- 1Department of Bioengineering, Faculty of Chemical and Metallurgical Engineering, Yildiz Technical University, Istanbul, Turkey
- 2Materials Technologies, Marmara Research Center, TUBITAK, Kocaeli, Turkey
- 3Department of Bioengineering, Faculty of Engineering, Marmara University, Istanbul, Turkey
- 4Center for Nanotechnology and Biomaterials Application and Research (NBUAM), Marmara University, Istanbul, Turkey
- 5Department of Biomedical Engineering, Fatih Sultan Mehmet Vakif University, Istanbul, Turkey
- 6Technology Transfer Office, Fatih Sultan Mehmet Vakif University, Istanbul, Turkey
- 7Health Biotechnology Joint Research and Application Center of Excellence, Istanbul, Turkey
In December 2019, Coronavirus pandemic (COVID-19) caused by Severe Acute Respiratory Syndrome Coronavirus 2 (SARS-CoV-2) viruses, which affected the whole world, is emerged. The details on the epidemiology, infection source, transmission mode, and prognosis of SARS-CoV-2 gave in this review. Universal infection control standards such as hand hygiene, environmental cleanliness, use of personal protective equipment, and quarantine used to prevent the spread of COVID-19 without vaccine. However, many vaccine candidate studies carried out globally with using traditional and technological approaches. Innovations in technology allow the development of nanotechnological tools and the formation of systems that will inactivate SARS-CoV-2 in patients. It expected to include technologies that combine different disciplines, especially robotic applications, antimicrobial nanotechnology, and tissue engineering for the future treatment of COVID-19. This review-based work discusses the relationship of COVID-19 and nanotechnology based working principles.
1 Introduction
Nanotechnology is used to achieve precision manufacturing at the nanometer scale (10–9 m). In its most comprehensive expression, it is the most current technology that enables the production of materials to be used in chemical, physical, and biological systems in submicron sizes and the integration of the obtained nanomaterials into larger systems (Nasrollahzadeh et al., 2019). The developed nanomaterials (Figure 1) are classified into four classes according to their dimensionality. First and second classes are miniature materials (nanospheres and clusters) and one-dimensional materials (nanotubes, wires, and rods), respectively. Other classes are called as two dimensional materials (thin films, plates, and layered structures), and three-dimensional materials (bulk nanomaterials, hydrogels, and polycrystals) (Poh et al., 2018). These materials have more functionality due to their small size, and contribution for developing the physical, chemical, electrical, mechanical, and optical properties of the systems in which they are used (Lan, 2022). Also, they bring many innovations from daily life to the industrial area (Nasrollahzadeh et al., 2019). The application of nanotechnology (Figure 2) has branched out into a large number of various areas of science as environmental applications (i.e., energy conversion/storage/transmission, and water treatment), agricultural industry applications, cosmetics, and nanomedicine (Kargozar and Mozafari, 2018). Furthermore, according to latest studies, the nanomaterials play an important role in the imaging, diagnosis, and treatment processes of diseases caused by viral infections, including SARS-CoV-2. Enzyme-based tests (Mahmoudinobar et al., 2021) and infection diagnostic kits (Singh et al., 2021) used for diagnosis of COVID-19; gloves, masks (Mallakpour et al., 2022), disinfectants (Talebian et al., 2020), and vaccines (Kashte et al., 2021) used to prevent spread of COVID-19; and drug delivery systems (Chowdhury et al., 2021) for the treatment of COVID-19. Considering the effect of the virus and the problems it causes, emergency solutions based on nanotechnology should be developed because the virus threatens the lives of hundreds of people every day (Campos et al., 2020; Ruiz-Hitzky et al., 2020).
1.1 Coronaviruses and SARS-CoV-2
The world is currently dealing with COVID-19, a serious and acute respiratory problem that started in December 2019 and is still an ongoing issue (Tavares et al., 2022). Since the disease began to spread, it has caused close to 600 million confirmed cases of COVID-19 and approximately 6.5 million deaths up to 26 August 2022 (WHO, 2022a). The disease was global pandemic in March 2020 by the World Health Organization (WHO). SARS-CoV-2 first emerged in Wuhan, China that belongs to the Coronavirinae subfamily (family: Coronaviridae) (Campos et al., 2020; Stewart et al., 2020). SARS-CoV-2 is a spherically enveloped RNA virus that leads to higher pathogenicity, contagiousness, and mortality rates than SARS-CoV, and the Middle East respiratory syndrome coronavirus (MERS-CoV) (Rossi et al., 2020). SARS-CoV-2 has structurally four main proteins as Spike (S), Envelope (E), Membrane (M), and Nucleocapsid (N) proteins (Figure 3). The S protein is the glycosylated main surface protein which covers the surface and is crucial for the attachment of viruses to the host cell and entering it (Xia, 2021). The E protein is the small structural protein and is involved in viral assembly (Schoeman and Fielding, 2019; Rahman et al., 2021). The M protein is the most abundant structural protein and especially involved in the formation of the viral envelope (Schoeman and Fielding, 2019). The N protein is the phosphoprotein that only binds to the RNA genome and involved in the formation of nucleocapsids (Dutta et al., 2020; Zeng et al., 2020; Gao et al., 2021). Understanding the entry mechanism of the virus is very important in the development of new treatments against its infectivity and pathogenesis. Therefore, it is necessary to investigate the functions of the main structural proteins and their effects on the entry mechanism of SARS-CoV-2 (Figure 3) in detail (Al Adem et al., 2020).
The life cycle of the virus begins with its entry into host cells. The SARS-CoV-2 attaches to the host cell surface protein angiotensin-converting enzyme 2 receptor (ACE2) which is the target receptor of SARS-CoV-2 (Coperchini et al., 2020). After membrane fusion, the virion can release RNA into the host. The translation of the structural proteins (except N protein) occurs in the ER due to post-translational modification, while only the translation of the N protein occurs in the cytoplasm. The newly assembled and matured virions are released from the host cell in three different ways by budding, exocytosis, or cell death. The released virions are ready to infect healthy cells via reported modes of transmission (Poduri et al., 2020; Chen et al., 2021; Khade et al., 2021; Pizzato et al., 2022).
1.2 COVID-19
The sources of infection and transmission are very important for developing new approaches and controlling the prevention of infection. Normally, the source of COVID-19 is unclear. However, it is believed that the first source may be bats (Yin and Wunderink, 2018; Rahman et al., 2020). It should be noted that, as with bats, other animals sold in Wuhan animal markets, such as civet cats, foxes, mink, and raccoon dogs, also carry SARS-CoV-2. When the samples taken from the patients who had contact with these animals and the samples from these animals are compared, it is observed that the virus types are the same. As a result, live animals susceptible to SARS-related CoV were identified as the main progenitors of COVID-19 (Lytras et al., 2021). For this reason, it is even believed that SARS-CoV-2, like other coronaviruses, is transmitted zoonically. However, COVID-19 can be directly transmitted from human to human (Jayaweera et al., 2020).
According to the statements of Chinese health authorities, the transmission in humans occurred via direct contact, aerosol, and droplet (Figure 3). Although direct transmission means direct contact with virus infected objects or surfaces, especially close contact with the virus infected people’s mucous membranes of the mouth, eyes, and tears (Crawford et al., 2022). In aerosol or droplet transmission, the diameter of droplet is a critical issue. According to WHO, the droplets have 5–10 μm diameter and the aerosols have less than 5 μm diameter. During respiratory activities such as coughing, breathing, laughing, or talking, both of these large and small droplets can be produced. The viral particles encapsulated within these droplets. Therefore, droplet transmission requires close contact between an infected person and a susceptible person (Ge et al., 2020; Wilson et al., 2020). Also, studies have shown that aerosols can travel more than six feet due to their ability to be suspended in the air (Kutter et al., 2018). Small aerosols are trapped deep in the lungs and cause infection in the lower respiratory tract, while large droplets are trapped in the upper respiratory tract (Jayaweera et al., 2020). According to data from patients, older adults have a higher risk of contracting the disease and a higher mortality rate, because, the levels of hormones in the immune system change during aging. In older adults, some comorbidities such as cardiovascular diseases, respiratory diseases, cancer, and diabetes problems become more prominent, making the elderly more susceptible to COVID-19 (Farshbafnadi et al., 2021). Also, severe COVID-19 is not limited to the elderly population as stated; children and young adults are also at risk. It is inevitable that patients with underlying diseases will experience dangerous symptoms and life-threatening complications if they are infected with the SARS-CoV-2 (Chao et al., 2020; Harrison et al., 2020).
1.2.1 Symptoms
The period between exposure to the virus and the onset of clinical symptoms is called the incubation period, and it is very important in determining the case definition and the establishment of public health programs aimed at taking new precautions against COVID-19 and reducing local transmission. According to WHO, the incubation period of SARS-CoV-2 ranged from 1 to 14 days (WHO, 2020a; Elias et al., 2021). Patients exposed to COVID-19 exhibit mild to moderate symptoms such as headache, fever, fatigue, dry cough, loss of taste and smell, diarrhea, dyspnea, sore throat, chest pain, muscle pain, and abdominal pain (Weng et al., 2021). The clinical features of patients infected with SARS-CoV-2 were first determined by Huang C. et al. (2020; Huang W. C. et al., 2020). According to the classification made by the National Health Institutes (NIH), the disease has five types. First, they are asymptomatic or presymptomatic patients who have positive tests for COVID-19, but do not show clinical symptoms. Secondly, patients with obvious COVID-19 symptoms such as fever, cough, malaise, headache, but no shortness of breath. Third is that patients with clinical symptoms in the lower respiratory tract. Fourth is severe illness that is determined according to a ratio of partial pressure of arterial oxygen to fraction of inspired oxygen. The last one is that critical patients that have especially multiple organ dysfunction, acute respiratory failure, and septic shock. The acute respiratory syndrome begins after 1 week the onset of symptoms and progresses critically (COVID-19 Treatment Guidelines Panel, 2022).
Here, the current nano-based approaches and developments for the prevention, diagnosis, and treatment of COVID-19 detailed in order to emphasize how nanotechnology can help to control viral infections. Moreover, the developed nanotechnological solutions and the connections of multidisciplinary fields during the COVID-19 pandemic are mentioned in sections.
2 Nanotechnology and personal protective equipment for COVID-19
2.1 Personal protective equipment
Although there are many treatment methods developed against COVID-19, there is still a lack of an effective method. In addition, problems such as vaccine and drug development strategies take time, and faster solutions need to be developed to prevent the spread of the virus. The prevention of SARS-CoV-2 without vaccination or other administrative controls could be achieved by using universal infection control standards such as hand hygiene, environmental sanitation, maintaining social distance, personal protective equipment (PPE), and quarantine. However, the most visible but limited control standard is the use of PPE. People who use PPE correctly, especially healthcare workers, are largely protected from the virus and the potential harm they can cause both to themselves and to the environment is reduced. The PPE can act as a physical barrier against viral pathogens. The frequently used PPE types are filtering face piece respirator masks (N types, R types and P types), surgical face masks, gowns/apron, gloves, eye shields, goggles, and boots/closed work shoes (Figure 4) (WHO, 2020b). The applied nanotechnological amplifications contribute to the properties of PPE such as UV protection, antimicrobial properties, and fire retardant (Chintagunta et al., 2021). In addition, nanotechnology can provide hydrophobic and comfortable products for fighting with COVID-19 (Campos et al., 2020).
2.1.1 Nanotechnology in masks
Masks are the most important equipment designed to prevent the entry of pathogens such as viruses from the mouth and nose and to protect both the wearer and the immediate environment from respirable harmful agents. The masks are divided into 3 classes according to their filtering types; single-use face masks, respirator masks, and surgical masks (Figure 5) (Tcharkhtchi et al., 2021).
An ideal mask filters bioaeresols effectively and offers a high level of comfort. However, some external factors like humidity, temperature and pattern of airflow and material properties of masks affect filtration efficiency and mask quality. During pandemic challenge, nanomaterials are the best developed solutions to improve the filtration efficiency of masks when added to textile products. Simultaneously, they can have direct impact on the survivability of viruses that come into contact with the mask. For example, both nanofibers and NPs introduced to masks for improving of antiviral properties, filtering capacity, and breathability properties. Nanofibers produced by electrospinning technology are very useful materials with average fiber diameters in the nanometer range because of their higher surface area to volume ratio that can be easily functionalized with desired property (Ullah et al., 2020). Microorganisms reproduce rapidly in a moist environment. For this reason, thermal optimization of the masks is crucial. In one example, Yang et al. gave a cooling effect and excellent particle filtering properties to masks by using nanofiber on nanoporous polyethylene (Yang et al., 2017).
Although NPs have many advantages, high surface/volume ratio causes an increase in toxicology. They carry many risks for both public health and the environment due to insufficient epidemiological studies (Valdiglesias and Laffon, 2020). Priority should be given to the development of new systems that can test the effects of nanoparticles on human health and the environment so that they can be applied to masks (Palmieri et al., 2021). As one of the new technologies, three dimensionally (3D) printing technology was applied to produce face masks against COVID-19. The new masks were designed with reusable purposes. In 3D-printed masks, only filter material requires consistent replacement. The other parts can be easily recycled and therefore 3D-printed masks are more sustainable than single-use face masks (Choong et al., 2020; Deng et al., 2022). According to new studies, Swennen et al. (Swennen et al., 2020) have produced 3D printed, custom-made, reusable N95, personalized masks. They used two reusable polyamide composite components (face mask and a filter membrane support) and two disposable components (a head fixation band and a filter membrane) during production. Although 3D face masks combined with FFP2/3 filter membranes are presented as a valid alternative source, there is no virological validation and no data on the safe reuse of the face mask with new filter membranes and headbands. In another study, a 3D printed mask was produced by Provenzano et al. (Provenzano et al., 2020). These simple materials are composed of reusable and printable headpiece to which different transparent plastic sheet can be connected to form a face shield. They can easily protect user’s eyes and mouth (Tino et al., 2020).
Although 3D-printed masks provide some protection and are more sustainable, their filtering performance has not yet been approved by any regulatory agency. Filtering efficiencies are very low due to leakages between the interfaces on the printed mask. Therefore, it is not recommended to use 3D-printed masks as an alternative to medical masks until sufficiently sensitive and reliable tests are developed to measure filtration efficiencies. In addition, the printing parameters should be improved, and further optimizations should be made by the researchers for increasing the filtration efficiency (Deng et al., 2022).
2.1.2 Nanotechnology in gloves
Medical gloves are one of the important PPE used by healthcare professionals to prevent the spread of the virus. There are several types of gloves (Tabary et al., 2021). However, nitrile and latex-based gloves have been used frequently in the pandemic. The biggest problem of gloves is the inappropriate use of them. If they are used uncontrollably, they can prepare a shelter environment for viruses and microorganisms. Especially, if hands are touched to the face after contact with unclean surfaces, the risk of virus transmission is very high. Therefore, with the addition of virucidal agents, the risk of virus transmission of gloves is reduced. As with masks, many studies have been carried out using nanotechnology in gloves. The antibacterial effects of silver nanoparticles (AgNPs) were used in glove production and it was proven to have virucidal activity (Aydemir and Ulusu, 2020). In another aspect, viruses can enter host cells by using the ACE2 receptor. If the ACE2 level of the body is decreased, the penetration of the viruses can be blocked and reduced. Therefore, the catching of the viruses before entry into cells by using nanotechnology would be exceedingly helpful. As a result of the studies, it has been observed that nanomaterials containing ACE2 reduce the infection rate. ACE2 proteins coated with NPs neutralize viruses and prevent them from entering host cells. It is extremely important to use nanotechnology-based, nanoparticle coated ACE2 proteins in the production of glove (Aydemir and Ulusu, 2020; Rasmi et al., 2021). However, without relying solely on nanotechnology, care must be taken to use gloves carefully (Yadav D. K. et al., 2020).
2.1.3 Nanotechnology in disinfectants
Disinfectants or antiseptics are chemical substances specially prepared to inactivate or destroy microorganisms. If it completely kills microorganisms in the places where they are used, sterilization is achieved. However, the effectiveness of a disinfectant depends on its chemical composition, the characteristics of the pathogen in which it is used, and the intracellular vulnerability. One of the most important ways to prevent COVID-19 is to reduce transmission routes by using disinfectants. The disinfectant types that are frequently used in the COVID-19 are classified as alcohol, phenol, formaldehyde and glutaraldehyde-based, oxidizing agents, chlorine, and iodine releasing agents. The mechanism of each disinfectant is different. For example, alcohols denature proteins in microorganisms and cause cell lysis by causing membrane damage. In particular, it was stated that ethanol showed virucidal activity on both living and non-living surfaces (Al-Sayah, 2020; Dhama et al., 2021). Another agent, chlorine, damages the cell wall of microbes by affecting the oxidation of lipids and proteins due to its electronegativity. If the lipid E protein, S protein, glycoproteins, and viral genome of the virus are damaged or disrupted, the virus loses its activation and infectivity (Al-Sayah, 2020).
Considering that the virus is likely to be transmitted to many surfaces during the pandemic, frequent disinfection and sanitization of hands, touched objects and surfaces are very important. However, the possible effects of constantly used disinfectants on humans, animals, the environment, and ecological balance should not be ignored. Therefore, the development of safer and more environmentally friendly disinfectants as an alternative will help reduce the side effects of chemical disinfectants. Nanotechnology enables the production of safer and healthier products in this regard. In particular, metallic NPs such as silver, copper, and titanium have been shown to have virucidal activity against SARS-CoV-2. According to the studies, multifunctional disinfectants were obtained by adding AgNPs to sanitizers using nanocolloidal techniques. The disinfectant obtained has a broad spectrum as it shows activity against viruses, bacteria, and fungi. The silver ions in the disinfectant inactivate the protein structures of microorganisms by denaturing them (Rasmi et al., 2021). Another significant topic is the size of the particles. It has been reported that NPs smaller than 20 nm can easily bind to pathogens including SARS-CoV-2 and cause death (Campos et al., 2020). NanoTech Surface Company, has developed a nanopolymer-based (containing silver and titanium dioxide NPs) disinfectant for disinfection of coronavirus-infected surfaces. The most important advantages of the developed disinfectant were that it was eco-friendly, non-flammable, and biodegradable (StatNano, 2022). In another study, nano-disinfectants that can neutralize microbes both in the air and on surfaces have been developed. The developed nano-disinfectant consists of various components such as deionized water, electrolyzed water, and hydrogen peroxide can be used in COVID-19 (Vaze et al., 2019).
3 Using nanoparticles in COVID-19 testing
Nanotechnologies integrated into NPs demonstrates that nanomaterial-based approaches can lead to an important improvement in NP target detection performance (Medhi et al., 2020; Chowdhury et al., 2021). Among the presented nanotechnology approaches is the use of NPs in personalized medicine applications that do not require complex or expensive equipment for signal detection (Abdellatif and Alsowinea, 2021). In addition, the ability to measure the test reading is important for devices used in personalized medicine applications. Therefore, integrated nanotechnologies in NPs should allow easy signal quantification, either semi- or full-quantitatively, according to the type of biomarker being studied (Izcovich et al., 2020). The early diagnosis of viral is critical to reduce the possibility of widespread outbreaks in hospitals and nearby populations. Normally, the diagnosis strategies can be investigated in molecular tests, serologic tests, and imaging techniques (Cascella et al., 2020). The first step of diagnosis is based on the history of patients. The second step is recommendation of Chest-X-Ray and CT (Computed Tomography) to detect glass opacity and patchy bilateral shadows in the lungs (Tavakol et al., 2021). The molecular identification of the SARS-CoV-2 genome in pharyngeal swab samples using a real-time back transcription-polymerase chain effect (rRT-PCR) test is the gold standard for COVID-19 diagnosis. rRT-PCR used to detect the RNA genome of SARS-CoV-2, however, it requires biosafety level 2 or above (Corman et al., 2020) and results of it are obtained for up to 3 days. Therefore, it is necessary to develop new point-of-care (POC), cost-effective, fast, and highly reliable devices. The serological tests for specific immunoglobulin -M (IgM) and -G (IgG) has been offered as an alternative method to decrease false negatives rate related with rRT-PCR test but the results showed that they have limited benefit (Latiano et al., 2021). Screening of symptomatic and asymptomatic patients is different. Upper respiratory tract specimens such as nasopharyngeal swabs or nasal aspirates are used to screen asymptomatic patients, while lower respiratory tract specimens such as sputum or bronchoalveolar lavage are used in symptomatic patients. In addition, saliva is also used to detect respiratory viruses. The most important advantage of using saliva is that it reduces waiting time for patients and reduces the risk of viral transmission to healthcare workers. It has also been reported that the rate of detecting respiratory viruses in saliva is comparable to Nasopharyngeal swabs (Ochani et al., 2021). All of the diagnosis tests and imaging procedures have their advantages and disadvantages. However, nanotechnology is the best tool to use in the development of new tests for detecting SARS- CoV-2 (Tharayil et al., 2021).
Biosensor technology plays a significant role in diagnosing COVID-19 (Behera et al., 2020; Choi, 2020; Leïchlé et al., 2020; Samson et al., 2020; Abid et al., 2021; E, S, Innovation Center et al., 2021; Maddali et al., 2021), because of their cost-effectiveness, high sensitivity, biocompatibility, and mass production potential (Behera et al., 2020). Biosensor is a device containing biological sensing material combined with a physicochemical transducer that can convert a biological signal into a measurable and processable electrical signal (Abid et al., 2021). Currently, various types of biosensors have great commercialization potential to meet the disadvantages by the COVID-19 (Fathi-Hafshejani et al., 2021). With the development of biosensor technology, it can be said that a new era has begun in disease diagnosis. In fact, it will be possible to keep the patient under constant observation thanks to the biosensors placed inside the body (Bahl et al., 2020). Here, biosensors, their working principles, importance technologies for COVID-19, and primary application in medicine briefly discussed.
3.1 Components and working principle of biosensors
The block diagram of the biosensor consists of three parts: sensor, transducer, and analyte. If the receptor is in a biomolecular structure, it is called a bio-receptor. Bio-receptors are biomolecules (enzymes, tissues, nucleic acids, antibodies, receptors, and organelles) that can recognize the analyte (Behera et al., 2020). Bio-receptors with the suitable combination transform this analyte with the analyte-specific bio-receptor structure (Behera et al., 2020; Al-Douri et al., 2021; Wu and Wu, 2021; Yasri and Wiwanitkit, 2022). Transducers, on the other hand, are formations that transform the neither chemical nor physical signal manufactured by the bio-receptor when recognizing the analyte into electrical signals (Behera et al., 2020). In biosensors; bio-receptor materials send detectable signals to the transducer which can be used in the system as piezoelectric, optical, electrical, electrochemical, thermal, and others. The bio-receptor and the transducer are associated to each other by an appropriate neither physical nor chemical pathway (Behera et al., 2020; Al-Douri et al., 2021; Wu and Wu, 2021; Yasri and Wiwanitkit, 2022).
The transducer is one of the most important element of biosensor (Zhao et al., 2021a). The moderately deliberate atmosphere of the biosensor reaction clearly relieves electrical noise purification anxiety (Pang et al., 2021). An analog wave or a signal is converted in a digital arrangement and recognized to a microprocessor progressive output. Here, information is processed, tailored to preferred units, and sent to data storage. Physical type biosensor applications are able to offer a direct advantageous effect in tackling challanges arising from the COVID-19 (Ashraf et al., 2021).
3.2 Biosensor technologies and COVID-19
Biosensor technologies have an essential role in diagnosing COVID-19 (Choi, 2020). In the last 20 years, the biosensor-based instruments have been accelerated in medical diagnostics, because of their biocompatibility, cost-effectiveness, high sensitivity, and potential for mass production (Bahl et al., 2020; Xu and Li, 2020; Mobed and Sepehri Shafigh, 2021; Zare et al., 2021). Currently, various types of biosensors have great commercialization potential to meet the challenges posed by the COVID-19 (Murugan et al., 2020; Fani et al., 2021; Saki et al., 2021). Certainly, these sensors have enormous potential in other fields, containing medical diagnostics, and warrant further research (More, 2021; Narita et al., 2021; Wu and Wu, 2021).
Industry 4.0’s digital technologies have the ability to detect COVID-19 symptoms (Wu and Wu, 2021). This helps to avoid confusion and predict the probability of contracting disease (Sharma et al., 2021). Thermometric and piezoelectric biosensors are types of physical sensors (Bahl et al., 2020). Further sensing is accomplished using NPs, while thermometric biosensors work to measure temperature changes associated with biological samples (Wu and Wu, 2021). Thermal biosensors are generally used to measure cholesterol (Dinnes et al., 2021). These biosensors can be easily worn on a daily basis and can be used with everyday clothing; t-shirts, pants, headphones, and wrist watches. Therefore, thanks to its easy use, the real-time health status of the patient can be checked (Kudr et al., 2021).
Various types of biosensors are present to supply medical care benefits to humans (Abid et al., 2021; Wu and Wu, 2021). Electrochemical, optical, physical, and wearable biosensors are the main categories of biosensors (Ribeiro et al., 2020; Shrivastav et al., 2021). Researchers combined nanotechnology with traditional optical biosensor technology (Murugan et al., 2020; Nag et al., 2020) to successfully detect SARS-CoV-2. A new nanotechnology-based biosensor named ‘Graphene-Field-Effect Transistor biosensors’ (Xu S. et al., 2021) can detect changes in the environment on their surface and provide ultra-sensitive and low-noise sensing. However, the performance of the developed nanotechnology-based biosensor is specified using antigen protein, cultured virus, and nasopharyngeal swab samples from COVID-19 patients (Vashist et al., 2012; Maheshwari et al., 2021). Nanocomposites based biosensors are able to be used to monitor and re-transport infections in food for COVID-19 patients. Nanomaterials increase their adaptability and sensing abilities (Huang et al., 2021). A newly released biosensor technology has been developed for COVID-19 that illustrated a high value of specificity and sensitivity (Lizhou Xu and Shoaie, 2020). This optical biosensor contains a gold nanostructure on a glass substrate. In the future, it is foreseen to develop a biosensor that can be used in busy places to determine the presence of coronavirus in the environment and measure its concentration in the air in real time, and the recorded data will be sent wirelessly and monitored in real time (Shand et al., 2022).
3.3 Lateral flow tests and COVID-19
Various types of biosensors have been advanced to determine biomarkers linked with various diseases (Gupta et al., 2020; Kim et al., 2021; Pérez-García et al., 2021). Lateral flow tests (LFTs) (Cui and Zhou, 2020; Rosati et al., 2021), which are paper-based devices, are able to meet the needs wait of a biosensor (specific identification of target analyte, low cost, stability, user-friendly test format, fast, and low sample volume) (Ozturk et al., 2021). In addition, proteins, nucleic acids, and whole cells are biomarkers of LFTs, and they serve as POC testing (Mlcochova et al., 2020). This can provide support for patients and personalized medicine. Paper-based POC immunoassays, particularly LFTs, have received great attention because of the requirement for joint qualitative and quantitative biosensing applications. For the purpose of diagnosing human health, different LFTs have been commercialized for the detection of different markers (Violan et al., 2021). Nanomaterials have been used for the development of POC diagnostics and delivery strategies. Despite the numerous benefits of LFTs, they have some challenges, such as difficulty of sensitivity detection of a particular target analyte without applying signal amplification strategies (Rodriguez-Manzano et al., 2020). Availability of cost-effective rapid diagnostic testing is very important for physicians in emergency departments, clinics and hospital. These diagnoses allow nurses and doctors staff to simply prioritize patients, and hinder further spread of disease (Uzay and Dinçer, 2022).
3.3.1 Observation and monitoring
Rapid identification of the disease is one of the factors preventing the spread of a mass infection. The success of a system depends on effective collaboration and communication between federal and state public health laboratories, hospitals, government agencies, and communities. WHO testing should be widely used to stop this epidemic (Bonnechère et al., 2021).
3.3.2 Therapeutics
Once individuals with COVID-19 are identified, patients need to be treated. These treatments can inhibit the replication of SARS-CoV-2 in the host. Fundamental studies of nano-bio interactions are about explaining how SARS-CoV-2 infects its cells (Singhera et al., 2021). Nucleic acid amplification tests (NAATs), such as polymerase chain reaction (PCR), primarily detect viral genomic RNA encoding S and N proteins (Kamat et al., 2021). Antigen tests, such as LFTs, primarily detect the N protein. NAATs are the gold standard diagnostic for SARS-CoV-2 infection (Tyagi et al., 2020). SARS-CoV-2 has developed many mutations that can result in immune escape (Moabelo et al., 2021). Since NAATs use nucleic acid primers to recognize and amplify their targets, the incorporation of one or more base substitutions into the targeted nucleotide sequence is potentially sufficient to inhibit the reaction. LFTs are inexpensive, user-friendly and rapid diagnosis that can be used at the POC (Jain et al., 2020).
In comparison with the S protein, the N protein is relatively conserved from variant to variant (Devi et al., 2021; Weinberg et al., 2021). Consequently, detection of N protein instead of the S protein should result in a test that is more resistant to diagnostic evasion. In fact, every rapid detection test approved by the FDA under Emergency Use Authorization since 2020 is still recommended for SARS-CoV-2 detection (Tinberg et al., 2013). Companies have developed rapid tests using mAbs previously isolated from SARS-CoV N protein immunizations (Gage et al., 2021) However, thanks to continuous accumulation of mutations in the N protein may be used to maintain high diagnostic efficiency for improved mAbs and diagnostic tests specifically for SARS-CoV-2 (Tinberg et al., 2013; Sandersjöö et al., 2015; Nilsson et al., 2017; Yadav N. et al., 2020; Gage et al., 2021). Prototype LFTs are available for detection of SARS-CoV-2 N protein in clinical samples (Jain et al., 2020; Dowlatshahi and Abdekhodaie, 2021).
4 Developing a vaccine against COVID-19
Vaccines are very special and an essential component of public health, as they protect individuals and communities (Sebastian et al., 2020; Excler et al., 2021). Edward Jenner made the first successful vaccine study in the late 1700s. To date, vaccine development and large-scale immunization campaigns have been used by society against worldwide epidemics of infectious diseases (Verbeke et al., 2021). There are some criteria for developing an ideal vaccine. The developed vaccine must be safe and highly effective, even in immunocompromised individuals. In addition, it should be inexpensive, free from toxicity/side effects, capable of long-term protection, and high thermal stability (Malik et al., 2021).
The development of a new vaccine is a complicated process that takes 10–15 years (Sebastian et al., 2020). The economic situation of the countries has a very important role in the creation of a new sustainable vaccine development model, in which significant investments make and which includes close cooperation between the public and private sectors. In low- and middle-income countries, most vaccines for diseases cannot be developed due to weak or lacking market incentives (Rappuoli et al., 2019). The process of the development of vaccines is mainly based on four different stages: discovery, pre-clinical development, clinical development (Phase I, Phase II, and Phase III), and post-marketing. However, unlike traditional vaccine development processes, it has enabled the rapid development of COVID-19 vaccines based on the knowledge available in vaccines developed for COVID-19 (Figure 6) (Ndwandwe and Wiysonge, 2021).
In a fast-paced research environment such as the COVID-19 pandemic, using samples collected in December 2019, and then Edward C. Holmes et al. published the full genome sequence of SARS-CoV-2 on virological.org on 10 January 2020. On 11 January 2020, vaccines against COVID-19 began to be developed, when the genome sequence of SARS-CoV-2 made available by the United States (US) NIH (Haque and Pant, 2020; Wang C. et al., 2020). The COVID-19 vaccines currently approved for use in the US are Pfizer/BioNTech, Moderna, Novavax, and Johnson & Johnson’s Janssen (CDCP, 2022). In the United Kingdom Moderna, Oxford/AstraZeneca, and Pfizer/BioNTech vaccines currently approved (National Health Service, 2022). In Türkiye vaccination first started with the inactivated CoronaVac, then with the mRNA BNT162b2 vaccine (Batibay et al., 2022). According to the WHO COVID-19 Dashboard on 19 September 2022, a total of 12.640.866.343 vaccine doses were administered (WHO, 2022b).
4.1 The types of vaccines for COVID-19
Adjuvants plays important role for enhancing vaccine efficacy (Lee et al., 2022). Rino Rappuoli and colleagues introduced the genome-based reverse vaccination method with strong antibody responses. However, investigation of other factors (i.e. adjuvants and antigen delivery), has gained momentum as they influence the induction of immune responses and immunological memory, which are important for vaccine efficacy (Raeven et al., 2019). Alum, complete Freund’s adjuvant, thiomersal, incomplete Freund’s adjuvant, montanide, lipovant, and adjuvant 65 are currently available adjuvants used to develop a subunit vaccine (Nevagi et al., 2019). In addition to finding new adjuvants, researchers are also modifying existing adjuvants to increase immune-inducing ability and reduce toxicity. It is known that their modification of the epitope further enhances the immunogenicity of subunit vaccines, increasing stability, half-life, and solubility which collectively increase the Th1 response (Mekonnen et al., 2022).
Multiple methodologies have been adopted for the development of vaccines against SARS-CoV-2, including both next-generation and conventional techniques (Malik et al., 2021). Researchers around the world have been racing to develop COVID-19 vaccines, with more than 175 candidate vaccines in the clinical evaluation stage and another 199 vaccines in preclinical evaluation as of 11 November 2022 (WHO, 2022b) (Figure 7). Nowadays, more than 150 companies or educational institutions offer are making efforts to combat the current coronavirus pandemic with strategies (Malik et al., 2021) such as live attenuated vaccines, inactivated vaccines, subunit vaccines, vector based vaccines, DNA-based vaccines, and mRNA-based vaccines.
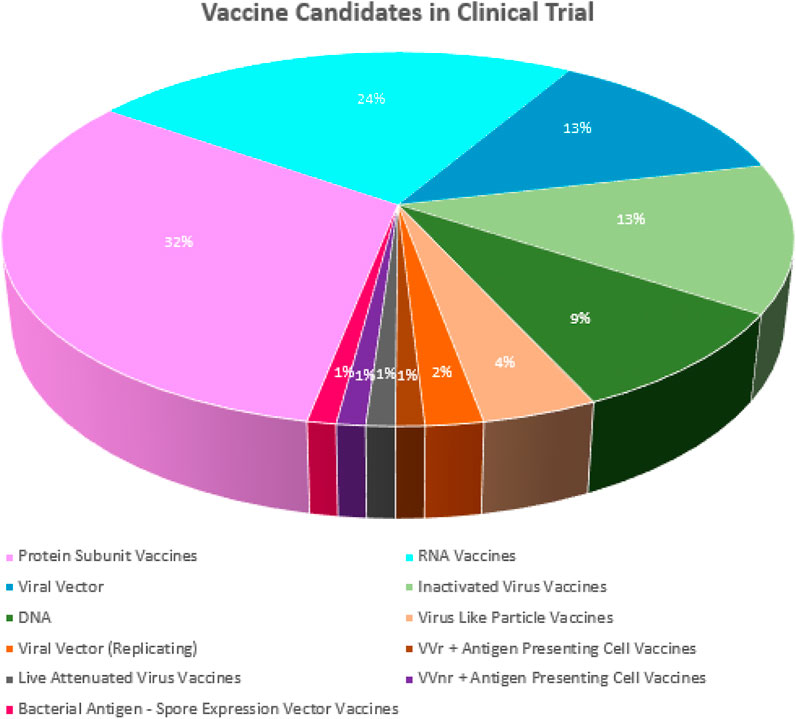
FIGURE 7. Current stage of development of clinical 11 November 2022 (Recreated from WHO (WHO, 2022b).
4.1.1 Live attenuated vaccines (LAV)
Chemically inactivated and genetically engineered vaccines have been developed since 1896, and up to 15 LAVs (against 12 viral and 3 bacterial diseases) have been used (Pöyhönen et al., 2019). Composed of live but attenuated microorganisms that aim to activate innate immune responses, LAVs do not cause disease in humans but also contain replication-competent viral vectors. LAVs can induce prolonged, robust cellular and humoral immune responses without the need for adjuvants (Mok and Chan, 2020). LAVs can be much more effective compared to other vaccines as they are similar to excellent mRNA vaccines or vector vaccines and can unparalleled manufacture multiple, endogenous and relatively large amounts of viral antigens. However, LAVs are not suitable for viruses that reproduce poorly in in vitro analysis (Chen, 2022). The bacille Calmette-Guerin (BCG) vaccine has been administered for the prevention of tuberculosis (Brooks et al., 2021). However, BCG can lead to heterologous immunity by the mechanism of stimulation of antigen-independent B and T Cells, resulting in reduced viral load of SARS-CoV-2 (Khera et al., 2021).
4.1.2 Inactivated vaccine
Purified inactivated vaccines used in conventional vaccine development have been found to be safe and effective in preventing diseases caused by pathogens such as influenza virus and polio virus (Gao et al., 2020). CoronaVac, which is an inactivated whole virus vaccine adjuvanted with aluminum hydroxide and developed by Sinovac Life Sciences (Beijing, China) for COVID-19, was one of the first vaccines to be distributed globally (Wilder-Smith and Mulholland, 2021; Wu Z. et al., 2021). PiCoVacc (Gao et al., 2020), Covaxin (BBV152) (Ahmed et al., 2022), and CoviVac (Kozlovskaya et al., 2021) are other candidate inactivated SARS-CoV-2 vaccines.
4.1.3 Subunit vaccine
Subunit vaccines show a new generation of vaccines that use pathogenic components (antigens) in parasites, bacteria, or viruses to stimulate adaptive immunity against them. Recently, protein and peptide antigens are in use worldwide (Malik et al., 2021). Characteristic subunit vaccines are built on antigens that are much safer and highly purified than whole organism-based preparations. In these vaccines, adjuvants are often included in the vaccine formulation to improve an immune response. One of the biggest disadvantages for subunit vaccine is developing adjuvants with no or minimal toxicity, as currently available adjuvants often lead to toxicity and reactogenicity (Nevagi et al., 2019). In the literature, the S1 domain and its RBD have been shown to induce much higher IgG and IgA antibody levels when immunized in mice and neutralize SARS-CoV-2 more efficiently when adjuvanted with alum. However, it is stated that Th1 response-prone adjuvants should be used for S1-based subunit COVID-19 vaccines to reduce the potential risk of increased antibody-induced infection (Wang et al., 2021). StriFK (Wu Y. et al., 2021), S-Trimer (Liang et al., 2021), ZF 2001 (Yang et al., 2021), and Novavax (Callaway and Mallapaty, 2021) are other types of subunit vaccine candidates.
4.1.4 DNA vaccines
DNA vaccines are vaccines that produce antigens by causing a protective immunological response and is able to stimulate a wide variety of immune responses, and the antigen encoded by the DNA vaccine is injected into cells by introducing an adjuvant that induces a concerted immune response (Duman et al., 2021). Inovio Pharmaceuticals has already developed experimental vaccines (INO-4700) against COVID-19 (Smith et al., 2020). The S protein is used as the antigen of all DNA vaccines currently being tested in clinical trials (Silveira et al., 2021). However, since 2016, DNA vaccines have not been released in the US (Duman et al., 2021).
4.1.5 Vector based vaccines
The viral vector acts as a delivery system, providing a means to attack the cell and insert the code for SARS-CoV-2 antigens that it does not cause illness by being chemically weakened. In this way, the body is able to be safely mount an immune response without contracting the disease (Ndwandwe and Wiysonge, 2021). Johnson & Johnson’s Janssen vaccine (Yang et al., 2022) and Sputnik V vaccines are vector based vaccines. Gam-COVID-Vac (Sputnik V), a heterologous recombinant adenovirus-based vaccine, is known to show a good safety profile in participants in phase 1/2 clinical trials and induce potent humoral and cellular immune responses (Logunov et al., 2021).
4.1.6 mRNA vaccines
Production of mRNA can be accomplished in a one-step enzymatic reaction using a capping analog, or in a two-step reaction in which closure is accomplished using a vaccine capping enzyme. Purification on a larger scale is achieved using well-established chromatographic strategies (Rosa et al., 2021). Moderna (mRNA-1273) and Pfizer-BioNTech (BNT162b2) have taken into clinical trials at an unprecedented rate in less than 1 month, obtaining clinical use authorization within 1 year, breaking the latest 4-year speed record held by the mumps vaccine (Ziqi et al., 2022), and CVnCoV, moved into Phase III clinical testing as a mRNA vaccine candidate (Verbeke et al., 2021).
4.2 Nanotechnology and COVID-19 vaccines
Nanotechnology allows the development of nanoscale systems derived from interactions between surfaces and biomolecules. The most obvious approach to nano-sized vaccines is based on VLPs, which can achieve enhanced safety (compared to all virus-based vaccines), a marked immunogenicity (relative to soluble antigens) due to their complexity, and potent and protective immune responses (Palestino et al., 2020). The role of nanotechnology in COVID-19 is crucial for designing effective nanocarriers to counter the traditional limitations of nanointervention, antiviral, and biological therapeutics (Chauhan et al., 2020). Unlike inactivated or killed virus vaccines, mRNA and other bioengineered vaccines can be quickly and inexpensively modified to match mutated antigenic epitopes (Shapiro, 2021). Due to the presence of RNA-degrading enzymes and their inability to easily pass through the negatively charged cell membrane, LNP-based delivery systems have been designed to maintain mRNA integrity and promote its intracellular uptake (Khurana et al., 2021). Moderna vaccine formulation contains lipid nanoparticle-encapsulated mRNA that encode S protein (Thanh Le et al., 2020). The use of engineered nanocarriers to control and eradicate the spread and recurrence of this pandemic has the potential to require a safe and effective vaccine strategy (Chauhan et al., 2020). It is significant to the rapid approval of two mRNA vaccines for nanotechnology’s common use in COVID-19, including protein NPs (for the delivery of protein vaccines), lipid NPs (for formulation with mRNAs), and nanobodies (as unique therapeutic antibodies) (Du et al., 2022). NPs are considered as carriers to which multiple ACE2-based peptide inhibitors are attached to their surface. It is important to design NPs vaccine carriers with greater protection, durability, immunization and greater accessibility to target cells of the COVID-19 vaccine (Jafari et al., 2022).
5 Nanomaterial-based drug delivery systems
At the beginning of the COVID-19, treatment options were quite limited. For this reason, many researchers, including clinical researchers, have made continuous efforts to develop new therapeutics and vaccines. In the initial course of the disease, antiviral agents, inflammation inhibitors, and hyperimmune immunoglobulins were used. While antiviral agents stop the progression of the disease, it has been observed that the use of both antiviral and immunomodulators gives good results in critically infected patients (Cascella et al., 2020). The main problem of antiviral agents is that they cause cell cytotoxicity. In addition, the mutagenic structure of the virus causes loss of efficacy of traditional therapeutics. However, if the virus mutates, they lose their effectiveness. Therefore, the development of new antiviral-based materials is critical. Nanotechnology offers interesting and valuable solutions in this regard. Therefore, existing approaches should be advanced based on nanotechnology (Campos et al., 2020).
Nanotechnology has wide applications in the treatment of COVID-19, and with its potential to inhibit virus-cell interaction, transcription, membrane fusion, cell internalization, translation, and viral replication as well as activating intracellular mechanisms (Mainardes and Diedrich, 2020). Due to composition, luminescence, shape, huge surface-to-volume ratio, tunable size, and the ability to reveal multiple interaction sites on the surface, inorganic NPs (INPs) such as mesoporous silica NPs (MSNPs) (Abdelhamid and Badr, 2021; Abdellatif and Alsowinea, 2021; Rasmi et al., 2021), gold NPs (AuNPs) (Miró et al., 2021), iron oxide NPs (IONPs) (Subhash and Chaudhary, 2021), silver nano-particles (AgNPs) (Douaki et al., 2020). On the other side, organic NPs (polymeric nps, lipid-based NPs, dendrimers, liposomes) are also advantageous because of the possibility of site-specific targeting of drugs, biodegradability, controlling drug release, biocompatibility, and non-toxicity (Abhyankar et al., 2021; Refaat et al., 2021; Thi et al., 2021), and nano micelles (Skwarek et al., 2021).
Biodegradable polymers approved by the FDA and the European Medicines Agency are used in the development of NPs for the delivery of new antiviral molecules. Polymeric nanoparticles are used against viral diseases as also they can be designed to achieve specific targets and inhibit virus binding to host cell receptors (Kamat et al., 2021; Pelosi et al., 2021; Li et al., 2022; Mazayen et al., 2022). It is necessary to improve the safety of antiviral drugs and overcome cellular drug resistance (Rana, 2021). Mesoporous silica NPs, which could supply an excellent platform to treat COVID-19 by preventing viral replication, have pores of adjustable size that allow molecules to dock inside and outside for co-delivery (Karaman et al., 2021; Tiamiyu et al., 2021; Xu L. D. et al., 2021).
Carbon nanotubes (CNTs) exhibit limitations in terms of toxicity for the treatment of COVID-19 (Sadighbayan et al., 2020; Jeong et al., 2021; Joshi et al., 2021). When exposed to the lungs, they activate macrophages in the lower respiratory tract, causing fibrosis and collagen formation in the lesions (Bisht et al., 2021; Galal et al., 2021; Özmen et al., 2021). Lipid-coated MSNPs containing antiviral agent (ML336) were developed as a strategy to improve antiviral circulation time and biocompatibility. In vitro results revealed a dose-dependent virus inhibition and an additional release of ML336 after cell endocytosis, while in vivo results showed that NPs showed significant antiviral activity and no toxicity (Siddiquie et al., 2020; Balkrishna et al., 2021; Duan et al., 2021).
Dendrimers with compact spherical structure and highly branched 3D structures have unique physicochemical properties such as solubility, low polydispersity, effective drug encapsulation capacity (Jana et al., 2019; Witika et al., 2020; Mazayen et al., 2022), biodegradability, and biocompatibility (Paull et al., 2020; Khaitov et al., 2021). The ability of dendrimers to form strong interactions with viruses may increase antiviral activity, making them promising systems against viral infections (Yuliani, 2021; Zhang D. et al., 2021; Zhang et al., 2022). Composed of synthetic or natural phospholipids, cholesterol, and sphingolipids, liposomes are bilayer structures used for drug delivery (Abhyankar et al., 2021; Refaat et al., 2021; Thi et al., 2021). Liposome-based carriers are used to encapsulate antivirals for the treatment of infected cells (Huang W. C. et al., 2020; Khurana et al., 2021; Pascolo, 2021; Tenchov et al., 2021; Vahedifard and Chakravarthy, 2021).
5.1 Cell-based therapy and COVID-19
Cell therapies are of great importance in the treatment of severe COVID-19 manifestations because of their personalized and regenerative functions (Shih et al., 2020). While the subacute and chronic sequelae of COVID-19 may be important data, it is vital that all research focuses on finding targeted therapies that not only reduce acute injury but also restore physiological function (Maurya et al., 2020; Shih et al., 2020; USA National Institutes of Health, 2020; Rafat et al., 2021; Vaka et al., 2022). Thanks to ACE2 (Ni et al., 2020), transmembrane protease-serine-2 (TMPRSS2), endosome-proteases-cathepsins B/L (H.-I. et al., 2020; USA National Institutes of Health, 2020) and Neuropilin 1 (NRP1) (Cantuti-Castelvetri et al., 2020; Daly et al., 2020; Abebe et al., 2021) virus can be taken up into the cell. SARS-CoV-2 selects and modifies various cellular proteins and pathways, many of which have not yet been fully clarified (Beyerstedt et al., 2021). Information from other coronaviruses has demonstrated the presence of CD147 and 78 kDa glucose/regulated/protein (Ibrahim et al., 2020; Sabirli et al., 2021) as putative alternative receptors. However, further research is being actively exploring the bulk tissue distribution of these factors correlates with viral tropism as well as illness symptoms (Abassi et al., 2020; Snyder and Johnson, 2020; South et al., 2020; Zhang et al., 2020; Gottschalk et al., 2021; Kragstrup et al., 2021).
6 Future research
Nanomedicine is a growing field that includes the application of nanotechnology to diseases; diagnose-treat-prevent (Tharayil et al., 2021; Yang, 2021). It incorporates a number of disciplines including material science, biology based fields (virology and molecular biology), and more to provide innovative disease management strategies. Many drugs are not specific to their targets, resulting in undesirable side effects and toxicity in patients (Rasmi et al., 2021; Vahedifard and Chakravarthy, 2021). Nanotechnology may allow targeted drug delivery to a specific cell population, as in the novel targeted cancer therapies. In addition, Pfizer and Moderna overcome challenges with the physical properties of the mRNA vaccines (i.e size and electrostatic charge, which hinder RNA’s ability to generate an immune response) with using particular type of NPs. Even though there are legitimate concerns about the price and safety of NPs in medicine, nanotechnology has been used valuably to increase vaccine efficiency against COVID-19 (Ruiz-Hitzky et al., 2020; Tharayil et al., 2021; Yang, 2021).
Nanotechnology provides benefits and simplifies the overall healing of pharmacological drug properties through nanosystems are used for drug encapsulation (i.e., liposomes, metallic/polymeric-NPs, and micelles). Antiviral agents for NPs can target binding. The major reason why inorganic NPs need modification is concerns about toxicity. Due to the close morphological and physicochemical properties of SARS-CoV-2 and synthetic NPs, it allows NPs to be an effective intervention method (Wang J. et al., 2020; Xie et al., 2020; Behbudi, 2021; Doagooyan, 2021; El-Megharbel et al., 2021).
6.1 Robotics applications in COVID-19
Robots can take on human-like activities and can be programmed profitably to replace some human interactions (Javaid et al., 2020; Hussain et al., 2021). They play a variety of roles in the medical field to perform specialized human treatment and surgery. Robotic technology helps to make up for the lack of doctors, it can also assist a surgeon during a complex operation and perform tasks that are risky for humans as in remote locations (Javaid et al., 2020). Certain robots are useful in managing COVID-19 patient cure in analysis (Javaid et al., 2020; Zhao et al., 2021b; Doğuç, 2021; Sarker et al., 2021; Shorten et al., 2021). For example, this technology is useful for overcoming various difficulties during the quarantine situation. Robots help maintain social distancing and monitor large populations. They are also used for packaging necessary medical equipment. Moreover, robots help incapacitated people and play an important role in their recovery process (Javaid et al., 2020; Firouzi et al., 2021; Khamis et al., 2021; Mbunge et al., 2021; Sarker et al., 2021; Shorten et al., 2021).
6.2 Tissue engineering and COVID-19
Tissue engineering (TE) is a field with a unique set of tools and technologies to develop diagnostics and treatments during the COVID-19 (Aydin et al., 2021; Shafiee et al., 2021). Tissue engineering is pioneered by the pandemic and can play an important role in preventive strategies and identifies methods that can be applied to the current COVID-19 crisis as well as future viral outbreaks (Harikrishnan and Krishnan, 2020; Tatara, 2020; Softa et al., 2021). TE covers the behavior and growth factors phenomenon of TE in vitro (Harikrishnan and Krishnan, 2020; Tatara, 2020; Aydin et al., 2021; Softa et al., 2021). This field of TE is able to support the treatment of COVID-19 patients and help combat current crises. Current TE studies report the greatest challenges faced during the COVID-19 situation, important developments in TE, and in medicine are listed in chronological order, the positive effects of TE during the COVID-19 crisis. The primary importance of this branch of science is to offer biological alternatives that can fully or partially fulfill the functions of damaged, dysfunctional organs or tissues in humans. It is particularly useful for the supply of convalescent plasma to patients during COVID-19. A donor is chosen based on a strictly confirmed case of COVID-19 transmission. The donor must confirm a negative molecular examination that does not show any symptoms (Harikrishnan and Krishnan, 2020; de Melo et al., 2021; Shafiee et al., 2021). With the contributions of talented scientists, TE’s future potential for the COVID-19 will be the realization of successful initiatives. More work needs to be done in the areas of healthcare and different methods to develop diagnostic products such as PCR kits and to implement TE in the COVID-19 (World Health Organization, 2020; Wang, 2021; Yunus et al., 2021; Zhang T. et al., 2021).
The application of TE provides extended support for the development of diagnostic products that help improve clinical processes (Tatara, 2020; Shafiee et al., 2021). Researchers use the TE animal model to describe it. In this ongoing COVID-19, TE has an important role in the application of the Bioscaffold matrix, the treatment of COVID-19 infection, the healthy functioning of the body and the examination of healing mechanisms. In the future, TE will play an important role in saving the life of a COVID-19 patient (Malavazos et al., 2020; Tatara, 2020; Bailey et al., 2021; Dorward et al., 2021; Kerch, 2021).
6.3 Antimicrobial nanotechnology for COVID-19
Antimicrobial studies have several roles during the pandemic. Primary role, agents such as azithromycin, lopinavir, and ritonavir as well as antivirals and remdesivir are being investigated in clinical trials as potential treatments for SARS-CoV-2. However, the trials did not clearly demonstrates improved clinical results compared to the standard treatment methods (Anvar et al., 2021; Erkoc and Ulucan-Karnak, 2021; Khorsandi et al., 2021). Second, antimicrobials are directly related to COVID-19, they are widely prescribed for the management possible or approved bacterial infections that can happen during long-term acceptance to intensive care. Available proof indicates that bacterial and/or fungal co-infection is low in patients and diseases with COVID-19. This is due to the lack of rapid diagnosis resulting in an increase in unnecessary antimicrobial use, which resulted the future risk of antimicrobial resistance (AMR) through the selection of Enterobacteriaceae that produces carbapenemase (Kaur et al., 2020; Kchaou et al., 2020). The potential avoidance of healthcare by patients due to self-isolation healthcare due to service changes limits the requisition but also limits the access to essential anti-microbials. Increased use of telemedicine, along with continued public interest discourses on the role of antibiotics in viral infections may reduce the use of antimicrobials and AMR (Karaman et al., 2021). In addition to this, hand hygiene can prevent the transmission of AMR as well as reduce the diseases that can lead to antibiotic use. This will likely have a significant impact on countries with limited resources and large numbers of endemic infectious diseases. After the initial global increase in COVID-19 cases, the importance of such measures is once again increasing until vaccine implementations take place. Delays in elective surgery and cancer treatment will increase the pressure, potentially resulting in increased healthcare needs and increased use of antimicrobials (Almeida et al., 2020; Johnson, 2021; Knight et al., 2021; Lai et al., 2021; Schouten et al., 2021; Khan et al., 2022).
7 Conclusion
Nanotechnology offers new solutions during fight against COVID-19 by providing better improved forms of treatment, prevention, and diagnosis. Advances in bio/nanotechnology and advanced nano/manufacturing, combined with open reporting and data sharing, lay the foundation for the rapid development of innovative technologies that will have an impact during the COVID-19 pandemic. Thanks to the increasing relationship between the COVID-19 pandemic and biotechnological methods, we should say that these biotechnological methods are used in the majority of vaccines developed for COVID-19. In addition, there are different approaches in the diagnosis and treatment of SARS-CoV-2. There still needs to be some development work done—for example, a system that concentrates aeroceles in the air and releases RNA from viruses. It is thought that the discovery of new methods with the development of technology and the use of these discovered methods for the COVID-19 will have an important share in the fight against this pandemic.
Author contributions
Conceptualization, SA, KA-C, and CU; data curation, SA, KA-C, and FC; writing—original draft, SA and KA-C; writing—review and editing, SA, KA-C, FC, and CU; visualization, SA, KA-C, and FC; supervision, All authors have read and agreed to the published version of the manuscript.
Conflict of interest
The authors declare that the research was conducted in the absence of any commercial or financial relationships that could be construed as a potential conflict of interest.
Publisher’s note
All claims expressed in this article are solely those of the authors and do not necessarily represent those of their affiliated organizations, or those of the publisher, the editors and the reviewers. Any product that may be evaluated in this article, or claim that may be made by its manufacturer, is not guaranteed or endorsed by the publisher.
References
Abassi, Z., Higazi, A. A. R., Kinaneh, S., Armaly, Z., Skorecki, K., and Heyman, S. N. (2020). ‘ACE2, COVID-19 infection, inflammation, and coagulopathy: Missing pieces in the puzzle’. Front. Physiology 11, 574753. doi:10.3389/fphys.2020.574753
Abdelhamid, H. N., and Badr, G. (2021). ‘Nanobiotechnology as a platform for the diagnosis of COVID-19: A review’. Nanotechnol. Environ. Eng. 6, 19. doi:10.1007/s41204-021-00109-0
Abdellatif, A. A. H., and Alsowinea, A. F. (2021). ‘Approved and marketed nanoparticles for disease targeting and applications in COVID-19’. Nanotechnol. Rev. 10, 1941–1977. doi:10.1515/ntrev-2021-0115
Abebe, E. C., Mengie Ayele, T., Tilahun Muche, Z., and Asmamaw Dejenie, T. (2021). ‘Neuropilin 1: A novel entry factor for sars-cov-2 infection and a potential therapeutic target’. Biol. Targets Ther. 15, 143–152. doi:10.2147/BTT.S307352
Abhyankar, M. M., Mann, B. J., Sturek, J. F., Brovero, S., Moreau, G. B., Sengar, A., et al. (2021). ‘Development of COVID-19 vaccine using a dual Toll-like receptor ligand liposome adjuvant’. npj Vaccines 6 (1), 137. doi:10.1038/s41541-021-00399-0
Abid, S. A., Ahmed Muneer, A., Al-Kadmy, I. M. S., Sattar, A. A., Beshbishy, A. M., El-Saber Batiha, G., et al. (2021). ‘Biosensors as a future diagnostic approach for COVID-19’. Life Sci. 273, 119117. doi:10.1016/j.lfs.2021.119117
Ahmed, T., Rishi, S., Irshad, S., Aggarwal, J., Happa, K., and Mansoor, S. (2022). ‘Inactivated vaccine Covaxin/BBV152: A systematic review’. Front. Immunol. 13, 863162. doi:10.3389/fimmu.2022.863162
Al Adem, K., Shanti, A., Stefanini, C., and Lee, S. (2020). ‘Inhibition of sars-cov-2 entry into host cells using small molecules’. Pharmaceuticals 13, 447. doi:10.3390/ph13120447
Al-Douri, Y., Mansoob Khan, M., Robert Jennings, J., and Abd El-Rehim, A. F. (2021). ‘Nanomaterial-based biosensors for COVID-19 detection’. Crit. Rev. Solid State Mater. Sci. 47, 955–978. doi:10.1080/10408436.2021.1989665
Al-Sayah, M. H. (2020). ‘Chemical disinfectants of COVID-19: An overview’. J. Water Health 18, 843–848. doi:10.2166/wh.2020.108
Almeida, A., Faustino, M. A. F., and Neves, M. G. P. M. S. (2020). ‘Antimicrobial photodynamic therapy in the control of COVID-19’. Antibiotics 9, 320. doi:10.3390/antibiotics9060320
Anvar, A. A., Ahari, H., and Ataee, M. (2021). ‘Antimicrobial properties of food nanopackaging: A new focus on foodborne pathogens’. Front. Microbiol. 12, 690706. doi:10.3389/fmicb.2021.690706
Ashraf, G., Aziz, A., Qaisrani, R. N., Chen, W., and Asif, M. (2021). ‘Detecting and inactivating severe acute respiratory syndrome coronavirus-2 under the auspices of electrochemistry’. Curr. Res. Chem. Biol. 1, 100001. doi:10.1016/j.crchbi.2021.100001
Aydemir, D., and Ulusu, N. N. (2020). ‘Correspondence: Angiotensin-converting enzyme 2 coated nanoparticles containing respiratory masks, chewing gums and nasal filters may be used for protection against COVID-19 infection’. Travel Med. Infect. Dis. 37, 101697. doi:10.1016/j.tmaid.2020.101697
Aydin, A., Cebi, G., Demirtas, Z. E., Erkus, H., Kucukay, A., Ok, M., et al. (2021). ‘Combating COVID-19 with tissue engineering: A review’. Emergent Mater. 4, 329–349. doi:10.1007/s42247-020-00138-6
Bahl, S., Javaid, M., Bagha, A., Singh, R., Haleem, A., Vaishya, R., et al. (2020). ‘Biosensors applications in fighting COVID-19 pandemic’. Apollo Med. 0, 0. doi:10.4103/am.am_56_20
Bailey, A. L., Dmytrenko, O., Greenberg, L., Bredemeyer, A. L., Ma, P., Liu, J., et al. (2021). ‘SARS-CoV-2 infects human engineered heart tissues and models COVID-19 myocarditis’. JACC Basic Transl. Sci. 6, 331–345. doi:10.1016/j.jacbts.2021.01.002
Balkrishna, A., Arya, V., Rohela, A., Kumar, A., Verma, R., Kumar, D., et al. (2021). ‘Nanotechnology interventions in the management of Covid-19: Prevention, diagnosis and virus-like particle vaccines’. Vaccines 9 (10), 1129. doi:10.3390/vaccines9101129
Batibay, S., Kocak Ulucakoy, R., Gunendi, Z., Fidan, I., Bozdayi, G., and Gogus, F. N. (2022). ‘AB1150 IMMUNOGENICITY AND SAFETY OF THE CORONOVAC AND BNT162b2 COVID-19 VACCINE IN PATIENTS WITH INFLAMMATORY RHEUMATIC DISEASES AND HEALTHY ADULTS: Comparison of different vaccines’. Ann. Rheumatic Dis. 81 (1), 1692. doi:10.1136/annrheumdis-2022-eular.3971
Behbudi, G. (2021). ‘Effect of silver nanoparticles disinfectant on Covid-19’, in Advances in Applied NanoBio-Technologies. Dubai, United Arab Emirates: Dorma Trading Est.
Behera, S., Rana, G., Satapathy, S., Mohanty, M., Pradhan, S., Panda, M. K., et al. (2020). ‘Biosensors in diagnosing COVID-19 and recent development’. Sensors Int. 1, 100054. doi:10.1016/j.sintl.2020.100054
Beyerstedt, S., Casaro, E. B., and Rangel, É. B. (2021). ‘COVID-19: Angiotensin-converting enzyme 2 (ACE2) expression and tissue susceptibility to SARS-CoV-2 infection’. Eur. J. Clin. Microbiol. Infect. Dis. 40, 905–919. doi:10.1007/s10096-020-04138-6
Bisht, A., Mishra, A., Bisht, H., and Tripathi, R. M. (2021). ‘Nanomaterial based biosensors for detection of viruses including SARS-CoV-2: A review’. J. Analysis Test. 5, 327–340. doi:10.1007/s41664-021-00200-0
Bonnechère, B., Sankoh, O., Samadoulougou, S., Yombi, J. C., and Kirakoya-Samadoulougou, F. (2021). ‘Surveillance of COVID-19 in Cameroon: Implications for policymakers and the healthcare system’. J. Public Health Afr. 12, 1415. doi:10.4081/JPHIA.2021.1415
Brooks, N. A., Puri, A., Garg, S., Nag, S., Corbo, J., Turabi, A. E., et al. (2021). ‘The association of coronavirus disease-19 mortality and prior bacille calmette-guerin vaccination: A robust ecological analysis using unsupervised machine learning’. Sci. Rep. 11, 774. doi:10.1038/s41598-020-80787-z
Callaway, E., and Mallapaty, S. (2021). ‘Novavax offers first evidence that COVID vaccines protect people against variants’. Nature 590, 17. doi:10.1038/d41586-021-00268-9
Campos, E. V. R., Pereira, A. E. S., de Oliveira, J. L., Carvalho, L. B., Guilger-Casagrande, M., de Lima, R., et al. (2020). ‘How can nanotechnology help to combat COVID-19? Opportunities and urgent need’. J. Nanobiotechnology 18, 125. doi:10.1186/s12951-020-00685-4
Cantuti-Castelvetri, L., Ojha, R., Pedro, L. D., Djannatian, M., Franz, J., Kuivanen, S., et al. (2020). ‘Neuropilin-1 facilitates SARS-CoV-2 cell entry and infectivity’. Science 370, 856–860. doi:10.1126/science.abd2985
Cascella, M., Rajnik, M., Aleem, A., Dulebohn, S. C., and Di Napoli, R. (2020). Features, evaluation and treatment coronavirus (COVID-19) - StatPearls - NCBI bookshelf. Statpearls-NCBI bookshelf.
Centers for Disease Control and Prevention (2022). Overview of COVID-19 vaccines. Available at: https://www.cdc.gov/coronavirus/2019-ncov/vaccines/different-vaccines/overview-COVID-19-vaccines.html (Accessed [ October 24, 2022).
Chao, J. Y., Derespina, K. R., Herold, B. C., Goldman, D. L., Aldrich, M., Weingarten, J., et al. (2020). ‘Clinical characteristics and outcomes of hospitalized and critically ill children and adolescents with coronavirus disease 2019 at a tertiary care medical center in New York city’. J. Pediatr. 223, 14–19.e2. doi:10.1016/j.jpeds.2020.05.006
Chauhan, G., Madou, M. J., Kalra, S., Chopra, V., Ghosh, D., and Martinez-Chapa, S. O. (2020). ‘Nanotechnology for COVID-19: Therapeutics and vaccine research’. ACS Nano 14, 7760–7782. doi:10.1021/acsnano.0c04006
Chen, J. M. (2022). ‘Should the world collaborate imminently to develop neglected live-attenuated vaccines for COVID-19?’. J. Med. Virology 94, 82–87. doi:10.1002/jmv.27335
Chen, Z., Du, R., Galvan Achi, J. M., Rong, L., and Cui, Q. (2021). ‘SARS-CoV-2 cell entry and targeted antiviral development’. Acta Pharm. Sin. B 11, 3879–3888. doi:10.1016/j.apsb.2021.05.007
Chintagunta, A. D., M, S. K., Nalluru, S., and N. S., S. K. (2021). ‘Nanotechnology: An emerging approach to combat COVID-19’. Emergent Mater. 4, 119–130. doi:10.1007/s42247-021-00178-6
Choi, J. R. (2020). ‘Development of point-of-care biosensors for COVID-19’. Front. Chem. 8, 517. doi:10.3389/fchem.2020.00517
Choong, Y. Y. C., Tan, H. W., Patel, D. C., Choong, W. T. N., Chen, C. H., Low, H. Y., et al. (2020). ‘The global rise of 3D printing during the COVID-19 pandemic’. Nat. Rev. Mater. 5, 637–639. doi:10.1038/s41578-020-00234-3
Chowdhury, N. K., Deepika, , Choudhury, R., Sonawane, G. A., Mavinamar, S., Lyu, X., et al. (2021). ‘Nanoparticles as an effective drug delivery system in COVID-19’. Biomed. Pharmacother. 143, 112162. doi:10.1016/j.biopha.2021.112162
Coperchini, F., Chiovato, L., Croce, L., Magri, F., and Rotondi, M. (2020). ‘The cytokine storm in COVID-19: An overview of the involvement of the chemokine/chemokine-receptor system’. Cytokine Growth Factor Rev. 53, 25–32. doi:10.1016/j.cytogfr.2020.05.003
Corman, V. M., Landt, O., Kaiser, M., Molenkamp, R., Meijer, A., Chu, D. K., et al. (2020). ‘Detection of 2019 novel coronavirus (2019-nCoV) by real-time RT-PCR’. Eurosurveillance 25, 2000045. doi:10.2807/1560-7917.ES.2020.25.3.2000045
COVID-19 Treatment Guidelines Panel (2022). Coronavirus disease 2019 (COVID-19) treatment guidelines. National Institutes of health. Available at: https://www.covid19treatmentguidelines.nih.gov/(Accessed October 24, 2022).
Crawford, F. W., Jones, S. A., Cartter, M., Dean, S. G., Warren, J. L., Li, Z. R., et al. (2022). ‘Impact of close interpersonal contact on COVID-19 incidence: Evidence from 1 year of mobile device data’. Sci. Adv. 8, eabi5499. doi:10.1126/sciadv.abi5499
Cui, F., and Zhou, H. S. (2020). ‘Diagnostic methods and potential portable biosensors for coronavirus disease 2019’. Biosens. Bioelectron. 165, 112349. doi:10.1016/j.bios.2020.112349
Daly, J. L., Simonetti, B., Klein, K., Chen, K. E., Williamson, M. K., Anton-Plagaro, C., et al. (2020). ‘Neuropilin-1 is a host factor for SARS-CoV-2 infection’. Science 370, 861–865. doi:10.1126/science.abd3072
de Melo, B. A. G., Benincasa, J. C., Cruz, E. M., Maricato, J. T., and Porcionatto, M. A. (2021). ‘3D culture models to study SARS-CoV-2 infectivity and antiviral candidates: From spheroids to bioprinting’. Biomed. J. 44, 31–42. doi:10.1016/j.bj.2020.11.009
Deng, W., Sun, Y., Yao, X., Subramanian, K., Ling, C., Wang, H., et al. (2022). ‘Masks for COVID-19’. Adv. Sci. 9, 2102189. doi:10.1002/advs.202102189
Devi, S., Sharma, N., Ahmed, T., Huma, Z. I., Kour, S., Sahoo, B., et al. (2021). ‘Aptamer-based diagnostic and therapeutic approaches in animals: Current potential and challenges’. Saudi J. Biol. Sci. 28, 5081–5093. doi:10.1016/j.sjbs.2021.05.031
Dhama, K., Patel, S. K., Kumar, R., Masand, R., Rana, J., Yatoo, M. I., et al. (2021). ‘The role of disinfectants and sanitizers during COVID-19 pandemic: Advantages and deleterious effects on humans and the environment’. Environ. Sci. Pollut. Res. 28, 34211–34228. doi:10.1007/s11356-021-14429-w
Dinnes, J., Deeks, J. J., Berhane, S., Taylor, M., Adriano, A., Davenport, C., et al. (2021). ‘Rapid, point-of-care antigen tests for diagnosis of SARS-CoV-2 infection’. Cochrane Database Syst. Rev. 2022. doi:10.1002/14651858.CD013705.pub2
Doagooyan, M. (2021). ‘COVID-19 therapy approaches and vaccine development: The role of nanotechnology’. Nanomedicine J. doi:10.22038/nmj.2021.08.001
Doğuç, Ö. (2021). ‘Robotic process automation (RPA) applications in COVID-19’. Contributions Manag. Sci., 233–247. doi:10.1007/978-3-030-72288-3_16
Dorward, D. A., Russell, C. D., Um, I. H., Elshani, M., Armstrong, S. D., Penrice-Randal, R., et al. (2021). ‘Tissue-specific immunopathology in fatal COVID-19’. Am. J. Respir. Crit. Care Med. 203, 192–201. doi:10.1164/rccm.202008-3265OC
Douaki, A., Demelash Abera, B., Cantarella, G., Shkodra, B., Mushtaq, A., Ibba, P., et al. (2020). ‘Flexible screen printed aptasensor for rapid detection of furaneol: A comparison of CNTs and AgNPs effect on aptasensor performance’. Nanomaterials 10 (6), 1167. doi:10.3390/nano10061167
Dowlatshahi, S., and Abdekhodaie, M. J. (2021). ‘Electrochemical prostate-specific antigen biosensors based on electroconductive nanomaterials and polymers’. Clin. Chim. Acta 516, 111–135. doi:10.1016/j.cca.2021.01.018
Du, L., Yang, Y., Zhang, X., and Li, F. (2022). ‘Recent advances in nanotechnology-based COVID-19 vaccines and therapeutic antibodies’. Nanoscale 14, 1054–1074. doi:10.1039/d1nr03831a
Duan, Y., Wang, S., Zhang, Q., Gao, W., and Zhang, L. (2021). ‘Nanoparticle approaches against SARS-CoV-2 infection’. Curr. Opin. Solid State Mater. Sci. 25, 100964. doi:10.1016/j.cossms.2021.100964
Duman, N., Alzaidi, Z., Aynekin, B., Taskin, D., Demirors, B., Yildirim, A., et al. (2021). ‘COVID-19 vaccine candidates and vaccine development platforms available worldwide’. J. Pharm. Analysis 11, 675–682. doi:10.1016/j.jpha.2021.09.004
Dutta, N. K., Mazumdar, K., and Gordy, J. T. (2020). ‘The nucleocapsid protein of SARS–CoV-2: A target for vaccine development’. J. Virology 94, 006477-e720. doi:10.1128/jvi.00647-20
E, S, Innovation CenterPart Lastic Center of Applied Science and TechnologyMashhad, Iran (2021). ‘Electrochemical biosensors for COVID-19’. Austin J. Infect. Dis. 8. doi:10.26420/austinjinfectdis.2021.1051
El-Megharbel, S. M., Alsawat, M., Al-Salmi, F. A., and Hamza, R. Z. (2021). Utilizing of (zinc oxide nano-spray) for disinfection against “SARS-CoV-2” and testing its biological effectiveness on some biochemical parameters during (COVID-19 pandemic)” ZnO nanoparticles have antiviral activity against (SARS-CoV-2)”. Coatings 11 (4), 388. doi:10.3390/coatings11040388
Elias, C., Sekri, A., Leblanc, P., Cucherat, M., and Vanhems, P. (2021). ‘The incubation period of COVID-19: A meta-analysis’. Int. J. Infect. Dis. 104, 708–710. doi:10.1016/j.ijid.2021.01.069
Erkoc, P., and Ulucan-Karnak, F. (2021). ‘Nanotechnology-Based antimicrobial and antiviral surface coating strategies’. Prosthesis 3 (1), 25–52. doi:10.3390/prosthesis3010005
Excler, J. L., Saville, M., Berkley, S., and Kim, J. H. (2021). ‘Vaccine development for emerging infectious diseases’. Nat. Med. 27, 591–600. doi:10.1038/s41591-021-01301-0
Fani, M., Zandi, M., Soltani, S., and Abbasi, S. (2021). ‘Future developments in biosensors for field-ready SARS-CoV-2 virus diagnostics’. Biotechnol. Appl. Biochem. 68, 695–699. doi:10.1002/bab.2033
Farshbafnadi, M., Kamali Zonouzi, S., Sabahi, M., Dolatshahi, M., and Aarabi, M. H. (2021). ‘Aging & COVID-19 susceptibility, disease severity, and clinical outcomes: The role of entangled risk factors’. Exp. Gerontol. 154, 111507. doi:10.1016/j.exger.2021.111507
Fathi-Hafshejani, P., Azam, N., Wang, L., Kuroda, M. A., Hamilton, M. C., Hasim, S., et al. (2021). ‘Two-Dimensional-Material-Based field-effect transistor biosensor for detecting COVID-19 virus (SARS-CoV-2)’. ACS Nano 15, 11461–11469. doi:10.1021/acsnano.1c01188
Firouzi, F., Farahani, B., Daneshmand, M., Grise, K., Song, J., Saracco, R., et al. (2021). ‘Harnessing the power of smart and connected health to tackle COVID-19: IoT, AI, robotics, and blockchain for a better world’. IEEE Internet Things J. 8, 12826–12846. doi:10.1109/JIOT.2021.3073904
Gage, A., Brunson, K., Morris, K., Wallen, S. L., Dhau, J., Gohel, H., et al. (2021). ‘Perspectives of manipulative and high-performance nanosystems to manage consequences of emerging new severe acute respiratory syndrome coronavirus 2 variants’. Front. Nanotechnol. 3. doi:10.3389/fnano.2021.700888
Galal, A., Ahmed, Y. M., Ahmed, M. H. M., and Atta, N. F. (2021). ‘Electrochemistry and determination of an antiviral drug at ionic liquids crystals-carbon nanotubes modified glassy carbon electrode’. J. Electrochem. Soc. 168, 116512. doi:10.1149/1945-7111/ac39d6
Gao, Q., Bao, L., Mao, H., Wang, L., Xu, K., Yang, M., et al. (2020). ‘Development of an inactivated vaccine candidate for SARS-CoV-2’. Science 369, 77–81. doi:10.1126/science.abc1932
Gao, T., Gao, Y., Liu, X., Nie, Z., Sun, H., Lin, K., et al. (2021). ‘Identification and functional analysis of the SARS-COV-2 nucleocapsid protein’. BMC Microbiol. 21, 58. doi:10.1186/s12866-021-02107-3
Ge, Z. yu, Yang, L. m., Xia, J. j., Fu, X. h., and Zhang, Y. z. (2020). ‘Possible aerosol transmission of COVID-19 and special precautions in dentistry’. J. Zhejiang Univ. Sci. B 21, 361–368. doi:10.1631/jzus.B2010010
Gottschalk, G., Knox, K., and Roy, A. (2021). ‘ACE2: At the crossroad of COVID-19 and lung cancer’. Gene Rep. 23, 101077. doi:10.1016/j.genrep.2021.101077
Gupta, R., Sagar, P., Priyadarshi, N., Kaul, S., Sandhir, R., Rishi, V., et al. (2020). ‘Nanotechnology-Based approaches for the detection of SARS-CoV-2’. Front. Nanotechnol. 2. doi:10.3389/fnano.2020.589832
Haque, A., and Pant, A. B. (2020). ‘Efforts at COVID-19 vaccine development: Challenges and successes’. Vaccines 8 (4), 739. doi:10.3390/vaccines8040739
Harikrishnan, P., and Krishnan, A. (2020). "Tissue engineering strategies in Covid-19 research," in Trends in biomaterials and artificial organs. India: Trends in Biomaterials and Artificial Organs, 34.
Harrison, A. G., Lin, T., and Wang, P. (2020). ‘Mechanisms of SARS-CoV-2 transmission and pathogenesis’. Trends Immunol. 41, 1100–1115. doi:10.1016/j.it.2020.10.004
Huang, C., Wang, Y., Li, X., Ren, L., Zhao, J., Hu, Y., et al. (2020). ‘Clinical features of patients infected with 2019 novel coronavirus in Wuhan, China’. Lancet 395, 497–506. doi:10.1016/S0140-6736(20)30183-5
Huang, W. C., Zhou, S., He, X., Chiem, K., Mabrouk, M. T., Nissly, R. H., et al. (2020). ‘SARS-CoV-2 RBD neutralizing antibody induction is enhanced by particulate vaccination’. Adv. Mater. 32, 2005637. doi:10.1002/adma.202005637
Huang, X., Zhu, Y., and Kianfar, E. (2021). ‘Nano Biosensors: Properties, applications and electrochemical techniques’. J. Mater. Res. Technol. 12, 1649–1672. doi:10.1016/j.jmrt.2021.03.048
Hussain, K., Wang, X., Omar, Z., Elnour, M., Ming, Y., et al. (2021). “‘Robotics and artificial intelligence applications in manage and control of COVID-19 pandemic’,” in 2021 International Conference on Computer, Control and Robotics (IEEE) (ICCCR). doi:10.1109/ICCCR49711.2021.9349386
Ibrahim, I. M., Abdelmalek, D. H., Elshahat, M. E., and Elfiky, A. A. (2020). ‘COVID-19 spike-host cell receptor GRP78 binding site prediction’. J. Infect. 80, 554–562. doi:10.1016/j.jinf.2020.02.026
Izcovich, A., Ragusa, M. A., Tortosa, F., Lavena Marzio, M. A., Agnoletti, C., Bengolea, A., et al. (2020). ‘Prognostic factors for severity and mortality in patients infected with COVID-19: A systematic review’. PLoS ONE 15, e0241955. doi:10.1371/journal.pone.0241955
Jafari, A., Danesh Pouya, F., Niknam, Z., Abdollahpour‑Alitappeh, M., Rezaei-Tavirani, M., and Rasmi, Y. (2022). ‘Current advances and challenges in COVID-19 vaccine development: From conventional vaccines to next-generation vaccine platforms’. Mol. Biol. Rep. 49, 4943–4957. doi:10.1007/s11033-022-07132-7
Jain, S., Singh, S. P., Mayya, C., Majumdar, S., and Bhatia, D. (2020). DNA aptamers in COVID-19 research’. Curr. Sci. 119, 1489. doi:10.18520/cs/v119/i9/1489-1498
Jana, S. S., Narula, P., Chugh, A., and Kulshreshtha, R. (2019). Efficient delivery of anti-miR-210 using Tachyplesin, a cell penetrating peptide, for glioblastoma treatment’. Int. J. Pharm. 572, 118789. doi:10.1016/j.ijpharm.2019.118789
Javaid, M., Haleem, A., Vaish, A., Vaishya, R., and Iyengar, K. P. (2020). Robotics applications in Covid-19: A review’. J. Industrial Integration Manag. 05, 441–451. doi:10.1142/S2424862220300033
Jayaweera, M., Perera, H., Gunawardana, B., and Manatunge, J. (2020). Transmission of COVID-19 virus by droplets and aerosols: A critical review on the unresolved dichotomy’‘. Environ. Res. 188, 109819. doi:10.1016/j.envres.2020.109819
Jeong, D. W., Kim, K. H., Kim, B. S., and Byun, Y. T. (2021). ‘Characteristics of highly sensitive and selective nitric oxide gas sensors using defect-functionalized single-walled carbon nanotubes at room temperature’. Appl. Surf. Sci. 550, 149250. doi:10.1016/j.apsusc.2021.149250
Johnson, T. (2021). ‘A trade-off: Antimicrobial resistance and COVID-19’. Bioethics 35, 947–955. doi:10.1111/bioe.12928
Joshi, P., Mishra, R., and Narayan, R. J. (2021). ‘Biosensing applications of carbon-based materials’. Curr. Opin. Biomed. Eng. 18, 100274. doi:10.1016/j.cobme.2021.100274
Kamat, S., Kumari, M., and Jayabaskaran, C. (2021). ‘Nano-engineered tools in the diagnosis, therapeutics, prevention, and mitigation of SARS-CoV-2’. J. Control. Release 338, 813–836. doi:10.1016/j.jconrel.2021.08.046
Karaman, D. Ş., Pamukcu, A., Karakaplan, M. B., Kocaoglu, O., and Rosenholm, J. M. (2021). ‘Recent advances in the use of mesoporous silica nanoparticles for the diagnosis of bacterial infections’. Int. J. Nanomedicine 16, 6575–6591. doi:10.2147/IJN.S273062
Kargozar, S., and Mozafari, M. (2018). ‘Nanotechnology and Nanomedicine: Start small, think big’. Mater. Today Proc. 5, 15492–15500. doi:10.1016/j.matpr.2018.04.155
Kashte, S., Gulbake, A., El-Amin III, S. F., and Gupta, A. (2021). ‘COVID-19 vaccines: Rapid development, implications, challenges and future prospects’. Hum. Cell 34, 711–733. doi:10.1007/s13577-021-00512-4
Kaur, M., Devi, G., Nagpal, M., Singh, M., Dhingra, G. A., and Aggarwal, G. (2020). ‘Antiviral essential oils incorporated in nanocarriers: Strategy for prevention from COVID-19 and future infectious pandemics’. Pharm. Nanotechnol. 8, 437–451. doi:10.2174/2211738508666201016151850
Kchaou, M., Abuhasel, K., Khadr, M., Hosni, F., and Alquraish, M. (2020). ‘Surface disinfection to protect against microorganisms: Overview of traditional methods and issues of emergent nanotechnologies’. Appl. Sci. 10 (17), 6040. doi:10.3390/app10176040
Kerch, G. (2021). ‘Tissue integrity and COVID-19’. Encyclopedia 1, 206–219. doi:10.3390/encyclopedia1010020
Khade, S. M., Yabaji, S. M., and Srivastava, J. (2021). ‘An update on COVID-19: SARS-CoV-2 life cycle, immunopathology, and BCG vaccination’. Prep. Biochem. Biotechnol. 51, 650–658. doi:10.1080/10826068.2020.1848869
Khaitov, M., Nikonova, A., Shilovskiy, I., Kozhikhova, K., Kofiadi, I., Vishnyakova, L., et al. (2021). ‘Silencing of SARS-CoV-2 with modified siRNA-peptide dendrimer formulation’. Allergy Eur. J. Allergy Clin. Immunol. 76, 2840–2854. doi:10.1111/all.14850
Khamis, A., Meng, J., Wang, J., Azar, A. T., Prestes, E., Li, H., et al. (2021). ‘Robotics and intelligent systems against a pandemic’. Acta Polytech. Hung. 18, 13–35. doi:10.12700/APH.18.5.2021.5.3
Khan, S., Hasan, S. S., Bond, S. E., Conway, B. R., and Aldeyab, M. A. (2022). ‘Antimicrobial consumption in patients with COVID-19: A systematic review and meta-analysis’. Expert Rev. Anti-Infective Ther. 20, 749–772. doi:10.1080/14787210.2022.2011719
Khera, D., Khasbage, S., and Singh, S. (2021). ‘Does bacille calmette-guérin vaccination provides protection against COVID-19: A systematic review and meta-analysis’. Indian J. Community Med. 46, 592. doi:10.4103/ijcm.IJCM_952_20
Khorsandi, K., Fekrazad, S., Vahdatinia, F., Farmany, A., and Fekrazad, R. (2021). ‘Nano antiviral photodynamic therapy: A probable biophysicochemical management modality in SARS-CoV-2’. Expert Opin. Drug Deliv. 18, 265–272. doi:10.1080/17425247.2021.1829591
Khurana, A., Allawadhi, P., Khurana, I., Allwadhi, S., Weiskirchen, R., Banothu, A. K., et al. (2021). ‘Role of nanotechnology behind the success of mRNA vaccines for COVID-19’. Nano Today 38, 101142. doi:10.1016/j.nantod.2021.101142
Kim, H. Y., Lee, J. H., Kim, M. J., Park, S. C., Choi, M., Lee, W., et al. (2021). ‘Development of a SARS-CoV-2-specific biosensor for antigen detection using scFv-Fc fusion proteins’. Biosens. Bioelectron. 175, 112868. doi:10.1016/j.bios.2020.112868
Knight, G. M., Glover, R. E., McQuaid, C. F., Olaru, I. D., Gallandat, K., Leclerc, Q., et al. (2021). ‘Antimicrobial resistance and Covid-19: Intersections and implications’. eLife 10, e64139. doi:10.7554/eLife.64139
Kozlovskaya, L. I., Piniaeva, A. N., Ignatyev, G. M., Gordeychuk, I. V., Volok, V. P., Rogova, Y. V., et al. (2021). ‘Long-term humoral immunogenicity, safety and protective efficacy of inactivated vaccine against COVID-19 (CoviVac) in preclinical studies’. Emerg. Microbes Infect. 10, 1790–1806. doi:10.1080/22221751.2021.1971569
Kragstrup, T. W., Singh, H. S., Grundberg, I., Nielsen, A. L. L., Rivellese, F., Mehta, A., et al. (2021). ‘Plasma ACE2 predicts outcome of COVID-19 in hospitalized patients’. PLoS ONE 16, e0252799. doi:10.1371/journal.pone.0252799
Kudr, J., Michalek, P., Ilieva, L., Adam, V., and Zitka, O. (2021). ‘COVID-19: A challenge for electrochemical biosensors’. TrAC - Trends Anal. Chem. 136, 116192. doi:10.1016/j.trac.2021.116192
Kutter, J. S., Spronken, M. I., Fraaij, P. L., Fouchier, R. A., and Herfst, S. (2018). ‘Transmission routes of respiratory viruses among humans’. Curr. Opin. Virology 28, 142–151. doi:10.1016/j.coviro.2018.01.001
Lai, C. C., Chen, S. Y., Ko, W. C., and Hsueh, P. R. (2021). ‘Increased antimicrobial resistance during the COVID-19 pandemic’. Int. J. Antimicrob. Agents 57, 106324. doi:10.1016/j.ijantimicag.2021.106324
Lan, J. (2022). ‘Overview of application of nanomaterials in medical domain’. Contrast Media & Mol. Imaging 2022, 1–5. doi:10.1155/2022/3507383
Latiano, A., Tavano, F., Panza, A., Palmieri, O., Niro, G. A., Andriulli, N., et al. (2021). ‘False-positive results of SARS-CoV-2 IgM/IgG antibody tests in sera stored before the 2020 pandemic in Italy’. Int. J. Infect. Dis. 104, 159–163. doi:10.1016/j.ijid.2020.12.067
Lee, A., Scott, M. K. D., Wimmers, F., Arunachalam, P. S., Luo, W., Fox, C. B., et al. (2022). ‘A molecular atlas of innate immunity to adjuvanted and live attenuated vaccines, in mice’. Nat. Commun. 13, 549. doi:10.1038/s41467-022-28197-9
Leïchlé, T., Nicu, L., and Alava, T. (2020). ‘MEMS biosensors and COVID-19: Missed opportunity’. ACS Sensors 5, 3297–3305. doi:10.1021/acssensors.0c01463
Li, M., Li, Y., Li, S., Jia, L., Wang, H., Li, M., et al. (2022). ‘The nano delivery systems and applications of mRNA’. Eur. J. Med. Chem. 227, 113910. doi:10.1016/j.ejmech.2021.113910
Liang, J. G., Su, D., Song, T. Z., Zeng, Y., Huang, W., Wu, J., et al. (2021). ‘S-Trimer, a COVID-19 subunit vaccine candidate, induces protective immunity in nonhuman primates’. Nat. Commun. 12, 1346. doi:10.1038/s41467-021-21634-1
Logunov, D. Y., Dolzhikova, I. V., Shcheblyakov, D. V., Tukhvatulin, A. I., Zubkova, O. V., Dzharullaeva, A. S., et al. (2021). ‘Safety and efficacy of an rAd26 and rAd5 vector-based heterologous prime-boost COVID-19 vaccine: An interim analysis of a randomised controlled phase 3 trial in Russia’. Lancet 397, 671–681. doi:10.1016/S0140-6736(21)00234-8
Lytras, S., Xia, W., Hughes, J., Jiang, X., and Robertson, D. L. (2021). ‘The animal origin of SARS-CoV-2’. Science 373, 968–970. doi:10.1126/science.abh0117
Maddali, H., Miles, C. E., Kohn, J., and O'Carroll, D. M. (2021). ‘Optical biosensors for virus detection: Prospects for SARS-CoV-2/COVID-19’. ChemBioChem 22, 1176–1189. doi:10.1002/cbic.202000744
Maheshwari, V., Mahmood, M. R., Sravanthi, S., Arivazhagan, N., ParimalaGandhi, A., Srihari, K., et al. (2021). ‘Nanotechnology-Based sensitive biosensors for COVID-19 prediction using fuzzy logic control’. J. Nanomater. 2021, 1–8. doi:10.1155/2021/3383146
Mahmoudinobar, F., Britton, D., and Montclare, J. K. (2021). ‘Protein-based lateral flow assays for COVID-19 detection’. Protein Eng. Des. Sel. 34, gzab010. doi:10.1093/protein/gzab010
Mainardes, R. M., and Diedrich, C. (2020). ‘The potential role of nanomedicine on COVID-19 therapeutics’. Ther. Deliv. 11 (7), 411–414. doi:10.4155/tde-2020-0069
Malavazos, A. E., Corsi Romanelli, M. M., Bandera, F., and Iacobellis, G. (2020). ‘Targeting the adipose tissue in COVID-19’. Obesity 28, 1178–1179. doi:10.1002/oby.22844
Malik, J. A., Mulla, A. H., Farooqi, T., Hyder Pottoo, F., Anwar, S., Rengasamy, K. R. R., et al. (2021). ‘Targets and strategies for vaccine development against SARS-CoV-2’. Biomed. Pharmacother. 137, 111254. doi:10.1016/j.biopha.2021.111254
Mallakpour, S., Behranvand, V., and Hussain, C. M. (2022). ‘Worldwide fight against COVID-19 using nanotechnology, polymer science, and 3D printing technology’. Polym. Bull., 1–19. doi:10.1007/s00289-021-04006-z
Maurya, C. K., Misra, R., Sharma, P., Singh, N., Awasthi, H., Agrawal, R., et al. (2020). ‘Novel stem cells and nucleic acid-based vaccine trials against viral outbreak: A systematic evaluation during COVID-2019 pandemic’. Indian J. Clin. Biochem. 35, 397–409. doi:10.1007/s12291-020-00907-4
Mazayen, Z. M., Ghoneim, A. M., Elbatanony, R. S., Basalious, E. B., and Bendas, E. R. (2022). ‘Pharmaceutical nanotechnology: From the bench to the market’. Future J. Pharm. Sci. 8, 12. doi:10.1186/s43094-022-00400-0
Mbunge, E., Akinnuwesi, B., Fashoto, S. G., Metfula, A. S., and Mashwama, P. (2021). ‘A critical review of emerging technologies for tackling COVID-19 pandemic’. Hum. Behav. Emerg. Technol. 3 (1), 25–39. doi:10.1002/hbe2.237
Medhi, R., Srinoi, P., Ngo, N., Tran, H. V., and Lee, T. R. (2020). ‘Nanoparticle-Based strategies to combat COVID-19’. ACS Appl. Nano Mater. 3, 8557–8580. doi:10.1021/acsanm.0c01978
Mekonnen, D., Mengist, H. M., and Jin, T. (2022). ‘SARS-CoV-2 subunit vaccine adjuvants and their signaling pathways’. Expert Rev. Vaccines 21, 69–81. doi:10.1080/14760584.2021.1991794
Miró, Ò., Llorens, P., Jiménez, S., Piñera, P., Burillo-Putze, G., Martín, A., et al. (2021). ‘Frequency, risk factors, clinical characteristics, and outcomes of spontaneous pneumothorax in patients with coronavirus disease 2019: A case-control, emergency medicine-based multicenter study’. Chest 159 (3), 1241–1255. doi:10.1016/j.chest.2020.11.013
Mlcochova, P., Collier, D., Ritchie, A., Assennato, S. M., Hosmillo, M., Goel, N., et al. (2020). ‘Combined point of care SARS-CoV-2 nucleic acid and antibody testing in suspected moderate to severe COVID-19 disease’. medRxiv 1. doi:10.1101/2020.06.16.20133157
Moabelo, K. L., Martin, D. R., Fadaka, A. O., Sibuyi, N. R. S., Meyer, M., and Madiehe, A. M. (2021). ‘Nanotechnology-based strategies for effective and rapid detection of sars-cov-2’. Materials 14, 7851. doi:10.3390/ma14247851
Mobed, A., and Sepehri Shafigh, E. (2021). ‘Biosensors promising bio-device for pandemic screening “COVID-19 ’. Microchem. J. 164, 106094. doi:10.1016/j.microc.2021.106094
Mok, D. Z. L., and Chan, K. R. (2020). ‘The effects of pre-existing antibodies on live-attenuated viral vaccines’. Viruses 12, 520. doi:10.3390/v12050520
More, P. (2021). ‘Technological tools and Biosensors for detection and diagnosis of COVID-19’. Res. J. Biotechnol. 16, 163–170. doi:10.25303/1610rjbt163170
Murugan, D., Bhatia, H., Sai, V. V. R., and Satija, J. (2020). ‘P-FAB: A fiber-optic biosensor device for rapid detection of COVID-19’. Trans. Indian Natl. Acad. Eng. 5, 211–215. doi:10.1007/s41403-020-00122-w
Nag, P., Sadani, K., and Mukherji, S. (2020). ‘Optical fiber sensors for rapid screening of COVID-19’. Trans. Indian Natl. Acad. Eng. 5, 233–236. doi:10.1007/s41403-020-00128-4
Narita, F., Wang, Z., Kurita, H., Li, Z., Shi, Y., Jia, Y., et al. (2021). ‘A review of piezoelectric and magnetostrictive biosensor materials for detection of COVID-19 and other viruses’. Adv. Mater. 33, 2005448. doi:10.1002/adma.202005448
Nasrollahzadeh, M., Sajadi, S. M., Sajjadi, M., and Issaabadi, Z. (2019). ‘An introduction to nanotechnology’. Interface Sci. Technol., 1–27. doi:10.1016/B978-0-12-813586-0.00001-8
National Health Service (2022). Coronavirus (COVID-19) vaccine. Available at: https://www.nhs.uk/conditions/coronavirus-covid-19/coronavirus-vaccination/coronavirus-vaccine/(Accessed October 24, 2022).
Ndwandwe, D., and Wiysonge, C. S. (2021). ‘COVID-19 vaccines’. Curr. Opin. Immunol. 71, 111–116. doi:10.1016/j.coi.2021.07.003
Nevagi, R. J., Skwarczynski, M., and Toth, I. (2019). ‘Polymers for subunit vaccine delivery’. Eur. Polym. J. 114, 397–410. doi:10.1016/j.eurpolymj.2019.03.009
Ni, W., Yang, X., Yang, D., Bao, J., Li, R., Xiao, Y., et al. (2020). ‘Role of angiotensin-converting enzyme 2 (ACE2) in COVID-19’. Crit. Care 24, 422. doi:10.1186/s13054-020-03120-0
Nilsson, A., Lindgren, J., and Eriksson Karlström, A. (2017). ‘Intramolecular thioether crosslinking to increase the proteolytic stability of affibody molecules’. ChemBioChem 18, 2056–2062. doi:10.1002/cbic.201700350
Ochani, R. K., Asad, A., Yasmin, F., Shaikh, S., Khalid, H., Batra, S., et al. (2021). ‘Covid-19 pandemic: From origins to outcomes. A comprehensive review of viral pathogenesis, clinical manifestations, diagnostic evaluation, and management’. Infezioni Med. 29 (1), 20–36.
Özmen, E. N., Kartal, E., Turan, M. B., Yazicioglu, A., Niazi, J. H., and Qureshi, A. (2021). ‘Graphene and carbon nanotubes interfaced electrochemical nanobiosensors for the detection of SARS-CoV-2 (COVID-19) and other respiratory viral infections: A review’. Mater. Sci. Eng. C 129, 112356. doi:10.1016/j.msec.2021.112356
Ozturk, A., Bozok, T., and Simsek Bozok, T. (2021). ‘Evaluation of rapid antibody test and chest computed tomography results of COVID-19 patients: A retrospective study’. J. Med. Virology 93, 6582–6587. doi:10.1002/jmv.27209
Palestino, G., Garcia-Silva, I., Gonzalez-Ortega, O., and Rosales-Mendoza, S. (2020). ‘Can nanotechnology help in the fight against COVID-19?’. Expert Rev. Anti-Infective Ther. 18, 849–864. doi:10.1080/14787210.2020.1776115
Palmieri, V., De Maio, F., De Spirito, M., and Papi, M. (2021). ‘Face masks and nanotechnology: Keep the blue side up’. Nano Today 37, 101077. doi:10.1016/j.nantod.2021.101077
Pang, S. N., Lin, Y. L., Yu, K. J., Chiou, Y. E., Leung, W. H., and Weng, W. H. (2021). ‘An effective sars-cov-2 electrochemical biosensor with modifiable dual probes using a modified screen-printed carbon electrode’. Micromachines 12, 1171. doi:10.3390/mi12101171
Pascolo, S. (2021). ‘Synthetic messenger rna-based vaccines: From scorn to hype’. Viruses 13, 270. doi:10.3390/v13020270
Paull, J. R. A., Castellarnau, A., Luscombe, C. A., Fairley, J. K., and Heery, P. (2020) ‘Astodrimer sodium, dendrimer antiviral, inhibits replication of SARS-CoV-2 in vitro’. Antivir. Res. 191, 105089. doi:10.1101/2020.08.20.260190
Pelosi, C., Duce, C., Wurm, F. R., and Tine, M. R. (2021). ‘Effect of polymer hydrophilicity and molar mass on the properties of the protein in protein-polymer conjugates: The case of PPEylated myoglobin’. Biomacromolecules 22, 1932–1943. doi:10.1021/acs.biomac.1c00058
Pérez-García, F., Romanyk, J., Moya Gutierrez, H., Labrador Ballestero, A., Perez Ranz, I., Gonzalez Arroyo, J., et al. (2021). ‘Comparative evaluation of Panbio and SD Biosensor antigen rapid diagnostic tests for COVID-19 diagnosis’. J. Med. Virology 93, 5650–5654. doi:10.1002/jmv.27089
Pizzato, M., Baraldi, C., Boscato Sopetto, G., Finozzi, D., Gentile, C., Gentile, M. D., et al. (2022). ‘SARS-CoV-2 and the host cell: A tale of interactions’. Front. Virology 1. doi:10.3389/fviro.2021.815388
Poduri, R., Joshi, G., and Jagadeesh, G. (2020). ‘Drugs targeting various stages of the SARS-CoV-2 life cycle: Exploring promising drugs for the treatment of Covid-19’. Cell. Signal. 74, 109721. doi:10.1016/j.cellsig.2020.109721
Poh, T. Y., Ali, N. A. B. M., Mac Aogain, M., Kathawala, M. H., Setyawati, M. I., Ng, K. W., et al. (2018). ‘Inhaled nanomaterials and the respiratory microbiome: Clinical, immunological and toxicological perspectives’. Part. Fibre Toxicol. 15, 46. doi:10.1186/s12989-018-0282-0
Pöyhönen, L., Bustamante, J., Casanova, J. L., Jouanguy, E., and Zhang, Q. (2019). ‘Life-Threatening infections due to live-attenuated vaccines: Early manifestations of inborn errors of immunity’. J. Clin. Immunol. 39, 376–390. doi:10.1007/s10875-019-00642-3
Provenzano, D., Rao, Y. J., Mitic, K., Obaid, S. N., Pierce, D., Huckenpahler, J., et al. (2020) Rapid prototyping of reusable 3D-printed N95 equivalent respirators at the george Washington university. Basel, Switzerland: MDPI Preprints, 2020030444. doi:10.20944/preprints202003.0444.v1
Raeven, R. H. M., van Riet, E., Meiring, H. D., Metz, B., and Kersten, G. F. A. (2019). ‘Systems vaccinology and big data in the vaccine development chain’. Immunology 156, 33–46. doi:10.1111/imm.13012
Rafat, M., Roshan, A., Abyar, M., Keramati, S., and Nikpoor, A. R. (2021). ‘Bioinformatic evaluation of the miRNAs targeting ACE2 gene in COVID-19’. Dis. Diagnosis 10, 135–143. doi:10.34172/ddj.2021.26
Rahman, H. S., Aziz, M. S., Hussein, R. H., Othman, H. H., Salih Omer, S. H., Khalid, E. S., et al. (2020). ‘The transmission modes and sources of COVID-19: A systematic review’. Int. J. Surg. Open 26, 125–136. doi:10.1016/j.ijso.2020.08.017
Rahman, M. S., Hoque, M. N., Islam, M. R., Islam, I., Mishu, I. D., Rahaman, M. M., et al. (2021). ‘Mutational insights into the envelope protein of SARS-CoV-2’. Gene Rep. 22, 100997. doi:10.1016/j.genrep.2020.100997
Rana, M. M. (2021). ‘Polymer-based nano-therapies to combat COVID-19 related respiratory injury: Progress, prospects, and challenges’. J. Biomaterials Sci. Polym. Ed. 32, 1219–1249. doi:10.1080/09205063.2021.1909412
Rappuoli, R., Black, S., and Bloom, D. E. (2019). ‘Vaccines and global health: In search of a sustainable model for vaccine development and delivery’. Sci. Transl. Med. 11, eaaw2888. doi:10.1126/scitranslmed.aaw2888
Rasmi, Y., Saloua, K. S., Nemati, M., and Choi, J. R. (2021). ‘Recent progress in nanotechnology for Covid-19 prevention, diagnostics and treatment’. Nanomaterials 11, 1788. doi:10.3390/nano11071788
Refaat, H., Mady, F. M., Sarhan, H. A., Rateb, H. S., and Alaaeldin, E. (2021). ‘Optimization and evaluation of propolis liposomes as a promising therapeutic approach for COVID-19’. Int. J. Pharm. 592, 120028. doi:10.1016/j.ijpharm.2020.120028
Ribeiro, B. V., Cordeiro, T. A. R., Oliveira e Freitas, G. R., Ferreira, L. F., and Franco, D. L. (2020). ‘Biosensors for the detection of respiratory viruses: A review’. Talanta Open 2, 100007. doi:10.1016/j.talo.2020.100007
Rodriguez-Manzano, J., Malpartida-Cardenas, k., Moser, N., Pennisi, N., Cavuto, M., Miglietta, L., et al. (2020). ‘A handheld point-of-care system for rapid detection of SARS-CoV-2 in under 20 minutes’. ACS Cent. Sci. 7 (2), 307–317. doi:10.1021/acscentsci.0c01288
Rosa, S. S., Prazeres, D. M., Azevedo, A. M., and Marques, M. P. (2021). ‘mRNA vaccines manufacturing: Challenges and bottlenecks’. Vaccine 39 (16), 2190–2200. doi:10.1016/j.vaccine.2021.03.038
Rosati, G., Idili, A., Parolo, C., Fuentes-Chust, C., Calucho, E., Hu, L., et al. (2021). ‘Nanodiagnostics to face SARS-CoV-2 and future pandemics: From an idea to the market and beyond’. ACS Nano 15, 17137–17149. doi:10.1021/acsnano.1c06839
Rossi, G. A., Sacco, O., Mancino, E., Cristiani, L., and Midulla, F. (2020). ‘Differences and similarities between SARS-CoV and SARS-CoV-2: Spike receptor-binding domain recognition and host cell infection with support of cellular serine proteases’. Infection 48, 665–669. doi:10.1007/s15010-020-01486-5
Ruiz-Hitzky, E., Darder, M., Wicklein, B., Ruiz-Garcia, C., Martin-Sampedro, R., del Real, G., et al. (2020). ‘Nanotechnology responses to COVID-19’. Adv. Healthc. Mater. 9, 2000979. doi:10.1002/adhm.202000979
Sabirli, R., Koseler, A., Goren, T., Turkcuer, I., and Kurt, O. (2021). ‘High GRP78 levels in covid-19 infection: A case-control study’. Life Sci. 265, 118781. doi:10.1016/j.lfs.2020.118781
Sadighbayan, D., Hasanzadeh, M., and Ghafar-Zadeh, E. (2020). ‘Biosensing based on field-effect transistors (FET): Recent progress and challenges’. TrAC - Trends Anal. Chem. 133, 116067. doi:10.1016/j.trac.2020.116067
Saki, E. F., Setiawan, S. A., and Wicaksono, D. H. B. (2021). ‘Portable tools for Covid-19 point-of-care detection: A review’. IEEE Sensors J. 21, 23737–23750. doi:10.1109/JSEN.2021.3110857
Samson, R., Navale, G. R., and Dharne, M. S. (2020). ‘Biosensors: Frontiers in rapid detection of COVID-19’. 3 Biotech. 10, 385. doi:10.1007/s13205-020-02369-0
Sandersjöö, L., Jonsson, A., and Löfblom, J. (2015). ‘A new prodrug form of Affibody molecules (pro-Affibody) is selectively activated by cancer-associated proteases’. Cell. Mol. Life Sci. 72, 1405–1415. doi:10.1007/s00018-014-1751-8
Sarker, S., Jamal, L., Ahmed, S. F., and Irtisam, N. (2021). ‘Robotics and artificial intelligence in healthcare during COVID-19 pandemic: A systematic review’. Robotics Aut. Syst. 146, 103902. doi:10.1016/j.robot.2021.103902
Schoeman, D., and Fielding, B. C. (2019). ‘Coronavirus envelope protein: Current knowledge’. Virology J. 16, 69. doi:10.1186/s12985-019-1182-0
Schouten, J., De Waele, J., Lanckohr, C., Koulenti, D., Haddad, N., Rizk, N., et al. (2021). ‘Antimicrobial stewardship in the ICU in COVID-19 times: The known unknowns’. Int. J. Antimicrob. Agents 58, 106409. doi:10.1016/j.ijantimicag.2021.106409
Sebastian, J., Ravi, M. D., and Kumar, T. P. (2020). ‘COVID-19 vaccine development, trials and tribulations’. Indian J. Pharm. Educ. Res. 54, s457–sS463. doi:10.5530/ijper.54.3s.144
Shafiee, A., Moradi, L., Lim, M., and Brown, J. (2021). ‘Coronavirus disease 2019: A tissue engineering and regenerative medicine perspective’. Stem Cells Transl. Med. 10, 27–38. doi:10.1002/sctm.20-0197
Shand, H., Dutta, S., Rajakumar, S., James Paulraj, S., Mandal, A. K., Kt, R. D., et al. (2022). ‘New age detection of viruses: The nano-biosensors’. Front. Nanotechnol. 3. doi:10.3389/fnano.2021.814550
Shapiro, R. S. (2021). ‘COVID-19 vaccines and nanomedicine’. Int. J. Dermatology 60, 1047–1052. doi:10.1111/ijd.15673
Sharma, S., Saini, S., Khangembam, M., and Singh, V. (2021). ‘Nanomaterials-Based biosensors for COVID-19 detection - a review’. IEEE Sensors J. 21, 5598–5611. doi:10.1109/JSEN.2020.3036748
Shih, H-I., Wu, C-J., Tu, Y-F., and Chi, C-Y. (2020). ‘Fighting COVID-19: A quick review of diagnoses, therapies, and vaccines’. Biomed. J. 43 (4), 341–354. doi:10.1016/j.bj.2020.05.021
Shorten, C., Khoshgoftaar, T. M., and Furht, B. (2021). ‘Deep learning applications for COVID-19’. J. Big Data 8, 18. doi:10.1186/s40537-020-00392-9
Shrivastav, A. M., Cvelbar, U., and Abdulhalim, I. (2021). ‘A comprehensive review on plasmonic-based biosensors used in viral diagnostics’. Commun. Biol. 4, 70. doi:10.1038/s42003-020-01615-8
Siddiquie, R. Y., Agrawal, A., and Joshi, S. S. (2020). ‘Surface alterations to impart antiviral properties to combat COVID-19 transmission’. Trans. Indian Natl. Acad. Eng. 5, 343–347. doi:10.1007/s41403-020-00096-9
Silveira, M. M., Moreira, G. M. S. G., and Mendonça, M. (2021). ‘DNA vaccines against COVID-19: Perspectives and challenges’. Life Sci. 267, 118919. doi:10.1016/j.lfs.2020.118919
Singh, P., Singh, D., Sa, P., Mohapatra, P., Khuntia, A., and Sahoo, S. (2021). ‘Insights from nanotechnology in COVID-19: Prevention, detection, therapy and immunomodulation’. Nanomedicine 16, 1219–1235. doi:10.2217/nnm-2021-0004
Singhera, G. K., Guo, T., Leung, J., Sin, D. D., and Dorscheid, D. R. (2021) ‘Effect of therapeutics on the modulation of ACE2 expression in airway epithelium: Implications for COVID-19’. Am. J. Respir. Crit. Care Med. 203, a1283. doi:10.1164/ajrccm-conference.2021.203.1_meetingabstracts.a1283
Skwarek, A., Gasecka, A., Jaguszewski, M., Szarpak, L., Dzieciatkowski, T., and Filipiak, K. (2021). ‘Nanoparticles: Breakthrough in COVID-19 prevention, diagnosis and treatment’. Archives Med. Sci. doi:10.5114/aoms/142103
Smith, T. R. F., Patel, A., Ramos, S., Elwood, D., Zhu, X., Yan, J., et al. (2020). ‘Immunogenicity of a DNA vaccine candidate for COVID-19’. Nat. Commun. 11, 2601. doi:10.1038/s41467-020-16505-0
Snyder, E. M., and Johnson, B. D. (2020). ‘ACE2 and COVID-19: Using antihypertensive medications and pharmacogenetic considerations’. Pharmacogenomics 21, 695–703. doi:10.2217/pgs-2020-0048
Softa, A., Bahl, S., Bagha, A. K., Sehgal, S., Haleem, A., and Javaid, M. (2021). ‘Tissue engineering and its significance in healthcare during COVID-19 pandemic: Potential applications and perspectives’. J. Industrial Integration Manag. 6, 221–233. doi:10.1142/S242486222150007X
South, A. M., Diz, D. I., and Chappell, M. C. (2020). ‘COVID-19, ACE2, and the cardiovascular consequences’. Am. J. Physiology - Heart Circulatory Physiology 318, H1084–H1090. doi:10.1152/AJPHEART.00217.2020
StatNano (2022). Technology against COVID-19: Nano insights into prevention, diagnosis, and treatment. Available at: https://statnano.com/technology-against-covid-19-nano-insights (Accessed October 24, 2022).
Stewart, C. L., Thornblade, L. W., Diamond, D. J., Fong, Y., and Melstrom, L. G. (2020). ‘Personal protective equipment and COVID-19’. Ann. Surg. 272, e132–e138. doi:10.1097/sla.0000000000003991
Subhash, P., and Chaudhary, A. (2021). ‘Emerging potential of metallodrugs to target coronavirus: Efficacy, toxicity and their mechanism of action’. Asian J. Chem. 33, 1191–1207. doi:10.14233/ajchem.2021.23228
Swennen, G. R. J., Pottel, L., and Haers, P. E. (2020). ‘Custom-made 3D-printed face masks in case of pandemic crisis situations with a lack of commercially available FFP2/3 masks’. Int. J. Oral Maxillofac. Surg. 49, 673–677. doi:10.1016/j.ijom.2020.03.015
Tabary, M., Araghi, F., Nasiri, S., and Dadkhahfar, S. (2021). ‘Dealing with skin reactions to gloves during the COVID-19 pandemic’. Infect. Control Hosp. Epidemiol. 42, 247–248. doi:10.1017/ice.2020.212
Talebian, S., Wallace, G. G., Schroeder, A., Stellacci, F., and Conde, J. (2020). ‘Nanotechnology-based disinfectants and sensors for SARS-CoV-2’. Nat. Nanotechnol. 15, 618–621. doi:10.1038/s41565-020-0751-0
Tatara, A. M. (2020). ‘Role of tissue engineering in COVID-19 and future viral outbreaks’. Tissue Eng. - Part A 26, 468–474. doi:10.1089/ten.tea.2020.0094
Tavakol, S., Zahmatkeshan, M., Mohammadinejad, R., Mehrzadi, S., Joghataei, M. T., Alavijeh, M. S., et al. (2021). ‘The role of nanotechnology in current COVID-19 outbreak’. Heliyon 7, e06841. doi:10.1016/j.heliyon.2021.e06841
Tavares, J. L., Cavalcanti, I. D. L., Santos Magalhaes, N. S., and Lira Nogueira, M. C. d. B. (2022). ‘Nanotechnology and COVID-19: Quo vadis?’. J. Nanoparticle Res. 24, 62. doi:10.1007/s11051-022-05452-0
Tcharkhtchi, A., Abbasnezhad, N., Zarbini Seydani, M., Zirak, N., Farzaneh, S., and Shirinbayan, M. (2021). ‘An overview of filtration efficiency through the masks: Mechanisms of the aerosols penetration’. Bioact. Mater. 6, 106–122. doi:10.1016/j.bioactmat.2020.08.002
Tenchov, R., Bird, R., Curtze, A. E., and Zhou, Q. (2021). ‘Lipid nanoparticles from liposomes to mRNA vaccine delivery, a landscape of research diversity and advancement’. ACS Nano 15, 16982–17015. doi:10.1021/acsnano.1c04996
Thanh Le, T., Andreadakis, Z., Kumar, A., Gomez Roman, R., Tollefsen, S., Saville, M., et al. (2020). ‘The COVID-19 vaccine development landscape’. Nat. Rev. Drug Discov. 19, 305–306. doi:10.1038/d41573-020-00073-5
Tharayil, A., Rajakumari, R., Chirayil, C. J., Thomas, S., and Kalarikkal, N. (2021). ‘A short review on nanotechnology interventions against COVID-19’. Emergent Mater. 4, 131–141. doi:10.1007/s42247-021-00163-z
Thi, T. T. H., Suys, E. J. A., Lee, J. S., Nguyen, D. H., Park, K. D., and Truong, N. P. (2021). ‘Lipid-based nanoparticles in the clinic and clinical trials: From cancer nanomedicine to COVID-19 vaccines’. Vaccines 9, 359. doi:10.3390/vaccines9040359
Tiamiyu, A. O. (2021). “‘Role of nanotechnology in coronavirus detection’,” in Detection and analysis of SARS coronavirus (Wiley). doi:10.1002/9783527832521.ch6
Tinberg, C. E., Khare, S. D., Dou, J., Doyle, L., Nelson, J. W., Schena, A., et al. (2013). ‘Computational design of ligand-binding proteins with high affinity and selectivity’. Nature 501, 212–216. doi:10.1038/nature12443
Tino, R., Moore, R., Antoline, S., Ravi, P., Wake, N., Ionita, C. N., et al. (2020). ‘COVID-19 and the role of 3D printing in medicine’. 3D Print. Med. 6, 11. doi:10.1186/s41205-020-00064-7
Tyagi, P. K., Tyagi, S., Mishra, M., and Dashora, K. (2020). ‘Prevention, diagnosis and treatment of COVID-19: A nanotechnological perspective’. Curr. Nanosci. 17, 418–422. doi:10.2174/1573413716999201014153916
Ullah, S., Ullah, A., Lee, J., Jeong, Y., Hashmi, M., Zhu, C., et al. (2020). ‘Reusability comparison of melt-blown vs nanofiber face mask filters for use in the coronavirus pandemic’. ACS Appl. Nano Mater. 3, 7231–7241. doi:10.1021/acsanm.0c01562
USA National Institutes of Health (2020). ‘Immune-Based therapy under evaluation for treatment of COVID-19’. COVID-19 Treat. Guidel. Panel. Coronavirus Disease 2019 (COVID-19) Treatment Guidelines.
Uzay, İ. A., and Dinçer, P. (2022). ‘CRISPR-based approaches for the point-of-care diagnosis of COVID19’. Acta Medica 53, 1–14. doi:10.32552/2022.actamedica.626
Vahedifard, F., and Chakravarthy, K. (2021). ‘Nanomedicine for COVID-19: The role of nanotechnology in the treatment and diagnosis of COVID-19’. Emergent Mater. 4, 75–99. doi:10.1007/s42247-021-00168-8
Vaka, R., Khan, S., Ye, B., Risha, Y., Parent, S., Courtman, D., et al. (2022). ‘Direct comparison of different therapeutic cell types susceptibility to inflammatory cytokines associated with COVID-19 acute lung injury’. Stem Cell Res. Ther. 13, 20. doi:10.1186/s13287-021-02699-7
Valdiglesias, V., and Laffon, B. (2020). ‘The impact of nanotechnology in the current universal COVID-19 crisis. Let’s not forget nanosafety ’. Nanotoxicology 14, 1013–1016. doi:10.1080/17435390.2020.1780332
Vashist, S. K., Venkatesh, A. G., Mitsakakis, K., Czilwik, G., Roth, G., von Stetten, F., et al. (2012). ‘Nanotechnology-Based biosensors and diagnostics: Technology push versus industrial/healthcare requirements’. BioNanoScience 2, 115–126. doi:10.1007/s12668-012-0047-4
Vaze, N., Pyrgiotakis, G., McDevitt, J., Mena, L., Melo, A., Bedugnis, A., et al. (2019). ‘Inactivation of common hospital acquired pathogens on surfaces and in air utilizing engineered water nanostructures (EWNS) based nano-sanitizers’. Nanomedicine Nanotechnol. Biol. Med. 18, 234–242. doi:10.1016/j.nano.2019.03.003
Verbeke, R., Lentacker, I., De Smedt, S. C., and Dewitte, H. (2021). ‘The dawn of mRNA vaccines: The COVID-19 case’. J. Control. Release 333, 511–520. doi:10.1016/j.jconrel.2021.03.043
Violan, C., Torán, P., Quirant, B., Lamonja-Vicente, N., Carrasco-Ribelles, L. A., Chacón, C., et al. (2021). ‘Antibody kinetics to SARS-CoV-2 at 13.5 months, by disease severity’. medRxiv. doi:10.1101/2021.09.10.21262527
Wang, C., Qu, G., and Chen, Y. (2020). ‘Coronavirus (COVID-19) combat: The power of chemistry’. Kexue Tongbao/Chinese Sci. Bull. 65, 2321–2325. doi:10.1360/TB-2020-0600
Wang, H. (2021). ‘A review of the effects of collagen treatment in clinical studies’. Polymers 13, 3868. doi:10.3390/polym13223868
Wang, J., Peng, Y., Xu, H., Cui, Z., and Williams, R. O. (2020). ‘The COVID-19 vaccine race: Challenges and opportunities in vaccine formulation’. AAPS PharmSciTech 21, 225. doi:10.1208/s12249-020-01744-7
Wang, Y., Wang, L., Cao, H., and Liu, C. (2021). ‘SARS-CoV-2 S1 is superior to the RBD as a COVID-19 subunit vaccine antigen’. J. Med. Virology 93, 892–898. doi:10.1002/jmv.26320
Weinberg, Z. Y., Hilburger, C. E., Kim, M., Cao, L., Khalid, M., Elmes, S., et al. (2021). ‘Sentinel cells enable genetic detection of SARS-CoV-2 Spike protein’. bioRxiv .doi:10.1101/2021.04.20.440678
Weng, L. M., Su, X., and Wang, X. Q. (2021). ‘Pain symptoms in patients with coronavirus disease (COVID-19): A literature review’. J. Pain Res. 14, 147–159. doi:10.2147/JPR.S269206
WHO (2022a). Coronavirus (COVID-19) dashboard. World health organization. Available at: https://covid19.who.int/(Accessed [ August 26, 2022).
WHO (2022b). COVID-19 vaccine tracker and landscape. World health organization. Available at: https://www.who.int/publications/m/item/draft-landscape-of-covid-19-candidate-vaccines (Accessed October 24, 2022).
WHO (2020b) Rational use of personal protective equipment for coronavirus disease 2019 (COVID-19): Interim guidance, 27 February 2020 (No. WHO/2019-nCov/IPCPPE_use/2020.1). WHO interim guidance. World Health Organization.
Wilder-Smith, A., and Mulholland, K. (2021). ‘Effectiveness of an inactivated SARS-CoV-2 vaccine’. N. Engl. J. Med. 385, 946–948. doi:10.1056/nejme2111165
Wilson, N., Corbett, S., and Tovey, E. (2020). ‘Airborne transmission of Covid-19’. BMJ 370, m3206. doi:10.1136/bmj.m3206
Witika, B. A., Makoni, P. A., Mweetwa, L. L., Ntemi, P. V., Chikukwa, M. T. R., Matafwali, S. K., et al. (2020). ‘Nano-Biomimetic drug delivery vehicles: Potential approaches for COVID-19 treatment’. Molecules 25, 5952. doi:10.3390/MOLECULES25245952
World Health Organization (2020). ‘Rational use of personal protective equipment for coronavirus disease 2019 (COVID-19) - interim guidance’. WHO interim Guid.
Wu, J., and Wu, Q. (2021). ‘The review of biosensor and its application in the diagnosis of COVID-19’. E3S Web Conf. 290, 03028. doi:10.1051/e3sconf/202129003028
Wu, Y., Huang, X., Yuan, L., Wang, S., Zhang, Y., Xiong, H., et al. (2021). ‘A recombinant spike protein subunit vaccine confers protective immunity against SARS-CoV-2 infection and transmission in hamsters’. Sci. Transl. Med. 13, eabg1143. doi:10.1126/scitranslmed.abg1143
Wu, Z., Hu, Y., Xu, M., Chen, Z., Yang, W., Jiang, Z., et al. (2021). ‘Safety, tolerability, and immunogenicity of an inactivated SARS-CoV-2 vaccine (CoronaVac) in healthy adults aged 60 years and older: A randomised, double-blind, placebo-controlled, phase 1/2 clinical trial’. Lancet Infect. Dis. 21, 803–812. doi:10.1016/S1473-3099(20)30987-7
Xia, X. (2021). ‘Domains and functions of spike protein in sars-cov-2 in the context of vaccine design’. Viruses 13, 109. doi:10.3390/v13010109
Xie, X., Gjorgjieva, T., Attieh, Z., Dieng, M. M., Arnoux, M., Khair, M., et al. (2020). ‘Microfluidic nano-scale QPCR enables ultra-sensitive and quantitative detection of SARS-CoV-2’. Processes 8, 1425. doi:10.3390/pr8111425
Xu, L., Li, D., Ramadan, S., Li, Y., and Klein, N. (2020). ‘Facile biosensors for rapid detection of COVID-19’. Biosens. Bioelectron. 170, 112673. doi:10.1016/j.bios.2020.112673
Xu, L., Shoaie, N., Jahanpeyma, F., Zhao, J., Azimzadeh, M., and Al−Jamal, K. T. (2020). ‘Optical, electrochemical and electrical (nano)biosensors for detection of exosomes: A comprehensive overview’. Biosens. Bioelectron. 161, 112222. doi:10.1016/j.bios.2020.112222
Xu, L. D., Zhu, J., and Ding, S. N. (2021). ‘Immunoassay of SARS-CoV-2 nucleocapsid proteins using novel red emission-enhanced carbon dot-based silica spheres’. Analyst 146, 5055–5060. doi:10.1039/d1an01010g
Xu, S., Wang, T., Liu, G., Cao, Z., Frank, L. A., Jiang, S., et al. (2021). ‘Analysis of interactions between proteins and small-molecule drugs by a biosensor based on a graphene field-effect transistor’. Sensors Actuators, B Chem. 326, 128991. doi:10.1016/j.snb.2020.128991
Yadav, D. K., Shah, P. K., Shah, S. P., and Yadav, A. K. (2020). ‘The use of disposable gloves by general public during COVID-19 increases the risk of cross-contamination’. Asia-Pacific J. Public Health 32, 289–291. doi:10.1177/1010539520932704
Yadav, N., Chauhan, M. K., and Chauhan, V. S. (2020). ‘Short to ultrashort peptide-based hydrogels as a platform for biomedical applications’. Biomaterials Sci. 8, 84–100. doi:10.1039/c9bm01304k
Yang, A., Cai, L., Zhang, R., Wang, J., Hsu, P. C., Wang, H., et al. (2017). ‘Thermal management in nanofiber-based face mask’. Nano Lett. 17, 3506–3510. doi:10.1021/acs.nanolett.7b00579
Yang, D. (2021). ‘Application of nanotechnology in the COVID-19 pandemic’. Int. J. Nanomedicine 16, 623–649. doi:10.2147/IJN.S296383
Yang, S., Li, Y., Dai, L., Wang, J., He, P., Li, C., et al. (2021). ‘Safety and immunogenicity of a recombinant tandem-repeat dimeric RBD-based protein subunit vaccine (ZF2001) against COVID-19 in adults: Two randomised, double-blind, placebo-controlled, phase 1 and 2 trials’. Lancet Infect. Dis. 21, 1107–1119. doi:10.1016/S1473-3099(21)00127-4
Yang, Y. J., Murphy, E. A., Singh, S., Sukhu, A. C., Wolfe, I., Adurty, S., et al. (2022). ‘Association of gestational age at coronavirus disease 2019 (COVID-19) vaccination, history of severe acute respiratory syndrome coronavirus 2 (SARS-CoV-2) infection, and a vaccine booster dose with maternal and umbilical cord antibody levels at delivery’. Obstetrics Gynecol. 139, 373–380. doi:10.1097/AOG.0000000000004693
Yasri, S., and Wiwanitkit, V. (2022). ‘Sustainable materials and COVID-19 detection biosensor: A brief review’. Sensors Int. 3, 100171. doi:10.1016/j.sintl.2022.100171
Yin, Y., and Wunderink, R. G. (2018). ‘MERS, SARS and other coronaviruses as causes of pneumonia’. Respirology 23, 130–137. doi:10.1111/resp.13196
Yuliani, S. H. (2021). ‘Nanoparticle as the strategy for the development of SARS-CoV-2 antiviral drugs’. Int. J. Appl. Pharm, 33–43. doi:10.22159/ijap.2021v13i5.42604
Yunus, M. H. M., Rashidbenam, Z., Fauzi, M. B., Bt Hj Idrus, R., and Bin Saim, A. (2021). ‘Evaluating feasibility of human tissue engineered respiratory epithelium construct as a potential model for tracheal mucosal reconstruction’. Molecules 26, 6724. doi:10.3390/molecules26216724
Zare, H., Aryan, E., Meshkat, Z., Gheybi, F., Neshani, A., Ghazvini, K., et al. (2021). ‘Development of biosensors for the detection of COVID-19’. Nanomedicine Res. J. doi:10.22034/nmrj.2021.01.002
Zeng, W., Liu, G., Ma, H., Zhao, D., Yang, Y., Liu, M., et al. (2020). ‘Biochemical characterization of SARS-CoV-2 nucleocapsid protein’. Biochem. Biophysical Res. Commun. 527, 618–623. doi:10.1016/j.bbrc.2020.04.136
Zhang, D., Atochina-Vasserman, E. N., Lu, J., Maurya, D. S., Xiao, Q., Liu, M., et al. (2022). ‘The unexpected importance of the primary structure of the hydrophobic part of one-component ionizable amphiphilic janus dendrimers in targeted mRNA delivery activity’. J. Am. Chem. Soc. 144, 4746–4753. doi:10.1021/jacs.2c00273
Zhang, D., Atochina-Vasserman, E. N., Maurya, D. S., Huang, N., Xiao, Q., Ona, N., et al. (2021). ‘One-Component multifunctional sequence-defined ionizable amphiphilic janus dendrimer delivery systems for mRNA’. J. Am. Chem. Soc. 143, 12315–12327. doi:10.1021/jacs.1c05813
Zhang, T., Yang, L., Yang, X., Tan, R., Lu, H., and Shen, Y. (2021). ‘Millimeter-Scale soft continuum robots for large-angle and high-precision manipulation by hybrid actuation’. Adv. Intell. Syst. 3, 2000189. doi:10.1002/aisy.202000189
Zhang, X., Li, S., and Niu, S. (2020). ‘ACE2 and COVID-19 and the resulting ARDS’. Postgrad. Med. J. 96, 403–407. doi:10.1136/postgradmedj-2020-137935
Zhao, Z., Huang, C., Huang, Z., Lin, F., He, Q., Tao, D., et al. (2021a). ‘Advancements in electrochemical biosensing for respiratory virus detection: A review’. TrAC - Trends Anal. Chem. 139, 116253. doi:10.1016/j.trac.2021.116253
Zhao, Z., Ma, Y., Mushtaq, A., Azam Rajper, A. M., Shehab, M., Ren, H., et al. (2021b). Applications of robotics, artificial intelligence, and digital technologies during COVID-19: A review. Disaster Med. Public Health Prep. 16, 1634–1644. doi:10.1017/dmp.2021.9
Keywords: COVID-19, pandemic, nanomedicine, nanotechnology, SARS CoV-2 (COVID-19)
Citation: Ayan S, Aranci-Ciftci K, Ciftci F and Ustundag CB (2023) Nanotechnology and COVID-19: Prevention, diagnosis, vaccine, and treatment strategies. Front. Mater. 9:1059184. doi: 10.3389/fmats.2022.1059184
Received: 01 October 2022; Accepted: 30 December 2022;
Published: 11 January 2023.
Edited by:
Alokesh Pramanik, Curtin University, AustraliaReviewed by:
Anupriya Baranwal, RMIT University, AustraliaIago Cavalcanti, Federal University of Pernambuco, Brazil
Copyright © 2023 Ayan, Aranci-Ciftci, Ciftci and Ustundag. This is an open-access article distributed under the terms of the Creative Commons Attribution License (CC BY). The use, distribution or reproduction in other forums is permitted, provided the original author(s) and the copyright owner(s) are credited and that the original publication in this journal is cited, in accordance with accepted academic practice. No use, distribution or reproduction is permitted which does not comply with these terms.
*Correspondence: Cem B. Ustundag, Y2J1c3R1bmRhZ0BnbWFpbC5jb20=