- 1University of Science and Technology of China, Hefei, China
- 2CAS Key Laboratory of High-Performance Synthetic Rubber and Its Composite Materials, Changchun Institute of Applied Chemistry, Chinese Academy of Sciences, Changchun, China
Polycarbonate-based polyurethanes (PCU) are frequently used in airship envelope materials because of its outstanding mechanical performances and aging resistance. However, the surface of PCU is likely damaged during processing and operating and the emerged minuscule cracks will lead to deterioration of perfomances for airship’s envelope materials. Herein, self-healing PCU/polypyrrole nanoparticles (PPy) composites were prepared by solution blending and quick healing of specific area for PCU was realized due to the high photo-thermal conversion merit of PPy. The results show that, the mechanical properties of PCU/PPy composites can be restored to more than 80% and the gas barrier properties can also be basically repaired when irradiating the destroyed surface using near-infrared light for only 60s. In addition, the ultraviolet visible (UV-vis) shielding performance of the PCU/PPy composites was enhanced significantly and the UV-vis transmittance was less than 14% and 2% with 0.25wt% and 0.5wt% PPy, respectively. Meanwhile when 0.25wt% PPy was added, the tensile strength increased from 17.9MPa to 21.7 MPa and the elongation at break increased from 647% to 829%. Besides, the thermal decomposition temperature at 5wt% loss increased from 277.8°C to 300.7°C and 304.88°C with 0.25 wt% and 0.5 wt% PPy, respectively. The prepared composites show promising application in aerospace domain.
1 Introduction
Polyurethane is a kind of elastomer materials whose chemical structure can be flexibly adjusted according to the requirements of use (Jiang et al., 2021). It is a commonly used polymer for envelope materials of airship owing to the advantages of excellent ozone resistance, low temperature adaptability, chemical resistance and toughness (Xie et al., 2019; Li et al., 2021; Mandlekar et al., 2022). It is inevitable to avoid the emergence of quantity folds and even micropores or minuscule cracks on envelope materials during the processing or transportation, which results in a significant reduction of the gas-barrier performance of the airship. Moreover, the aging process of the multi-folded part will be obvious accelerated compared with the normal part, which seriously shortens the service life (Marcano et al., 2019). However, the big size of airship makes it almost impossible to carry out subsequent repairing work if the envelope materials suffering damage. Therefore, polyurethane with self-healing function is favored to maintain the barrier and anti-aging properties without affecting the aging properties. Once the polymer is damaged, a simple process can realize self-healing, which could improve the safety and save the maintenance cost and thereby prolong the lifetime of materials (Hornat and Urban et al., 2020; Eom et al., 2021; James et al., 2021; Wang et al., 2022; Pan et al., 2022).
Most self-healing polymers require additional stimuli to trigger repairing, such as light, heat, electricity and microwave etc (Burnworth et al., 2011; Amamoto et al., 2012; Tee et al., 2012; Wang et al., 2020; Yu et al., 2021; Irez et al., 2022). Amongst, as an environmental-friendly energy, light initiation can realize the self-healing of specific area through remote site-direction without contact, which could avoid the extra damage to other intact area which are probably brought via heat or microwave. Typically, there are nanoparticles with excellent photo-thermal conversion distributing in the matrix, which could absorb light efficiently and serve as nano-heater to elevate the temperature of polymer. At this case, polymer chains could move and rearrange to fill the damaged area. Most used photo-thermal converters include gold nanoparticles (Zhang et al., 2021), silver nanowires, graphene oxide (Liu et al., 2022), carbon nanotubes (Kausar, 2021) etc. Compared with these mentioned above, polypyrrole (PPy) is a kind of conjugated polymer with the merits of easy preparation, adjustable particle size and excellent photo-thermal properties (Dong et al., 2021; Sander and Ferreira, 2018; Zhao et al., 2021; Zha et al., 2013a; Zare et al., 2021). It has been widely used in photo controlled tumor drugs (Phan et al., 2018). Zha et al. prepared PPy nanoparticles with uniform particle size by one-step water dispersion method. The PPy nanoparticles obtained exhibit significant photo-thermal conversion efficiency, which is superior to the known gold nanorods (Zha et al., 2013b). Zeng et al. used PPy to prepare antitumor drugs with good photo-thermal conversion efficiency under near-infrared (NIR) irradiation, which can effectively remove tumors (Chen et al., 2019). Yang groups synthesized thermoplastic polyurethanes (TPU) using polycaprolactone diol and polytetrahydrofuran as soft segment, and developed a NIR induced self-healing TPU/PPy nanocomposites with rapid repair performance (Wu et al., 2020).
In this paper, we introduced PPy into the polycarbonate-based polyurethanes (PCU) to prepare airship envelope materials used self-healing PCU/PPy composites by solution blend. The result shows that the addition of PPy not only endows composite with the ability of quick repair under NIR irradiation, but also improves the ultraviolet visible (UV-vis) shielding performance. The mechanical and gas-barrier properties could recover after irradiation and the thermal stability gets improved substantially, which has a broad application prospect in airship envelope materials and other fields.
2 Experimental
2.1 Materials
Polycarbonate diol (PCDL) with Mn ≈ 2000g/mol was purchased from UBE Corporation and was dried in vacuum oven at 100°C for 2 h before using. Dicyclohexylmethane-4,4′-diisocyanate (HMDI, 99%), dibutyltin dilaurate (DBTDL, 95%),1,4-butanediol (BDO, 99.5%), pyrrole (Py) and iron chloride hexahydrate (FeCl₃·6H₂O, 99%) were all purchased from Aladdin Biochemical Technology Co., Ltd. Polyvinyl alcohol (PVA, Mn=1750 ± 50, ≥99.0%) was purchased from Sinopharm Chemical Reagent Co., Ltd. N,N-Dimethylformamide (DMF, stored on molecular sieves) was purchased from Energy Chemicals Co., Ltd.
2.2 Preparation of PCU
PCU was synthesized by two-step method with DMF as the solvent (Figure 1). First, HMDI (5.24g) was put into a dry flask and stirred in oil bath at 80°C. PCDL (20g) and DBTDL (0.01wt% of PCU) dissolved in DMF were added dropwise to the flask and stirred under Ar atmosphere at 80°C for 2 h. During the reaction, the content of -NCO was monitored by chemical titration (toluene di-n-butylamine titration). After prepolymerization reaction, BDO was added to the reactor for chain extension. When the reaction was over, the mixture was put into a Teflon mould and dried in vacuum at 60°C for 24 h to obtain the final product PCU.
2.3 Preparation of PPy nanoparticles
PPy nanoparticles were prepared from PVA, FeCl3·6H2O, and pyrrole in deionized water according to the literature (Hong et al., 2010). Typically, PVA was added into deionized water to make PVA aqueous solution with a concentration of about 1.0wt%, which was stirred in an oil bath at 80°C to dissolve it fully. After dissolution of PVA in deionized water, FeCl3·6H2O was added into the PVA aqueous solution. The molar ratio of FeCl3·6H2O to pyrrole was 2.3. Then the PVA aqueous solution was cooled in an ice bath. Pyrrole was added to the PVA aqueous solution drop by drop and stirred continuously for 8 h. After completion of polymerization, the resulting nanoparticles were separated from the dispersion solution by centrifugation (15000rpm, 30min) and washed with deionized water repeatedly to remove impurities. PPy nanoparticles could be obtained after freeze-drying for more than 48 h.
2.4 Preparation of PCU/PPy nanocomposites
PCU/PPy nanocomposites were prepared by solution blending. A certain amount of PCU and PPy (0.1, 0.25, 0.5, and 1.0wt% of PCU) was dispersed in DMF. The mixture was ultrasonic dispersed for 1h to make the dispersion of PPy nanoparticles more uniform. After pouring the mixture into the Teflon mould and vacuum drying at 60°C for 48 h, PCU/PPy-x (x represents the wt% of PPy added to PCU) nanocomposites film could be obtained.
3 Characterization
The FTIR spectra were obtained using Vertex 70 (Bruker, Germany) in the range of 4000-500cm− 1 with a resolution of 4cm−1.
1H NMR spectra were recorded on a spectrometer (Unity Inova, 400 MHz, United States) using DMSO-d6 as the solvent, Bruker AV.
The molecular weight and polydispersity index (PDI) of polymers were determined using gel permeation chromatography (GPC) instrument (Polymer Laboratories, PL-GPC120, United Kingdom).
Thermo gravimetric analysis (TGA, Mettler Toledo, Switzerland) was used to demonstrate the thermal stability of nanocomposites. The test was carried out from 25°C to 600°C at a heating rate of 10°C/min under an N2 atmosphere.
Scanning electron microscopy (SEM, XL-30 ESEM FEG, FEI Company) was used to observe the morphology of PPy nanoparticles and the fracture surface of PCU/PPy nanocomposites.
The laser system (LSR808H–5W-FA) with a NIR energy source (808 nm) was applied for investigating the NIR light-responsive of the synthesized composites. The optical microscope images of self-healing process were analyzed by polarizing microscope (Leica, DM2500P)
Ultraviolet-visible-near infrared spectrophotometer (PerkinElmer LAMBDA 1050) was used to demonstrate the UV-vis transmittance of PCU/PPy nanocomposites.
Helium permeability of samples was tested at room temperature using VAC-V2 gas permeameter (Languang, Jinan). Helium permeability was measured by differential-pressure method according to GB/T 1038–2000.
According to GB/T 1040.3–2006, the tensile and self-healing tests of the nanocomposites were used by the universal testing machine (Instron 1121) at a strain rate of 200mm/min−1. The dimensions of the tensile test samples were 50mm×4mm×0.5 mm. The tensile test samples were the same as the self-healing samples. At least five specimens of each sample were tested and the result for each sample corresponded to the average of five test results.
Self-healing properties were evaluated by comparison the tensile strength of samples before and after self-healing. Each sample was cut into two parts, each part was spliced together and irradiated under 808 nm NIR light for a period of time to realize self-healing. The sample was tested for ultimate tensile strength (σ). The self-healing efficiency was defined as follows:
4 Results and discussions
4.1 Structural characterization
FTIR spectroscopy was used to confirm whether the reaction was complete. As shown in Figure 2A, the peaks for PPy located at 1549, 1179, 1037, and 892cm−1 represented the characteristic peak of C-C, C-N, C-H, N-H, respectively. SEM was used to measure the size of PPy nanoparticles, as shown in Figures 2B,C. As can be seen, the prepared PPy nanoparticles displayed good uniformity and possessed a narrow particle size distribution, mainly between 75 and 85nm, with an average particle size of about 80.6 nm.
The molecular weight and PDI of PCU were determined by GPC: Mn=42548 g/mol, Mw=83991 g/mol, PDI=1.97. As shown in Figure 3, the characteristic peak of -NCO near 2270cm−1 was absent in PCU, which indicated that the polymerization reaction of PCU had been completed (Yang et al., 2017). FTIR spectra of PCU/PPy nanocomposites were also displayed in Figure 3. Compared to PCU/PPy-0, the characteristic peaks of C-O bonds of nanocomposites with PPy move to low wavenumbers. This may be due to the formation of intramolecular hydrogen bond between PPy and PCU. However, because the addition of PPy was less than 1.0wt%, PCU/PPy nanocomposites did not significantly change the characteristic peaks of PCU.
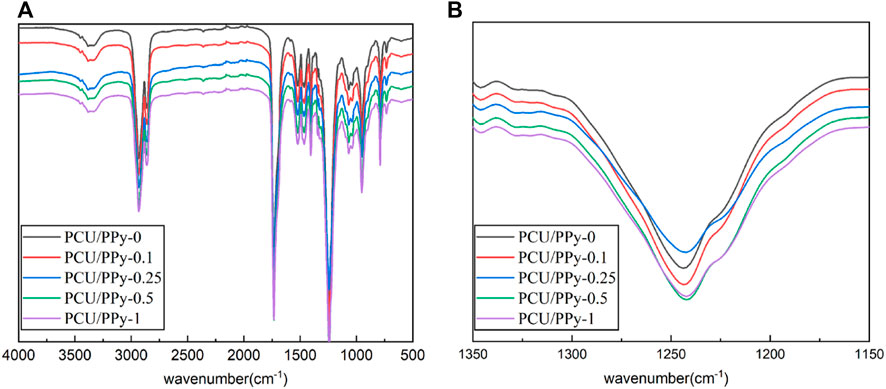
FIGURE 3. (A) FT-IR spectra of PCU/PPy nanocomposites (B) amplified spectra of PCU/PPy nanocomposites.
The 1HNMR spectrum of the above synthesized PCU was shown in Figure 4. The chemical shifts at 4.04ppm and 3.91ppm were attributed to methylene proton linked by ester oxygen and between two six-membered rings, respectively. The chemical shifts at 1.06–1.79ppm were attributed to protons on methylene proton from PCDL and BDO.
4.2 Morphology of PCU/PPy-x
In order to realize photo induced self-healing, the uniform dispersion of photo-thermal nanoparticles in the system was very important. Therefore, microscopic morphology of PCU/PPy nanocomposites was characterized by SEM to analyze the dispersion of PPy in polymer system. The fracture surface of samples was given in Figure 5. The surface of PCU/PPy-0 presented a smooth and flat morphology. When the content of PPy was 0.1wt%, there were basically no PPy particles on the surface due to the well dispersion in polymer matrix. However, with the increase of PPy content, the fracture surface of samples became rougher and PPy particles could be clearly seen on the polymer surface. Especially, when the content reached 1wt%, as show in Figures 5E,F, obvious aggregation phenomenon appeared on the polymer surface.
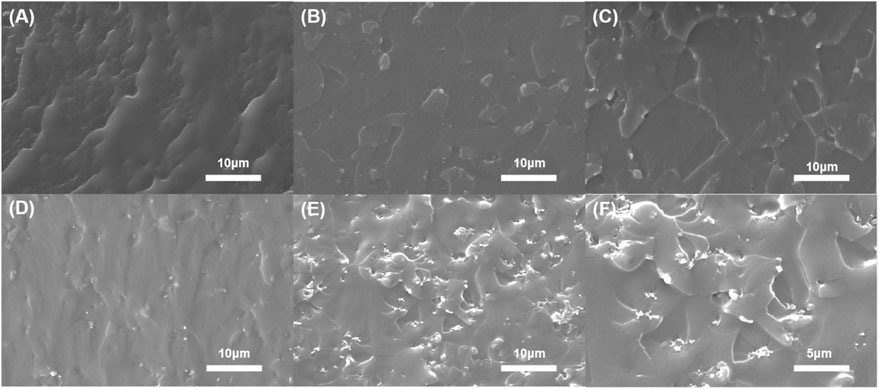
FIGURE 5. SEM images of (A) PCU/PPy-0, (B) PCU/PPy-0.1, (C) PCU/PPy-0.25, (D)PCU/PPy-0.5, (E) (F) PCU/PPy-1 (the scale bar of A,B,C,D,E,: 10 μm and the scale bar of F: 5 μm).
4.3 Mechanical properties and thermal properties of PCU/PPy
The mechanical properties of materials were directly related to their usability, so the effect of PPy on the mechanical properties of composites was analyzed by tensile testing. The results were illustrated in Figure 6 and Table 1. As listed, the tensile strength and elongation at break of PCU/PPy-0 were 17.9 MPa and 647%, respectively. With the increase of PPy addition, the mechanical properties of PCU/PPy nanocomposites gradually improved. The tensile strength and elongation at break of PCU/PPy-0.25 reached 21.7 ± 0.5MPa and 829 ± 75%, respectively. In view of this, the incorporation of PPy was helpful to improve the mechanical properties of PCU. The results were mainly attributed to the strong interaction between PPy and PCU. Many physical cross-linking points were formed in the PCU, which significantly improves the mechanical properties of nanocomposites. However, when the addition of PPy exceeded 0.5wt%, the tensile strength began to decrease from 21.7 ± 0.5MPa to 19.5 ± 1.0MPa, this may be due to the agglomeration effect caused by the excessive addition of PPy, resulting in the reduction of mechanical properties, which could refer to the SEM results.
Thermal stability was another critical parameter influencing the actual application of the composites. TGA was used to analyze the effect of PPy on the thermal stability of the composites. The results were shown in Figure 7 and Table 1. With the increasing dosage of PPy, the thermal properties of the composites had been significantly improved. The temperature at 5% weight loss (Td5%) of PCU/PPy-0.25 and PCU/PPy-1 were 300.72°C and 305.56°C, which was 22.92°C and 27.76°C higher than that of PCU/PPy-0. Besides, the temperature at maximum weight loss (Tdmax) of the materials got dramatic enhanced with about 40°C when adding 1wt% PPy. The reason for this phenomenon may be that the surface of PPy contains a large number of N–H, which can form hydrogen bonds with urethane and ester bonds in polyurethane, and the formation of this physical crosslinking can improve the thermal stability of the composite (Wen et al., 2001; Kotal et al., 2011; Yanilmaz and kalaoglu, 2012).
4.4 Photothermal properties of PCU/PPy
Nanoparticles with photo-thermal conversion properties can make the composite temperature rise and reach a higher temperature in a short time, so as to realize rapid self-healing. In order to study this performance, the sample was placed under an NIR laser light for irradiation. The thermocouples were used to record the temperature of the surface of the sample in real time to study the variation of the surface temperature for sample with the irradiation time and irradiation intensity.
The nanocomposites with different content of PPy were irradiated under the NIR light and the output power of NIR light was 1.1w/cm2. The result was shown in Figure 8. PCU/PPy-0 had almost no photo-absorbing properties. In comparison, the samples containing PPy could basically reach the equilibrium temperature within 60 s, and the equilibrium temperature that could be achieved was relatively higher. Among them, it took only 30s for PCU/PPy-0.5 to increase from room temperature to 100°C above, which indicated that the addition of PPy could significantly improve the heating rate of nanocomposites (Figure 8A). The heating rate and equilibrium temperature of NIR radiation responsive nanocomposites were not only related to the content of photothermal effect nanoparticles, but also depended on the output power of the light emitter. Here, PCU/PPy-0.5 was used as the sample to explore the effect of different radiation power on surface temperature. When the output power was 0.7w/cm2, the temperature of the sample surface was about 78°C after 90 s of irradiation. While the output power is 1.1w/cm2, the temperature of the sample surface could reach 118°C after the same time of irradiation (Figure 8B).
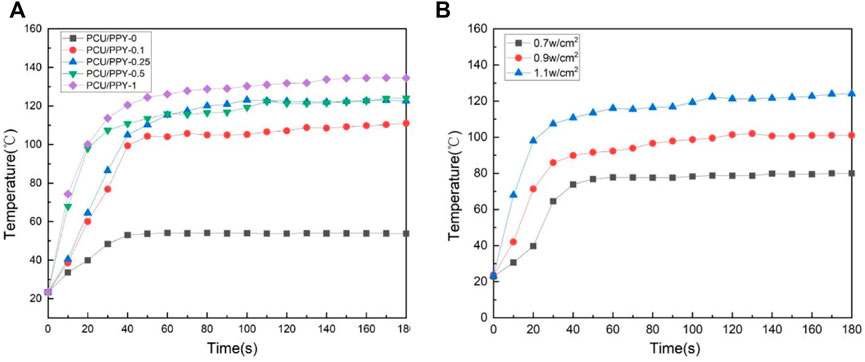
FIGURE 8. (A) Photothermal properties of PCU/PPy-x under NIR light (1.1w/cm2). (B) Photothermal properties of different output power.
4.5 Self-healing behavior of PCU/PPy
In order to study the self-healing behavior under NIR light, the helium permeability and mechanical performance of the PCU/PPy-x nanocomposites were research. The optical microscope images of the samples before and after self-healing were analyzed by polarizing microscope.
First, the circular samples with the diameter of about 97 mm and the thickness of about 180 μm were prepared and the helium permeability of the samples were tested and recorded. Then a puncture on the sample was stabbed with a fixed size needle (Figure 9). After that the sample was placed under NIR light (1.1W/cm2) for irradiation to conduct self-healing. After 60s irradiation, the sample was placed in air at room temperature for 48 h and the helium permeability was tested to evaluate the self-healing ability (Table 2).
The helium permeability of PCU/PPy-0 original sample was 2.65L/m2·24 h·atm. The gas barrier of PCU/PPy-0 sample after punching was broken. Even after 120s NIR light irradiation, the sample still exhibit leakage, which proved that PCU/PPy-0 did not realize self-healing. The helium permeability of PCU/ppy-0.25, PCU/ppy-0.5 and PCU/ppy-1 before and after irradiation were 1.74 and 2.14L/m2·24 h·atm, 1.65 and 1.85L/m2·24 h·atm, 2.27 and 2.44 L/m2·24 h·atm, respectively. Thus it can be seen, after NIR light irradiation, the helium permeability of nanocomposites containing PPy were close to the original sample, which implies that the gas barrier was basically restored. These results also proved that the nanocomposites containing PPy can realize self-healing after NIR light irradiation.
PCU/PPy-0.25 was chosen as the object sample and the process as well as mechanism of self-healing under NIR light was shown in Figure 9. As could be seen under the microscope, there was an obvious hole on the surface of the sample after puncturing. After 60s NIR light irradiation, the original hole had been completely repaired. This is all ascribed to the efficient light-thermal conversion of PPy. Upon irradiation, PPy nanoparticles could absorb light efficiently and transform it into heat, which made the temperature rise. With the increase of temperature, PCU chains could move, rearrange, entangle together and finally fill the hole. The filled state was fixed and the damaged hole was healed when cooled down.
To study the repair efficiency of the PCU/PPy nanocomposites, PCU/ppy-0.25 was chosen as the representative sample. First, dumbbell shaped samples were cut into two parts from the middle, and the two cut surfaces were brought back together. Soon after irradiating the fracture for tens of seconds, the sample was placed in air at room temperature for 48 h. Finally, tensile tests were carried out to evaluate the self-healing efficiency, which could be calculated by formula (1). The results are summerized in Figure 10 and Table 3. PCU/PPy-0 was hardly repaired under NIR light even for 120s. Apparently, the self-healing efficiency of PCU/PPy-0.25 increased from 80.7% to 89.4%, 89.6% and 90.9% with the prolongation of irradiation time from 30 s to 60s, 90s and 120 s. As illustrated in the former Figure 8, the temperature rose sharply to 100°C above within 60s and gradually got to a plateau. Hence the enhancement of self-healing efficiency of sample was limited when irradiation time exceeded to 60s. The quick repairing rate and contactless initiation of self-healing render PCU/PPy great potential in application of envelope materials for airship.
4.6 UV–vis shielding property of PCU/PPy
The UV-vis shielding property of the PCU/PPy nanocomposites was accessed by UV-vis spectroscopy and the results were demonstrated in Figure 11. PCU/PPy-0 exhibited high UV-vis transmittance clearly with a transmittance in the range of 200–320nm and 400–800 nm of about 6% and 91%, respectively. Menwhile the transmittance in the range of 320–380 nm of PCU/PPy-0 increased from 6% to 85%. However, with the increase of PPy content, the transmittance of nanocomposites in the UV-vis region decreased significantly. The transmittance of nanocomposites containing PPy were close to 0% in the range of 200–370 nm. In the range of 400–800nm, the transmittance of nanocomposites decreased gradually with the increasing dosage of PPy. For PCU/PPy-0.25, the transmittance of nanocomposites in the range of 400–800 nm was about 12%. When the addition of PPy exceeded 0.5wt%, the transmittance in the range of 400–800 nm was less than 2%. This implied that the prepared PCU/PPy could be candidate for weathering layer of envelope materials to protect the inner layer from UV damage.
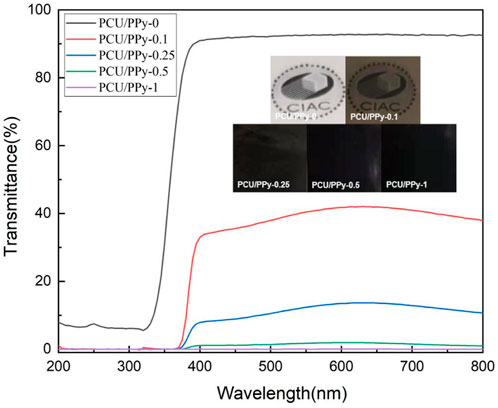
FIGURE 11. UV-vis light transmittance spectra of the PCU/PPy nanocomposites (Inserted is appearance of the PCU/PPy-x nanocomposites on the pattern).
To better show the light shielding properties of fabricated composites, photographs of samples covering the logo of CIAC were displayed in Figure 11 (inserted part). Obviously, the transparency of PCU/PPy decreased significantly with the increase of PPy content. For PCU/PPy-0, the logo of CIAC covered could also be clearly seen. Once the dosage of PPy exceeded to 0.5wt%, it was completely invisible to discovery the logo and the surface of sample became total black. This result was consistent with the UV-vis transmittance test.
5 Conclusion
In this article, the self-healing PCU/PPy composites were successfully fabricated by solution blending, which possessed rapid repair ability under NIR irradiation. Even though only 0.25wt% PPy was added, the mechanical properties of the intentionally damaged PCU/PPy composite could be restored to more than 80% and the gas barrier could also be almost repaired after only 60s NIR treatment. With the increase of PPy, the thermal stability got dramatic improvement with about 30 C and 40 C elevate of Td5% and Tdmax, respectively. Moreover, the UV-transmission of the PCU/PPy composite is less than 5% with 0.5wt% PPy. The PCU/PPy composite is suitable for high self-healing and aging resistance requirements applications such as airships and so on.
Data availability statement
The original contributions presented in the study are included in the article/supplementary material, further inquiries can be directed to the corresponding authors.
Author contributions
XZ carried out the experiment. XZ and ZY wrote the manuscript, ZH fabricated the PCU sample. YY helped supervise the project and conceived the original idea.
Funding
The authors gratefully acknowledge the National Natural Science Foundation of China (Grant No. 22175171).
Conflict of interest
The authors declare that the research was conducted in the absence of any commercial or financial relationships that could be construed as a potential conflict of interest.
Publisher’s note
All claims expressed in this article are solely those of the authors and do not necessarily represent those of their affiliated organizations, or those of the publisher, the editors and the reviewers. Any product that may be evaluated in this article, or claim that may be made by its manufacturer, is not guaranteed or endorsed by the publisher.
References
Amamoto, Y., Otsuka, H., Takahara, A., and Matyjaszewski, K. (2012). Self-healing of covalently cross-linked polymers by reshuffling thiuram disulfide moieties in air under visible light. Adv. Mat. 24, 3975–3980. doi:10.1002/adma.201201928
Burnworth, M., Tang, L., Kumpfer, J. R., Duncan, A. J., Beyer, F. L., Fiore, G. L., et al. (2011). Optically healable supramolecular polymers. Nature 472, 334–337. doi:10.1038/nature09963
Chen, J., Liu, J., Thundat, T., and Zeng, H. (2019). Polypyrrole-doped conductive supramolecular elastomer with stretchability, rapid self-healing, and adhesive property for flexible electronic sensors. ACS Appl. Mat. Interfaces 11, 18720–18729. doi:10.1021/acsami.9b03346
Dong, Y., Wu, K., Yin, Y., Geng, C., and Zhou, Q. (2021). Shape memory self-healing coating based on photothermal effect of PPy@PDA nanoparticles. Synth. Met. 280, 116869. doi:10.1016/j.synthmet.2021.116869
Eom, Y., Kim, S. M., Lee, M., Jeon, H., Park, J., Lee, E. S., et al. (2021). Mechano-responsive hydrogen-bonding array of thermoplastic polyurethane elastomer captures both strength and self-healing. Nat. Commun. 12, 621. doi:10.1038/s41467-021-20931-z
Hong, J. Y., Yoon, H., and Jang, J. (2010). Kinetic study of the formation of polypyrrole nanoparticles in water-soluble polymer/metal cation systems: A light-scattering analysis. Small 6, 679–686. doi:10.1002/smll.200902231
Hornat, C. C., and Urban, M. W. (2020). Shape memory effects in self-healing polymers. Prog. Polym. Sci. 102, 101208. doi:10.1016/j.progpolymsci.2020.101208
Irez, A. B., Okan, C., Kaya, R., and Cebe, E. (2022). Development of recycled disposable mask based polypropylene matrix composites: Microwave self-healing via graphene nanoplatelets. Sustain. Mater. Technol. 31, e00389. doi:10.1016/j.susmat.2022.e00389
James, E., Ellingford, C., Pan, M., Wemyss, A. M., Bowen, C., and Wan, C. (2021). Challenges and opportunities of self-healing polymers and devices for extreme and hostile environments. Adv. Mat. 33, 2008052. doi:10.1002/adma.202008052
Jia, P., Shi, Y., Song, F., Yu, B., Huang, C., Zhang, M., et al. (2022). Bio-based and degradable vitrimer-graphene/graphene oxide composites with self-healing ability stimulated by heat, electricity and microwave as temperature and fire warning sensors. Compos. Sci. Technol. 227, 109573. doi:10.1016/j.compscitech.2022.109573
Jiang, C., Zhang, L., Yang, Q., Huang, S., Shi, H., Long, Q., et al. (2021). Self-healing polyurethane-elastomer with mechanical tunability for multiple biomedical applications in vivo. Nat. Commun. 12, 4395. doi:10.1038/s41467-021-24680-x
Kausar, A. (2021). Self-healing polymer/carbon nanotube nanocomposite: A review. J. Plastic Film Sheeting 37 (2), 160–181. doi:10.1177/8756087920960195
Kotal, M., Srivastava, S. K., and Paramanik, B. (2011). Enhancements in conductivity and thermal stabilities of polypyrrole/polyurethane nanoblends. J. Phys. Chem. C 115 (5), 1496–1505. doi:10.1021/jp1081643
Li, X., Chen, X., Zhang, S., Yin, Y., and Wang, C. (2021). UV-resistant transparent lignin-based polyurethane elastomer with repeatable processing performance. Eur. Polym. J. 159, 110763. doi:10.1016/j.eurpolymj.2021.110763
Liu, Y., Mao, J., Guo, Z., Hu, Y., and Wang, S. (2022). Polyvinyl alcohol/carboxymethyl chitosan hydrogel loaded with silver nanoparticles exhibited antibacterial and self-healing properties. Int. J. Biol. Macromol. 220, 211–222. doi:10.1016/j.ijbiomac.2022.08.061
Mandlekar, N., Joshi, M., and Butola, B. S. (2022). A review on specialty elastomers based potential inflatable structures and applications. Adv. Industrial Eng. Polym. Res. 5, 33–45. doi:10.1016/j.aiepr.2021.05.004
Marcano, A., Fatyeyeva, K., Koun, M., Dubuis, P., Grimme, M., and Marais, S. (2019). Recent developments in the field of barrier and permeability properties of segmented polyurethane elastomers. Rev. Chem. Eng. 35 (4), 445–474. doi:10.1515/revce-2017-0033
Phan, T. T. V., Bui, N. Q., Cho, S. W., Bharathiraja, S., Manivasagan, P., Moorthy, M. S., et al. (2018). Photoacoustic imaging-guided photothermal therapy with tumor-targeting HA-FeOOH@PPy nanorods. Sci. Rep. 8, 8809. doi:10.1038/s41598-018-27204-8
Sander, M. M., and Ferreira, C. A. (2018). Synthesis and characterization of a conductive and self-healing composite. Synth. Met. 243, 58–66. doi:10.1016/j.synthmet.2018.06.003
Tee, B. K., Wang, C., Allen, R., and Bao, Z. (2012). An electrically and mechanically self-healing composite with pressure- and flexion-sensitive properties for electronic skin applications. Nat. Nanotechnol. 7, 825–832. doi:10.1038/nnano.2012.192
Wang, S., and Urban, M. W. (2020). Self-healing polymers. Nat. Rev. Mat. 5, 562–583. doi:10.1038/s41578-020-0202-4
Wang, Z., Gong, X. B., Wang, Y. F., Ge, X., and Xing, R. G. (2022). Self-healable recyclable thermoplastic polyurethane elastomers: Enabled by metal–ligand bonds between the cerium(III) triflate and phloretin. Chem. Eng. J. 446 (4), 137228. doi:10.1016/j.cej.2022.137228
Wang, Z., Zhou, J., Liang, H., Ye, S., Zou, J., and Yang, H. (2020). A novel polyurethane elastomer with super mechanical strength and excellent self-healing performance of wide scratches. Prog. Org. Coatings 149, 105943. doi:10.1016/j.porgcoat.2020.105943
Wen, T. C., Hung, S. L., and Digar, M. (2001). Effect of polypyrrole on the morphology and ionic conductivity of TPU electrolyte containing LiClO4. Synth. Met 118 (1–3), 11–18. doi:10.1016/S0379-6779(00)00272-1
Wu, H., Sheng, D., Liu, X., Zhou, Y., Dong, L., Ji, F., et al. (2020). NIR induced self-healing polyurethane/polypyrrole nanocomposites. Polymer 189, 122181. doi:10.1016/j.polymer.2020.122181
Xie, F., Zhang, T., Bryant, P., Kurusingal, V., Colwell, J. M., and Laycock, B. (2019). Degradation and stabilization of polyurethane elastomers. Prog. Polym. Sci. 90, 211–268. doi:10.1016/j.progpolymsci.2018.12.003
Yang, Y., Lu, X., and Wang, W. (2017). A tough polyurethane elastomer with self-healing ability. Mat. Des. 127, 30–36. doi:10.1016/j.matdes.2017.04.015
Yanilmaz, M., Kalaoglu, F., Karakas, H., and Sarac, A. S. (2012). Preparation and characterization of electrospun polyurethane–polypyrrole nanofibers and films. J. Appl. Polym. Sci. 125 (5), 4100–4108. doi:10.1002/app.36386
Yu, C., de Luna, M. S., Marotta, A., Ponti, C., Esposito, I., Scherillo, F., et al. (2021). NIR light-triggered self-healing waterborne polyurethane coatings with polydopamine-coated reduced graphene oxide nanoparticles. Prog. Org. Coatings 161, 106499. doi:10.1016/j.porgcoat.2021.106499
Zare, E. N., Agarwal, T., Zarepour, A., Pinelli, F., Ali, Z., Rossi, F., et al. (2021). Electroconductive multi-functional polypyrrole composites for biomedical applications. Appl. Mater. Today 24, 101117. doi:10.1016/j.apmt.2021.101117
Zha, Z., Yue, X., Ren, Q., and Dai, Z. (2013b). Uniform polypyrrole nanoparticles with high photothermal conversion efficiency for photothermal ablation of cancer cells. Adv. Mat. 25, 777–782. doi:10.1002/adma.201202211
Zha, Z., Wang, J., Qu, E., Zhang, S., Jin, Y., Wang, S., et al. (2013a). Polypyrrole hollow microspheres as echogenic photothermal agent for ultrasound imaging guided tumor ablation. Sci. Rep. 3, 2360. doi:10.1038/srep02360
Zhang, K., Zhang, J., Liu, Y., Wang, Z., Yan, C., Song, C., et al. (2021). A NIR laser induced self-healing PDMS/Gold nanoparticles conductive elastomer for wearable sensor. J. Colloid Interface Sci. 599, 360–369. doi:10.1016/j.jcis.2021.04.117
Keywords: polycarbonate-based polyurethanes, self-healing, UV-vis shielding properties, polypyrrole nanoparticles, nanocomposites
Citation: Xiong Z, Zhang H, Zhou Y and Yang Y (2022) Preparation of PCU/PPy composites with self-healing and UV shielding properties. Front. Mater. 9:1043355. doi: 10.3389/fmats.2022.1043355
Received: 13 September 2022; Accepted: 30 September 2022;
Published: 18 October 2022.
Edited by:
Wenliang Li, Jilin Medical University, ChinaReviewed by:
Yusheng Qin, Yantai University, ChinaQingbao Guan, Donghua University, China
Jun Xu, Research Center for Eco-environmental Sciences (CAS), China
Copyright © 2022 Xiong, Zhang, Zhou and Yang. This is an open-access article distributed under the terms of the Creative Commons Attribution License (CC BY). The use, distribution or reproduction in other forums is permitted, provided the original author(s) and the copyright owner(s) are credited and that the original publication in this journal is cited, in accordance with accepted academic practice. No use, distribution or reproduction is permitted which does not comply with these terms.
*Correspondence: Yan Zhou, yzhou1992@ciac.ac.cn; Yuming Yang, ymyang@ciac.ac.cn