
AASTHO T313-19 (2019). Standard Method of Test for Determining the Flexural Creep Stiffness of Asphalt Binder Using the Bending Beam Rheometer (BBR). Washington, D.C. American Association of State Highway and Transportation Officials.
Al-Qadi, I. L., Yang, S.-H., Elseifi, M., Dessouky, S., Loulizi, A., Masson, J.-F., et al. (2008). Characterization of Low Temperature Creep Properties of Crack Sealants Using Bending Beam Rehometry, University of Illinois at Urbana-Champaign, Technical Report FHWA-ICT-08-029.
Arand, W. (1998). Einfluss von Temperatur und Temperaturrate auf den Verformungswiderstand frisch verlegter Asphaltdeckschichten während abkühlung und Wiedererwärmung. Asphalt, Heft 4, 33–34.
Arand, W. (1983). Zum Einfluss tiefer Temperaturen auf das Ermüdungsverhalten von Asphalten. Straße und Autobahn, Jahrgang 34, Heft 10. Bonn: Kirschbaum Verlag, 424–431.
Baglieri, O., Dalmazzo, D., Barazia, M., Tabatabaee, H. A., and Bahia, H. U. (2012). Influence of Physical Hardening on the Low-Temperature Properties of Bitumen and Asphalt Mixtures. Proced. - Soc. Behav. Sci. 53, 504–513. doi:10.1016/j.sbspro.2012.09.901
Bouldin, M. G., Dongre, R. N., Rowe, G. M., Sharrock, M. J., and Anderson, D. A. (2000). Predicting Thermal Cracking of Pavements from Binder Properties - Theoretical Basis and Field Validation. J. Assoc. Asphalt Paving Technologists 69, 455–496.
Büchner, J. (2021). Prüfung von Asphaltmastix im Dynamischen Scherrheometer. Dissertation, Technische Universität Braunschweig. Braunschweig: Institut für Straßenwesen, Schriftenreihe Straßenwesen. doi:10.24355/dbbs.084-202107151007-0
Büchner, J., and Wistuba, M. P. (2022a). “Analysis of Low Temperature Relaxation Properties of Asphalt Binder and Asphalt Mastic Using a Dynamic Shear Rheometer, Accepted for Publication,” in Proc., 1th International Conference on the Bearing Capacity of Roads, Railways and Airfields, Trondheim, Norway, June 28-30, 2022.
Büchner, J., and Wistuba, M. P. (Forthcoming 2022b). Assessing Creep Properties of Asphalt Binder, Asphalt mastic and Asphalt Mixture. Road Mater. Pavement Des., 1–15. doi:10.1080/14680629.2021.2020153
Büchner, J., Wistuba, M. P., Dasek, O., Staschkiewicz, M., Soenen, H., Zofka, A., et al. (Forthcoming 2020). Interlaboratory Study on Low Temperature Asphalt Binder Testing Using Dynamic Shear Rheometer with 4 Mm Diameter Parallel Plate Geometry. Road Mater. Pavement Des., 1–17. doi:10.1080/14680629.2020.1851291
Büchner, J., and Wistuba, M. P. (2021). Entwicklung einer Methodik zur Bewertung der Bindemittel- und Mastixeigenschaften im Asphaltstraßenbau, Schlussbericht zum Forschungsprojekt Bit-Q, finanziert durch die Arbeitsgemeinschaft „ARGE Bit-Q“ bestehend aus Eiffage Infra-Südwest, Kemna, Leonhard Weiß, Matthäi, TPA/Strabag, Winkler. Braunschweig: Technische Universität Braunschweig, Institut für Straßenwesen.
Büchner, J., and Wistuba, M. P. (2020). “Relating Asphalt Mixture Performance to Asphalt Mastic Rheology,” in Proceedings of the 9th International Conference on Maintenance and Rehabilitation of Pavements - Mairepav9, Dübendorf, Switzerland, July 01-03, 2020. Editor C. Raab (Springer), 639–649.76
Büchner, J., Wistuba, M. P., Remmler, T., and Wang, D. (2019). On Low Temperature Binder Testing Using DSR 4 Mm Geometry. Mater. Struct. 52, 113. doi:10.1617/s11527-019-1412-3
Cannon (2019). Thermo-Electric Bending Beam Rheometer, Instruction & Operation Manual. State College, PA: Cannon Instrument Company.
Cannone Falchetto, A., Moon, K. H., Wang, D., and Riccardi, C. (2018). Investigation on the Cooling Medium Effect in the Characterization of Asphalt Binder with the Bending Beam Rheometer (BBR). Can. J. Civ. Eng. 45 (7), 594–604. doi:10.1139/cjce-2017-0586
D'Angelo, J. A. (2012). “Polyphosphoric Acid Modification of Asphalt Binders,” In:Transportation Res. Circular E-C160. A Workshop, Minneapolis, Minnesota, April 7–8, 2009 (Washington: Transportation Research Board).
Dongré, R., D’Angelo, J., and Antle, C. (2001). Effect of Silicone Rubber Molds on Low-Temperature Binder Grading Parameters: Bending Beam Rheometer S[60] and M-Value. Transportation Res. Rec. 1766, 24–31. doi:10.3141/1766-04
EN 12607-1 (2014). Bitumen and bituminous binders – Determination of resistance to hardening und influence of heat and air – Part 1: RTFOT method. Brussels: European Committee for Standardization.
EN 14769 (2012). Bitumen and Bituminous Binders - Accelerated Long-Term Ageing Conditioning by a Pressure Ageing Vessel (PAV). Brussels: European Committee for Standardization.
EN 14771 (2012). Bitumen and Bituminous Binders – Determination of the Flexural Creep Stiffness – Bending Beam Rheometer (BBR). Brussels: European Committee for Standardization.
Falchetto, A. C., Turos, M. I., and Marasteanu, M. O. (2012). Investigation on Asphalt Binder Strength at Low Temperatures. Road Mater. Pavement Des. 13 (4), 804–816. doi:10.1080/14680629.2012.735793
Farrar, M. J., Sui, C., Salmans, S., and Quin, Q. (2015). Determining the Low-Temperature Rheological Properties of Asphalt Binder Using a Dynamic Shear Rheometer (DSR). Technical White Paper. Laramie, WY: Western Research Institute.
Gehrke, M. (2017). Komplexe Charakterisierung bitumenhaltiger Bindemittel anhand temperatur-, frequenz- und belastungsabhängiger Kennwerte. Dissertation, Ruhr-Universität Bochum, Lehrstuhl für Verkehrswegebau, 27. Bochum: Schriftenreihe Lehrstuhl für Verkehrswegebau.
Gražulytė, J., Soenen, H., Blom, J., Vaitkus, A., Židanavičiūtė, J., and Margaritis, A. (2019). Analysis of 4-mm DSR Tests: Calibration, Sample Preparation, and Evaluation of Repeatability and Reproducibility. Road Mater. Pavement Des. 22 (3), 557–571. doi:10.1080/14680629.2019.1634636
Grellmann, W., and Seidler, S. (Editors) (2013). Polymer Testing. 2nd Edition (Munich: Hanser Publishers).
Hajj, R., Filonzi, A., Rahman, S., and Bhasin, A. (2019). Considerations for Using the 4 Mm Plate Geometry in the Dynamic Shear Rheometer for Low Temperature Evaluation of Asphalt Binders. Transportation Res. Rec. 2673, 649–659. doi:10.1177/0361198119855332
Isacsson, U., and Zeng, H. (1998). Low-temperature Cracking of Polymer-Modified Asphalt. Mat. Struct. 31 (1), 58–63. doi:10.1007/BF02486415
ISO 178 (2019). Plastics - Determination of Flexural Properties. Geneva: International Organization for Standardization.(
ISO 6721-5 (2019). Plastics - Determination of Dynamic Mechanical Properties - Part 5: Flexural Vibration - Non-resonance Method. Geneva: International Organization for Standardization.
Kim, Y. S., Sigwarth, T., Büchner, J., and Wistuba, M. P. (2021). Accelerated Dynamic Shear Rheometer Fatigue Test for Investigating Asphalt Mastic. Road Mater. Pavement Des. 22, S383–S396. doi:10.1080/14680629.2021.1911832
Laukkanen, O.-V., Soenen, H., Winter, H. H., and Seppälä, J. (2018a). Low-temperature Rheological and Morphological Characterization of SBS Modified Bitumen. Construction Building Mater. 179, 348–359. doi:10.1016/j.conbuildmat.2018.05.160
Laukkanen, O.-V., Winter, H. H., and Seppälä, J. (2018b). Characterization of Physical Aging by Time-Resolved Rheometry: Fundamentals and Application to Bituminous Binders. Rheol Acta 57 (11), 745–756. doi:10.1007/s00397-018-1114-8
Li, J., Zhang, J., Qian, G., Zheng, J., and Zhang, Y. (2019). Three-Dimensional Simulation of Aggregate and Asphalt Mixture Using Parameterized Shape and Size Gradation. J. Mater. Civil Eng. 31 (3), 4019004. doi:10.1061/(ASCE)MT.1943-5533.0002623
Lu, X., and Isacsson, U. (2000). Laboratory Study on the Low Temperature Physical Hardening of Conventional and Polymer Modified Bitumens. Construction Building Mater. 14 (2), 79–88. doi:10.1016/S0950-0618(00)00012-X
Lu, X., Uhlback, P., and Soenen, H. (2017). Investigation of Bitumen Low Temperature Properties Using a Dynamic Shear Rheometer with 4 Mm Parallel Plates. Int. J. Pavement Res. Tech. 10 (1), 15–22. doi:10.1016/j.ijprt.2016.08.010
Mamlouk, M. S., and Zaniewski, J. P. (2006). Materials for Civil and Construction Engineers. 2nd ed. Upper Saddle River, NJ: Pearson Prentice Hall.
Marasteanu, M., Cannone Falchetto, A., Balamurugan, S., and Negulescu, I. I. (2018). Influence of Cooling Medium on Low Temperature Strength of Asphalt Binders. Construction Building Mater. 162 (7), 80–87. doi:10.1016/j.conbuildmat.2017.12.007
Moon, K. H., Cannone Falchetto, A., Wang, D., Riccardi, C., Kim, J. C., and Wistuba, M. P. (2018). “Effect of Cooling Medium on the Low Temperature Measurements of Asphalt Binder with the Bending Beam Rheometer,” in Proc., 98th Annual Meeting, Washington, D.C, USA, January 11, 2018 (Transportation Research Board).
Oshone, M. (2018). Performance Based Evaluation of Cracking in Asphalt Concrete Using Viscoelastic and Fracture Properties. New Hampshire, Durham: Dissertation, University of New Hampshire.
Pszczola, M., Jaczewski, M., Rys, D., Jaskula, P., and Szydlowski, C. (2018). Evaluation of Asphalt Mixture Low-Temperature Performance in Bending Beam Creep Test. Materials 11, 100. doi:10.3390/ma11010100
Radenberg, M., and Gehrke, M. (2016). “Assessing Bitumen in the Whole Service-Temperature-Range with the Dynamic Shear Rheometer,” in Proc., 6th Eurasphalt & Eurobitume Congress, EAPA & Eurobitume, Prague, Czech Republic, June 1-3, 2016. doi:10.14311/ee.2016.259
Radenberg, M., and Staschkiewicz, M. (2021). “Development of a Test Method to Determine the Low Temperature Performance of Bitumen,” in 7th E&E Congress Proceedings, Spain, June 16-18, 2021.
Riccardi, C., Cannone Falchetto, A., Wang, D., and Wistuba, M. P. (2017). Effect of Cooling Medium on Low-Temperature Properties of Asphalt Binder. Road Mater. Pavement Des. 18, 234–255. doi:10.1080/14680629.2017.1389072
Santagata, E., Baglieri, O., Dalmazzo, D., and Tsantilis, L. (2016). “Experimental Investigation on the Combined Effects of Physical Hardening and Chemical Ageing on Low Temperature Properties of Bituminous Binders,” in 8th RILEM International Symposium on Testing and Characterization of Sustainable and Innovative Bituminous Materials, Ancona, Italy, October 7-9, 2015. Editors F. Canestrari, and M. N. Partl (Springer), 631–641. doi:10.1007/978-94-017-7342-3_5111
Santosh, K., and Kim, Y.-R. (2019). Investigation of DSR Test Methods to Determine Binder Low Temperature Properties, Final Report 26-1121-4041-001. Lincoln, Nebraska: Nebraska Department of Transportation.
Sui, C., Farrar, M. J., Harnsberger, P. M., Tuminello, W. H., and Turner, T. F. (2011). New Low-Temperature Performance-Grading Method. Transportation Res. Rec. 2207, 43–48. doi:10.3141/2207-06
Sun, Z., Li, S., Zhang, J., and Zeng, Y. (2020). Adhesion Property of Bituminous Crack Sealants to Different Asphalt Mixtures Based on Surface Energy Theory. Construction Building Mater. 261, 120006. doi:10.1016/j.conbuildmat.2020.120006
Wang, D., Cannone Falchetto, A., Riccardi, C., Westerhoff, J., and Wistuba, M. P. (2019b). Investigation on the Effect of Physical Hardening and Aging Temperature on Low-Temperature Rheological Properties of Asphalt Binder. Road Mater. Pavement DesignTaylor Francis 22 (5), 1117–1139. doi:10.1080/14680629.2019.1665089
Wang, D., Falchetto, A. C., Riccardi, C., Poulikakos, L., Hofko, B., Porot, L., et al. (2019a). Investigation on the Combined Effect of Aging Temperatures and Cooling Medium on Rheological Properties of Asphalt Binder Based on DSR and BBR. Road Mater. Pavement Des. 20, S409–S433. doi:10.1080/14680629.2019.1589559
Wei, H., Li, J., Wang, F., Zheng, J., Tao, Y., and Zhang, Y. (Forthcoming 2021). Numerical Investigation on Fracture Evolution of Asphalt Mixture Compared with Acoustic Emission. Int. J. Pavement Eng., 1–11. doi:10.1080/10298436.2021.1902524
Wilhelm, E. (2010). “Heat Capacities: Introduction, Concepts and Selected Applications,” in Heat Capacities: Liquids, Solutions and Vapours. Editors E Wilhelm, and T. M Letcher (London, UK: The Royal Society of Chemistry), 1–26.
Wistuba, M. P., Büchner, J., Hilmer, T., Steineder, M., Eberhardsteiner, L., Donev, V., et al. (2019). Vereinfachung der prüftechnischen Ansprache des Gebrauchsverhaltens von Asphalt (VEGAS) - Ergebnisbericht zum Werkvertrag, FFG Projektnummer: 863063, FFG-Programm: D-A-CH Kooperation Verkehrsinfrastrukturforschung. Braunschweig: Technische Universität Braunschweig, Institut für Straßenwesen (Projektleitung), Kooperation mit der Technischen Universität Wien und der Eidgenössischen Materialprüfanstalt.
Wistuba, M. P., Monismith, C. L., Bahia, H. U., Renken, P., Olard, F., Blab, R., et al. (2009). Asphaltverhalten bei tiefen Temperaturen / Asphalt material behaviour at low temperatures, Festschrift zu Ehren des 80. Geburtstags von Professor Wolfgang Arand / Essays in honour of Professor Wolfgang Arand 80th birthday, 23. Braunschweig: Technische Universität Braunschweig, Institut für Straßenwesen, Schriftenreihe Straßenwesen, Heft.
Wistuba, M. P. (2019). Straßenbaustoff Asphalt. Erste Auflage, Technische Universität Braunschweig. Braunschweig: Institut für Straßenwesen.
Keywords: low temperature, asphalt binder testing, three-point bending beam test, dynamic shear rheometer, bending beam rheometer
Citation: Kim YS, Büchner J, Wistuba MP, Agudo JR, Rochlani M and Schäffler M (2022) Asphalt Binder Testing at Low Temperature: Three-Point Bending Beam Test in Dynamic Shear Rheometer. Front. Mater. 9:831443. doi: 10.3389/fmats.2022.831443
Received: 08 December 2021; Accepted: 26 January 2022;
Published: 17 February 2022.
Edited by:
Hui Yao, Beijing University of Technology, ChinaReviewed by:
Zhaoxing Xie, Louisiana State University, United StatesJunhui Zhang, Changsha University of Science and Technology, China
Copyright © 2022 Kim, Büchner, Wistuba, Agudo, Rochlani and Schäffler. This is an open-access article distributed under the terms of the Creative Commons Attribution License (CC BY). The use, distribution or reproduction in other forums is permitted, provided the original author(s) and the copyright owner(s) are credited and that the original publication in this journal is cited, in accordance with accepted academic practice. No use, distribution or reproduction is permitted which does not comply with these terms.
*Correspondence: Johannes Büchner, j.buechner@tu-bs.de