- 1School of Highway, Chang’an Universitiy, Xi’an, China
- 2Gansu Road and Bridge Third Highway Engineering Co., Ltd., Lanzhou, China
- 3Jinhua Highway and Transportation Management Center, Jinhua, China
Basalt fiber, as a natural silicate fiber, has excellent engineering performance in high and low temperature resistance, high fracture strength, toughness, shear and elastic modulus in nature. Shotcrete was widely used in tunnel lining, slope support, coal mine roadway and other projects. With the continuous development of shotcrete technology, scholars found that adding fiber to shotcrete would greatly improve the performance of shotcrete. Compared with traditional plain shotcrete and steel fiber shotcrete, shotcrete incorporated with basalt fiber can dramatically improve the bending resistance, toughness and durability, therefore effectively improving the engineering performance of concrete structure, such as lining support. This paper summarized and discussed the mechanical properties, early age performance, durability and practical applications of basalt fiber reinforced concrete with a focus on basalt fiber shotcrete (BFSC). Meanwhile, it focused on the engineering performance improvement and enhancement mechanisms of basalt fiber as a mineral admixture into shotcrete, and assessed the deficiencies existing in the current research. Eventually, the suggestions and prospects for future BFSC related research were proposed.
Introduction
With the rapid development of the global economy, the mileage of road tunnel, railway tunnel and other transportation infrastructure is increasing and the demand for energy sources such as coal remains high. Both complex engineering environment of tunnel construction and underground mine environment leading to the deep need effective lining support means to ensure safety (Feng, 2021; Zhang, 2021). Shotcrete is a type of concrete materials that is formed using a jet machine to spray the mixture in a certain proportion to the target surface. It has the characteristics of short final setting time, fast hydration and hardening speed, high strength in early age and simple construction technology (Hewlett and Liska, 2019), and plays an important role in supporting and lining of underground engineering and wall protection structure of geotechnical engineering. The variable construction environment and complex construction technology of shotcrete require that shotcrete has good construction and mechanical properties. However, the strength grade of existing shotcrete is low, so as to difficult adapt to the lining engineering environment in complex areas. At the same time, in the process of research and application of shotcrete, rapid setting and early strength are often pursued, while the development and change of its later strength are ignored, resulting in a series of durability problems. Therefore, it is necessary to comprehensively improve the early performance, mechanical properties and durability of shotcrete (Zhang, 2021). With the continuous development of shotcrete, scholars have found that adding fibers can greatly improve the compactness, durability and mechanical properties of shotcrete, such as bending and tensile resistance.
At present, the commonly used shotcrete fibers mainly include steel fiber (SF), basalt fiber (BF), polypropylene fiber, Polyacrylonitrile, PET fiber, carbon fiber, etc. Table 1 showed the types of fibers commonly used in concrete and the corresponding material parameters. Steel fiber has higher crack resistance, shearing resistance, high tensile strength and impact resistance, but it also has a lot of insurmountable shortcomings: 1. the steel fiber is not resistant to acid and alkali and easy to corrosion in concrete. 2. the weight of steel fiber is larger, which will not only lead to the difficult operation of steel fiber reinforced concrete in the construction, but also easy to sink in the concrete resulting in uneven distribution. Thus, the excellent performance of steel fiber cannot be effectively exhibited (Chen, 2019); Under the condition of appropriate dosage, the mechanical properties of polypropylene fiber shotcrete may not be as good as steel fiber shotcrete. However, the excellent performance of steel fiber in shotcrete will gradually fail, while polypropylene fiber shotcrete can be effective for a long time in the corrosion environment. Polypropylene fiber has outstanding advantages in corrosion resistance and strengthening the toughness of concrete, but its bond with concrete is poor and easy to aging (Li et al., 2021). Polyacrylonitrile fiber is a kind of synthetic fiber, which belongs to hydrophilic fiber, so it can be evenly dispersed in concrete, which can improve the bonding performance between fiber and concrete. Compared with polypropylene fiber, polyacrylonitrile fiber has higher elastic modulus and breaking strength. When it is added in concrete, the service life of polyacrylonitrile fiber concrete can be extended (Wang, 2019; Wang et al., 2021). PET fiber is made from the recycle PET bottles such as beverage bottles, and it is considered to the ecofriendly fiber, while it has poor combination with inorganic matrix (Marthong and Sarma, 2016; Cui et al., 2019). Compared with other fibers, high price is the disadvantage of carbon fiber (Jiang et al., 2014).
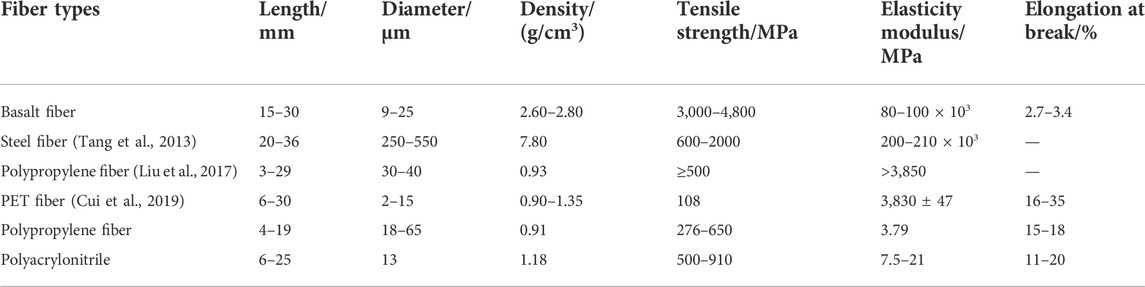
TABLE 1. Fiber types of shotcrete and its material parameter (Cheng and Li, 2008).
The raw material of basalt fiber (BF) is natural basalt, which is made by melting at high temperature of 1,450–1,500°C, Figure 1 (High et al., 2015; Girgin and Yildirim, 2016) showed the macroscopic and microscopic morphology characteristics of short-cut BF for concrete. BF has numerous excellent engineering properties, such as high and low temperature resistance (−200–880°C), low thermal conductivity, alkali corrosion resistance, high elongation, high elastic modulus, and low density (Yu et al., 2015; Pantawane and Sharma, 2022). Moreover, due to the nature of BF, there are almost no toxic and harmful substances, waste gas, waste water, waste residue and other substances discharge in the process of production and processing. Compared with glass fiber and carbon fiber, BF needs less energy and does not add any additives in the process of manufacturing, which is the reason of lower production cost. Therefore, it is called as the pollution-free green industrial materials and new materials for the 21st century (Fiore et al., 2015). Compared with other fibers, basalt fibers can simultaneously satisfy the corrosion resistance, environmental protection, good adhesion to concrete, high elastic modulus, light weight, low production energy consumption and low cost, and it can not only overcome the shortcomings of uneven distribution of fibers in the concrete matrix and the difficulty of shotcrete pumping construction, but also effectively prevent the development of cracks in the concrete. Therefore, the concrete material with basalt fiber is often considered as a kind of fiber reinforced cement-based material with good development prospects (Gao et al., 2014; Khandelwal and Rhee, 2020).
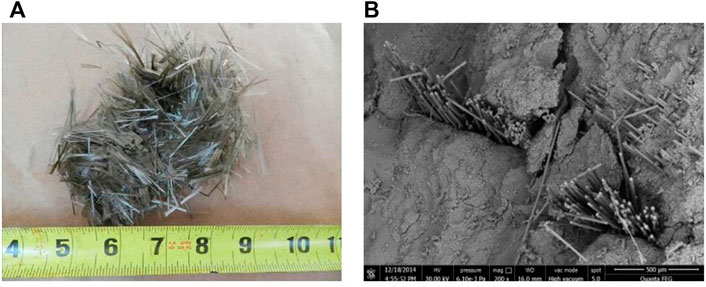
FIGURE 1. Morphology of basalt fiber and SEM (High et al., 2015; Girgin and Yildirim, 2016) (A) Macroscopic morphology (B) SEM morphology.
At present, both BFRC and BFSC have been applied in practical projects worldwide. Yang Yang (2018) selected a test section in the Huilai West Harbour seawall project to carry out the engineering application of basalt fiber-reinforced geopolymeric concrete, focusing on the construction performance, temperature rise and deformation monitoring at the construction site. Compared with ordinary concrete, basalt fiber reinforced geopolymer concrete was more suitable for rapid construction of seawall at tidal level, and it was beneficial to reduce the temperature shrinkage of seawall panels. Huan Huan (2013) took the restoration and reinforcement project of the existing tunnel “Yongxiang Tunnel” as the background, compared the initial support structure forms of three different materials, namely basalt fiber shotcrete, composite fiber shotcrete and shotcrete. The conclusion was that composite fiber shotcrete and basalt fiber shotcrete had better flatness and compactness than plain concrete, and basalt fiber shotcrete was more economical than composite fiber shotcrete. However, compare with the application of steel fiber reinforced concrete and polypropylene fiber concrete (Hagelia, 2011; Bhasin and Aarset, 2020; Jiang et al., 2020; Alencar Monteiro and Andrade Silva, 2021; Manquehual et al., 2022), the application of basalt fiber reinforced concrete was limited. Basalt fiber is a kind of new inorganic fiber material with good physical and mechanical properties, and it has the characteristics of high cost performance and easy access to materials. Therefore, basalt fiber reinforced concrete has a very broad development prospects. Further exploration of the properties of basalt fiber reinforced concrete and the promotion of its engineering application can create good economic and social benefits.
Nowadays, the relevant researches on BFSC were still in its infancy and most of them focus on BFRC. The experiments on different types of fiber reinforced concretes showed that (Leung et al., 2005), there was a certain similarity between the related properties of fiber reinforced concrete and fiber shotcrete. A series of relevant standards for basalt fiber and basalt fiber reinforced concrete were formulated in China, such as Short-cut basalt fiber for cement concrete and mortar, Basalt fiber classification and code, Highway engineering basalt fiber and its products (GB/T 23265-2009, 2009; Institute of Highway Science, 2010; GB/T 38111-2019, 2019). In addition, scholars conducted a lot of research on basalt fiber reinforced concrete, and studied the influence of adding fiber on the early shrinkage, early cracking, impact toughness, compressive strength, flexural and tensile strength, flexural strength and impermeability of concrete (Dong, 2011; He et al., 2012; Sun et al., 2022; Su, 2012; Hemalatha and Ramesh, 2019; Ralph et al., 2019; Alekseev and Kurilko, 2020; Hong et al., 2020; Khan et al., 2021; Li et al., 2021; Li et al., 2022a). However, most studies only focus on a certain aspect of the performance of BFRC, which was not systematic and comprehensive. This paper summarized the current research results of BFSC and BFRC, explained the influence of basalt fiber on the early performance, mechanical properties and durability of concrete from the macroscopic and microscopic mechanism perspectives. At the same time, this paper also summarized the current application of BFSC. Some suggestions to improve the performance indexes of BFSC were put forward, which provided a reference for further research and practical application of BFSC.”
Early performance of BFSC
Workability of BFSC
Since shotcrete is constructed by dry spraying or wet spraying, the workability of concrete is an important factor in determining its construction quality during wet spraying. The workability of BFRC mainly include fluidity, cohesiveness and segregation resistance. The current commonly used method to evaluate its fluidity is the slump test. Under normal circumstances, the fluidity of fresh concrete decreased after adding BF. The main factors affecting its fluidity were the volume of fiber, the ratio of length to diameter, the length of fiber, and the number of fiber per unit volume and so on. Lu et al. (2015) studied on BF for self-compacting concrete by the relationship between Bingham model and expansion degree, and it was found that the expansion degree of BF self-compacted concrete decreased with the increase of BF fiber volume content and fiber length. Meanwhile, the influence of fiber volume content was greater than that of fiber length. Wang et al. (2019a) also concluded that the influence of fiber volume was greater than that of fiber length, and the incorporation of basalt fiber reduced the slump of basalt fiber reinforced concrete by studying the influence of fiber length and fiber content on the slump of basalt fiber reinforced concrete. Jiang et al. Jiang et al. (2014) found in their study that the slump of concrete mixed with BF 22 mm was greater than that mixed with BF 12 mm under the same volume fraction, as shown in Figure 2.
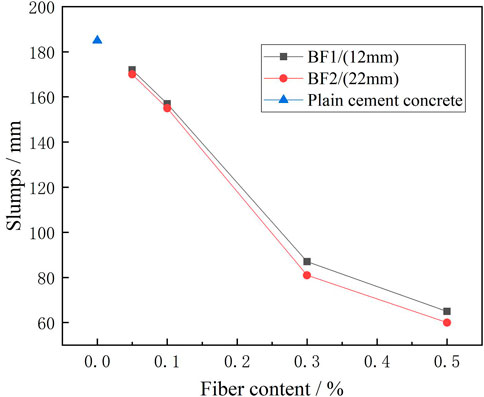
FIGURE 2. Influence of basalt fiber length and volume content on concrete slump (Jiang et al., 2014).
The actual effectiveness of the fiber depended greatly on their dispersion degree in the composites. The homodisperse of short basalt fibers could be achieved by changing the way of basalt fiber incorporation into cement paste and increasing the fluidity of BFRC. He et al. (2013) replaced 10%, 15%, 20%, 25% and 30% of cement with fly ash respectively, and it was found that fly ash had a good promotion effect on the fluidity and mechanical properties of BFRC, as shown in Figure 3. When the amount of fly ash replaced cement was less than 25%, the compressive strength and tensile strength of cement at 28 days could be improved. Li (2018) studied the effect of three fiber incorporation methods on the slump of the mixture. It was found that putting the fiber into concrete directly led to the decrease of the fluidity of the mixture. However, the method of replacing the aggregate with the same volume of steel fiber and changing the sand ratio had a better effect on improving the fluidity of the steel fiber concrete. Under the condition that the volume ratio of basalt fiber increased by 0.05%, increasing the slurry to aggregate ratio by 0.2 or decreasing the sand ratio by 2% improved the fluidity of concrete, and reducing the sand ratio was more economical than increasing the amount of cementitious material. Kabay (2014) proposed that fibers would deteriorate the workability of concrete, which was a characteristic of all fibers. The different quantity of superplasticizer was used in order to make the mixture achieve the desired slump. However, higher amount of admixture might retard the time of set of concrete and might also cause excessive slump which in turn might cause segregation problems. The slump test was used by Borhan (2013) to evaluate the workability of fresh concrete. It was found that the slump of concrete decreased with the increase of volume fraction of basalt fiber, but the unit weight was not sensitive to the slump of concrete as the fiber content was low in all mixes.
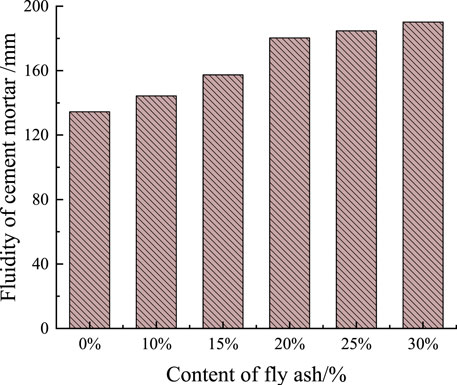
FIGURE 3. The effect of fly ash on the fluidity of BFRC (He et al., 2013).
The main reasons for the decrease of concrete fluidity caused by the incorporation of BF could be summarized as follows:
1) The fibers distributed in concrete form a network structure, which inhibited the segregation and free flow of the mixture, making it difficult to separate the slurry and aggregate, and improving the cohesion of the concrete (Zuo et al., 2010).
2) When BF, which has a high fiber content and large specific surface area, was added into concrete, more cement slurry was needed to wrap the fiber. At the same time, the cement slurry used to wrap the aggregate and lubricate gradually decreased. An increase in the viscosity of the mixture resulted in slump loss (Li, 2017).
3) For fibers with shorter lengths, their distribution number and density per unit volume are greater, and it is easier to form a network structure, thereby further reducing the slump of concrete (Jiang et al., 2014).
The measures used to overcome the reduction in slump could be summarized as follows:
1) Replacing a certain amount of cement with fly ash was an effective way to increase the fluidity of BFRC (He et al., 2013).
2) The workability of BFRC could be improved by changing the amount of cementitious materials, such as increasing the slurry to aggregate ratio and decreasing the sand ratio (Li, 2018).
3) The fluidity of BFRC could be increased by adding appropriate amount of superplasticizer (Kabay, 2014).
4) Optimizing the content and length of BF, improving the comprehensive performance of BFRC, so that the mechanical properties of the concrete were improved and the liquidity were reduced less (Borhan, 2013; Wang et al., 2019a; Li, 2021)
However, although BF reduced the fluidity of BFRC, the network structure, formed by fibers in concrete, could effectively restrain the segregation of lightweight aggregates. Li et al. Li et al. (2019a) Studied the effect of BF incorporation on the water film thickness (WFT) of mortar and the effect of fiber and WFT on the early performance of mortar, and used the sieve segregation index (SSI) to characterize the cohesion of mortar (the smaller SSI value means the better cohesion). It is concluded that basalt fiber effectively increased the cohesiveness of mortar, as shown in Figure 4. Wei (2017) tested and evaluated the workability of the fiber self-compacting concrete through slump expansion, V-funnel and L-groove tests, and came to the conclusion that the cohesion, the integrity and the segregation resistance of the self-compacting concrete mixed with fiber were improved. Zhang (2011) also used the slump method to test the workability of fiber concrete, and he proposed that concrete mixed with fiber not only had better segregation resistance than concrete without fiber, but also had better performance of surface water retention.
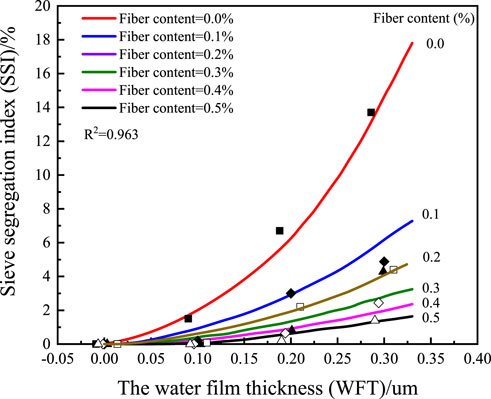
FIGURE 4. Effect of fiber length and WFT on cohesiveness of mixtures (Li et al., 2019a).
Air content and density
The distribution of pore structure in concrete was directly affected by the amount of air content The unexpected large air bubbles may lead to the uncompacted concrete, so that the workability, the mechanical properties and the durability of concrete would be greatly affected. Studies believed that the incorporation of fibers improved the pore structure of concrete. Niu et al. (2019) analyzed the relationship between fractal dimension and air content, and found that incorporation of BF could improve the pore structure of the mixture, reducing the air content and increasing the spacing coefficient, that is, the pore size and the number of pores were reduced, and the pore structure was refined and optimized. Zhao et al. (2017a) came to the same conclusion that the addition of basalt fiber not only reduced the air content of concrete, but also refined the large pores in concrete, made the distribution of pores more uniform, optimized the internal pore structure, and made the internal matrix more compact. Deng (2010) tested pore structures of three different fiber reinforced concrete by mercury injection method. From the test results, it could be concluded that: Fiber improved the pore structure inside concrete to a certain extent, and the overall trend was to reduce the formation of less harmful holes, harmful holes and more harmful holes in concrete, so that the large holes in the internal structure were refined, and the distribution of small holes was increased. Because the cohesion between basalt fiber and cement paste was better than polypropylene fiber. Therefore, the harmful pore size distribution rate of basalt fiber concrete is smaller than that of polypropylene fiber coagulation. Because the cement paste had better cohesion with basalt fiber than polypropylene fiber, the harmful pore size distribution rate of basalt fiber concrete was smaller than that of polypropylene fiber. The pore structure is an important content of concrete microstructure, which directly affects many properties of concrete. Therefore, BF incorporation not only optimizes the pore structure of concrete, but also plays an important role in improving the macroscopic properties of concrete.
Early cracking
Cracks in concrete structures are an important factor affecting the quality of construction. Usually, cracks in concrete are caused by many factors, such as temperature stress, maintenance after construction, time of mold removal, and the distribution of concrete internal structures and so on. The majority of cracks caused by the large temperature difference between day and night are surface cracks, which have little influence on the strength of concrete. After the concrete pouring is finished, the water loss during the curing process will lead to shrinkage cracks in the concrete. However, the early time of mold removal will lead to insufficient bearing capacity of concrete and the large deformation cracks, which will directly affect the mechanical properties of concrete. When the design of concrete gradation is not reasonable, and the distribution of internal components is not uniform, the deformation is not consistent when shrinkage occurs, resulting in cracks (Li and Zhao, 2020).
BF can reduce and refine the crack of concrete, which could avoid stress concentrating at the cracks and make cracks become the weak part of a concrete structure. Compared with ordinary silicate concrete, the number of cracks in the early stage of fiber concrete is reduced, while the length is shorter and the width of cracks decreases. Wang et al. (2017) studied the effect of basalt fiber length and content on early cracking of concrete. In this study, the average fracture area ratio (cracked area of BFRC/cracked area of plain concrete), fracture number ratio per unit area, the total fracture area ratio per unit area and the crack reduction coefficient were used as cracking indexes. It was found that the shrinkage crack of BFRC decreased first and then increased slowly with the increase of fiber length, and the optimal crack resistance length of BF was 18 mm. With the increase of fiber volume content, the early shrinkage crack decreased gradually, and the crack basically disappeared when the fiber volume content reached 0.2%, as shown in Figure 5.
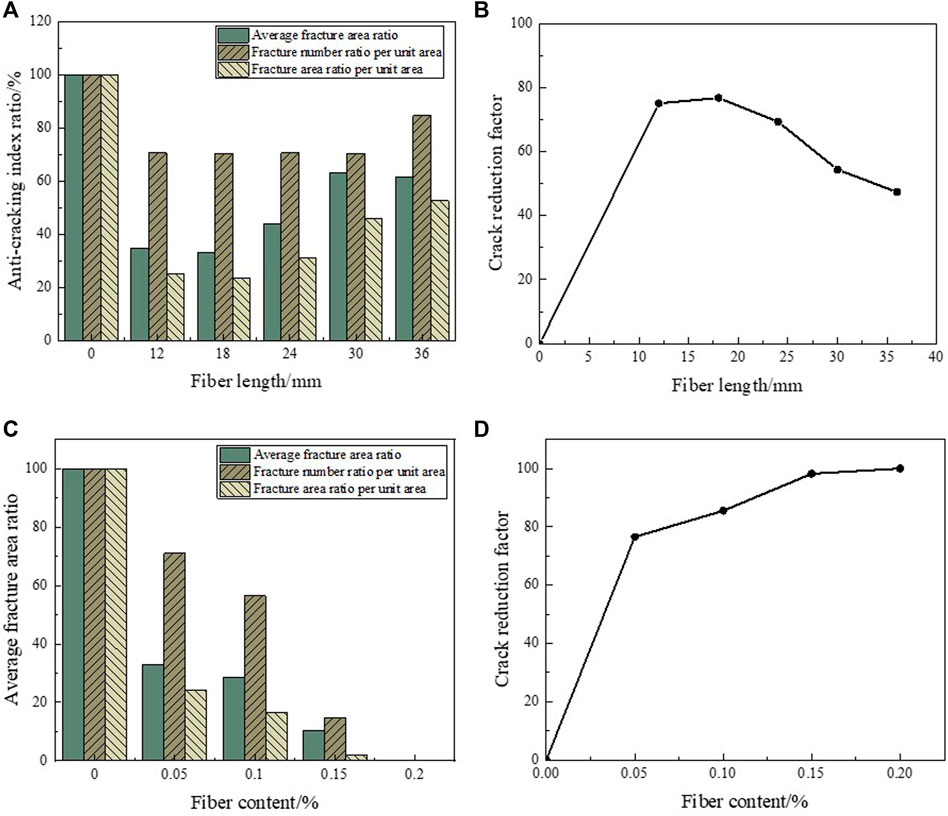
FIGURE 5. Comparison of crack parameters of basalt fiber reinforced concrete with concrete of different length and different volume content of BF (Wang et al., 2017) (A) Anti-cracking index ratio influenced by fiber length (B) Crack reduction factor influenced by fiber length (C) Anti-cracking index ratio influenced by fiber content (D) Crack reduction factor influenced by fiber content.
The improvement mechanism of BF to crack resistance of concrete is that BF forms a uniform disorderly support system in the concrete interior. When the micro-crack develops towards the fine crack, it is inevitable to encounter a number of fibers in different directions. Due to the energy consumed by the resistance of the fibers, it is difficult for the cracks to further develop. When the fiber volume content increases, the fiber quantity at the crack section of concrete increases, and more energy is needed to pull out the fiber for crack development (Yin, 2015; Wang et al., 2020).
Mechanical properties of BFSC
Shotcrete was widely used in roadway support and tunnel lining, etc. In the process of surrounding rock support, its bearing capacity should be guaranteed to meet the requirements. The common evaluation indexes of shotcrete strength are as follows: compressive strength, tensile strength, flexural strength, shear strength and bonding strength. After adding BF into concrete, most of the aforementioned properties of concrete can be improved.
Impact toughness
Concrete materials have the inherent weaknesses of large shrinkage, large brittleness, easy cracking and low fracture toughness, which restrict the long-term development of concrete. Therefore, increasing the properties of crack resistance, impact resistance, tensile resistance and ductility of cement-based materials had become a problem of the engineering field (Ralph et al., 2019; Deng et al., 2022). Recent studies showed that adding basalt fiber into concrete was an effective way to improve the toughness and impact resistance of concrete (Di Ludovico et al., 2010; Dong, 2011; He et al., 2012; Sun et al., 2022; Sun et al., 2014; Yu et al., 2015; Alekseev and Kurilko, 2020; Goud et al., 2020; He et al., 2020; Çelik and Bingöl, 2020; Li et al., 2022a; Deng et al., 2022). The increase of concrete toughness was related to the content and length of BF, the distribution of BF in concrete matrix and the degree of binding between fiber and concrete matrix (Dong, 2011; Sun et al., 2019a). Dong Dong (2011) found that the impact resistance of concrete increased first and then decreased with the increase of basalt fiber content, and the impact resistance of concrete was the best when the basalt fiber content was 1.5 kg/m3. Fan et al. Fan et al. (2011) used split hopkinson pressure bar (SHPB) with a diameter of 100 mm to carry out impact tests on BFRC with four volume contents (0, 0.1%, 0.2, 0.3%), and the test results showed that the impact compression strength and toughness were higher when the fiber volume content was 0.1%. According to the toughness study of BFRC by Liang et, al. Liang et al. (2021), the addition of basalt fiber had a good effect on the fracture toughness of concrete, but after the content of basalt fiber reaches 3 kg/m3, the improvement of fracture toughness by increasing the content was not significant. Yu et al. (2015) found that under the condition of the same basalt fiber content, the improvement effect of 6 mm fiber on the impact resistance of concrete was better than that of 18 mm fiber, as shown in Figure 6.
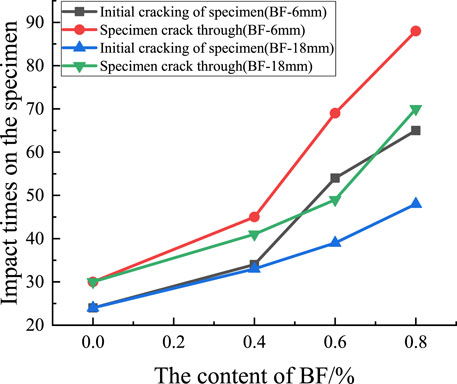
FIGURE 6. Effect of BF length and content on impact resistance of concrete (Yu et al., 2015).
The reason (Fan et al., 2011; Sun et al., 2014; Liang et al., 2021) for the existence of the best content of BF in concrete was that with the increase of the content of fiber, the uniformity of fiber dispersion becomes worse, and the probability of fiber clumping also increased in the mixing process, which was easy to leave gaps in the matrix and affect the overall strength of concrete. The improvement effect of short fiber on the impact resistance of concrete was better than that of long fiber. The reason was that short fiber could bond with concrete better and distribute more evenly. With the same content, the number of short fiber was more and the number of fiber passing through cracks increased. Therefore, short fiber could play a greater role in preventing the development of cracks (Di Ludovico et al., 2010; Yu et al., 2015). Figure 7 (Sun et al., 2019a) showed the microstructure of basalt fiber concrete with different contents by SEM scanning. It could be seen that cracks and pores appeared in ordinary concrete. When the content of BF was 0.2%, BF and matrix bonded and evenly distributed in the mortar, when BFRC was subjected to external load, the load on BFRC could be transferred to BF due to the bonding of BF and concrete, which delayed the development of cracks. When the content of BF was 0.5%, BF agglomerated in concrete and separated from concrete. The reduced cohesion of BF and concrete reduced the load transfer capacity, which adversely affected the performance of BFRC. Therefore, it is necessary to control the content and length of BF in concrete to ensure the uniform distribution of fiber, so as to maximize the improvement of impact toughness of BFRC.
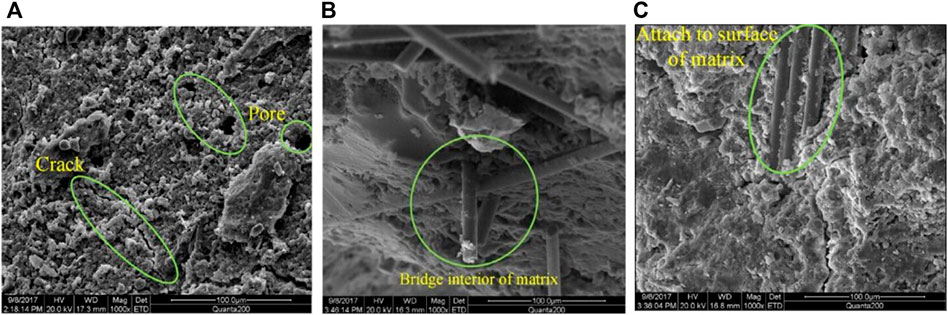
FIGURE 7. Microstructure of BFRC with BF content of 0%, 0.2% and 0.5% (Sun et al., 2019a) (A) BF content of 0% (B) BF content of 0.2% (C) BF content of 0.5%.
Bending and tensile properties
At present, most of the research results showed that BF could improve the flexural performance of concrete significantly. (Xu, 2018) studied the bending fatigue performance of BFRC and found that the bending fatigue life of concrete increases with the increase of volume content under the action of the same stress level. With the same volume dosage, the fatigue life of high stress level was enhanced more obviously. Compared with plain concrete, the average fatigue life was 37.86 times stronger. Gribniak et al. (2015) studied the young’s modulus and strength results of bending and tensile tests of BF concrete according to ASTM790-71 and other testing methods, and found that the young’s modulus of both were basically the same, while the strength obtained by bending test seemed to be much larger than that obtained by tensile test. Liu et al. (2018), Sun et al. (2019b) studied the bending impact parameters such as bending strength, bending modulus, ultimate strain, maximum strain and energy absorption capacity of basalt fiber fabric reinforced concrete specimens under different impact velocities, and found that with the increase of impact velocity, the bending modulus first increased and then decreased. According to the research of Jiao et al. (2019a), Fu et al. (2019), the maximum flexural strength of BFSC at 7 and 28 day was increased by about 2.5–9.8% and 1.6–6.8%, respectively, compared with that of normal shotcrete. When the fiber content was 3 kg/m3, the 28 d compressive strength, splitting tensile strength and bending strength of BFSC reached the maximum value. Compared with plain shotcrete, the improvement rates were 18.1%, 16.8% and 6.8%, respectively. In addition, BF had a greater effect on enhancing tensile and flexural strength at early age. At the same time, with the increase of BF content, the slump of BFSC presents a continuous downward trend. When the fiber content reaches 7.5 kg/m3, the slump of BFSC was 26 mm lower than that of ordinary SC. Figure 8 (Jiao et al., 2019b) showed the equivalent bending strength test results of BFSC with different BF admixtures.
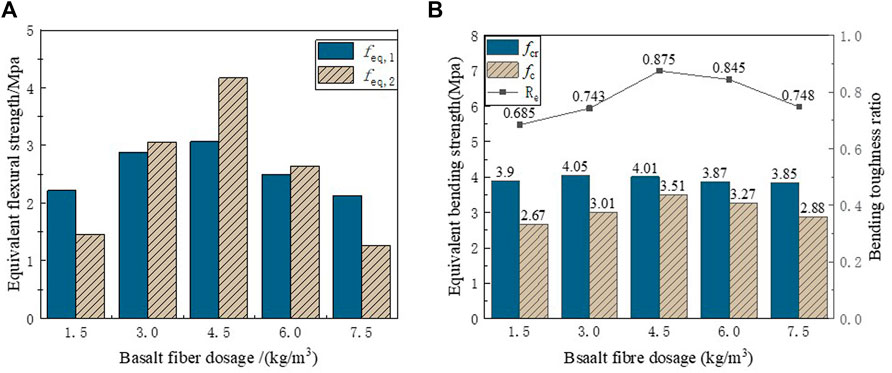
FIGURE 8. Equivalent bending strength measured by DBV-1998 and JSCE SF-4 (Jiao et al., 2019b) (A) The results measured by DBV-1998 (B) The results measured by JSCE SF-4.
The reasons for improving the flexural strength of BFSC by BF could be summarized as follows (Jiao et al., 2019b; Yan et al., 2020):
1) Cement and BF were the same type of silicate materials, which had good chemical compatibility. The fiber and shotcrete were bonded with each other, and the surface of BF was covered with dense cement hydration matrix, while BF was wrapped tightly by AFt and C-S-H, forming a large mechanical interlocking effect.
2) When cracks appear under external load, the fibers crossing the cracks consume energy to restrain the development of cracks through the de-stick slip of fibers, thus delaying the propagation driving force of cracks in concrete. When the existing crack driving force was suppressed, energy accumulates in the concrete. The anisotropic distribution of BF would find new cracks in the weak parts of the matrix and re-release energy.
3) The internal pore structure of concrete had an important effect on its performance. According to the types, it could be divided into closed pores, capillary pores and connected pores, among which connected pores had a great adverse effect on the performance of concrete. As shown in Figure 9 (Jiao et al., 2019b), when BF was at the optimal dosage in the BFSC, the number of connected pores was small, and then the flexural and tensile properties of concrete were improved accordingly.
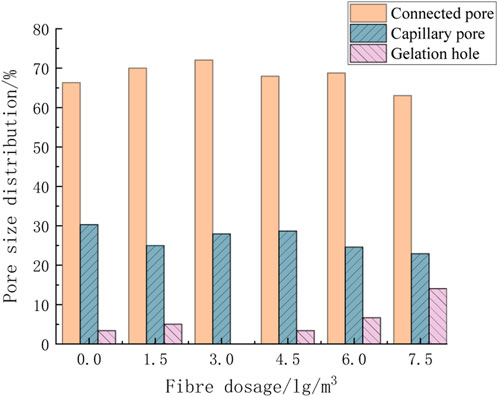
FIGURE 9. Distribution of different pore contents in BFSC measured by NMR (Jiao et al., 2019b).
Compressive strength
At present, the research on the effect of BF on the compressive strength of concrete was still controversial. Some scholars found that BF could improve the compressive strength of concrete, while some held the opposite point of view.
Gao et al. (2018) explored the effect of fiber content and length on the compressive strength of concrete at different ages by means of concrete compression test. It found that the addition of BF could improve the compressive properties of concrete, and the effect was better when the fiber length was 6 mm, but the compressive strength increases small when the content of BF increases. Kirthika et al. Kirthika and Singh (2018) found that the compressive strength of BFRC respectively increased by 20.85% and 26.80% at 7d and 28 d. Jiang et al. Jiang et al. (2014) analyzed the influence of the volume fraction and length of BF on the mechanical properties of fiber reinforced concrete, and found that the 28 d compressive strength of concrete mixed with BF with length of 12 and 22 mm respectively increased by 4.68% and 5.72%, and the improvement effect was not significant. Zhang et al. Zhang et al. (2017) found that the dynamic compressive strength of BFRC increased with the increase of loading rate, and the higher the loading rate was, the higher the dynamic compressive strength was. The addition of BF could significantly improve the dynamic compressive strength of concrete.
Shaikh and Taweel (2015) studied and compared the residual compressive strength and failure performance of steel fiber reinforced concrete and basalt fiber reinforced concrete under the condition of high temperature. The results showed that the strength retention capacity of basalt fiber reinforced concrete was lower than that of plain cement concrete, while the residual compressive strength of steel fiber reinforced concrete was higher than that of plain cement concrete. Ayub et al. (2014) found that the addition of BF had no effect on the compressive strength of high performance basalt fiber concrete, and the average strength of cube and cylinder specimens of high performance concrete containing BF varied in the range of 4%. At the same time, Li also found that BF could improve the compressive strength of concrete in the early age than in the later age, and had a partially negative effect on the compressive strength of 90 days. When the curing age reached 90 days, the compressive strength of basalt fiber reinforced concrete increases by 1.92% and 3.17%, as shown in Figure 10 (Li, 2017).
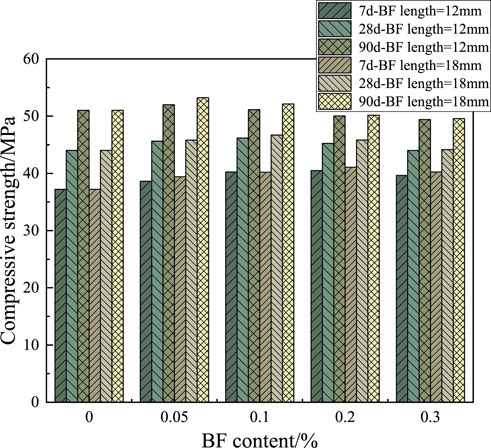
FIGURE 10. Effect of fiber content and length on compressive strength of concrete at 7, 28, and 90 d (Li, 2017).
Based on the above analysis, it could be found that there was no unified view on the improvement of concrete compressive strength from BF. Generally, the main reasons for the improvement of compressive strength of concrete by BF were as follows:
1) In concrete, the interface between aggregate, cement mortar and fiber was the weakest, and it could be seen that there were micro-protrusions on the fiber surface from the micro-morphological analysis of BF. The micro-protrusions could form mechanical occlusion between the interfaces, and then improved the compressive strength of concrete by improving the interface strength (Gao et al., 2018).
2) The crack resistance effect of BF could reduce the generation of concrete cracks to a certain extent, so as to improved the compressive strength of concrete (Li, 2017).
The main reasons for the decrease of concrete strength due to the incorporation of BF were as follows:
1) The addition of BF led to the gap between fiber and cement mortar, which led to the formation of weak interfacial layer and the decrease of compressive strength (Bernard, 2015; Gao et al., 2018).
2) When BF was mixed into concrete, the fiber was not uniformly dispersed in the matrix, resulting in the phenomenon of fiber agglomeration, which led to the loosening of the interface area and the decrease in compressive strength (Kabay, 2014; Jiao et al., 2019c).
3) The bonding between the fiber and the cement matrix interface produced the aging phenomenon, and the toughness and ductility of the matrix decreased with the increase of curing age (Bernard, 2015).
Engineering support effect
During the construction, the shotcrete is mainly sprayed by air and other forces, and the concrete sprayed on the construction surface will inevitably resilience during the construction. One index to describe the resilience performance of the shotcrete is the resilience rate, which is mainly the proportion of the resilience concrete quality to the total shotcrete mass. When the rebound rate is too large, it will lead to the waste of concrete materials and the decline of engineering economy. It was found that compared with the traditional steel fiber shotcrete, the steel fiber-basalt fiber hybrid concrete mixed with 5 kg/m3 BF could reduce the resilience rate of steel fiber by about 14%, and increased the utilization rate of steel fiber in shotcrete, and improved the resilience obviously (Liang et al., 2021).
Zhou et al. (2016) carried out an experimental study on the performance of BFSC under heat damage environment. It was found that the bond strength of concrete was greatly improved after adding BF in dry and hot environment. The failure condition of brittleness and the loss of bearing capacity due to premature cracking were improved. It was worth noting that the concrete material composed of BF and steel fiber material had similar or better toughness as steel fiber concrete, and its toughness index was excellent (Liang et al., 2021).
Basalt fiber reinforced concrete could better meet the requirements of soft surrounding rock tunnel to close the rock surface as soon as possible, while providing supporting force as soon as possible and having a certain deformation capacity. According to the study of Huan (2013), when using BFSC for initial structural support, grade IV surrounding rock could meet the safety requirements of surrounding rock structure when the initial support thickness was adjusted to 10 cm, grade III surrounding rock was 8 cm, steel mesh was cancelled and other structures remain unchanged.
In the secondary support section, the maximum 80-day deformation of the BFSC support roadway was 2.14 mm, which was about 56% lower than that of the ordinary shotcrete support roadway (Fu et al., 2019). Cui et al. Cui et al. (2017) found that the toughness of the lining structure was enhanced after adding BF, and the lining could still bear large bending moment and deformation after the initial crack. In the aspect of strengthening earthquake-damaged concrete beams and slabs, BFSC could effectively improve the ultimate deformation capacity of earthquake-damaged and undamaged concrete frame beams and slabs, and the BFSC reinforced layer of 7 mm c restore the ultimate displacement of earthquake-damaged beams and slabs to the level of no earthquake damage (Gao, 2013).
Durability performance of BFSC
The durability of concrete mainly includes frost resistance, permeability resistance and ionic erosion resistance and so on, while the impermeability is very important for the lining of water-exposed structure such as permeable surrounding rock. At the same time, when the frost resistance of concrete is insufficient, it is easy to cause a series of problems such as concrete structural damage and durability decline under the action of freeze-thaw cycle. The current research on BFSC found that when concrete is mixed with BF, its durability has a corresponding improvement effect from an overall perspective.
Frost resistance
After adding BF to concrete, it could effectively improve the frost resistance of concrete. In general, the evaluation of concrete frost resistance mainly used indicators such as dynamic elastic modulus, mass loss rate, and mechanical strength loss. Li et al. (2019b) found that after freezing-thawing cycle, the appearance of C60 concrete mixed with BF was improved in the degree of spalling damage, and the cycle times of frost damage increased with the increase of the volume fraction of BF. The mass loss rate of basalt fiber concrete was lower than that of ordinary concrete. When the mixing amount of BF reaches 0.1% and the freeze-thaw cycle was 75 times, the mass loss rate was 26% lower than that of ordinary concrete, and the relative modulus reduction phase was also lower than that of ordinary cement concrete. For BFRC with fiber content of 0.2%, the relative dynamic elastic modulus was 14.8% higher than that of ordinary concrete after 75 freeze-thaw cycles, and its relative dynamic elastic modulus was proportional to the change in fiber volume ratio (Qi, 2016). Guo et al. (2020) found that the fatigue failure cycles of BFRC were significantly higher than that of plain cement concrete at the same number of freeze-thaw cycles, and the higher the fiber content was, the stronger the ability of cement soil to resist freeze-thaw cycles was.
Some scholars also studied the cracking performance of BFRC under freeze-thaw cycles. Zhao et al. (2017b) found that the addition of BF could improve the fracture toughness of concrete under freeze-thaw cycles to a certain extent. Moreover, the increase of fiber content for instability toughness was greater than fracture toughness, but the gain ratio of both increased first and then decreased with the increase of fiber output. With the increase of the number of freeze-thaw cycles, the fracture energy of the BFRC specimens decreased significantly, and the rate of decrease was as high as 49.6%. At the same time, the ductility of the BFRC specimens increased first and then decreased with the increase of the BF content. The reason for the above phenomenon could be explained as that the BF slowed down the expansion rate of the micro-cracks of the specimen in the stress process of the specimen, but excessive BF caused more internal defects and weak transition layers to form between it and the matrix (Zhao et al., 2019a). Figure 11 (Li et al., 2019b) showed the change of the relative dynamic modulus of BFRC with the number of freeze-thaw cycles under different BF volume and fiber length. It could be found that the relative dynamic modulus of BFRC with different content decreased more slowly than that of plain concrete. At the same time, the relative modulus of BFRC of 18 mm changed slowly with the freeze-thaw cycles.
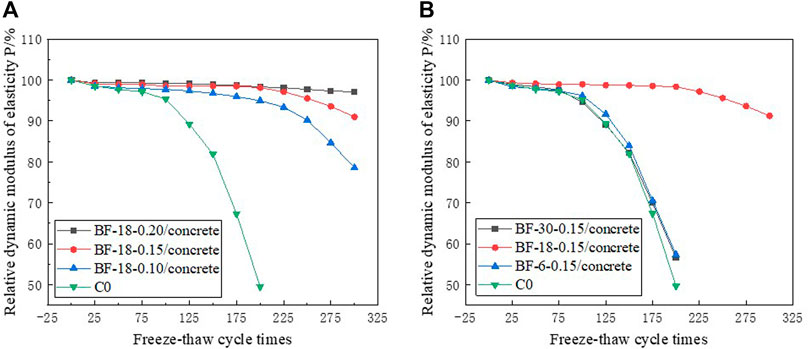
FIGURE 11. The relative dynamic modulus of BFRC varies with the number of freeze-thaw cycles under different basalt fiber volume content and fiber length (Li et al., 2019b) (A) Different volume content of basalt fiber (B) Different fiber length.
There were two main factors affecting the frost resistance of concrete: one was external factor such as temperature, humidity, and the time of freeze-thaw cycle, and the other was the internal characteristics of concrete, such as porosity, fiber content, toughness, and ultimate tensile strain and so on. The damage degree of BFRC increased with the increase of pore structure parameters such as air content, bubble spacing coefficient, average chord length of bubble and decrease with the increase of pore specific surface area. Among them, the biggest influence on frost resistance was the bubble spacing coefficient and the bubble average chord length (Zhao et al., 2019b). The main principles for improving the frost resistance of concrete by BF were:
1) Basalt fibers were randomly distributed in concrete and connected to each other, avoiding the formation of connecting pores. During the concrete mixing and forming process, the random distributed fine basalt fibers were entangled with each other to prevent the overflow of gas inside the concrete and increase the gas content.
2) BF changed the structure of cement stone and improved the impermeability of concrete, which made it difficult for external moisture to penetrate into the internal pores, reducing the freezing water in the concrete pores (Fan et al., 2014).
3) In the early stage of setting, while the elastic modulus of BF was higher than that of fresh concrete, the tensile strength of BFRC was improved in the early hardening stage, and internal micro-cracks were also reduced. Reasonable mixing amount and mixing technology guaranteed the uniform distribution and smaller spacing of fiber in concrete.
4) BF had the strong resistance of acid and alkali corrosion, it not undergo significant changes due to the corrosion of the salt solution in the concrete, so it could continue to play a positive role in supporting the framework and connecting bonds in the corrosive environment. The surrounding concrete was tightly wrapped together by BF, which slowed down the change process of concrete from dense to loose, slowed down the decrease of relative dynamic elastic modulus during the freeze-thaw cycle of concrete, and enhanced the ability of concrete to resist freeze-thaw damage (Jin et al., 2015).
Impermeability
Impermeability refers to the ability of concrete to resist the penetration of water, gas and ions, and many failure processes of concrete durability are almost closely related to water, so impermeability is an important index to evaluate concrete durability. It is generally considered that the impermeability is the primary factor to evaluate the durability of concrete. As long as the permeability of concrete is very low, there is a better resistance to water and other impermeability test corrosion medium immersion ability, thus the concrete of the various durability indicators will be improved accordingly.
The experimental study on the impermeability of BFRC showed that when the content of BF was 0.05% and 0.10%, the average seepage height of concrete was 32.7 and 28.2 cm respectively, which was 4.9% and 18.0% lower than that of plain concrete (Yin, 2015; Wang et al., 2020). When the content of BF was more than 0.15%, the average seepage height of concrete was higher than that of plain concrete, indicating that the impermeability began to deteriorate, and the deterioration tend to accelerate with the increase of fiber content. When the content of BF reached 0.35%, the seepage height of concrete increased by 46.7% compared with that of plain concrete, and the permeability coefficient also increased by an order of magnitude. It could be seen that the improvement effect of BF content on the impermeability of concrete was not monotonically enhanced with the increase of fiber content, and there was a relatively optimal content. At the same time, Hu et al. Liang et al. (2021) showed that the impermeability of BFSC could almost reach the impermeability grade of S12, and its seepage height was lower than 7% of ordinary concrete.
Jiao et al. (2019a) used NMR technology to study the internal pore size distribution of BFSC and found that after adding appropriate amount of BF, the internal pores of concrete decreased, while the proportion of micro-pores and harmless pores increased. The test results of the test block with 3 kg/m3 content showed that the proportion of harmful non-capillary pores was only 0.25%. When the content of BF increased, the maximum proportion of BF/SC non-capillary pores reached 14.03%. The specific surface area and pore size analysis tester, the nitrogen adsorption method was used to detect the microstructure of BFRC through the study of Yin (2015) and the results were shown in Table 2. When the content of BF was 0.05%–0.10%, the number of pores smaller than 100 nm in concrete, especially those smaller than 20 nm (harmless pore level), increased significantly, and the average pore size also decreased significantly. This showed that when an appropriate amount of BF was mixed into concrete, a large number of uniformly distributed micro bubbles awere introduced, the pore structure was refined, and the connected pore system was greatly reduced due to the barrier. In addition, the three-dimensional disordered fiber system weakened the stress concentration at the end of the micro-crack in the concrete, and restrains the extension of the crack to a certain extent, so that the impermeability of basalt fiber reinforced concrete was improved. When the content of BF was more than 0.20%, the cumulative pore volume of concrete increases rapidly, and the impermeability of concrete decreased rapidly. This phenomenon was caused by that the excessive fiber increased the bond interface in concrete, which had small density, large voids and high flake calcium hydroxide content. The bond interface was weak links in concrete and easy to produce micro-cracks under load, and it is easy to connect with other pores through capillaries to form infiltration channels in concrete (Yin, 2015; Wang et al., 2020).
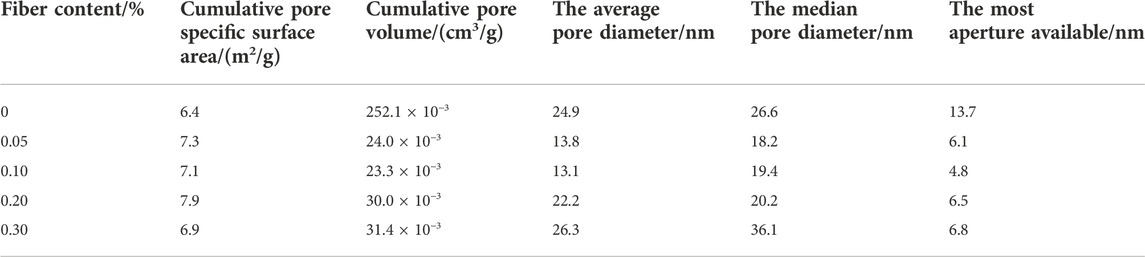
TABLE 2. Pore size distribution of BFRC with different fiber content (Yin, 2015).
The gas permeability of concrete is closely related to its internal structure (Li and Su, 2021). At present, there were few researches on the gas permeability of fiber reinforced concrete based on different pore size range. Zhang et al. Zhang et al. (2018) measured the gas permeability coefficient and effective chloride diffusion coefficient of concrete by Cembureau method and ASTM C1202 test respectively, and the mercury intrusion porosimetry (MIP) and nuclear magnetic resonance (NMR) were used to determine the pore structure of concrete respectively. It was found that there was a good positive correlation among gas permeability coefficient and the contributive porosity of pore size within 10–1,000 nm and 100–1,000 nm, as shown in Figure 12. Li and Ji (2019) also believe that the denser the concrete, the greater the cube compressive strength and splitting tensile strength, and the stronger the permeability/chloride resistance of concrete. As for the influence of BF on the air permeability of BFRC, Li and Su (2021) believed that the gas diffusivity of concrete mixed with polypropylene fiber and basalt fiber was generally smaller than that of ordinary concrete, which is because the three-dimensional distribution structure formed by the incorporated fiber in concrete could improve the bridging effect between fiber and matrix, reduce the air content of concrete and improve the compactness. The same conclusion was also reached in the study of literature (Almeida et al., 2013).
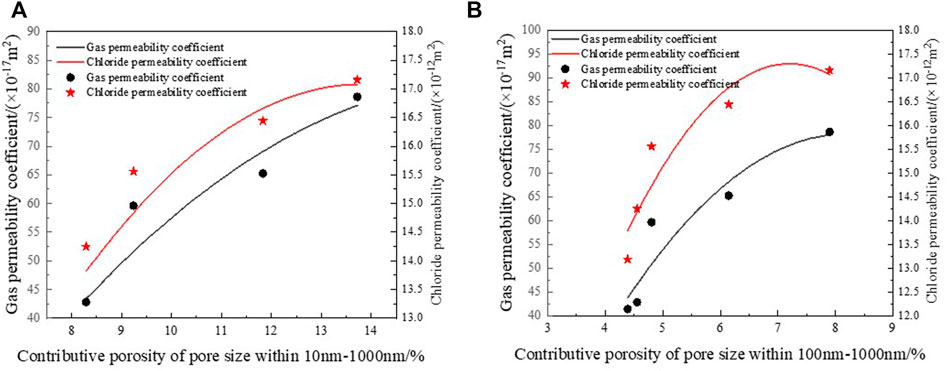
FIGURE 12. Relationship between permeability and the contributive porosity of poresize in concrete (Zhang et al., 2018) (A) Pore size within 10–1000 nm (B) Pore size within 100–1000 nm.
In addition to water penetration and gas penetration, concrete structures also be eroded by various ionic solutions in the course of service. At present, the research on the anti-ion penetration ability of BFRC was still controversial. Some scholars thought that the ion penetration resistance of concrete improved after adding BF, but some scholars thought the opposite.
Zhao et al. (2020) studied the chloride ion impermeability of BF activated concrete and found that when the water-binder ratio was constant, the electric flux first decreased and then increased with the increase of fiber volume fraction. Under the condition that the water-binder ratio was 0.22, the chloride permeability resistance of the specimen was the best when the basalt fiber volume fraction was 0.10%. Wang and Wu (2020) added 0, 0.5%, 1%, 2% and 3% basalt fibers into concrete to improve the air content and permeability of concrete, and found that with the increase of fiber content, the air content and permeability of concrete gradually decreased, and there was an optimal mixing amount of 2%. According to the study of Guo et al. (2022), the impermeability of concrete increased first and then decreased with the increase of the length and content of basalt fiber. Based on the impermeability, the optimal length of basalt fiber was recommended to be 12 mm, and the optimal BF mass fraction was 0.08%. Liu et al. (2011) also found that in the compression area, the greater the load, the denser the concrete in the compression area, which limited the occurrence of micro-cracks in concrete, leading to the difficulty of chloride ion erosion. In the tension zone, concrete was subjected to tensile stress, and the greater the load, the more likely the tensile zone of the concrete was to crack, which made it easy for chloride ion to erode concrete, leading to the increase of chloride ion concentration of concrete at the same depth. Fu et al. (2019) studied the internal structure by scanning electron microscopy (SEM), and found that BF was evenly distributed in the shotcrete matrix, closely combined with the matrix, forming a stable three-dimensional network load-bearing structure, effectively restraining the cracks in the weak interface area and reducing the internal defects of BFSC. These studies showed that the addition of BF could effectively improve the microstructure of shotcrete, and BFSC had good impermeability.
However, some studies showed that adding BF had no effects on increasing the impermeability of concrete, and even led to the decrease of the compactness of concrete and its ability to resist ion erosion. Li et al. (2022b) studied the effects of basalt fiber and calcium sulfate whisker on chloride ion permeability resistance of concrete. It was found that the influence of basalt fiber on the chloride permeation resistance of concrete was not obvious, while calcium sulfate whisker improved the chloride permeation resistance of concrete, and the appropriate amount of basalt fiber and calcium sulfate whisker also improved the chloride permeation resistance of concrete. Guo et al. (2019) studied the common effects of BF and mineral admixture (fly ash and silicon powder) on the chloride penetration resistance of concrete at different curing ages. It was found that the density of BFRC decreased with the addition of BF, and the chloride resistance decreased gradually, while mineral admixture could reduce the adverse effect of BF. The reasons for this phenomenon could be summarized as follows: 1) A loose matrix was formed around the BF, which reduced the compactness of the concrete. 2) The formation of BF network structure suppresses the segregation and flow of cement, which made the voids and air bubbles in concrete difficult to be eliminated by vibration. 3) Adding BF to concrete needed more admixtures to maintain slump, and air-entraining admixtures would produce additional pores.
In addition, some scholars also studied the ion erosion ability of BFRC in alkaline solution such as NaOH, and found that (Wang et al., 2019b; Guo et al., 2019):
1) BF could effectively prevent the migration of Na+ in alkaline environment, but its effect was gradually weakened with the extension of corrosion time.
2) Compared with ordinary concrete, the addition of BF in alkaline environment could improve the bearing capacity of concrete to a certain extent, but insufficient or excessive addition adversely affected the durability of concrete.
3) When the content of BF was 0.1%, the porosity, durability and compressive bearing capacity of concrete were improved most obviously
Summary of the influence of BF on the performance of concrete
Table 3 showed the summary of the improvement of shotcrete performance by parameters such as BF content and length, which was expressed as a percentage of the corresponding performance change compared with plain shotcrete.
Summary and prospect
This article summarized the research results of shotcrete with basalt fiber, and analyzed the effect of BF on the workability, mechanical properties and durability of shotcrete. At present, there were still some problems in BFSC research, which needed to be further explored. The existing problems and future research directions of BFSC research could be summarized as follows:
1) In terms of the workability, there was no effective way to improve the pumping ability and liquidity of BFSC during construction. The fluidity of BFSC is closely related to the material mix ratio, the content and length of fiber, and the construction environment. Therefore, it is necessary to study the parameters such as the mix ratio, fiber length-diameter ratio, and construction technology for the actual application environment of BFSC to improve the pumping performance and ejection ability of BFSC.
2) There was no unified conclusion on mechanical strength indexes such as compressive strength of BFSC, and the mechanism of its mechanical strength improvement is not clear.
3) In terms of durability, there were limited studies on the impermeability and freezing resistance of BFSC in complex environments and the influence of basalt fiber on the microstructure of concrete. The influence of BF on the pore structure in BFSC needs to be improved, and the bond mechanism between basalt fiber and concrete cannot be explained well. Future research should focus on complex working conditions and establish a constitutive model through more detailed microstructure analysis, so as to make the research more widely applicable.
4) There had been many studies on composite materials composed of basalt fiber and other fibers abroad, but there were few studies in China. At present, the research on fiber concrete has developed from a single type of fiber to mixed fiber.
5) There were few application cases of BFSC at home and abroad, and most studies were limited to laboratory analysis. Therefore, it needs further research and development to summarize the laboratory research and apply it to practical engineering.”
Author contributions
XW and FJ: Resources, Funding acquisition Conceptualization, Methodology, Supervision, Validation. PS and QL: Writing—original draft, Writing—review and editing, Data curation. YW and LL: Investigation, Validation, Writing—review and editing.
Funding
This study was partially supported by the National Key Research and Development Project (2021YFB2601000), the National Natural Science Foundation Project (52178185), the fellowship of China Postdoctoral Science Foundation, (Grant No. 2021MD703885), and the Science and Technology Planning Project of Zhejiang Provincial Department of Transportation (202224).
Acknowledgments
The authors gratefully acknowledge the support provided by above funding.
Conflict of interest
Authors LL and YW were employed by Gansu Road and Bridge Third Highway Engineering Co., Ltd, China.
The remaining authors declare that the research was conducted in the absence of any commercial or financial relationships that could be construed as a potential conflict of interest.
Publisher’s note
All claims expressed in this article are solely those of the authors and do not necessarily represent those of their affiliated organizations, or those of the publisher, the editors and the reviewers. Any product that may be evaluated in this article, or claim that may be made by its manufacturer, is not guaranteed or endorsed by the publisher.
Supplementary material
The Supplementary Material for this article can be found online at: https://www.frontiersin.org/articles/10.3389/fmats.2022.1048228/full#supplementary-material
References
Alekseev, K., and Kurilko, A. (2020). Strength characteristics of fiber-reinforced light shotcrete. E3S Web Conf. 192, 01020. doi:10.1051/e3sconf/202019201020
Alencar Monteiro, V. M., and Andrade Silva, F. (2021). On the design of the fiber reinforced shotcrete applied as primary rock support in the cuiaba underground mining excavations: A case study. Case Stud. Constr. Mater. 15, e00784. doi:10.1016/j.cscm.2021.e00784
Almeida, A. E. F. S., Tonoli, G. H. D., Santos, S. F., and Savastano, H. (2013). Improved durability of vegetable fiber reinforced cement composite subject to accelerated carbonation at early age. Cem. Concr. Compos. 42, 49–58. doi:10.1016/j.cemconcomp.2013.05.001
Ayub, T., Shafiq, N., and Nuruddin, M. F. (2014). Effect of chopped basalt fibers on the mechanical properties and microstructure of high performance fiber reinforced concrete. Adv. Mater. Sci. Eng. 2014, 1–14. doi:10.1155/2014/587686
GB/T 38111-2019 Basalt fiber classification classification and code: GB/T 38111-2019, 2019. Beijing, China: Ministry of Transport.
Bernard, E. S. (2015). Age-dependent changes in post-crack performance of fibre reinforced shotcrete linings. Tunn. Undergr. Space Technol. 49, 241–248. doi:10.1016/j.tust.2015.05.006
Bhasin, R., and Aarset, A. (2020). “Application of Norwegian Method of Tunnelling (NMT) Principles to bypass landslides in mountainous terrain,” in Geotechnics for sustainable infrastructure development (Berlin, Germany: Springer), 273–279.
Borhan, T. M. (2013). Thermal and mechanical properties of basalt fibre reinforced concrete. Proc. World Acad. Sci. Eng. Technol. 2013 (76), 313. doi:10.5281/zenodo.1058277
Çelik, Z., and Bingöl, A. F. (2020). Fracture properties and impact resistance of self-compacting fiber reinforced concrete (SCFRC). Mat. Struct. 53 (3), 50–16. doi:10.1617/s11527-020-01487-8
Chen, B. Q. (2019). Steel fiber-basalt fiber high-strength concrete mechanical properties and crack resistance. Huainan, Anhui Province: Anhui University of Science and Technology.
Cheng, L. K., and Li, X. F. (2008). Rock and soil anchorage· Soil nail· Shotcrete: Principle, design and application. Beijing: China Building Industry Press, 596–642.
Cui, G. Y., Wang, Y. D., and Ni, S. Z., (2017). Model tests on bearing characteristics of basalt fiber-reinforced concrete tunnel linings. Chin. J. Geotechnical Eng. 39 (2), 311–318. doi:10.11779/CJGE201710008
Cui, X., Liu, G., Wang, C., and Qi, Y. (2019). Effects of PET fibers on pumpability, shootability, and mechanical properties of wet-mix shotcrete. Adv. Civ. Eng. 2019, 1–14. doi:10.1155/2019/2756489
Deng, W. Q. (2010). Pore structure characteristics and durability analysis of fiber reinforced concrete. Dalian, Liaoning Province: Dalian Jiaotong University.
Deng, Y. G., Zhao, B. J., Dai, T. T., Li, G., and Li, Y. (2022). Study on the dispersibility of modified basalt fiber and its influence on the mechanical properties of concrete. Constr. Build. Mater. 350, 128839. doi:10.1016/j.conbuildmat.2022.128839
Di Ludovico, M., Prota, A., and Manfredi, G. (2010). Structural upgrade using basalt fibers for concrete confinement. J. Compos. Constr. 14 (5), 541–552. doi:10.1061/(asce)cc.1943-5614.0000114
Dong, J. Q. (2011). Study on impact resistance of basalt fiber reinforced concrete. Qinhuangdao, Hebei Province: Yanshan University.
Fan, F., Xu, J., and Bai, E., (2011). Experimental study on impact-mechanics properties of basalt fibre reinforced concrete. Adv. Build. Mater.168–170, 1910. doi:10.4028/www.scientific.net/AMR.168-170.1910
Fan, X., Wu, D., and Chen, H., (2014). Experimental research on the freeze-thaw resistance of basalt fiber reinforced concrete. Advanced construction technologies 919–921, 1912. doi:10.4028/www.scientific.net/AMR.919-921.1912
Feng, L. J. (2021). Study on load-bearing characteristics and engineering application of fiber reinforced geopolymer support structure. Xuzhou, Jiangsu Province: China University of Mining and Technology.
Fiore, V., Scalici, T., Di bella, G., and Valenza, A. (2015). A review on basalt fibre and its composites. Compos. Part B Eng. 74, 74–94. doi:10.1016/j.compositesb.2014.12.034
Fu, Y. J., Han, Z. Y., and Chen, X. M. (2019). Mechanical properties of basalt fiber shotcrete (BFRS) concrete and its application study in engineering. China Concr. Cem. Prod. 50-53, 57. doi:10.19761/j.1000-4637.2019.08.050.05
Gao, L., Hu, G. H., and Xu, N., (2014). Advances in research on engineering properties of basalt fiber. Chin. J. Undergr. Space Eng. 10 (Z2), 1749–1754.
Gao, R. (2013). Experimental research on seismic performance of damaged concrete frame T-shape beams strengthened by spraying BFRP. Wuhan, Hubei Province: Wuhan university of technology.
Gao, Z., Cao, P., and Sun, X. J., (2018). Compressive strength analysis and microscopic characterization of basalt fiber reinforced concrete. J. Hydroelectr. Eng. 37 (8), 111–120. doi:10.11660/slfdxb.20180812
Girgin, Z. C., and Yildirim, M. T. (2016). Usability of basalt fibres in fibre reinforced cement composites. Mat. Struct. 49 (8), 3309–3319. doi:10.1617/s11527-015-0721-4
Goud, E. G. P., Singh, D., Reddy, V. S., and Reddy, K. J. (2020). Stress-Strain behaviour of basalt fibre reinforced concrete. E3S Web Conf. 184, 01081. doi:10.1051/e3sconf/202018401081
Gribniak, V., Arnautov, A. K., Kaklauskas, G., Tamulenas, V., Timinskas, E., and Sokolov, A. (2015). Investigation on application of basalt materials as reinforcement for flexural elements of concrete bridges. Baltic J. Road Bridge Eng. 10 (3), 201–206. doi:10.3846/bjrbe.2015.25
Guo, S. L., Lu, Q., and Lin, Y. L., (2020). Experimental study on fatigue resistance of basalt fiber reinforced cement soil under freeze-thaw condition. J. Water Resour. Water Eng. 31 (1), 200–206. doi:10.11705/j.issn1672-643X.2020.01.30
Guo, Y., Hu, X., and Lv, J. (2019). Experimental study on the resistance of basalt fibre-reinforced concrete to chloride penetration. Constr. Build. Mater. 223, 142–155. doi:10.1016/j.conbuildmat.2019.06.211
Guo, Y. C., Xie, B., and Zhou, L. C., (2022). Impermeability attenuation and mechanism of basalt fiber reinforced concrete under dynamic fatigue load. Bull. Chin. Ceram. Soc. 41 (03), 810–817. doi:10.16552/j.cnki.issn1001-1625.2022.03.018
Hagelia, P. (2011). “Sprayed concrete in aggressive subsea environment the Oslofjord test site,” in Proceedings of the 6th International Symposium on Sprayed Concrete - Modern Use of Wet Mix, Sprayed Concrete for Underground Support, Tromsø, Norway, September, 2011. Available at: https://www.researchgate.net/publication/263168979.
He, C. J., Yao, Y., Tao, J. L., et al. Study on impact failure resistance of basalt fiber reinforced concrete (2012). Proceedings of the 3rd National Engineering Safety and Protection Academic Conference, Wuhan, China, November 16, 2012, 622–626.
He, W., Chen, S., Wang, C., Zhang, X. G., and Xiong, R. (2013). Effect of fluidity of cement mortar and dispersion of basalt fibers on mechanical properties of BFRC composites. Adv. Mat. Res. 671-674, 1869–1872. doi:10.4028/www.scientific.net/AMR.671-674.1869
He, W., Kong, X., Fu, Y., Zhou, C., and Zheng, Z. (2020). Experimental investigation on the mechanical properties and microstructure of hybrid fiber reinforced recycled aggregate concrete. Constr. Build. Mater. 261, 120488. doi:10.1016/j.conbuildmat.2020.120488
Hemalatha, T., and Ramesh, G. (2019). Mitigation of plastic shrinkage in fly ash concrete using basalt fibres. Can. J. Civ. Eng. 46 (8), 759–769. doi:10.1139/cjce-2018-0075
Hewlett, P., and Liska, M. (2019). Lea's chemistry of cement and concrete. Oxford, United Kingdom: Butterworth-Heinemann.
High, C., Seliem, H. M., El-safty, A., and Rizkalla, S. H. (2015). Use of basalt fibers for concrete structures. Constr. Build. Mater. 96, 37–46. doi:10.1016/j.conbuildmat.2015.07.138
Hong, L., Chen, Y. D., Li, T. D., Gao, P., and Sun, L. (2020). Microstructure and bonding behavior of fiber-mortar interface in fiber-reinforced concrete. Constr. Build. Mater. 232, 117235. doi:10.1016/j.conbuildmat.2019.117235
Huan, G. K. (2013). The research and application of basalt fiber sprayed concrete using in the existing tunnel’s reinforcement. China: Southwest Jiaotong University.
Institute of Highway Science (2010). Highway engineering basalt fibers and their products Part 1: Basalt short-cut fibers: JT/T 776.1-2010, Beijing, China: Institute of Highway Science.
Jiang, C., Fan, K., Wu, F., and Chen, D. (2014). Experimental study on the mechanical properties and microstructure of chopped basalt fibre reinforced concrete. Mater. Des. 58, 187–193. doi:10.1016/j.matdes.2014.01.056
Jiang, W. P., Fang, J. H., Xia, X., and Su, Q. (2020). Experimental study and engineering application of polypropylene fiber shotcrete for bored tunnels in water-rich strata. Arab. J. Sci. Eng. 45 (10), 8655–8666. doi:10.1007/s13369-020-04815-w
Jiao, H., Wu, Y., and Chen, X., (2019). Flexural toughness of basalt fibre-reinforced shotcrete and industrial-scale testing, Adv. Mater. Sci. Eng., 2019. 8. doi:10.1155/2019/6568057
Jiao, H. Z., Han, Z. Y., and Chen, X. M., (2019). Flexural toughness evolution of basalt fiber reinforced shotcrete based on NMR technology. J. China Coal Soc. 44 (10), 2990–2998. doi:10.13225/j.cnki.jccs.2018.1344
Jiao, H. Z., Han, Z. Y., and Chen, X. M., (2019). Influence mechanism of basalt fiber on the toughness and microstructure of spray concrete. Acta Mater. Compos. Sin. 36 (08), 1926–1934. doi:10.13801/j.cnki.fhclxb.20180929.001
Jin, S. J., Li, Z. L., and Zhang, J., (2015). Experimental study on anti-freezing and thawing performance of reinforced concrete of basalt fiber under corrosion condition. Eng. Mech. 32 (5), 178–183. doi:10.6052/j.issn.1000-4750.2014.04.0265
Kabay, N. (2014). Abrasion resistance and fracture energy of concretes with basalt fiber. Constr. Build. Mater. 50, 95–101. doi:10.1016/j.conbuildmat.2013.09.040
Khan, M., Cao, M., Xie, C., and Ali, M. (2021). Efficiency of basalt fiber length and content on mechanical and microstructural properties of hybrid fiber concrete. Fatigue Fract. Eng. Mat. Struct. 44 (8), 2135–2152. doi:10.1111/ffe.13483
Khandelwal, S., and Rhee, K. Y. (2020). Recent advances in basalt-fiber-reinforced composites: Tailoring the fiber-matrix interface. Compos. Part B Eng. 192, 108011. doi:10.1016/j.compositesb.2020.108011
Kirthika, S. K., and Singh, S. K. (2018). Experimental investigations on basalt fibre-reinforced concrete. J. Inst. Eng. India. Ser. A 99 (4), 661–670. doi:10.1007/s40030-018-0325-4
Leung, C. K. Y., Lai, R., and Lee, A. Y. F. Properties of wet-mixed fiber reinforced shotcrete and fiber reinforced concrete with similar composition (2005). Cem. Concr. Res. 35 (4), 95–788. doi:10.1016/j.cemconres.2004.05.033
Li, C. C., Hu, J., and Yuan, C. F., (2019). Experimental study on the freezing resistance of fiber reinforced high strength concrete. Acta Mater. Compos. Sin. 36 (8), 1977–1983. doi:10.13801/j.cnki.fhclxb.20181015.001
Li, C. C., Zhang, W. B., and Zhang, P., (2022). Experimental study on chloride penetration resistance of calcium sulfate whisker and basalt fiber concrete. Ind. Constr. 2022, 1–7. doi:10.13204/j.gyjzG21112207
Li, D. C., and Zhao, C. X. (2020). Research on basic mechanical properties of basalt fiber reinforced concrete. Highway 65 (6), 237–241.
Li, F., Cheng, Y., Wu, F., Su, C., and Li, G. (2021). Compressive test characteristics and constitutive relationship of wet polypropylene macrofiber-reinforced shotcrete. Shock Vib. 2021, 1–9. doi:10.1155/2021/6649283
Li, G. X. (2021). Experimental study on the strength of high strength basalt fiber pumping concrete. Tianjin: Tianjin Chengjian University.
Li, J. (2017). Effects of chopped basalt fiber on mechanical properties and microstructure of slag fly ash concrete. Bull. Chin. Ceram. Soc. 36 (2), 727–732. doi:10.16552/j.cnki.issn1001-1625.2017.02.055
Li, L. G., Zeng, K. L., Ouyang, Y., and Kwan, A. (2019). Basalt fibre-reinforced mortar: Rheology modelling based on water film thickness and fibre content. Constr. Build. Mater. 229, 116857. doi:10.1016/j.conbuildmat.2019.116857
Li, Y., Zhang, J., He, Y., Huang, G., Li, J., Niu, Z., et al. (2022). A review on durability of basalt fiber reinforced concrete. Compos. Sci. Technol. 225, 109519. doi:10.1016/j.compscitech.2022.109519
Li, Y. F. (2018). The fluidity and mechanical properties of high performance concrete with fibers mixed in different way. Jiaozuo, Henan Province: Henan Polytechnic University.
Li, Y., and Ji, S. P. (2019). Macro-properties and microstructure of hybrid fiber reinforced concrete in winter construction. J. Jilin Univ. Eng. Technol. Ed. 49 (03), 781–787. doi:10.13229/j.cnki.jdxbgxb20180161
Li, Y., and Su, Y. Q. (2021). Gas permeability and meso-structure of fiber reinforced concrete under carbonation based on different pore sizes. J. Jilin Univ. Eng. Technol. Ed. 51 (04), 1287–1295. doi:10.13229/j.cnki.jdxbgxb20200209
Liang, N., Ren, L., Tian, S., Liu, X., Zhong, Z., Deng, Z., et al. (2021). Study on the fracture toughness of polypropylene–basalt fiber-reinforced concrete. Int. J. Concr. Struct. Mat. 15 (1), 35–23. doi:10.1186/s40069-021-00472-x
Liu, G., Cheng, W., and Chen, L. (2017). Investigating and optimizing the mix proportion of pumping wet-mix shotcrete with polypropylene fiber. Constr. Build. Mater. 150, 14–23. doi:10.1016/j.conbuildmat.2017.05.169
Liu, H. T., Zhang, J. Z., and Fu, Z. Q., (2011). Experiment on concrete elements for chloride erosion of chopped basalt fiber. Concrete 2011 (1), 14–15. doi:10.3969/j.issn.1002-3550.2011.01.004
Liu, S., Zhu, D., Li, G., Yao, Y., Ou, Y., Shi, C., et al. (2018). Flexural response of basalt textile reinforced concrete with pre-tension and short fibers under low-velocity impact loads). Constr. Build. Mater. 169, 859–876. doi:10.1016/j.conbuildmat.2018.02.168
Lu, R., Zhu, D. Y., and Zhan, B. G. (2015). Study of rheological properties of basalt fiber reinforced self-consolidating concrete. J. Hefei Univ. Technol. Sci. 38 (9), 1249–1253. doi:10.3969/j.issn.1003-5060.2015.09.021
Manquehual, C. J., Jakobsen, P. D., Holter, K. G., De Weerdt, K., Danner, T., and Bruland, A. (2022). Comparison of the condition of steel fiber-reinforced shotcrete with water-glass and alkali-free activators after more than 20 years of service in a subsea road tunnel. Constr. Build. Mater. 328, 127090. doi:10.1016/j.conbuildmat.2022.127090
Marthong, C., and Sarma, D. K. (2016). Influence of PET fiber geometry on the mechanical properties of concrete: An experimental investigation. Eur. J. Environ. Civ. Eng. 20 (7), 771–784. doi:10.1080/19648189.2015.1072112
Niu, D., Huang, D., Zheng, H., Su, L., Fu, Q., and Luo, D. (2019). Experimental study on mechanical properties and fractal dimension of pore structure of basalt–polypropylene fiber-reinforced concrete. Appl. Sci. 9 (8), 1602. doi:10.3390/app9081602
Pantawane, R., and Sharma, P. K. (2022). A critical review on basalt fibre geo-polymer concrete. J. Phys. Conf. Ser. 2267 (1), 012014. doi:10.1088/1742-6596/2267/1/012014
Qi, G. H. (2016). Experimental study on freezing-thawing resistance of basalt fiber reinforced concrete. Jilin, jilin province: JiLin University.
Ralph, C., Lemoine, P., Summerscales, J., Archer, E., and McIlhagger, A. (2019). Relationships among the chemical, mechanical and geometrical properties of basalt fibers. Text. Res. J. 89 (15), 3056–3066. doi:10.1177/0040517518805376
Shaikh, F. U. A., and Taweel, M. (2015). Compressive strength and failure behaviour of fibre reinforced concrete at elevated temperatures. Adv. Concr. Constr. 3 (4), 283–293. doi:10.12989/acc.2015.3.4.283
Su, Q. Q. (2012). Research on durability of basalt fiber reinforced concrete. Tianjin: Tianjin Chengjian University.
Sun, X., Gao, Z., Cao, P., Zhou, C., Ling, Y., Wang, X., et al. (2019). Fracture performance and numerical simulation of basalt fiber concrete using three-point bending test on notched beam. Constr. Build. Mater. 225, 788–800. doi:10.1016/j.conbuildmat.2019.07.244
Sun, X., Gao, Z., Cao, P., and Zhou, C. (2019). Mechanical properties tests and multiscale numerical simulations for basalt fiber reinforced concrete. Constr. Build. Mater. 202, 58–72. doi:10.1016/j.conbuildmat.2019.01.018
Sun, X. J., Bao, Z. C., and Chen, Y. L., (2014). Research on the toughness of basalt fiber reinforced cement mortar. J. Hefei Univ. Technol. Sci. 37 (6), 700–704. doi:10.3969/j.issn.1003-5060.2014.06.013
Sun, X., Ou, Z., and Xu, Q. (2022). Feasibility analysis of resource application of waste incineration fly ash in asphalt pavement materials. Environ. Sci. Pollut. Res., 1–16. doi:10.1007/s11356-022-22485-z
Tang, H., Najafi, M., and Ma, S. (2013). “Trenchless technology-the best choice for underground pipeline construction and renewal,” in Proceedings of the International Conference on Pipelines and Trenchless Technology, Xi'an, China, October 16–18, 2013.
Wang, D., Qi, J., Cui, G., Yang, Y., and Chang, J. (2020). Model test on bearing characteristics of basalt fiber-reinforced concrete lining. Adv. Mater. Sci. Eng. 2020, 1–10. doi:10.1155/2020/3891343
Wang, L., He, T., Zhou, Y., Tang, S., Tan, J., Liu, Z., et al. (2021). The influence of fiber type and length on the cracking resistance, durability and pore structure of face slab concrete. Constr. Build. Mater. 282, 122706. doi:10.1016/j.conbuildmat.2021.122706
Wang, R. Z., and Wu, S. (2020). Performance evolution of fiber reinforced concrete under freeze-thaw cycles. Cryogenics (04), 38–41+67.
Wang, X., He, J., Mosallam, A. S., Li, C., and Xin, H. (2019). The effects of fiber length and volume on material properties and crack resistance of basalt fiber reinforced concrete (BFRC). Adv. Mater. Sci. Eng. 2019, 1–17. doi:10.1155/2019/7520549
Wang, X. H., Li, C. X., and Ling, J. Y., (2017). Experimental study on early crack of basalt fiber reinforced concrete. Bull. Chin. Ceram. Soc. 36 (11), 3860–3866. doi:10.16552/j.cnki.issn1001-1625.2017.11.047
Wang, Z. S., Li, H. W., and Wu, B., (2019). Experimental study on alkali corrosion resistance and mechanical properties of basalt fiber concrete. Chin. J. Appl. Mech. 36 (5), 1258–1259. doi:10.11776/cjam.36.05.B059
Wang, Z. W. (2019). Study on properties of polyacrylonitrile fiber concrete. Yangzhou, Jiangsu Province: Yangzhou University.
Wei, J. (2017). Study on alkali corrosion performance of the hybrid fiber reinforced self-compacting concrete in early age. Dalian, Liaoning Province: Dalian Jiaotong University.
Xu, B. (2018). Experimental study on flexural fatigue behavior of basalt fiber reinforced concrete. China: Kunming University of Science and Technology.
Yan, S., Jiao, H., Yang, X., et al. (2020). Bending properties of short-cut basalt fiber shotcrete in deep soft rock roadway, Adv. Civ. Eng., 2020. 9. doi:10.1155/2020/5749685
Yang, Y. M. (2018). Design and engineering application of highly seawater corrosion resistant geopolymer concrete reinforced by BFRP bar. Guangzhou, Guangdong Province: South China University of Technology.
Yin, Y. L. (2015). Experimental study on mechanical properties and durability of basalt fiber reinforced concrete. Chongqing: Chongqing Jiaotong University.
Yu, Y., Zhu, H., and Zhu, X. C., (2015). Study on impact resistance of basalt fiber reinforced concrete. J. Build. Struct. 36 (S2), 354–358. doi:10.4028/www.scientific.net/AMR.194-196.1103
Zhang, G. (2021). Performance improving mechanism and mix design method of shotcrete. Beijing: Beijing Jiaotong University.
Zhang, H., Wang, B., Xie, A., and Qi, Y. (2017). Experimental study on dynamic mechanical properties and constitutive model of basalt fiber reinforced concrete. Constr. Build. Mater. 152, 154–167. doi:10.1016/j.conbuildmat.2017.06.177
Zhang, J., Bian, F., Zhang, Y., Fang, Z., Fu, C., and Guo, J. (2018). Effect of pore structures on gas permeability and chloride diffusivity of concrete. Constr. Build. Mater. 163, 402–413. doi:10.1016/j.conbuildmat.2017.12.111
Zhang, Y. (2011). Research on basic mechanical properties of chopped basalt fiber reinforced concrete. Harbin, Heilongjiang Province: Northeast Forestry University.
Zhao, H., Wang, L., Wang, D., Bai, J., and Fan, Y. (2020). Effect of basalt fiber on chloride ion penetration of reactive powder concrete. IOP Conf. Ser. Mat. Sci. Eng. 711, 012067–12067. doi:10.1088/1757-899x/711/1/012067
Zhao, Y. R., Song, B., and Wang, L., (2019). Fracture properties of basalt fiber reinforced concrete after freeze-thaw cycles. J. Build. Mater. 22 (4), 575–583. doi:10.3969/j.issn.1007-9629.2019.04.011
Zhao, Y. R., Guo, Z. L., and Fan, X. Q. (2017). Basalt fiber reinforced concrete stress-strain relationship and pore structure analysis. Bull. Chin. Ceram. Soc. 36 (12), 4142–4150. doi:10.16552/j.cnki.issn1001-1625.2017.12.031
Zhao, Y. R., Wang, L., and Han, X. F., (2017). Study on fracture toughness of basalt fiber concrete under freezing-thawing condition. Eng. Mech. 34 (9), 92–101. doi:10.6052/j.issn.1000-4750.2016.04.0262
Zhao, Y. R., Wang, Z. H., and Wang, L., (2019). Grey entropy analysis of macro and micro properties of BFRC after freeze-thaw cycles. J. Build. Mater. 22 (1), 45–53. doi:10.3969/j.issn.1007-9629.2019.01.007
Zhou, P., Wang, Z. J., and Yang, Y., (2016). Experimental study of the performance of basalt fiber shotcrete under heat damage. J. Civ. Environ. Eng. 38 (1), 69–76. doi:10.11835/j.issn.1674-4764.2016.01.010
Keywords: basalt fiber, fiber reinforced concrete, shotcrete, mechanical properties, durability
Citation: Liu Q, Song P, Li L, Wang Y, Wang X and Fang J (2022) The effect of basalt fiber addition on cement concrete: A review focused on basalt fiber shotcrete. Front. Mater. 9:1048228. doi: 10.3389/fmats.2022.1048228
Received: 19 September 2022; Accepted: 04 November 2022;
Published: 23 November 2022.
Edited by:
Lei Wang, Xi’an University of Architecture and Technology, ChinaReviewed by:
E. Chen, Chalmers University of Technology, SwedenSaid Kenai, University of Blida, Algeria
Copyright © 2022 Liu, Song, Li, Wang, Wang and Fang. This is an open-access article distributed under the terms of the Creative Commons Attribution License (CC BY). The use, distribution or reproduction in other forums is permitted, provided the original author(s) and the copyright owner(s) are credited and that the original publication in this journal is cited, in accordance with accepted academic practice. No use, distribution or reproduction is permitted which does not comply with these terms.
*Correspondence: Xuhao Wang, d2FuZ3hoQGNoZC5lZHUuY24=