- 1School of Materials Science and Engineering, Wuhan Institute of Technology, Wuhan, China
- 2Hubei Key Laboratory of Plasma Chemistry and Advanced Materials, Wuhan Institute of Technology, Wuhan, China
- 3School of Chemistry and Chemical Engineering, Ankang University, Ankang, China
- 4Department of Food Science and Chemical Engineering, Hubei University of Arts and Science, Xiangyang, China
In this study, using chitosan (CS) and carboxymethyl cellulose (CMC) as backbone and introducing citric acid (CA)to enhance the electrostatic interaction of the system, citric acid/chitosan/carboxymethyl cellulose (CA/CS/CMC) aerogel is obtained by simple freeze-drying. CA/CS/CMC composite aerogel exhibits light weight, low density, high porosity, outstanding hydrophilic and water retention properties, and satisfactory underwater oleophobicity. The water adsorption capacity of the obtained aerogels can reach 43.87–80.28 g/g, which are far more than that of carboxymethyl cellulose and chitosan aerogels (14.27–20.08 g/g). In addition, with strong hydrophilicity, underwater oleophobicity and water retention endowed by the rough internal microstructure and the rich hydroxyl, amino, and carboxyl groups, the fabricated aerogel can also be used as a filter to achieve effective separation of oil-in-water emulsions and oil/water mixtures. The separation efficiency of aerogel for oil/water mixtures are higher than 90.7%. Because the developed preparation method is green, simple and mild and the raw materials are readily available and environmentally friendly, the obtained CA/CS/CMC aerogel with strong water absorption capacity and good separation efficiency displays a promising application in water-oil separation.
1 Introduction
The constant happening of crude oil leakage incidents, coupled with the massive discharge of oily wastewater in people’s daily life and industry, will cause huge pollution to the environment and seriously threaten human health and economic development (Li et al., 2018; Zhong et al., 2021; Chen et al., 2014; Franco et al., 2014). The application of filter paper (Li et al., 2019; Cheng et al., 2018), membrane (Zhong et al., 2021; Wu et al., 2022) and aerogel (Korhonen et al., 2011; Laitinen et al., 2017) in water-oil separation has also been reported. Currently, one of most effective methods is separating oil and water using physical adsorption of porous materials, which is also inexpensive, environmentally friendly and easy-operated (Korhonen et al., 2011; Laitinen et al., 2017; Saleem et al., 2018). In the early 1930s, a new type of material, aerogel, was proposed by Kistler (Kistler, 1931) Aerogel is obtained by replacing liquid medium of gel with gaseous medium with no significant changing its internal structure. Aerogel has many excellent characteristics, like light weight, abundant pores, and extremely strong adsorption. At present, it has been widely applicated in aerospace (Jones, 2006), thermal insulation (Shi et al., 2013; Takeshita and Yoda, 2015), adsorption materials (Huang and Shi, 2014), drug loading (Işıklan et al., 2011) and other fields. Aerogel is also ideal material for liquid absorption and storage due to their porous nature. The research directions of the aerogels for water-oil separation are divided mainly into two types: one is to improve hydrophobicity and oil-absorbing properties of aerogels by impregnation, solvent exchange, atomic layer deposition, gelation (Yang et al., 2020), and then the good water-oil separation effect will be produced (Han et al., 2016; Wan et al., 2015; Cao et al., 2015; Akhlamadi and Goharshadi, 2021; Wang and Liu, 2019). However, due to the lipophilic nature of this aerogel, oil can easily stick to the aerogel or even get blocked by the oil, resulting in contamination of the material. In addition, the adsorbed oil cannot be cleaned away, which will increase the difficulty of oil recovery and the risk of secondary pollution in post-processing. High density of water also can form a water barrier that hinders the gravity-driven separation process (Zhang et al., 2013). The other direction is to use the hydrophilicity of aerogels to realize water-oil separation and no hydrophobic modification is performed. For instance, Sun et al. found that superabsorbent fibers can absorb 10–50 times their own weight of ordinary tap water (Sun, 2008). This kind of annular filter made of fibers can allow the passage of water and block oil particles with smaller diameters, so as to achieve the good water-oil separation. This material with superhydrophilic and underwater superoleophobic characteristics is able to solve the problem of low separation efficiency caused by water barrier and the problem of pores blocking of the hydrophobic materials (Wang et al., 2020). Thus, this material with hydrophilic/oleophobic properties provides a viable alternative for water-oil separation (Zhang et al., 2020).
Nowadays, there are numerous studies on the preparation of hydrophilic/oleophobic materials by constructing nano/micro-scale structures and introducing appropriate chemicals. However, most reported materials have intolerant surfaces and poor water retention ability (Yuan et al., 2017; Ge et al., 2018; Xu et al., 2018; Koh et al., 2019; Zhang et al., 2020), which is unfavorable for stable separation properties. Specially, the multi-scale topography is customarily in need of complex manufacturing course, which sets a limit for practicability of this materials. Hydrophilic aerogels can be obtained from hydrogels by appropriate drying methods. For instance, Liu et al. synthesized chitosan/lithium sulfonate hydrogel by electron beam irradiation, and obtained an aerogel with porous structure and CO2 capture ability by freeze-drying (Liu et al., 2021); He et al. prepared a multifunctional polyimide (PI) aerogel adsorbent from poly(amic acid) ammonium salt (PAS) hydrogel by freeze-drying (He et al., 2017). Carboxymethyl cellulose (CMC) is rich in hydrophilic groups like carboxyl and hydroxyl groups (Kono et al., 2016; Xiang et al., 2018), and it is an anionic polysaccharide. The molecular chain of chitosan (CS) also has many hydroxyl and amino groups (Guibal et al., 2014; Rajoka et al., 2019; Cheung et al., 2015), and it is a natural biodegradable cationic polymer. Due to the existence of abundant polar hydrophilic groups, CMC and CS are suitable for developing composite hydrogels based on natural biopolymers. The two materials have the advantages of non-polluting, abundant sources, low cost, biodegradability and biocompatibility (Uyanga et al., 2020).
In this study, composite aerogels were synthesized by using CS and CMC as the double-polymer aerogel framework and introducing CA to enhance the electrostatic interaction of the system. The detailed goals of this study are to (a) synthesize environmentally sustainable ion cross-linked CA/CS/CMC composite aerogel with good underwater oleophobicity and strong water absorption and evaluate the effect of changing the content of two raw materials on the performances of the aerogel; (b) evaluate the oil/water separation property of fabricated aerogel. The studies show CA/CS/CMC composite aerogel with strong water absorption-retention properties and good oil/water separation effect has promising applications in oil-polluted water and oil-in-water emulsions.
2 Experimental
2.1 Materials
Carboxymethyl cellulose III (CMC) (M.W. 250000, DS = 0.9, 1,500–3,100 mPa s) was obtained from Shanghai Macklin Biochemical Co., Ltd. Chitosan (CS) (BR grade) was acquired from Shanghai Sinopharm Chemical Reagent Co., Ltd. The rest of the chemical reagents were acquired from Shanghai Sinopharm Chemical Reagent Co., Ltd. and all are AR grade. All chemicals were used as received without further purification.
2.2 Preparation of CA/CS/CMC composite aerogels
An appropriate amount of CS powder was added to 1% acetic acid solution (v/v) and stirred at room temperature to obtain 0.5wt%, 1wt%, 1.5wt%, 2wt%, 2.5wt%, 3wt%, 3.5wt% and 4wt% the CS solution. An appropriate amount of CMC powder was added to deionized water and stirred at 80°C to obtain 0.5wt%, 1wt%, 1.5wt%, 2wt%, 2.5wt%, 3wt%, 3.5wt% and 4wt% CMC solutions. In room temperature, after mixing the same volume of CS solution and CMC solution evenly, added CA (15% of CS mass), stirred until completely dissolved, and maintained at -18°C for at least 6 h. CA/CS/CMC composite aerogels were obtained by freeze-drying for 48 h. CA/CS/CMC composite aerogels’ names are determined by the mass fraction of raw material solution. For instance, the raw material solution used to synthesize S1M1A aerogel is 1 wt% CS and 1 wt% CMC. (Supplementary Tables S1, S2).
2.3 Characterizations
The sample was thoroughly dried, mixed with potassium bromide (KBr) powder and ground into powder, and Fourier Transform infrared spectrum was tested (Nicolet 6,700, American Thermo Electron Co., Ltd.). The internal microstructure of composite aerogels was detected by scanning electron microscope (SEM) with energy dispersive spectroscopy (EDS) (JSM-5510LV, JEOL Ltd.). Liquid contact angle (CA) was determined by means of CA measuring device (DSA100, Germany Kruss Ltd.). The water droplet and chloroform droplet for CA measurements were 5 μL and 20 μL, respectively. The compressive strength of the aerogel was measured by an electronic universal testing machine (CMT, MTS System Ltd.).
2.4 Apparent densities and porosities
The diameter (D) and height (H) of the sample was measured with a vernier caliper, the mass (m) of the sample was tested with an electronic balance, and the apparent density (ρb) of the aerogel is determined by Eq. 1. The automatic true density analyzer (3H-2000TDI, Beijing Beishide Instrument Technology Co., Ltd.) was applied to measure the true density (ρs) of the sample, and the aerogel porosity (P) can be measured by using Eq. 2.
2.5 Adsorption properties and oil−water separation experiment
The sample (m0) was put into a beaker containing deionized water or an organic solvent. After a certain interval, the sample was taken out and drained for 2s, the mass (m) was measured.
The adsorption rate (C) of deionized water or organic solvent by aerogel was calculated by Eq. 3.
The oil was poured into deionized water (20% v/v) and stirred (600 rpm/min) at least 15 h to get the oil-in-water emulsion. Oil-water mixture (20% v/v) was prepared by simply mixing deionized water with oil. Optical microscope (OM) (BK6000, Chongqing Auto Optical Instrument Co., Ltd.) was used to observe oil-in-water emulsions, oil/water mixtures, and filtrates.
After sample was put into the funnel and saturated with deionized water, a certain volume (V0) of oil/water mixture and (oil density< 1) was poured into the funnel and the oil will be blocked on the aerogel. The volume (V) of the remaining oil was measured after the water is completely filtered. Each sample should be measured at least 3 times and averaged. The water-oil separation efficiency (R) of aerogel was calculated by Eq. 4.
3 Results and discussion
3.1 Preparation and wettability
Ion-crosslinking CA/CS/CMC composite aerogel was prepared using CMC, CS with high hydroscopicity and moisture retention as the matrix and introducing CA. Figure 1A shows illustration for the manufacturing procedure of CA/CS/CMC composite aerogel. To study the wettability of CA/CS/CMC composite aerogel, the contact angle was measured. Figures 1B,C show the water wetting behavior in air and oil wetting behavior underwater of CA/CS/CMC composite aerogels. In air, when the water droplet drops on aerogel’s surface, the strong hydrophilicity makes the water droplet be completely absorbed by CA/CS/CMC composite aerogel within 2.272s, and the water CA is 0°. This is ascribed to the internal microstructure and the eminently abundant hydroxyl, amino, and carboxyl groups contained (Wang et al., 2020). When chloroform droplet was dropped on the aerogel’s surface under water, it presents the underwater CA of 137.7 ± 1.7° on the aerogel surface, which was attributed to the porous structure inside the aerogel and hydrated form of polar groups (Zhang et al., 2020). The water wetting behavior in aerogel pores and oil-rejection behavior was displayed. When the surrounding atmosphere is air, the hydrophilicity of the CA/CS/CMC composite aerogel coupled with the porous nature allows water to continuously penetrate the aerogel (Figure 1D). Before oil-water separation, CA/CS/CMC composite aerogel was completely wetted by water, causing its rough structure to be flooded by a major expenditure of water, and the repulsion between water and oil would prevent the aerogel from being contaminated by oil (Figure 1E) (Wang et al., 2020).
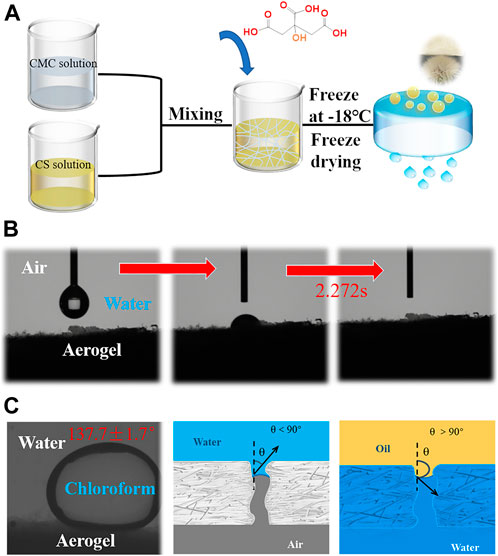
FIGURE 1. (A) The illustration for the manufacture procedure of CA/CS/CMC composite aerogel, (B) water droplet on aerogel surface in air, (C) chloroform droplet on aerogel surface underwater, (D) and (E) wetting behavior and process of water in the pores of aerogel.
3.2 Morphology, structure, apparent density, and porosity
As can be seen from SEM images of CA/CS/CMC composite aerogels developed at different mass concentration (Figures 2A–H), it is clear that the CA/CS/CMC composite aerogel framework is mainly comprised of stacking of secondary sheet-like layers. And through the EDS point scanning spectrums of aerogel, it can be seen that there are C, N and O elements on the surface of aerogel with different mass concentration (Supplementary Figure S1). As the increase of CS/CMC total mass from 0.5 wt% to 4 wt%, the lamellae become more regular, which also makes the maximum compressive strength of aerogels increase gradually at 60% strain (Supplementary Figure S2). There are a large number of non-uniform pores among the lamellae, which is as a result of the construction of multiple ice crystals when the solvent is frozen below the freezing point at low temperature. The dispersibility of CS and CMC in water is different (Ma et al., 2021), which affects the growth of ice crystals. After the ice crystals are sublimated into gas under vacuum conditions, uneven pores will be formed. The large number of folds not only increases the surface roughness of the composite aerogel, but also makes the aerogel ultralight (Xu et al., 2020). Such rough surface and CS/CMC has an excellent affinity for water, which enable the aerogel to have excellent hydrophilicity and underwater oleophobicity (Padaki et al., 2015; Yue et al., 2019; Wang et al., 2020).
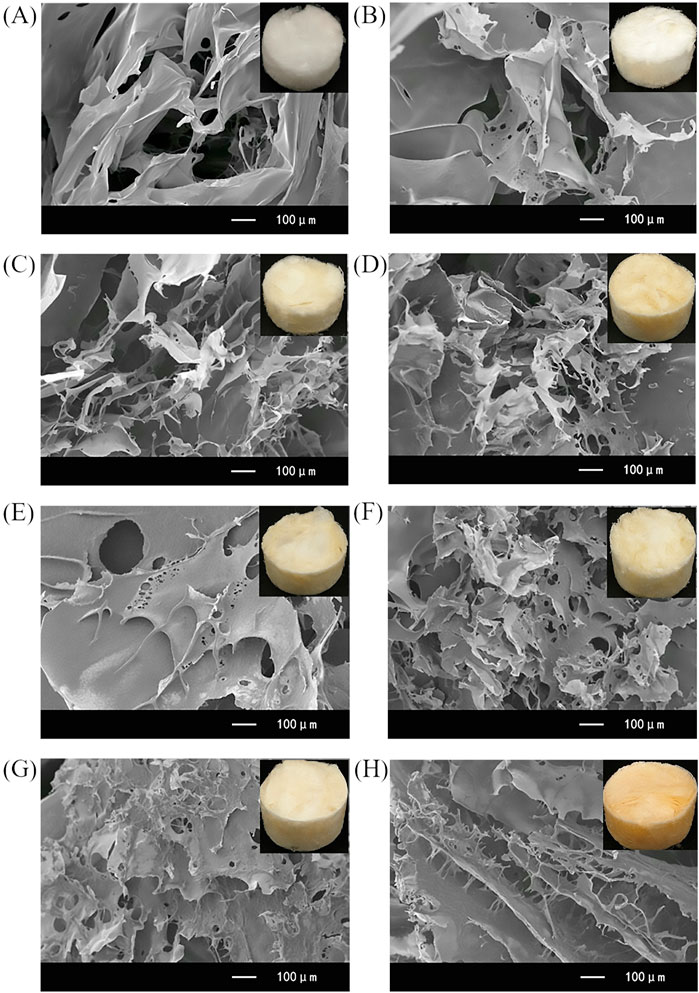
FIGURE 2. SEM images of the S0.5M0.5A (A), S1M1A (B), S1.5M1.5A (C), S2M2A (D), S2.5M2.5A (E), S3M3A (F), S3.5M3.5A (G), and S4M4A (H) composite aerogels.
Figure 3A displays the FT-IR spectra of CMC, CS and S1M1A.It can be seen from the figure that the characteristic absorption peaks of both CS and CMC are displayed in the infrared image of the composite aerogel, and the peak intensity of S1M1A is obviously stronger than that of the two raw materials. All three samples have broad absorption peaks at 3,700 cm−1∼3,000 cm−1. The peak of CMC is attributed to the O-H stretching vibration, while CS and composite aerogels show this peak in addition to the effect of the above bond, as well as the role of N-H stretching vibration. Although it is superimposed by the broad absorption peak at 1581cm−1, it can still be seen that a new peak belonging to NH3+ appears at 1633cm−1(Yong et al., 2002; De Vasconcelos et al., 2006; Ostrowska-Czubenko et al., 2009). The peaks at 1581cm−1 and 1405cm−1 belong to the symmetrical and antisymmetric stretching vibrations of [O-C=O]―. The strong infrared absorption peaks at these two places prove that S1M1A is rich in carboxyl groups (Yong et al., 2002; De Vasconcelos et al., 2006). These results suggest that CMC, CS and CA are bound together by electrostatic interactions. Overall, a plentiful of hydrophilic functional groups including hydroxyl, amino, and carboxyl groups exist in S1M1A, which will contribute to the efficient adsorption of water molecules. The FTIR images of composite aerogels obtained at different raw material ratios (Figure 3B) shows that the absorption peak of S1M1A is significantly stronger than that of other aerogels, which was more conducive to water absorption.
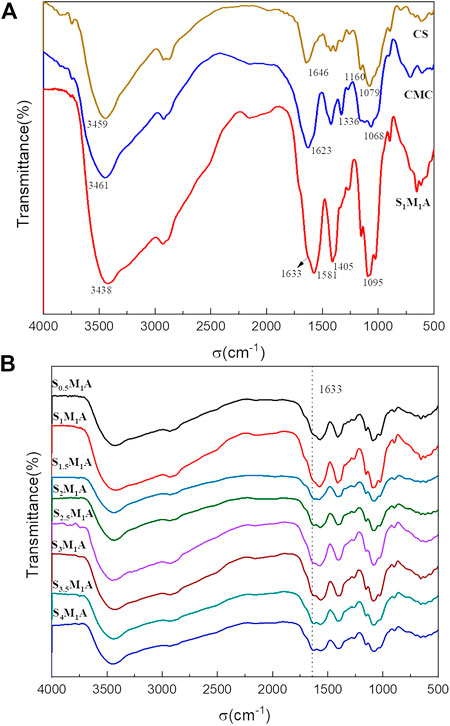
FIGURE 3. FT-IR spectra of (A) S1M1A, CMC, CS; and (B). aerogels prepared at different CS/CMC ratio.
Light weight and porous structure are essential characteristics of aerogels (Padaki et al., 2015; Yue et al., 2019). The apparent density and porosity of the material can directly reflect these two characteristics. As can be seen from the variation trends of the apparent density and porosity of the aerogel with different mass concentration, the apparent density of the prepared aerogels are within the scope of 0.0083–0.0636 g/cm3 and the porosity is above 96% (Figure 4). The two indicators prove that the fabricated aerogels are low-density porous material. As the increase of CS and CMS content in the system, the apparent density of the aerogel shows increasing trend. The results can be given explanatory notes by the fact that, at a same volume, the distance between polymer molecules decreases with the increase in the overall content of the feedstock, resulting in a decrease in porosity. Although the porosity of the aerogel decreases with the increase in the overall content of the feedstock, the lowest porosity still reaches above 96%. The porosity of aerogels is crucial for separation performance without any external force. The high porosity ensures that the gravity-driven water penetration can be achieved by the aerogel, and the separation process is fast and efficient (Wang et al., 2020).
3.3 Adsorption capacity and solvent retention performance
In the kinetics curves of water adsorption (Figures 5A,B), the water adsorption capacity of CA/CS/CMC aerogel reach about 18.17–64.64 g/g within 1 s, showing strong hydrophilicity. The adsorption reaches saturation in 30 s. This suggests that the aerogel has a fast water absorption ability due to the high porosity and the abundant hydrophilic groups (Jia et al., 2018). In general, the water absorption rates of all different formulations of aerogels increase significantly at first and then decrease with prolonging time. Because polymer molecules were not fully stretched in water at the beginning. With the increase of time, water molecules fill the aerogel pores due to existence of capillary force among pores and abundant hydrophilic group in aerogel, and the water adsorption will finally reach saturation. Figure 5C displays the sorption kinetics of S1M1A for the organic solvents. The test results show that S1M1A can reach adsorption saturation within 1 s for five organic solvents, including ethanol, methanol, acetone, toluene, and chloroform. The water absorption capacity of aerogels with different formulations is shown in Figure 6A, B. It can be seen that the water absorption capacity of CA/CS/CMC composite aerogels firstly increases and then decreases. S1M1A has a maximum absorption capacity of 80.67 g/g. This is mainly because the hydrophilic groups (hydroxyl, amino, and carboxyl groups) in S1M1A are exceedingly abundant. Increasing the total mass of CS and CMC will cause the decrease of the distance among polymer molecules and the porosity, resulting in the decrease of water absorption capacity (Yuan et al., 2016). Figure 6C shows that the adsorption capacities of S1M1A for different organic solvents are about 27–44 g/g. For methanol, acetone and toluene, the adsorption capacities are all around 27.15 g/g; for chloroform, the adsorption capacity is 44.81 g/g. The highest adsorption capacity for chloroform is due to the large density. As can be seen from the comparison of the as-prepared aerogels and several reported water absorbents, the water absorption capacity of CA/CS/CMC aerogel is far higher than that of other absorption materials (Table 1). Figure 7A shows the retention properties of S1M1A for water, ethanol, methanol, acetone, toluene, and chloroform. The aerogels saturated with organic solvents have obvious quality changes after 16 min. For chloroform, the obvious quality change can be found after 1 min. The quality of aerogels saturated with water deceases after 120 min and decreases sharply after 180 min. Moreover, the organic solvent in the aerogel volatilized completely in 32–120 min, but the volatilization of water needs to spend 2,880 min. This is because the prepared aerogels have abundant hydrophilic groups, which results in better water retention than organic solvents retention. Figure 7B is the picture of the aerogel before and after water absorption, which shows that the aerogel is hydrogel-like after absorbing water. The water-absorbing aerogel still can float on the water surface after reaching adsorption saturation (Figure 7C), which is because of the low density and high porosity of the aerogel.
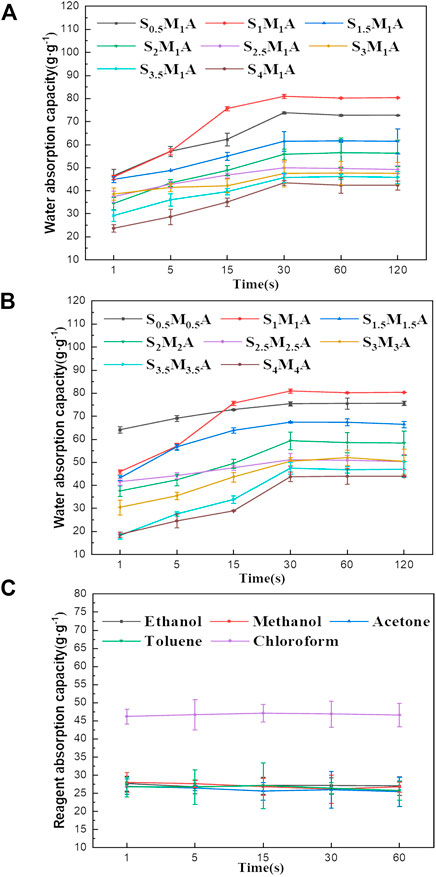
FIGURE 5. (A) Water sorption kinetics of composite aerogels prepared at 1 wt% CMC solution and different CS solutions, (B) water sorption kinetics of composite aerogels prepared at different mass concentration, (C) the sorption kinetics of S1M1A for the five types of organic solvents.
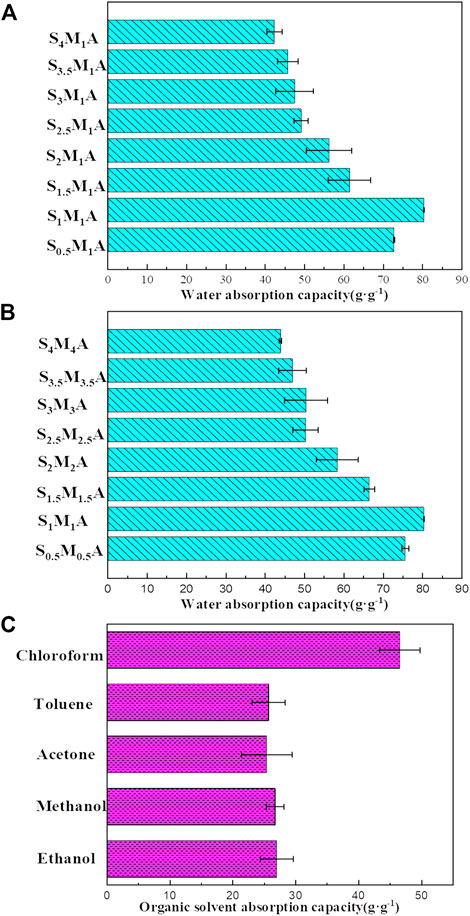
FIGURE 6. (A) Water absorption capacity of composite aerogels at 1 wt% CMC solution and different CS solutions, (B) water absorption capacity of composite aerogels prepared at different mass concentration, (C) the sorption capacity of S1M1A for five types of organic solvents.
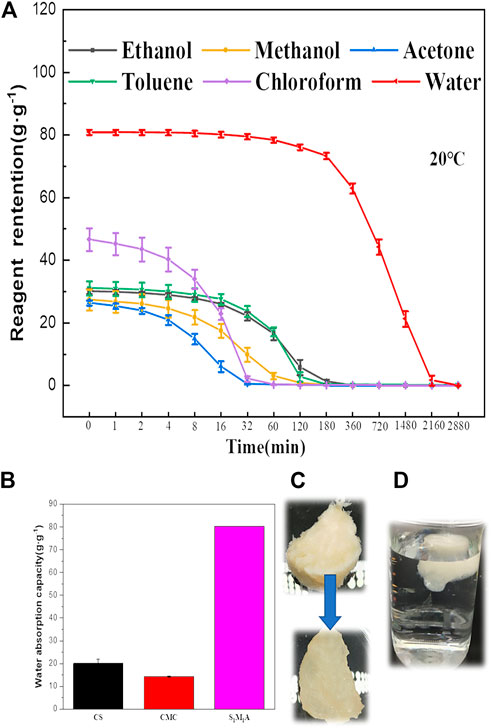
FIGURE 7. (A) Solvents and water retention performance of S1M1A, (B) water absorption capacity of raw material and S1M1A aerogels, (C) the aerogel before and after absorbing water, (D) the floating aerogel after water absorption.
3.4 Oil/water separation ability
For purpose of evaluating the separation ability of CA/CS/CMC aerogels to water on oil, the aerogel fully absorbed water and reached saturation before separation. Small amounts of methylene blue-stained water, coffee, milk, and tea on chloroform surface were fully absorbed by the water-wetted composite aerogel within several seconds, and no obvious residual liquid was found on the chloroform in the beaker (Figure 8). This indicates that CA/CS/CMC composite aerogel has remarkable separation ability for water-oil mixtures (Wang et al., 2015).
The oil/water separation process is shown in Figure 9A. When oil gets in touch with the water-saturated aerogel, an oil/water/solid three-phase interface will be generated. Formation of the hydrated shell, coupled with water-oil incompatibility, can tremendously diminish the range of oil in direct contact with solid surfaces. Thus, water flows out from the lower end through the aerogel under the influence of gravity and oil is blocked in the upper layer, causing the good separation effect (Zhang et al., 2020). Besides the excellent water adsorption properties, the S1M1A aerogel also shows great potential in separating oil-in-water emulsion and oil/water mixture. The S1M1A immobilized in funnel was used as the filter to evaluate separation performance. Rapeseed oil-in-water emulsion was prepared for separation experiment (Figure 9B). After the emulsion was poured into the funnel for a while, the water will flow out of the lower end of the funnel through the aerogel under the gravity. Because of the microporous structure and underwater oleophobicity (Zhang et al., 2020), the oil droplets in the upper layer cannot penetrate the aerogel saturated with water. The rapeseed oil-in-water emulsion was pale yellow and the oil droplets with uneven particle size were detected in the emulsion (Figure 9C). The filtrate after the treating is colorless and the number of oil droplets is greatly reduced in optical image (Figure 9D). It shows that S1M1A aerogel has good oil-in-water emulsion separation performance. The separation of oil/water mixture was carried out using corn oil-in-water mixture as a sample (Figure 9E). After the aerogel is saturated with water, the hydrated shell in aerogel will be formed as a result of the forceful hydrogen bonds between water molecules with hydroxyl amino and carboxyl groups, which can weaken the van der Waals interaction between CA/CS/CMC composite aerogel with oil (Koh et al., 2019). In Figure 9F, the corn oil/water mixture was cloudy and micron-sized oil droplets were detected in the mixture. Filtrate after the treatment is colorless, and there were no oil droplets in optical image (Figure 9G). The separation efficiency of water-saturated aerogels for rapeseed oil, corn oil, liquid paraffin, olive oil, motor oil and kerosene in water was over 90.7% (Figure 9H). For corn oil and liquid paraffin, the separation efficiency can reach about 96%.
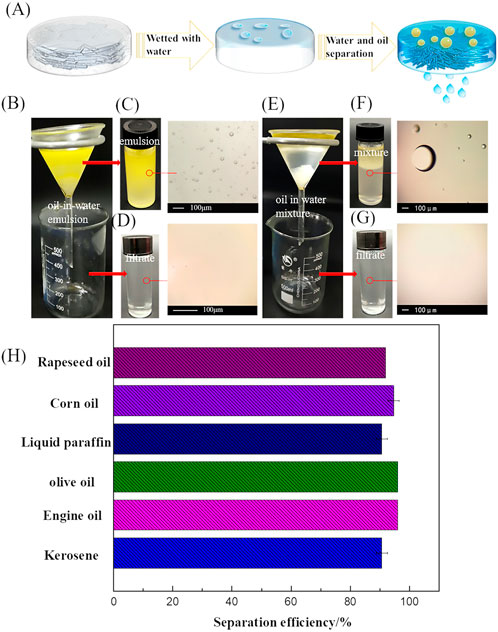
FIGURE 9. (A) Schematic diagrams of the oil/water separation mechanism of CA/CS/CMC composite aerogels, (B) Separation for oil-in-water emulsion, the photographs and OM images of (C) rapeseed oil-in-water emulsion and (D) the filtrate, (E) separation for oil/water mixture, the photographs and OM images of (F) corn/water oil mixture and (G) the filtrate, (H) separation efficiency of S1M1A for different oil/water mixtures.
4 Conclusion
In conclusion, a novel CA/CS/CMC composite aerogel with light weight, low density, high porosity, good hydrophilic and oleophobicity, and strong water retention was synthesized through simple and green strategy. The aerogel can absorb water up to absorption capacity of 43.87–80.28 g/g. Compared with CMC and CS aerogels, the fabricated aerogel exhibits the highest water absorption capacity. As a result of strong hydrophilicity, underwater oleophobicity and water retention caused by the rough internal microstructure and the polar groups (hydroxyl, amino, and carboxyl groups), the aerogel can be used as a filter to effectively separate oil-in-water emulsions and oil/water mixtures. The raw materials for CA/CS/CMC composite aerogel preparation are green and easy to be obtained and the preparation conditions are simple and mild, which greatly reduces the risk of secondary pollution. It is believed that the prepared aerogel displays good application prospects water treatments Li et al., 2019.
Data availability statement
The raw data supporting the conclusions of this article will be made available by the authors, without undue reservation.
Author contributions
HL: Writing—original draft, Methodology. XJ: Validation, Investigation, Visualization, Project administration. JW: Conceptualization, Supervision, Writing—review and editing. RH: Investigation, review and editing.
Funding
This research was supported by the National Natural Science Foundation of China (No. 51273154) and Graduate Innovative Fund of Wuhan Institute of Technology (No. CX2021208).
Conflict of interest
The authors declare that the research was conducted in the absence of any commercial or financial relationships that could be construed as a potential conflict of interest.
Publisher’s note
All claims expressed in this article are solely those of the authors and do not necessarily represent those of their affiliated organizations, or those of the publisher, the editors and the reviewers. Any product that may be evaluated in this article, or claim that may be made by its manufacturer, is not guaranteed or endorsed by the publisher.
Supplementary material
The Supplementary Material for this article can be found online at: https://www.frontiersin.org/articles/10.3389/fmats.2022.1093164/full#supplementary-material
References
Akhlamadi, G., and Goharshadi, E. K. (2021). Sustainable and superhydrophobic cellulose nanocrystalbased aerogel derived from waste tissue paper as a sorbent for efficient oil/water separation. Process Saf. Environ. Prot. 154, 155–167.doi:10.1016/j.psep.2021.08.009
Alhwaige, A. A., Ishida, H., and Qutubuddin, S. (2020). Chitosan/polybenzoxazi-ne/clay mixed matrix composite aerogels: Preparation, physical properties, and water absorbency. Appl. Clay Sci. 184, 105403.doi:10.1016/j.clay.2019.105403
Cao, X. F., Peng, X. W., Sun, S. N., Zhong, L. X., Chen, W., Wang, S., et al. (2015). Hydrothermal conversion of xylose, glucose, and cellulose under the catalysis of transition metal sulfates. Carbohydr. Polym. 118, 44–51.doi:10.1016/j.carbpol.2014.10.069
Chen, Y., Bai, Y., Chen, S., Ju, J., Li, Y., Wang, T., et al. (2014). Stimuli-Responsive composite particles as solid-stabilizers for effective oil harvesting. ACS Appl. Mat. Interfaces 6 (16), 13334–13338.doi:10.1021/am504124a
Cheng, Q. Y., Guan, C. S., Wang, M., Li, Y. D., and Zeng, J. B. (2018). Cellulose nanocrystal coated cotton fabric with superhydrophobicity for efficient oil/water separation. Carbohydr. Polym. 199, 390–396.doi:10.1016/j.carbpol.2018.07.046
Cheung, R. C. F., Ng, T. B., Wong, J. H., and Chan, W. Y. (2015). Chitosan: An update on potential biomedical and pharmaceutical applications. Mar. Drugs 13 (8), 5156–5186.doi:10.3390/md13085156
De Vasconcelos, C. L., Bezerril, P. M., Dos Santos, D. E. S., Dantas, T. N. C., Pereira, M. R., and Fonseca, J. L. C. (2006). Effect of molecular weight and ionic strength on the formation of polyelectrolyte complexes based on poly(methacrylic acid) and chitosan. Biomacromolecules 7 (4), 1245–1252.doi:10.1021/bm050963w
Franco, C. A., Cortés, F. B., and Nassar, N. N. (2014). Adsorptive removal of oil spill from oil-in-fresh water emulsions by hydrophobic alumina nanoparticles functionalized with petroleum vacuum residue. J. Colloid Interface Sci. 425, 168–177.doi:10.1016/j.jcis.2014.03.051
Ge, J. L., Zong, D. D., Jin, Q., Yu, J. Y., and Ding, B. (2018). Biomimetic and superwettable nanofibrous skins for highly efficient separation of oil-in-water emulsions. Adv. Funct. Mat. 28 (10), 1705051.doi:10.1002/adfm.201705051
Guibal, E., Vincent, T., and Navarro, R. (2014). Metal ion biosorption on chitosan for the synthesis of advanced materials. J. Mat. Sci. 49 (16), 5505–5518.doi:10.1007/s10853-014-8301-5
Han, S. J., Sun, Q. F., Zheng, H. H., Li, J. P., and Jin, C. D. (2016). Green and f-acile fabrication of carbon aerogels from cellulose-based waste newspaper for solving organic pollution. Carbohydr. Polym. 136, 95–100.doi:10.1016/j.carbpol.2015.09.024
He, X. Y., Zhang, L., Meng, D. P., and Wu, J. T. (2017). From hydrogel to aerogel: A green fabrication of multifunctional polyimide absorbents. Eur. Polym. J. 89, 461–467. doi:10.1016/j.eurpolymj.2017.02.039
Huang, S. Y., and Shi, J. F. (2014). Monolithic macroporous carbon materials as high-performance and ultralow-cost sorbents for efficiently solving organic pollution. Ind. Eng. Chem. Res. 53 (12), 4888–4893.doi:10.1021/ie5003558
Işıklan, N., İnal, M., Kurşun, F., and Ercan, G. (2011). pH responsive itaconic acid grafted alginate microspheres for the controlled release of nifedipine. Carbohydr. Polym. 84 (3), 933–943.doi:10.1016/j.carbpol.2010.12.054
Jia, J. R., and Wang, C. X. (2018). A facile restructuring of 3D high water absorption aerogels from methoxy polyethylene glycol-polycaprolactone (mPEG-PCL) nanofibers. Mater. Sci. Eng. C 94, 965–975. doi:10.1016/j.msec.2018.10.044
Jones, S. M. (2006). Aerogel: Space exploration applications. J. Solgel. Sci. Technol. 40, 351–357.doi:10.1007/s10971-006-7762-7
Koh, J. J., Lim, G. J. H., Zhou, X., Zhang, X. W., Ding, J., and He, C. B. (2019). Correction to “3d-printed anti-fouling cellulose mesh for highly efficient oil/water separation applications”. ACS Appl. Mat. Interfaces 11 (38), 35510.doi:10.1021/acsami.9b15215
Kono, H., Oshima, K., Hashimoto, H., Shimizu, Y., and Tajima, K. (2016). NMR characterization of sodium carboxymethyl cellulose: Substituent distribution and mole fraction of monomers in the polymer chains. Carbohydr. Polym. 146, 1–9.doi:10.1016/j.carbpol.2016.03.021
Korhonen, J. T., Kettunen, M., Ras, R. H. A., and Ikkala, O. (2011). Hydrophobic nanocellulose aerogels as floating, sustainable, reusable, and recyclable oil absorbents. ACS Appl. Mat. Interfaces 3 (6), 1813–1816.doi:10.1021/am200475b
Laitinen, O., Suopajarvi, T., Osterberg, M., and Liimatainen, H. (2017). Hydrophobic, superabsorbing aerogels from choline chloride-based deep eutectic solvent pretreated and silylated cellulose nanofibrils for selective oil removal. ACS Appl. Mat. Interfaces 9 (29), 25029–25037.doi:10.1021/acsami.7b06304
Li, F. R., Wang, Z. R., Huang, S. C., Pan, Y. L., and Zhao, X. Z. (2018). Flexible, durable, and unconditioned superoleophobic/superhydrophilic surfaces for controllable transport and oil-water separation. Adv. Funct. Mat. 28 (20), 1706867. doi:10.1002/adfm.201706867
Li, S., Yang, S., Zhu, X., Jiang, X., and Kong, X. Z. (2019). Easy preparation of superoleophobic membranes based on cellulose filter paper and their use for water–oil separation. Cellulose 26, 6813–6823. doi:10.1007/s10570-019-02552-4
Liu, Z. Y., Ma, R., Du, W. J., Yang, G., and Chen, T. (2021). Radiation-initiated high strength chitosan/lithium sulfonate double network hydrogel/aerogel with porosity and stability for efficient CO2 capture. RSC Adv. 11 (33), 20486–20497.doi:10.1039/d1ra03041h
Ma, C. Q., Shi, W. Z., Cui, S. S., Zhang, M. Y., and Zhou, H. (2021). Research progress in polyvinyl plcohol⁃based porous pomposities. China Plast. 35 (04), 116–123. [in Chinese].doi:10.19491/j.issn.1001-9278.2021.04.019
Mallepally, R. R., Bernard, I., Marin, M. A., Ward, K. R., and McHugh, M. A., and (2013). Superabsorbent alginate aerogels. J. Supercrit. Fluids 79, 202–208. doi:10.1016/j.supflu.2012.11.024
Ostrowska-Czubenko, J., and Gierszewska-Drużyńska, M. (2009). Effect of ionic crosslinking on the water state in hydrogel chitosan membranes. Carbohydr. Polym. 77 (3), 590–598.doi:10.1016/j.carbpol.2009.01.036
Padaki, M., Murali, R. S., Abdullah, M. S., Misdan, N., Moslehyani, A., Kassim, M. A., et al. (2015). Membrane technology enhancement in oil-water separation. a review. Desalination 357, 197–207.doi:10.1016/j.desal.2014.11.023
Rajoka, M. S. R., Zhao, L. Q., Mehwish, H. M., Wu, Y. G., and Mahmood, S. (2019). Chitosan and its derivatives: Synthesis, biotechnological applications, and future challenges. Appl. Microbiol. Biotechnol. 103 (4), 1557–1571.doi:10.1007/s00253-018-9550-z
Saleem, J., Riaz, M. A., and McKay, G. (2018). Oil sorbents from plastic wast-es and polymers: A review. J. Hazard. Mat. 341, 424–437.doi:10.1016/j.jhazmat.2017.07.072
Shi, J. J., Lu, L. B., Guo, W. T., Zhang, J. Y., and Cao, Y. (2013). Heat insulation performance, mechanics and hydrophobic modification of cellulose–SiO2 composite aerogels. Carbohydr. Polym. 98 (1), 282–289.doi:10.1016/j.carbpol.2013.05.082
Sun, S. J. (2008). Application of water absorbent materials in oil-water separation. New Chem. Mater. 36 (01), 85–86. [in Chinese].
Takeshita, S., and Yoda, S. (2015). Chitosan aerogels: Transparent, flexible thermal insulators. Chem. Mat. 27 (22), 7569–7572.doi:10.1021/acs.chemmater.5b03610
Uyanga, K. A., Okpozo, O. P., Onyekwere, O. S., and Daoud, W. A. (2020). Citric acid crosslinked natural bi-polymer-based composite hydrogels: Effect of polymer ratio and beta-cyclodextrin on hydrogel microstructure. React. Funct. Polym. 154, 104682.doi:10.1016/j.reactfunctpolym.2020.104682
Wan, C. C., Lu, Y., Jiao, Y., Jin, C. D., Sun, Q. F., and Li, J. (2015). Ultralight and hydrophobic nanofibrillated cellulose aerogels from coconut shell with ultrastrong adsorption properties. J. Appl. Polym. Sci. 132 (24), 42037.doi:10.1002/app.42037
Wang, C., He, G. H., Cao, J. L., Fan, L. H., Cai, W. Q., and Yin, Y. H. (2020). Underwater superoleophobic and salt-tolerant sodium alginate/n-succinyl chitosan composite aerogel for highly efficient oil-water separation. ACS Appl. Polym. Mat. 2 (3), 1124–1133.doi:10.1021/acsapm.9b00908
Wang, J. T., Geng, G. H., Wang, A. Q., Liu, X., Du, J. H., Zou, Z. L., et al. (2015). Double biomimetic fabrication of robustly superhydrophobic cotton fibe-r and its application in oil spill cleanup. Ind. Crops Prod. 77, 36–43.doi:10.1016/j.indcrop.2015.08.044
Wang, J. T., and Liu, S. Y. (2019). Remodeling of raw cotton fiber into flexible, squeezing-resistant macroporous cellulose aerogel with high oil retention capability for oil/water separation. Sep. Purif. Technol. 221, 303–310.doi:10.1016/j.seppur.2019.03.097
Wu, J. X., Dong, Y., Xia, J. Y., Chen, Y., Cao, Y. F., and Liu, Z. L. (2020). Pr-eparation and characterization of lignocellulosic aerogel with high liquid a-bsorbability. Chem. Industry For. Prod. 40 (03), 9. [in Ch-inese].doi:10.3969/j.issn.0253-2417.2020.03.007
Wu, M. M., Xiang, B., Mu, P., and Li, J. (2022). Janus nanofibrous membrane with special micro-nanostructure for highly efficient separation of oil–water emulsion. Sep. Purif. Technol. 297, 121532. doi:10.1016/j.seppur.2022.121532
Xiang, C., Wang, C., Guo, R. H., Lan, J. W., Lin, S. J., Jiang, S. X., et al. (2018). Synthesis of carboxymethyl cellulose-reduced graphene oxide aerogel for efficient removal of organic liquids and dyes. J. Mat. Sci. 54 (2), 1872–1883.doi:10.1007/s10853-018-2900-5
Xu, W. L., Chen, S., Zhu, Y. N., Xiang, X. X., Bo, Y. Q., Lin, Z. M., et al. (2020). Preparation of hyperelastic graphene/carboxymethyl cellulose composite aerogels by ambient pressure drying and its adsorption applications. J. Mat. Sci. 55 (24), 10543–10557.doi:10.1007/s10853-020-04720-5
Xu, Z., Zhu, Z. P., Li, N., Ye, T., and Lei, J. (2018). Continuous in situ extraction toward multiphase complex systems based on superwettable membrane with micro-/nanostructures. ACS Nano 12 (10), 10000–10007.doi:10.1021/acsnano.8b04328
Yang, X., Cao, Q. H., Hong, S., Peng, J. Y., Du, J. T., Xu, Z., et al. (2020). Review of oil-water separation materials based on cellulose. J. For. Eng. 5 (05), 13–20. [in Chinese].doi:10.13360/j.issn.20961359.201908015
Yong, H., Jiang, X. Q., Yin, D., Ge, H. X., Yuan, Y. Y., and Yang, C. Z. (2002). Synthesis and characterization of chitosan-poly(acrylic acid) nanoparticles. Biomaterials 23 (15), 3193–3201.doi:10.1016/S0142-9612(02)00071-6
Yuan, S. S., Strobbe, D., Kruthb, J. P., Van Puyvelde, P., and Van der Bruggen, B. (2017). Super-hydrophobic 3D printed polysulfone membranes with a switchable wettability by self-assembled candle soot for efficient gravity-driven oil/water separation. J. Mat. Chem. A 5 (48), 25401–25409.doi:10.1039/C7TA08836A
Yuan, Y. J., Li, R. Y., Sun, K. W., and Li, G. (2016). Preparation of citric acid crosslinked cellulose aerogel and characterization of the aerogel’s network structure. Mater. Rep. 30 (14), 5. [in Chinese].
Yue, X. J., Li, Z. D., Zhang, T., Yang, D. Y., and Qiu, F. X. (2019). Design and fabrication of superwetting fiber-based membranes for oil/water separation applications. Chem. Eng. J. 364, 292–309.doi:10.1016/j.cej.2019.01.149
Zhang, F., Zhang, W. B., Shi, Z., Wang, D., Jin, J., and Jiang, L. (2013). Nanowire-haired inorganic membranes with superhydrophilicity and underwater ultralow adhesive superoleophobicity for high-efficiency oil/water separation. Adv. Mat. 25 (30), 4192–4198.doi:10.1002/adma.201301480
Zhang, Y., Yin, M. L., Li, L., Fan, B. J., Liu, Y., Li, R., et al. (2020). Construction of aerogels based on nanocrystalline cellulose and chitosan for high efficient oil/water separation and water disinfection. Carbohydr. Polym. 243, 116461.doi:10.1016/j.carbpol.2020.116461
Keywords: chitosan, carboxymethyl cellulose, water absorption (A), water retention, aerogel, emulsion separation
Citation: Lu H, Jiang X, Wang J and Hu R (2022) Degradable composite aerogel with excellent water-absorption for trace water removal in oil and oil-in-water emulsion filtration. Front. Mater. 9:1093164. doi: 10.3389/fmats.2022.1093164
Received: 08 November 2022; Accepted: 01 December 2022;
Published: 14 December 2022.
Edited by:
Anthony Chun Yin Yuen, University of New South Wales, AustraliaReviewed by:
Jian Li, Northwest Normal University, ChinaWei Wang, University of New South Wales, Australia
Copyright © 2022 Lu, Jiang, Wang and Hu. This is an open-access article distributed under the terms of the Creative Commons Attribution License (CC BY). The use, distribution or reproduction in other forums is permitted, provided the original author(s) and the copyright owner(s) are credited and that the original publication in this journal is cited, in accordance with accepted academic practice. No use, distribution or reproduction is permitted which does not comply with these terms.
*Correspondence: Xueliang Jiang, amlhbmd4bEB3aXQuZWR1LmNu; Jintao Wang, d2p0MTk4NjEyMEAxNjMuY29t; Ruofei Hu, cmh1dmlwQGhidWFzLmVkdS5jbg==