- 1Key Laboratory of High-Performance Synthetic Rubber and Composite Materials, Changchun Institute of Applied Chemistry, Chinese Academy of Sciences, Changchun, China
- 2University of Science and Technology of China, Hefei, China
Herein, a simple and efficient strategy was proposed to improve the gas barrier properties of waterborne polyurethane (WPU). Graphene oxide was first modified with dopamine and then cross-linked by boric acid (BA) to bring the graphene sheets closer together, thereby increasing the complexity of the gas pathway. FTIR, XPS, and SEM analyses proved that boric acid was uniformly dispersed into WPU, meanwhile, SEM images demonstrated the defects between the composites that were filled by the boric acid network. In addition, the mechanical properties of the composite were also enhanced. The tensile strength of the material increased from 25.2 to 34.2 MPa, and Young’s modulus increased from 63.3 to 93.7 MPa. The helium gas permeability of the composites was reduced by 85%, compared with the unmodified WPU film. The arrangement structure of GO modified with dopamine (DGO) in polyurethane is the main reason for the excellent gas barrier performance of the material This work provides great potential with GO/polyurethane for industrial application.
Introduction
Polyurethane has various advantages of good elasticity, high strength, low-temperature resistance, wear resistance, ozone resistance and good chemical resistance, which is widely used in textile, construction, aerospace, medical equipment city and other fields (Ramezanzadeh, et al., 2015; Gómez, et al., 2018; Li, et al., 2018). Polyurethane elastomer materials have high gas permeability, and the gas barrier properties of polyurethane can be improved by blending with high barrier materials, to prepare polyurethane-based materials with high gas barrier properties (Kim, et al., 2010; Ramezanzadeh, et al., 2015; Bandyopadhyay, et al., 2017).
Mussels are ubiquitous in seawater and can stick to reefs or the bottom of ships. In recent years, there have been many studies on mussels, effectively proving that the dopamine content and the adhesion ability of fetal shellfish in water are excellent. Researchers also found that dopamine hydrochloride and its derivatives are similar to the molecular structure of the dopamine in the mussels, in an alkaline environment through polymerization in metal, glass, polymer materials and various substrate surface stable adhesion (Li, et al., 2009; Jiang, et al., 2011; Ding, et al., 2014). Wang, et al. (2018) deposited polydopamine nanoclusters on the surface of cross-linked polyacrylonitrile/hyperbranched polyethylene imine film to prepare electrospun nanofiber film with a multilayer structure. Yang, et al. (2014) prepared the oil-water separation membrane by co-deposition of PDA and polyethylene imine (PEI) on the surface of the polypropylene (PP) ultrafiltration membrane. Zhang et al. fixed TiO2 particles on the surface of the membrane modified by PDA to improve hydrophilicity (Zhang, et al., 2013). In addition, although the application and deposition conditions of PDA have been extensively studied, the mechanism of polymerization and the molecular structure of PDA have not been determined. Many research groups have analyzed the structure of PDA through a variety of testing methods, and the mechanism of polymerization has been speculated and verified (Moon, et al., 2010).
As a two-dimensional nano material, graphene is compounded with polymers to improve the performance of the material and meet higher demands. Graphene composites can not only improve the mechanical properties of polymers, but also exhibit other unique properties (Kim, et al., 2010), such as piezoelectric properties, shape memory properties, gas barrier properties (Zhu, et al., 2010; Bandyopadhyay, et al., 2017; Kim, et al., 2019), and optical performance. Which become one of research hot spot in the polyurethane matrix composites in a short period time (Zhang, et al., 2018). However, related reports pointed that the nature of graphene with a lamellar structure is not ideal for hydrophilicity and lipophilicity. Due to the large specific surface area, it is difficult to obtain nanoscale uniform dispersion in the polymer resin, and the interaction force between graphene and polyurethane matrix is also weak (Ma, et al., 2018). Therefore, researchers have made a lot of attempts in the modification and pretreatment of graphene. Jiang et al. used vacuum suction filter self-assembly and successfully synthesized graphene oxide/MoS2/PU composite membrane by imitating the ternary structure of pearl shells. Then they proved the high mechanical properties of graphene oxide and MoS2 of nanometer lubricating synergistic toughening effect, at the same time, the composites has good electrical conductivity (Qin, et al., 2018). The ternary synthetic pearl with excellent toughness has broad application prospects in aerospace, flexible supercapacitor electrode, artificial muscle, tissue engineering, and other fields (Yan, et al., 2020). Li et al. used boric acid as a cross-linking agent to prepare PVA/GO blend film (Li, et al., 2018). The material transmittance was less than 0.005 cc m−2 day−1, the permeability was less than 5.0*10−20 cm3 cm cm−2 and the transmittance was more than 85% at 550 nm, which has the potential to be used as industrial productions. Possible reasons for the insufficient barrier performance could be resulted from, the aggregation of GO at high content (low aspect ratio) and the poor adhesion between the polymer and GO sheets (interfacial spaces). Kim, et al. (2010) used boric acid as a cross-linking agent to prepare the GO/boric acid/EVOH ternary structure. Through boric acid cross-linking, a blend film with excellent barrier properties, mechanical properties, and corrosion resistance was successfully obtained. The gas permeability of the barrier property was reduced by 96.1% compared with pure epoxy resin. The corrosion rate and corrosion rate ratios are 3.43.10−3 mm/year and 98.46%, respectively. This blended material can be used in gas storage, food packaging, and anti-corrosive coatings (Yan, et al., 2020). Seo crosslinked MXene and GO through 3-aminopropyl triethoxysilane (APTES) by employing carbodiimide reaction. The 10 wt% f-MXene-GO/EAA coated layer showed a very low H2 permeability coefficient (0.03 cc.mm.m−2.d−1.atm−1) and a significant 89% reduction in the H2 gas transmission rate with respect to nylon 6 (Seo, et al., 2021).
In this study, GO was first modified and treated with dopamine hydrochloride, then used borate ions to cross-link WPU and DGO, which enhanced the interfacial adhesion. Bio-inspired cross-linking networks were produced, and then improved the barrier properties of WPU/DGO composites. A simple technique that combines solution blending and cross-linking methods were used to prepare WPU/BA/DGO composite films as shown in Figure 1.
Experiments
Materials
Graphite, boric acid, dopamine hydrochloride and potassium permanganate were purchased from Aladdin. Sulfuric acid (H2SO4, AR), phosphoric acid (H3PO4, AR), hydrochloric acid (HCl, AR) and triethylamine hydrogen peroxide (AR, 99%, Macklin Biochemical Co. Ltd) were used without any treatment. The type of waterborne polyurethane is 1721 (The shi bang, Wenzhou). Other materials were used directly without further treatment.
Synthesis of DGO
GO was prepared by the modified Hummers method. 3 g of graphite and 18 g of potassium permanganate were stirred in a three-mouth flask, and the mixture of concentrated sulfuric acid and concentrated phosphoric acid (volume ratio 360 ml: 40 ml) were slowly added to the reaction bulb. All reagents were stirred vigorously at 50°C for 12 h. After cooling, the mixture was slowly poured into the ice frozen with 400 ml deionized water, stirring until all dissolved, then 22 ml of hydrogen peroxide and 400 ml of 0.5 vol% hydrochloric acid was added. The mixture was left overnight to remove the supernatant and wash with deionized water several times. A brown-yellow gel was obtained after centrifugation at 8000 rpm for 30 min. And GO solid was obtained after freeze-drying.
GO was evenly dispersed in an aqueous solution and added 100 ml PH = 8.5 10 mM Tris-HCl buffer solution. After fully stirring, an aqueous dopamine hydrochloride solution was slowly added and the reaction was carried out for 24 h. The resulting solution was centrifuged at 15000 rpm for 3 times to obtain the lower solid. The GO modified by dopamine was obtained after freeze-drying, which was denoted as DGO.
Synthesis of WPU/BA/DGO
Preparation of WPU/DGO blend and WPU/BA/DGO blend: DGO of 0.1wt%, 0.5wt%, 1wt%, and 2wt%, respectively was added in the WPU (solid content 30%), stirring at 70°C for 6 h. All mixtures were put in the refrigerator after freeze-drying, and then further hot-pressing film for characterization. At the same time, 7 g WPU (solid content 30%) was immersed in polyurethane film with 2wt% DGO, 0.5 mg/ml boric acid solution and 1 mg/ml boric acid solution, respectively. The surface was cleaned with deionized water and then put into the oven at 50°C to dry.
Characterizations
The infrared test of the polyurethane film adopts the total reflection mode, the test wave number range is 4000 cm−1−500 cm−1, the resolution is 4 cm−1, and the instrument is Vertex 70 of Bruker Company, Germany. GO and dopamine-modified graphite oxide powders were tested by an X-ray diffractometer (model D8Advance, Bruker, Germany) with a wavelength of 1.54 angstroms, Cu target, and test Angle of 1°–40°. The dried naturally formed graphene oxide film was used as the test sample. We use TEM (JOEL-1400, Japan) to observe the microstructure and morphology of GO and DGO. The instrument model is Thermo ESCALAB 250 electron spectrometer. The sample was prepared with a concentration of 0.01 mg/ml of GO deionized aqueous solution, which was drip-coated on a quartz sheet, dried to form a film, and AFM (Bruker MultiMode-V) was tested tapped mode with MikroMasch Tap probe (NAC15/ALBS). The blend sheet was quenched in liquid nitrogen and after drying, the cross-section was sprayed with gold. SEM (JAK-840ofJEOL, Japan) was used to observe the cross-section morphology of the samples. Stress-strain testing was performed using a universal tensile testing machine (Instron 1121). The samples were cut into dumbbell-shaped splines with a standard cutter, and the tensile rate was 50 mm/min, and each sample was tested at least 5 times. The gas permeability coefficient of the film was measured by a gas permeability meter (Labthink VAC-V2). According to GB 1038-2000. Three parallel samples were tested for each sample.
Results and discussions
Chemical structure of DGO
The FTIR spectra of GO and DGO are shown in Figure 2A. 3000 cm−1−3500 cm−1 is the stretching vibration peak of the hydroxyl group (O-H) on the GO surface, 1730 cm−1 is the stretching vibration peak of the carbonyl group, 1620 cm−1 is the vibration peak of the C=C bond, and 1048 cm−1 is the stretching vibration peak of C-O bond. The comparison between the two shows that the N-H bending vibration peak appears at 1500 cm−1, the C-N vibration peak appears at 1379 cm−1, and the disappearance of the carb-based peak at 1734cm−1 indicates that dopamine is successfully grafted to GO. To further demonstrate that polydopamine coats the GO surface, XPS characterization was performed. Figures 2C,D shows the C1s spectra of GO and DGO. As can be seen from the figure, the C-O peak at 286.7 eV has a significant decrease, while a new C-N peak appears at 285.5 eV, which proves that polydopamine is grafted on DGO lamellae and contains amino groups (Ni, et al., 2010).
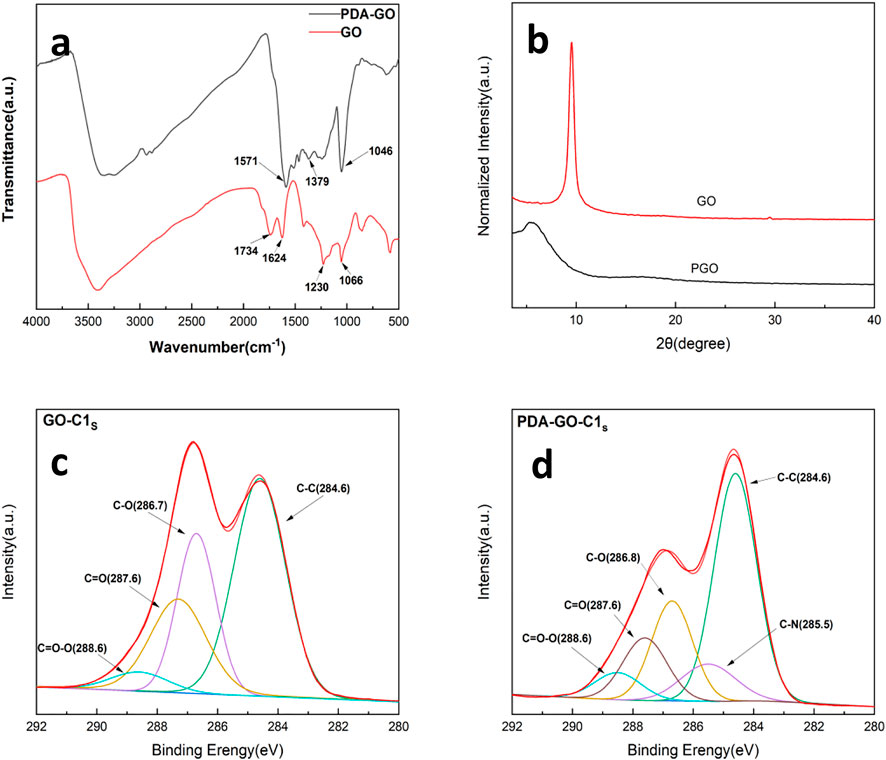
FIGURE 2. (A) FT-IR spectra of GO and DGO, (B) XRD spectra of GO and DGO, XPS C1s spectra of (C) GO and (D) DGO.
XRD is an effective method to study the spacing of nano lamellae. It can be judged by the change of diffraction angle corresponding to the change of the crystal plane spacing. The Figure 2B shows the WAXD spectra of GO and DGO. According to the Bragg equation: 2d sinθ = nλ (λ = 0.154 nm, n = 1), the layer spacing of graphite oxide is 0.90 nm. The diffraction peak of DGO shifted to a low Angle and was located at 5.2°. The DGO layer spacing is 1.6 nm. This is due to the fact that the polydopamine coating limits the aggregation of graphite oxide lamellae and therefore increases the graphite oxide lamellae spacing.
AFM was used to characterize the morphology and thickness of synthesized GO and DGO. The Figure 3 shows the morphology and height of the lamellae of GO and DGO. The thickness of the prepared GO lamellae is 1.48 nm and the roughness is 0.214. The layer thickness of the synthesized DGO is 1.52 nm and the roughness is 3.535. The thickness of the material increases slightly and the roughness increases significantly at the same time. The AFM results successfully demonstrated the synthesis of GO lamellae and DGO lamellae.
Chemical structure of WPU/BA/DGO
The FTIR spectra of WPU and WPU/DGO/BA are shown in Figure 4. It can be seen that the stretching vibration peak of O-H and the peak of hydrogen bond appear in 3000cm−1−3500 cm−1 (Mondal, et al., 2005; Li, et al., 2011). The peaks at 1411 cm−1 and 1085 cm−1 are the bending vibration peaks of B-O-C, which indicates that surface boric acid successfully cross-linked polydopamine and polyurethane on DGO.
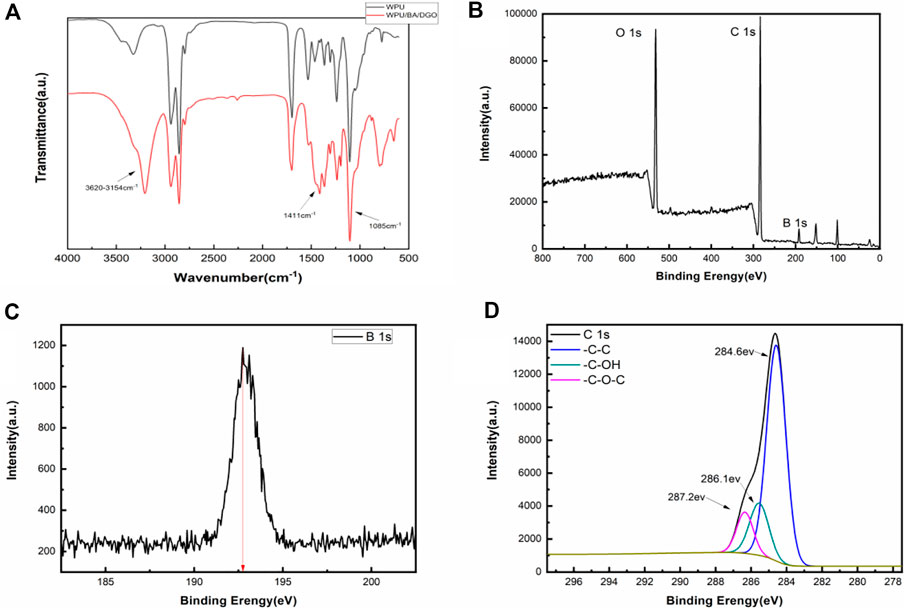
FIGURE 4. (A) FT-IR spectra of WPU and WPU/BA/DGO, (B) XPS spectra of WPU/BA/DGO, (C) XPS B1s spectra of WPU/BA/DGO, (D) XPS C1s spectra of WPU/BA/DGO.
In order to further verify the cross-linking of boric acid in modified GO and WPU, the XPS test was performed on the material. The Figure 4B shows the XPS spectra of WPU/BA/DGO samples. As can be seen from the Figures 4A,C new characteristic peak B1s appears at 193 eV, and the peak of C1s is divided as shown in the Figure 4D. It can be seen that the C-O bond of the material is significantly reduced, which demonstrated boric acid has been successfully cross-linked to modify GO.
Morphology of WPU/BA/DGO
The TEM images of GO and DGO are shown in Figure 5. By comparison, it can be seen that the thickness of GO has significantly increased and the surface roughness has significantly changed, proving that polydopamine was grafted on the surface of GO. As can be seen from the Figure 5, with increasing in the amount of GO, the defects and gullies of the section also increased significantly. Moreover, there was no aggregation phenomenon until the solid content reached 2%. After cross-linking with boric acid, it can be seen that the defect of the membrane section has been significantly reduced and even disappeared. This is due to the reaction of boric acid with dopamine on GO while forming hydrogen bonds with polyurethane to form a cross-linking network.
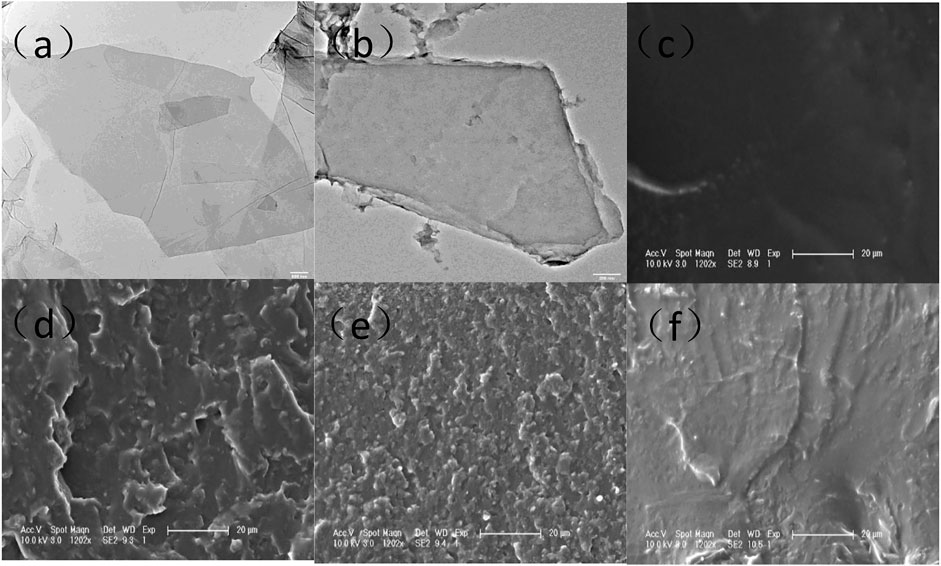
FIGURE 5. TEM spectra of (A) GO and (B) DGO, (C–F) TEM spectra of WPU, WPU/0.5% DGO, WPU/2% DGO, and WPU/BA/2% DGO.
Helium permeability and mechanical properties of WPU/BA/DGO
The tensile strength, tensile modulus, elongation at break with content of WPU/DGO and WPU/BA/DGO films are shown in Figure 6A; Table 1. With the increase of DGO, the tensile strength and modulus of the material increase. After boric acid was introduced, the tensile strength increased to 34.2 MPa and the tensile modulus increased to 93.7 MPa. Because there is a strong hydrogen bonding interaction between DGO and the hard segment of WPU, the addition of boric acid further enhances the hydrogen bond interaction and cross-links the whole network.
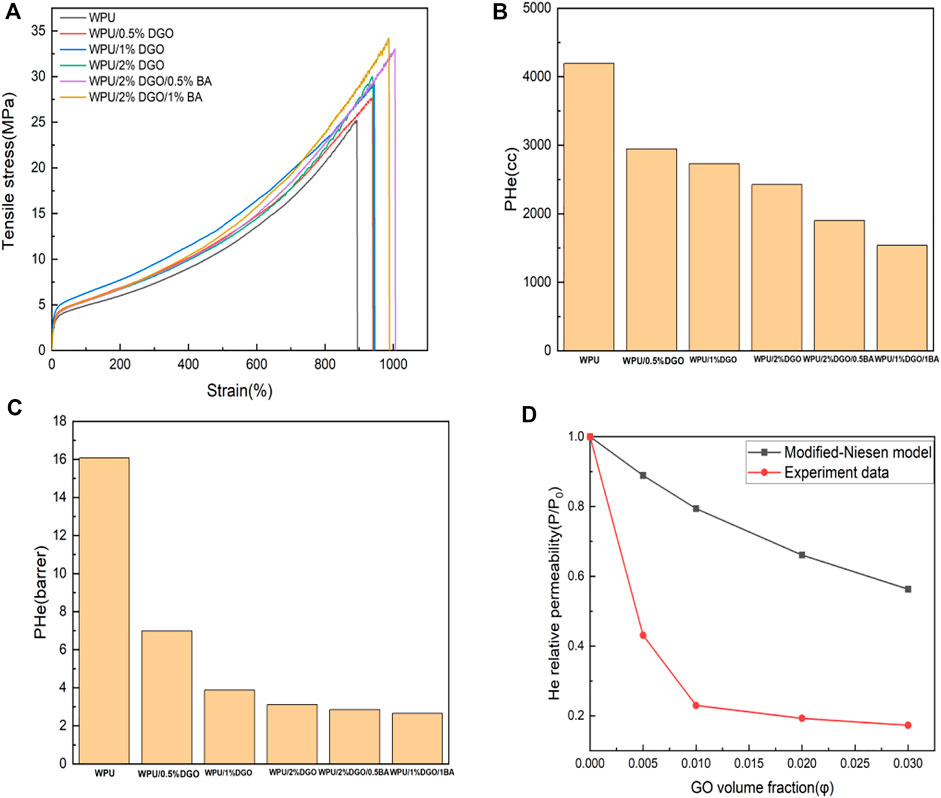
FIGURE 6. (A)Stress-strain curve for WPU and its composite, (B) He permeability map of the composite, (C) Helium permeability plot of the composite, (D) Comparison of model formula and experimental data.
As can be seen from the Figures 6B,C, along with the increase of the content of DGO, WPU relative helium permeability coefficient decreases gradually. When the content of DGO is above 2 wt%, the blend of the relative coefficient of permeability of helium into balance. This is likely to be higher DGO gathered in the WPU matrix, prompting gases to trap properties of the blend is not increased with the increase of nanofiller content. When boric acid content increases, boric acid reacts with modified GO and forms hydrogen bonds with polyurethane at the same time. The path of gas through the material is extended, thus increasing the gas barrier ability of the material. (Choudalakis, et al., 2009; Liu, et al., 2016; Choi, et al., 2017). The permeability of the material decreased from 4198.3 to 1538.6, while the permeability coefficient decreased from 16.0 to 2.7. Helium permeability was reduced by 85% (Figures 6B,C).
The gas barrier properties of PU/DGO can be described by the Nielsen model, which takes into account the dispersion and orientation of the modified graphene sheets in the matrix (Yang, et al., 2013):
The gas permeability of P and P0 blends and polymers, respectively; φ is the volume fraction of nano-filled particles; α = L/W is the specific surface area of the lamellae; S′ stands for orientation. When the lamellae are parallel to the direction of gas penetration, S′ = −0.5, S′ = 1 when the lamellae are perpendicular to the direction of gas penetration, and S′ = 0 when the lamellae are disordered (Layek, et al., 2014) (Wang, et al., 2016).
The width ratio α measured by AFM is about 270, and the modified Nielsen model is:
By comparing the data obtained with the pseudo-cooperation diagram, it can be seen that the introduction of boric acid makes the two-dimensional materials orderly arranged in the polyurethane matrix, and then makes the path length of the gas through the material, and the gas barrier ability of the material is enhanced. As shown in Figure 6D; Tables 2, 3 The principle of the gas barrier is shown in the Figure 7. When there is no filler in the matrix, the gas has the shortest path through the material vertically. When fillers are randomly distributed in the matrix, the path of the gas is slightly prolonged. When the filling in the matrix is orderly and parallel to the direction of the gas, the path taken by the gas increases greatly, and the barrier performance of the material also significantly increases.
Conclusion
In this work, GO was prepared by the modified Hummers method and then was modified by dopamine. The WPU/DGO and WPU/BA/DGO nano blends were prepared by the solution blending method. The dispersion, gas barrier, and mechanical properties of DGO and boric acid in the WPU matrix were studied. SEM results showed that the dispersion of DGO in the WPU matrix was better with the introduction of boric acid. This is mainly due to the strong hydrogen bond interaction between DGO and the hard segment of WPU, and the addition of boric acid further enhances the hydrogen bond interaction and cross-links the whole network. The results of the gas permeability experiment show that with the increase of GO and DGO content, the gas barrier performance of WPU improves. When the content of GO and DGO is 2 wt%, the permeability of WPU decreases from 4198.3 to 1538.6, and the permeability coefficient decreases from 16.0 to 2.7. Helium permeability was reduced by 85%. The addition of BA and DGO promote the tensile strength and modulus of the blend. Due to the strong hydrogen bond between DGO and TPU hard segment, the WPU/BA/DGO blend exhibits high tensile strength and tensile modulus. In essence, the study revealed that dopamine modified GO or other high aspect ratio nanofiller would be promising for aircrafts and food packaging. Seo and Saha, 2021.
Data availability statement
The raw data supporting the conclusions of this article will be made available by the authors, without undue reservation.
Author contributions
All authors listed have made a substantial, direct, and intellectual contribution to the work and approved it for publication.
Acknowledgments
The authors gratefully acknowledge the National Natural Science Foundation of China (Grant No. 22175171).
Conflict of interest
The authors declare that the research was conducted in the absence of any commercial or financial relationships that could be construed as a potential conflict of interest.
Publisher’s note
All claims expressed in this article are solely those of the authors and do not necessarily represent those of their affiliated organizations, or those of the publisher, the editors and the reviewers. Any product that may be evaluated in this article, or claim that may be made by its manufacturer, is not guaranteed or endorsed by the publisher.
References
Bandyopadhyay, P., Nguyen, T. T., et al. (2017). Enhanced hydrogen gas barrier performance of diaminoalkane functionalized stitched graphene oxide/polyurethane composites. J}, Compos. Part B Eng. 117, 101–110. doi:10.1016/j.compositesb.2017.02.035
Choi, D.-w., Park, H., et al. (2017). Three-dimensionally stacked Al2O3/graphene oxide for gas barrier applications{J. Carbon 125, 464–471. doi:10.1016/j.carbon.2017.09.061
Choudalakis, G., and Gotsis, A. D. (2009). Permeability of polymer/clay nanocomposites: A review{J. Eur. Polym. J. 454, 967–984. doi:10.1016/j.eurpolymj.2009.01.027
Ding, Y., Weng, L. T., et al. (2014). Insights into the aggregation/deposition and structure of a polydopamine film{J. Langmuir 3041, 12258–12269. doi:10.1021/la5026608
Gómez, J., Recio, I., et al. (2018). Processing influence on dielectric, mechanical, and electrical properties of reduced graphene oxide–TPU nanocomposites{J. J. Appl. Polym. Sci. 13612. doi:10.1002/app.47220
Jiang, J., Zhu, L., et al. (2011). Surface characteristics of a self-polymerized dopamine coating deposited on hydrophobic polymer films{J. Langmuir 2723, 14180–14187. doi:10.1021/la202877k
Kim, D. W., Kim, H., et al. (2019). Impermeable gas barrier coating by facilitated diffusion of ethylenediamine through graphene oxide liquid crystals. J}, Carbon 148, 28–35. doi:10.1016/j.carbon.2019.03.039
Kim, H., Miura, Y., et al. (2010). Graphene/polyurethane nanocomposites for improved gas barrier and electrical conductivity. J}, Chem. Mater. 2211, 3441–3450. doi:10.1021/cm100477v
Layek, R. K., Das, A. K., et al. (2014). Layer-structured graphene oxide/polyvinyl alcohol nanocomposites: Dramatic enhancement of hydrogen gas barrier properties{J. J. Mater. Chem. A 231. doi:10.1039/c4ta02346c
Li, B., Liu, W., et al. (2009). Ultrathin and stable active layer of dense composite membrane enabled by poly(dopamine){J. Langmuir 2513, 7368–7374. doi:10.1021/la900262p
Li, W., Tang, X.-Z., et al. (2011). Simultaneous surface functionalization and reduction of graphene oxide with octadecylamine for electrically conductive polystyrene composites. J}, Carbon 4914, 4724–4730. doi:10.1016/j.carbon.2011.06.077
Li, X., Bandyopadhyay, P., et al. (2018). Enhanced gas barrier and anticorrosion performance of boric acid induced cross-linked poly(vinyl alcohol-co-ethylene)/graphene oxide film. J}, Carbon 133, 150–161. doi:10.1016/j.carbon.2018.03.036
Liu, H., Bandyopadhyay, P., et al. (2016). Surface modified graphene oxide/poly(vinyl alcohol) composite for enhanced hydrogen gas barrier film{J. Polym. Test. 50, 49–56. doi:10.1016/j.polymertesting.2015.12.007
Ma, J., Pan, J., et al. (2018). High performance of poly(dopamine)-functionalized graphene oxide/poly(vinyl alcohol) nanocomposites. J}, Appl. Surf. Sci. 427, 428–436. doi:10.1016/j.apsusc.2017.07.040
Mondal, S., and Banthia, A. K. (2005). Low-temperature synthetic route for boron carbide{J. J. Eur. Ceram. Soc. 252-3, 287–291. doi:10.1016/j.jeurceramsoc.2004.08.011
Moon, I. K., Lee, J., et al. (2010). Reduced graphene oxide by chemical graphitization{J. Nat. Commun. 1, 73. doi:10.1038/ncomms1067
Ni, Z., Wang, Y., et al. (2010). Raman spectroscopy and imaging of graphene{J. Nano Res. 14, 273–291. doi:10.1007/s12274-008-8036-1
Qin, S., Pour, M. G., et al. (2018). Super gas barrier and fire resistance of nanoplatelet/nanofibril multilayer thin films. J}, Adv. Mater. Interfaces 62. doi:10.1002/admi.201801424
Ramezanzadeh, B., Ghasemi, E., et al. (2015). Covalently-grafted graphene oxide nanosheets to improve barrier and corrosion protection properties of polyurethane coatings{J. Carbon 93, 555–573. doi:10.1016/j.carbon.2015.05.094
Seo, O. B., Saha, S., et al. (2021). Preparation of functionalized MXene-stitched-graphene oxide/poly (ethylene-co-acrylic acid) nanocomposite with enhanced hydrogen gas barrier properties{J. J. Membr. Sci. 640. doi:10.1016/j.memsci.2021.119839
Wang, H., Zhang, H., et al. (2016). Structure and properties of the poly(vinyl alcohol-co-ethylene)/montmorillonite-phosphorylated soybean protein isolate barrier film{J. RSC Adv. 635, 29294–29302. doi:10.1039/c6ra03158g
Wang, J., Hou, L. a., et al. (2018). Polydopamine nanocluster decorated electrospun nanofibrous membrane for separation of oil/water emulsions{J. J. Membr. Sci. 547, 156–162. doi:10.1016/j.memsci.2017.10.028
Yan, X., Tao, W., et al. (2020). Layer-by-layer assembly of bio-inspired borate/graphene oxide membranes for dye removal{J. Chemosphere 256, 127118. doi:10.1016/j.chemosphere.2020.127118
Yang, H.-C., Liao, K.-J., et al. (2014). Mussel-inspired modification of a polymer membrane for ultra-high water permeability and oil-in-water emulsion separation{J. J. Mater. Chem. A 226, 10225–10230. doi:10.1039/c4ta00143e
Yang, Y. H., Bolling, L., et al. (2013). Super gas barrier and selectivity of graphene oxide-polymer multilayer thin films. J}, Adv. Mater. 254, 503–508. doi:10.1002/adma.201202951
Zhang, R.-X., Braeken, L., et al. (2013). Novel binding procedure of TiO2 nanoparticles to thin film composite membranes via self-polymerized polydopamine{J. J. Membr. Sci. 437, 179–188. doi:10.1016/j.memsci.2013.02.059
Zhang, Y., Tian, J., et al. (2018). Thin nacre-biomimetic coating with super-anticorrosion performance{J. ACS Nano 1210, 10189–10200. doi:10.1021/acsnano.8b05183
Keywords: waterborne polyurethane, boric acid, cross-linked, helium permeability, gas
Citation: Tian X, Sun Y, Xie H, Shi B, Zhong J, Sheng D and Yang Y (2022) Preparation of graphene oxide/ waterborne polyurethane via boric acid cross-linked dopamine enhanced barrier and mechanical properties. Front. Mater. 9:1046125. doi: 10.3389/fmats.2022.1046125
Received: 16 September 2022; Accepted: 17 November 2022;
Published: 15 December 2022.
Edited by:
Pingan Song, University of Southern Queensland, AustraliaReviewed by:
Xugang Dang, Shaanxi University of Science and Technology, ChinaChaoqun Zhang, South China Agricultural University, China
Copyright © 2022 Tian, Sun, Xie, Shi, Zhong, Sheng and Yang. This is an open-access article distributed under the terms of the Creative Commons Attribution License (CC BY). The use, distribution or reproduction in other forums is permitted, provided the original author(s) and the copyright owner(s) are credited and that the original publication in this journal is cited, in accordance with accepted academic practice. No use, distribution or reproduction is permitted which does not comply with these terms.
*Correspondence: Dekun Sheng, dksheng@ciac.ac.cn; Yuming Yang, ymyang@ciac.ac.cn