- 1Research Centre for Biocomposite Materials for Medical, Agricultural and Food Industry, Suranaree University of Technology, Nakhon Ratchasima, Thailand
- 2School of Polymer Engineering, Suranaree University of Technology, Nakhon Ratchasima, Thailand
Biogenic calcium carbonate derived from waste shells has received significant attention in the last 2 decades as a replacement for limestone due to its contribution to reducing environmental impact by turning wastes into value-added biomaterial and moving global society toward net-zero waste. Recently, several review papers have been published regarding applying biogenic derived calcium carbonate in engineering, biomedical, electrochemical, and environmental technologies. This review stands apart from other reviews on this topic in terms of focusing and reviewing the published papers used and reported indirect methods only to obtain calcium carbonate from biogenic waste shells and not by direct methods. The direct or untreated methods include simple grinding, ball milling, or mortar and pestle techniques. In contrast, the indirect methods covered in this review paper consist of precipitation and different chemical treatment techniques. Therefore, this review paper aims to comprehensively summarize the usage of calcium carbonate derived from eggshells and seashells by indirect methods and glance at its recent development for advanced material applications including water treatment, bio-filler, and reinforcement in polymer composites, energy application, pharmaceutical, biomedical, and drug delivery applications.
1 Introduction
Calcium carbonate, which is usually minded from limestone sedimentary rock, is one of the most abundant biominerals on earth and is widely used in various applications due to its biocompatibility and low cost. However, an alternative and more sustainable method to obtain calcium carbonate is from biogenic waste shells such as poultry eggshells and seashells. Annually, millions of tons of biogenic waste shells are disposed of in landfills which tend to pose massive environmental pollution (Yoo et al., 2009; Awogbemi et al., 2020). According to the Environmental Protection Agency (EPA), biogenic waste shells have been ranked as the 15th major food industry pollution problem (Waheed et al., 2020). Therefore, turning those biogenic waste shells into usable value-added biomaterials would be distinctly eco-friendly and economical. The structure and chemical composition of eggshell, cockle shell, and mussel shells are presented in Figure 1 and Table 1.
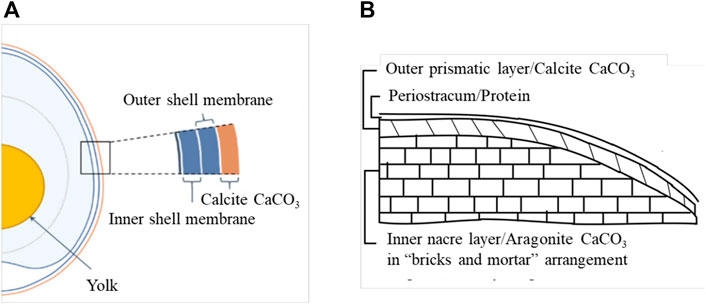
FIGURE 1. Schematics microstructures illustration of (A) Eggshell. Reproduced from Shi et al. (2021) “Avian eggshell membrane as a novel biomaterial A review” https://www.mdpi.com/2304-8158/10/9/2178 licensed under CC BY 4.0. (B) mussel shell. Reproduced with permission from Matthews and Asadov (2020).
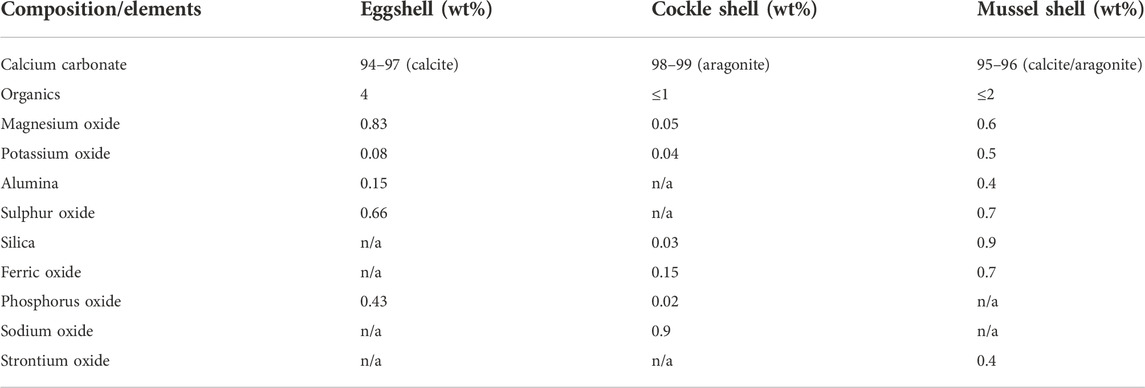
TABLE 1. Chemical composition of eggshell, cockle shell, and mussel shell (Hunton., 2005; Awang et al., 2007; Hamester et al., 2012; Chakraborty et al., 2020; Baláž et al., 2021).
Different methods recently came to light to process the calcium carbonate derived from eggshells and seashells. Thousands of research papers and more than 500 patents have emerged since the turn of the 21st century for sustainable development growth (SDG) (Ahmed et al., 2021). As a result, several review papers have been published about eggshell, and seashells derived calcium carbonate and its applications as filler in polymer composite preparations and pharmaceuticals applications (Rodríguez-navarro et al., 2015; Waheed et al., 2019; Hart, 2020; Baláž et al., 2021). However, there is no review covering different approaches such as precipitation to obtain the specific polymorphic structure of calcium carbonate from biogenic wastes. The precipitation of calcium carbonate from biogenic waste shells enables the opportunity for the formation of four designs, including the amorphous phase and three polymorphs of vaterite, aragonite, and calcite (Trushina et al., 2016). This review presents a comprehensive overview of obtaining indirect (precipitated or treated) calcium carbonate from biogenic waste shells and covering its application in water treatment, bio-filler, and reinforcement in polymer composites, energy application, pharmaceutical, medical application, and drug delivery. Over and above that, the present review should serve as a solid platform for emerging novel methodologies to obtain pure treated or precipitated calcium carbonate with engineered polymorph structures and desired morphology and particle sizes from biogenic waste shells for the development of advanced materials application. Therefore, it will be a contribution from science and technology to urge and support the bio-circular green (BCG) economy and globs SDG.
2 Production of reduced size biogenic calcium carbonate
In order to obtain fine powders of calcium carbonate with nano/micron sizes, different approaches have been reported in the literature so far, including mechanochemical and precipitation methods. The mechanochemical method was employed using the ball milling or grinding process. However, this technique can also be combined with other methods to obtain more speciality properties. For instance, Hassan et al. (2013) have developed a combination of mechanochemical and sonochemical techniques to produce high surface area calcium carbonate nanoparticles from eggshells with the size of ∼10 nm. However, a very recent review paper (Baláž et al., 2021) covered only a mechanochemical approach to producing calcium carbonate particles from eggshell wastes. The disadvantage of the mechanochemical process would be the inability to control the polymorph structures of calcium carbonate, the chance of having impurity from biogenic wastes as it is used without any purification, and a high chance of contamination during the ball milling process.
However, the eggshells or seashells waste can be digested in acid and reformed in highly controlled conditions in the precipitation technique. Azarian et al. (Azarian and Sutapun, 2022) have recently precipitated the calcium carbonate particles from eggshells and showed that morphologies and polymorphs structures of precipitated calcium carbonate (PCC) particles could be effectively tunned by changing the experimental parameters such as initial calcium ions molar concentration, using different polyelectrolytes (non-ionic or ionic) with different molecular weight, and concentration of polyelectrolyte solution. The advantages of this technique are the possibility of controlling the size and obtaining calcium carbonate with desired morphologies, i.e., star-shaped, yarn or spherical shapes with various polymorph crystal structures such as vaterite or calcite.
3 Calcium carbonate structures
There are six structures of calcium carbonate, one hydrous in the form of amorphous calcium carbonate and five crystalline polymorphs. The five crystalline polymorphs are three anhydrous crystallines, i.e., vaterite, aragonite, and calcite, and the other two hydrated crystalline forms of ikaite hexahydrate and monohydrate calcite with limited occurrence in nature (Beck and Andreassen, 2010; Bushuev et al., 2015). It is interesting to note that all of those structures naturally exist. They can also be synthesized or precipitated with different morphologies or sizes and convert to other polymorphs structures by several techniques such as microwave-assisted (Qi and Zhu, 2006; Qi et al., 2014; Skubiszewska-Zięba et al., 2017), carbonation (Hou and Feng, 2005; Beuvier et al., 2011; Udrea et al., 2012; Lai et al., 2015; Yuan et al., 2015), sonochemical (Zhou et al., 2004; He et al., 2005; Luo et al., 2021), hydrothermal (Zhao et al., 2011; Sulimai et al., 2017) and wet precipitation technique (Brečević et al., 1996). The neat calcium carbonate from eggshells exists in the calcite structure. However, the seashells are in the form of an aragonite and calcite mixture (Table 1).
3.1 Amorphous
Amorphous calcium carbonate (ACC) is the unstable phase before vaterite and calcite and is produced naturally by various invertebrates, which later grows into complex shapes crystals (Hincke et al., 2012). Thebservable circular voids with a mean diameter of 313 ± 78 nm in Figure 2A are evidence regarding the presence of ACC in eggshell mineralization. Figure 2B relatively shows the ACC calcium carbonate spherical particles with sub-micron size synthesized in the laboratory from calcium chloride and sodium carbonate in a controlled condition. The dimensions of circular voids in eggshells are comparable to the synthetic ACC with the size of 850 ± 150 nm. Navarro et al. (Rodríguez-navarro et al., 2015) have evaluated the mineralogy and crystallinity of eggshells during its formation process by XRD spectroscopy and SEM microscopy right after ovulation when nucleation sites appear at early mineralization and during the crystalline growth phase and rapid calcification about 14 h after ovulation. From SEM, eggshell mineral originates from the early accumulation of flat disk-shaped ACC particles on the eggshell membrane. As shown in Figure 3 1D-XRD patterns of non-mineralized eggshell membranes collected at the 5th hour show two broad bands attributed to the membrane’s organic components and there is no peak related to Ca formation. However, in a sample collected after 5 h, with primitive calcium carbonate mineralization covering the surface no further diffraction peaks appear, which indicates that the calcium carbonate possesses an amorphous phase. At the 6th hour, while the membrane becomes further mineralized, weak calcite diffraction peaks appeared at two Theta 30○. With additional mineralization over 7 h and 14 h, the intensities of calcite peaks increased and became sharper, indicating highly crystalline calcite formations.
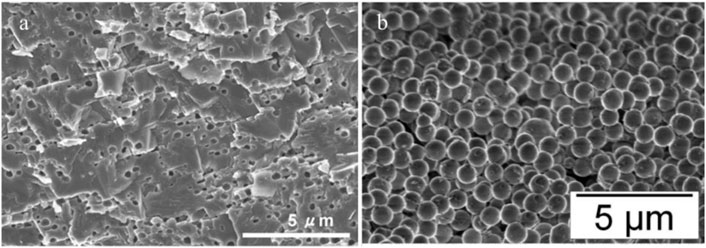
FIGURE 2. (A) Eggshell calcite and small spherical voids regarding the presence of ACC prior to calcite formation reproduced with permission from Hincke et al. (2008), (B) ACC calcium carbonate synthesis in the lab from calcium chloride and sodium carbonate. Adapted with permission from Faatz et al. (2005), American Chemical Society.
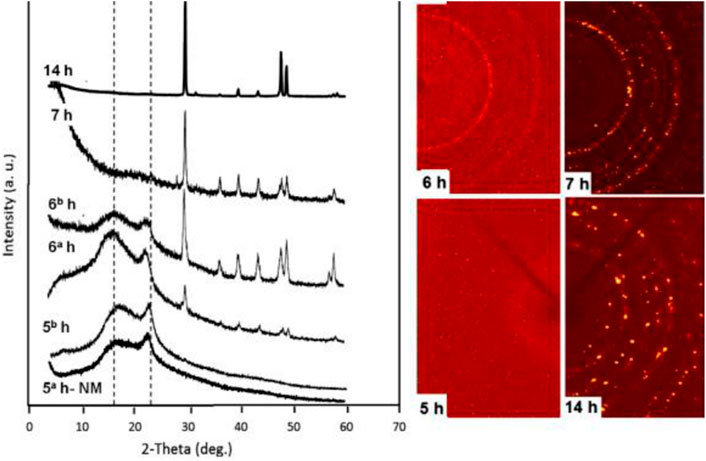
FIGURE 3. 1D and 2D-XRD patterns of eggshell collected at different time intervals. Reproduced with permission from Rodríguez-navarro et al. (2015).
Another study reported by Lakshminarayanan et al. (2006) evaluated the biogenic calcium carbonate from quail eggshell biomineralization by the calcium carbonate precipitation technique. The precipitation was performed by passing purified CO2 gas through dispersed eggshell powder in water. After they dissolved, the solution was filtered, and CO2 gas was bubbled through for another 30 min. Finally, the solution was placed on a petri dish undisturbedly, and calcium carbonate precipitated at various time intervals. The authors found that ACC was precipitated in the early stages, transforming into the most stable crystalline calcite phase (Figure 4). These results showed that precipitation of calcium carbonate follows the same mechanism, mineralogy, and crystallinity as the eggshell formation process.
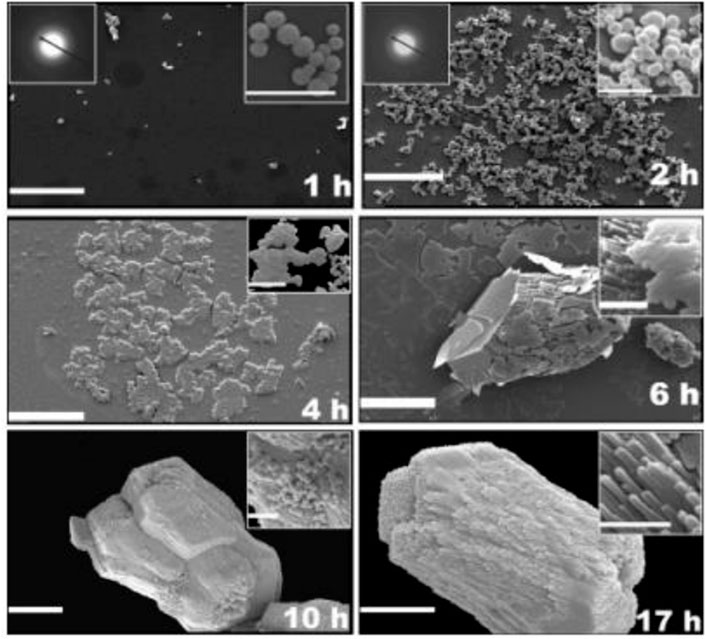
FIGURE 4. SEM micrographs of the calcium carbonate grown by the precipitation experiment at various time intervals. Scale bar and inset scale bar are 10 µm and 2 µm, respectively. Reproduced with permission from Lakshminarayanan et al. (2006), American Chemical Society.
3.2 Vaterite
Vaterite calcium carbonate with hexagonal crystal structure and spherical-like morphology has been formed and exists naturally in birds and many marine livings such as gastropods, mollusc pearls, fish otoliths, and ascidians (Jessop et al., 2008; Soldati et al., 2008; Portugal et al., 2018). As a metastable phase, it is likely to occur as a transient intermediate between the ACC phase and more stable calcite or aragonite (Christy, 2017). Vaterite is as unstable as ACC, with a spherical shape but bigger size than ACC. Lin et al. (2020) have prepared vaterite calcium carbonate microparticles from discarded oyster shells by digestion and precipitation of calcium carbonate in hydrochloric acid (Figure 5). The grinded oyster shells have irregular morphology prior to precipitation. After the precipitation, the morphology has been changed to spherical shape with the size of 4 ± 2 μm. The XRD analysis was performed on obtained microsphere and diffraction peaks located at 2θ of 20.9° (004), 24.8° (110), 27° (112), 32.8° (114), 43.7° (300), 50.1° (118), and 55.9° (224) indicated the existence of crystalline phase in vaterite structure. In the related research conducted by Sutapun et al. (2012), chicken eggshells were precipitated after digestion in hydrochloric acid with the addition of sodium carbonate in which vaterite and calcite mixture was obtained, and the surface area from untreated chicken eggshell particles increased from 2.55 [m2/g] to 4.38 [m2/g] after precipitation (Figure 6).
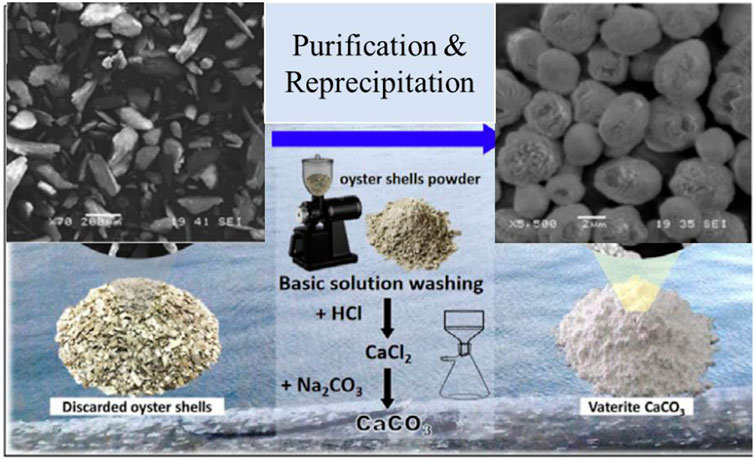
FIGURE 5. Schematic preparation process of vaterite calcium carbonate microspheres from oyster shells and SEM images of oyster shells and vaterite calcium carbonate microspheres. Reproduced with permission from Lin et al. (2020).
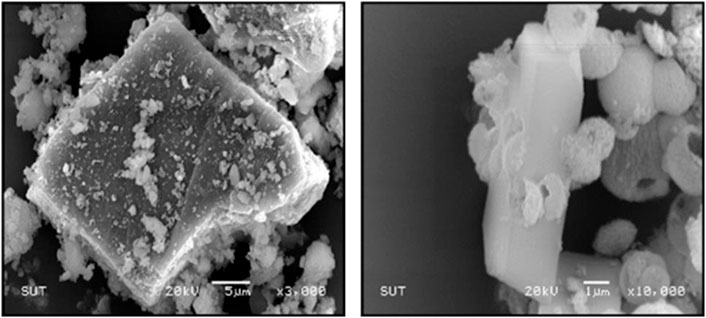
FIGURE 6. SEM microimages of chicken eggshell and precipitated chicken eggshells with a magnification of 3,000 X and 10,000 X, respectively. Reproduced with permission from Trans Tech Publications, Ltd., (Sutapun et al., 2012).
3.3 Aragonite
Aragonite calcium carbonate with orthorhombic crystal structure and needle-like morphology found naturally in almost all mollusc shells, widely used as inorganic filler in polymers (Ramakrishna et al., 2017) and for biomedical applications (Mailafiya et al., 2019). For instance, Nurul et al. (Islam et al., 2013) have used a mechanical grinding in the presence of a biomineralization catalyst, i.e., dodecyl dimethyl betaine, to produce pure aragonite nanoparticles with an average diameter of 20 nm from cockle shell wastes. In another example, Habte et al. (2020) synthesized aragonite crystals from waste chicken eggshells and seashells (abalone, mussel, manila clam, scallop, and oyster shells) through wet carbonation method in aqueous suspensions with constraint carbon dioxide injection at 80°C. The authors reported that the obtained green aragonite calcium carbonate could be used for numerous applications and have the potential for commercial-scale synthesis from eggshells and bivalve seashells biogenic wastes as cheap and abundant precursors. Figures 7A,B show the TEM images of cockle shell powders before and after treatment, respectively. The cockle shell powders have irregular morphology which mainly transformed to needle shapes after treatment. Furthermore, Figures 7C,D show the SEM images of needle shape aragonite polymorph obtained from chicken eggshells and needle shape aragonite polymorph obtained from mixed bivalve seashells, respectively.
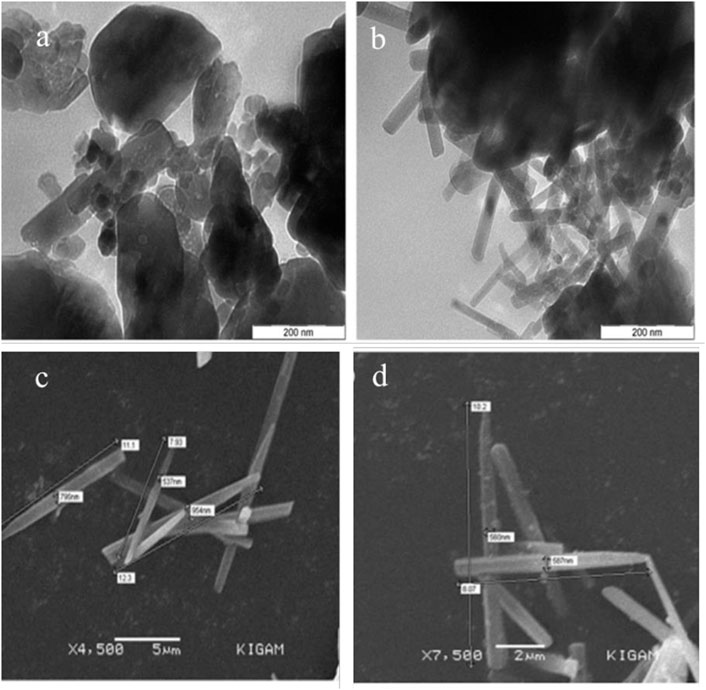
FIGURE 7. TEM microimages of aragonite crystals from cockle shell powders before (A) and after (B) the powder treatment. Reproduced with permission from Islam et al. (2013). SEM microimages of (C) aragonite crystals obtained from chicken eggshells and (D) aragonite crystals obtained from mixed bivalve seashells. Reproduced from Habte et al. (2020) “Synthesis, characterization and mechanism study of green aragonite crystals from waste biomaterials as calcium supplement” https://www.mdpi.com/2071-1050/12/12/5062 licensed under CC BY 4.0.
3.4 Calcite
Calcite with a rhombohedral crystal structure is the most abundant calcium carbonate and the major incorporator of poultry eggshells, limestone, and chalk (Athanasiadou et al., 2018; Portugal et al., 2018). The rhombohedral phased calcite usually exists as cubic particles (Luo et al., 2020). Calcite calcium carbonate is widely used in cement and metallurgy industries due to its high corrosion resistance and good thermo-chemical stabilities (Yang and Xu, 2011). In the study published by Hariharan et al. (2014), calcite calcium carbonate nanoparticles were prepared from cockle shells by precipitation technique using chitosan as a precursor. The ground cockle shells calcium carbonate was digested in concentrated hydrochloric acid and mixed with dissolved chitosan in acetic acid. This solution was then mixed with sodium carbonate solution and slightly heated overnight to obtain precipitated pure calcite calcium carbonate particles. In a related study, Putkham et al. (2018) have synthesized pure calcite calcium carbonate from eggshell waste via a simple liquid-liquid precipitation method. The eggshells were digested in hydrochloric acid with different concentrations to obtain calcium chloride solution first, which later reacted with sodium carbonate in various temperatures and agitation time to obtain different polymorphs of calcium carbonate. This liquid-liquid precipitation method allowed the production of micron particle sizes ranging from 4 to 11 µm with narrow particle size distribution. Notably, the pure cubic shape of calcium carbonate was formed at high reaction temperatures and low initial concentrations of calcium chloride solution. Figures 8A,B shows the SEM images of untreated eggshells powders and precipitated eggshells calcite calcium carbonate powders, respectively. These results indicated the importance of the precipitation technique to produce pure calcite calcium carbonate with controlled particle size and morphology.
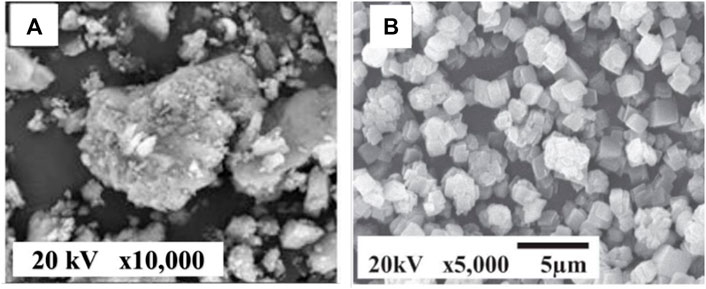
FIGURE 8. SEM microimages of (A) untreated calcite eggshell powders. Reproduce from Madiabu et al. (2021) “Equilibrium and Kinetic Study of Removal Copper(II) from Aqueous Solution Using Chicken Eggshells: Low Cost Sorbent” https://ojs.jmolekul.com/ojs/index.php/jm/article/view/658 licensed under CC BY 4.0. (B) Precipitated calcite eggshell powders. Reproduced with permission from Putkham et al. (2018).
4 Applications
Since the interest in using biogenic calcium carbonate has increased significantly, this brings a severe need to seek novel applications in advanced materials. To broaden its application, researchers have been trying in vast fields of materials science and developed new technologies accordingly. Figure 9 shows the schematic illustration of biogenic calcium carbonate used in different applications. Recently several commercial companies have emerged worldwide using calcium carbonate derived from biogenic waste to produce various products on a large industrial scale. For instance, EUROATOMIZADO, S.A. company (Tiles Made With Eggshell. 2022), actively uses biogenic calcium carbonate to design, produce, and commercialize novel ceramic formulations at a pilot and industrial scale.
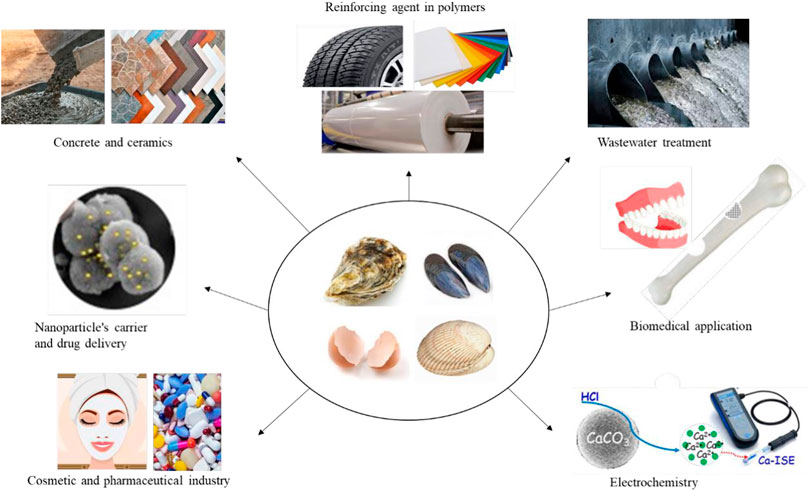
FIGURE 9. Overview of biogenic derived calcium carbonate applications from waste shells. Adapted and reproduced with permission from Royal Society of Chemistry, (Yu et al., 2010; Fu et al., 2017b). Reproduced with permission from Liu and Lian (2019).
Similarly, EGGNOVO Co., Ltd. (Eggnovo, 2019) produces functional ingredients based on calcium carbonate derived from eggshell waste for the cosmetic, nutraceutical and pharmaceutical industries. Also, Justegg Co., Ltd. (JustEgg Chilled Foods Ltd., 2019) produces eggshell powders as a source of bio-calcium carbonate for possible application as bio-filters for reinforcing the plastics mainly used in Silvergate Plastics Co., Ltd. (Silvergate Plastics. 2003) masterbatch plastic products. The following sections will cover all the published research papers so far dealing with treated or modified bio-calcium carbonate in different applications.
4.1 Electrochemistry
Neat calcium carbonate derived from eggshells and seashells has the potential to be employed as electrodes in electrochemical cells. The pure calcite calcium carbonate can be used as a cathode, while calcinated calcium carbonate at relatively high temperatures may operate as an anode. At a temperature >700°C, calcium carbonate is mainly oxidized to calcium oxide form, which is suitable to employ as an anode in an electrochemical energy device. Setiawan et al. (2017) synthesized Ca-doped Li4Ti5O12 (LTO) anode in the form of Li4-x CaxTi5O12 (x =0, 0.05, 0.075, and 0.1) using calcined calcium carbonate derived from waste eggshells as a source of calcium for lithium-ion battery application. The preliminary results indicated that calcium carbonate-derived eggshells could be used as a good dopant source for LTO, with the highest charge-discharge capacity of 177.14 mAh/g and 181.92 mAh/g obtained for Li3.95Ca0.05Ti5O12 anode system. In a similar study (Minakshi et al., 2018), the authors reported that calcium carbonate derived from waste eggshells could be used as an active electrode in Li-ion capacitors to store and release charges with a wide range electrochemical stability window. Initially, a slurry of chicken eggshell powders was prepared by mixing with carbon black (20 wt%) and polyvinylidene fluoride (10 wt%) as a binder and used as cathode against Li anode. The eggshell-Li electrochemical cell’s initial discharge capacitance and reversible capacitance were 232 F g−1 and 120 F g−1, respectively. Moreover, the eggshell-Li electrochemical cell maintained outstanding capacitance retention of 92% over 1,000 cycles, nearly equivalent to those electrochemical properties of commercialized activated carbon cathode.
In a relevant study, Minakshi et al. (2019) have demonstrated the use of wasted chicken eggshells in energy storage application and found that calcium carbonate derived from eggshell wastes are pretty suitable for both cathode and anode as they show a quasi-box-shaped potentiostatic curve indicating their capacitor-type behaviour. The pure calcium carbonate was used as a cathode and showed a modest discharge capacitance of 10 F g−1. However, the calcined calcium carbonate (calcium oxide) or quicklime was used as the anode, which exhibited an excellent capacitance value of 47.5 F g−1. The electrodes in both positive and negative regions showed a discharge capacitance of 55 F g−1 with close retention to about 100% after 1,000 cycles, indicating good electrochemical stability of calcium carbonate derived waste shells.
4.2 Polymer bio-filler composite
Inexpensive bio-fillers can be introduced to polymer matrix in order to improve properties and reduce the cost of final products. Calcite calcium carbonate obtained from limestone is the most widely used filler in polymer formulations. As a filler, calcium carbonate allows cost reduction and improved mechanical properties. Numerous papers have reported in the literature so far that the addition of calcium carbonate from seashells and eggshells is an effective way to improve the mechanical properties of the polymer matrix (Toro et al., 2007; Kang et al., 2010; Feng et al., 2014; Hassen et al., 2015; Shafiur et al., 2016; Essabir et al., 2017; Murugan et al., 2017; Hayeemasae et al., 2019; Munusamy et al., 2019; Santu et al., 2020). Recently, two review papers were published by Vandeginste (Vandeginste, 2021), and Owuamanam et al. (Owuamanam and Cree, 2020) regarding the usage of eggshells and seashells derived calcium carbonate in composites with respect to different matrixes such as a polymer (thermoset, thermoplastics and rubber), ceramics and metal matrix. However, this section reviews only the precipitated or treated calcium carbonate from biogenic waste shells with modified structures as bio-filler. For instance, Shuhadah et al. (Shuhadah and Ghani 2009) have modified the eggshell powders with an isophthalic acid coupling agent, incorporated them in low-density polyethylene (LDPE), and compared the mechanical properties with LDPE filled with unmodified eggshell powders. The authors found that the tensile strength decreases with increasing filler loading, and LDPE/modified eggshell powder composites have higher strength and better interaction than LDPE/unmodified eggshell powder composites. However, improvement of elastic modulus was observed with increasing filler loading. The authors suggested that an increase in elastic modulus with more filler is due to higher stiffness than the neat polymer, making it less deformable against a greater load. Furthermore, elastic modulus for LDPE/modified eggshell powders composites was lower than LDPE/unmodified eggshell powders composites due to the presence of a coupling agent, which toughens the composites and thus decreases elastic modulus. In a similar study, Yang et al. (2011) modified the chicken eggshell wastes with pimelic acid (PA) through the solution and then incorporated them into polypropylene (PP) through the melt blending technique. The modification by PA improved the dispersion and interfacial bonding of eggshell calcium carbonate in PP and the impact strength of PP was enhanced by 228% compared to neat PP. However, the tensile properties and flexural properties of PP slightly decreased. This might be due to, the thermal decomposition of eggshells membrane, which have not been completely removed during the cleaning process of eggshells.
Yan Li et al. (Li et al., 2012) have used the waste shellfish shells as a precursor of calcium carbonate and prepared granules of shellfish shells by removing the cuticle, crushing, grinding and treating them with shearing emulsification. The treated shells were further introduced as a bio-filler to reinforce PP polymer. The authors claimed that the mechanical behaviour of PP composite shows a higher yield strain, yield strength, tensile strength, and elongation at break in comparison to conventional calcium carbonate filled PP and yield strength of PP composite improved by 11.1% with only 2 wt% loading of treated shellfish shells. The study by Hassan et al. (2014) showed that high surface area calcium carbonate nanoparticles could be obtained from waste shells by using an ultrasonic irradiation treatment. As low as 2 phr of obtained calcium carbonate nanoparticles were incorporated within Bioplast GS 2189 thermoplastic polymer, and mechanical results revealed flexural strength and elastic modulus of polymer enhanced by 35.3% and 30.5%, respectively.
Similarly, Fombuena et al. (2014) have treated the calcium carbonate from seashells with a silane coupling agent, used it as a bio-filler in an epoxy matrix, and found that silane treatment can effectively prevent particle aggregation, thus leading to optimum calcium carbonate dispersion. Loading of 30 wt% of treated seashell increased the mechanical properties as well as flexural modulus (over 50%), hardness (around 6%) and thermal properties. Li et al. (2016) modified and functionalized the eggshell particles with phenyl phosphonic acid and incorporated them into polylactic acid (PLA) by melt blending to improve the crystallization behaviour and enhance the mechanical properties enzymatic hydrolysis of PLA polymer. With 20 phr loading of treated eggshell particles, the crystallization half-time of a PLA composite decreased significantly from 27.09 to 0.69 min at 130°C accordingly. Furthermore, the storage and tensile moduli enhanced with increasing modified eggshells loading in which tensile strength, tensile modulus and elongation at break were 53.4 MPa, 2,460 MPa, and 2.5%, respectively.
In another investigation, Tiimob et al. (2016) have used ultrasonication treatment with 50 amplitude after the ball milling process and successfully reduced the size of calcium carbonate particles derived from eggshell to less than 100 nm. 1, 2, 3, 4, 5, and 10 wt% of the obtained nano-eggshell particles were loaded to epoxy resin, and DMA/TMA analysis revealed significant improvements of 7–22% in the storage moduli and 3–17% in coefficient of thermal expansion, respectively. Furthermore, the flexure strength, modulus, and toughness significantly improved by 6–31%, 11–37%, and 10–36%, respectively. In another study, chicken eggshell particles were chemically modified with a silane coupling agent and introduced to high-density polyethylene (HDPE) to study its effects on tensile strength and elastic modulus (Sharmeeni et al., 2016). The authors have also incorporated the unmodified eggshell particles for comparison purposes. Both systems exhibit higher tensile strength than neat HDPE at 10 phr and 20 phr filler loading. As the filler loading increases above 20 phr, the tensile strength drops for both systems due to the aggregation of eggshell particles and weakening the interfacial adhesion between eggshell particles and HDPE polymer matrix. However, modified eggshell particles/HDPE composites exhibit higher tensile strength at all filler loading up to 40 phr since the coupling agent promotes a better adhesion between polymer matrix and eggshell particles. The authors claimed that coupling agent treatment could improve the shear bond strength of eggshell particles towards the HDPE polymer matrix.
Similarly, Tiimob et al. (2017) have synthesized eggshell/Ag nanoparticles by ball milling of eggshell and AgNO3 in polypropylene glycol (PPG) solution as a deflocculating agent. The polymer films obtained by dispersing obtained eggshell/Ag nanoparticles into poly (butylene adipate-co-terephthalate) (PBAT)/agro-based polylactic acid (PLA) blend by 3D printing and hot melt extrusion techniques for food packaging application. The tensile strength was reduced by incorporating the eggshell/Ag nanoparticles with 0.5–2.0 wt% due to poor interaction between the polymer matrix and eggshell/Ag nanoparticles. However, the thermal analysis revealed the onset of thermal decomposition improved. In addition, the results from the antimicrobial study showed that the films have bacteriostatic effects on Salmonella Enteritidis and Listeria Monocytogenes bacteria. In another similar study, the silver nanoparticles were embedded in calcium carbonate particles (Ag/CaCO3) by a precipitation method and further loaded in poly (L-lactic acid) nanofibers by electrospinning technique with potential usage for antimicrobial food adsorbent pads application (Zin and Sutapun, 2022).
This is worthy of mentioning that all of the research works available in the literature reported the chemical or physical treatment methods to reduce the size or modify eggshell particles to decrease the surface tension between eggshell particles and polymer matrix. Despite achieving nearly optimal eggshell particles dispersion through polymer matrix by those treatments, manipulation of eggshells particles to obtain different calcium carbonate crystal structures by precipitation technique and incorporate them into the polymer matrix is unexplored and remains as a large gap.
4.3 Biomedical materials
Among the polymorphs of calcium carbonate, aragonite calcium carbonate is the most reported crystal structure of calcium carbonate in bone tissue engineering and biomedical sciences application (Awang et al., 2007; Fu W. et al., 2017; Jaji et al., 2017; Ananthu et al., 2018). This is mainly due to its superior density over the other polymorphs structure (calcite and vaterite), making it more biocompatible to be integrated, resolved, and replaced by bones (Islam et al., 2011; Shafiu Kamba et al., 2013). This high-density feature of aragonite is very interested due to its similarities to the human bone density (Bharatham et al., 2014). Nurul et al. (Islam et al., 2012) have synthesized aragonite calcium carbonate nanoparticles from cockle shells suitable for repairing fractured bone and tissue scaffolds. The rod-shaped aragonite nanoparticles with a mean average diameter of 30 ± 5 nm were produced by mechanically mixing fine cockle shells powders with dodecyl dimethyl betaine (BS-12) for 90 min at ambient temperature.
Similarly, Mony et al. (2015) evaluated the remineralization potential of chicken eggshell powder solution and found that the digested chicken eggshells in 4% acetic acid solution can effectively remineralise the enamel surface lesion in the tooth. In a related study, Huang et al. (2020) coated the eggshell particles with MgO nanoparticles and fabricated a biomimetic active scaffold through the chemical crosslinking of the modified eggshell nanocomposite carboxymethyl chitosan (CMC) and bone morphogenetic protein 2. The results showed that the modulus and compressive strength of the new composite scaffold based on modified eggshells were higher than those of the CMC scaffold. Moreover, the composite scaffold exhibited significant mineralization ability and strong osteogenic (bone-forming) differentiation potential.
4.4 Wastewater treatment
Nowadays, contamination of water resources is a major environmental challenge worldwide. Especially, the relatively high content of heavy metals in water may bioaccumulate in food resources and impose a serious risk on the health. Calcium carbonate derived from biogenic waste shells can effectively adsorb and remove heavy metals from aqueous solutions such as Cd2+, Pb2+, and Cu2+ (Ahmad et al., 2012; Tizo et al., 2018). Up to now, there is scarcely any research in the literature regarding the use of treatment or precipitated biogenic calcium carbonate with tailored morphology for water treatment. For instance, Lin et al. (2020) have used the precipitated technique to obtain vaterite calcium carbonate granules from discarded oyster shells as an adsorbent for heavy metal ions removal. The vaterite calcium carbonate showed an excellent removal efficiency of 99.9%, 99.5%, 99.3%, and 57.1% for Pb2+, Cr3+, Fe3+, and Cu2+ metal ions, respectively. The conventional calcium carbonate was also studied for the heavy metals removal efficiency compared with precipitated oyster shells, and results showed better removal efficiencies of vaterite calcium carbonate particles. Figure 10 shows the SEM image of vaterite calcium carbonate before and after Pb2+ removal. The removal efficiency of heavy metal ions consists of ion exchange reactions between vaterite calcium carbonate and heavy metal ions, which resulted in the recrystallization of calcium carbonate. The vaterite calcium carbonate particles from oyster shells obtained by precipitation technique have the advantage of low price, easy synthesis which can be potentially incorporated into household drinking water filtration systems. Other than heavy metals, calcium carbonate derived biogenic waste have been also reported as effective adsorbent for removing organic dyes from waste water such as direct blue (Murcia-Salvador et al., 2020), methyl violet (Foroutan et al., 2019), red congo (Seyahmazegi et al., 2016), rhodamine B (Borhade and Kale, 2017) and acid orange (Tsai et al., 2008; Yari et al., 2015).
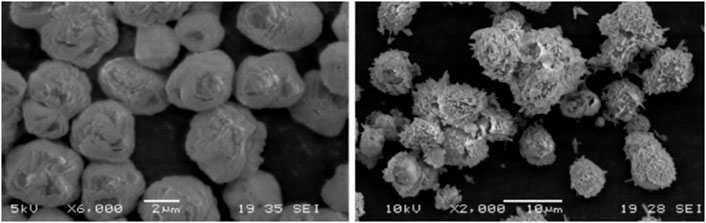
FIGURE 10. SEM microimages of vaterite calcium carbonate microspheres before and after Pb+2 removal. Reproduced with permission from Lin et al. (2020).
4.5 Nanoparticle’s carrier and drug delivery
The calcium carbonate particles can be employed as vehicles for carrying nanoparticles or various drugs due to their biocompatibility (Trushina et al., 2023). In a study conducted by Than et al. (2012), modified eggshell powder as a pharmaceutical excipient in tablet and acetaminophen as a model drug were investigated. The eggshell powders were treated by surface modification using 1.0% w/v stearic acid in deionized water, ethanol and chloroform and untreated eggshell powder was used as control. The tablets, including acetaminophen, microcrystalline cellulose and treated eggshell powder, were prepared by direct compression method, and demonstrated that immediate release of acetaminophen was obtained from tablets containing untreated eggshell powder. In contrast, sustained release of the drug was obtained from the tablet formulations containing modified eggshell particles. Recently, silver nanoparticles loaded with calcium carbonate (Ag/CaCO3) particles were prepared by precipitation technique and reported by Azarian et al (Azarian and Sutapun, 2022). As illustrated in Figure 11, spherical precipitated calcium carbonate (PCC) particles were deposited by silver nanoparticles and energy-dispersive X-ray spectroscopy mapping (EDS) images depicted a clear spherical pattern, indicating their loading at the outer layer of PCC particles with sliver atomic weight percentage of 4.27 wt%.
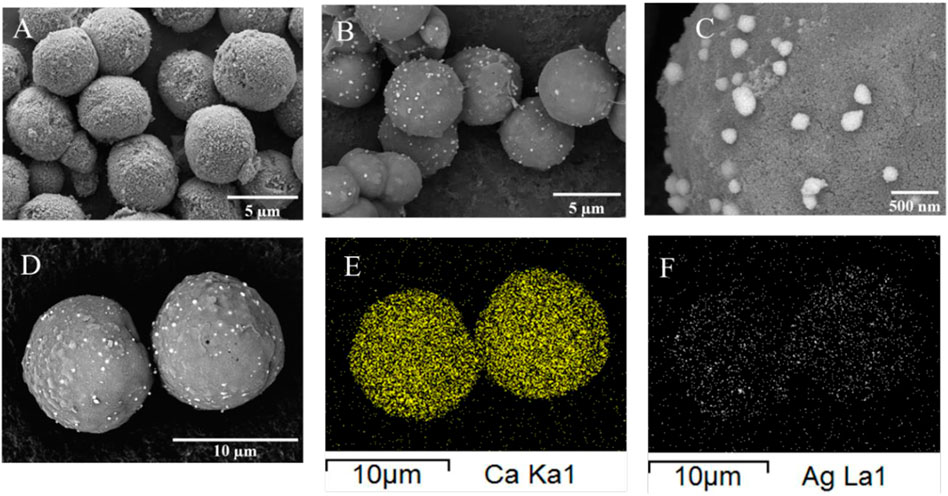
FIGURE 11. (A) SEM microimage of PCC particles without silver loading, (B) SEM microimage of PCC particles loaded with silver nanoparticles, (C) Higher magnification of SEM microimage (B), (D) SEM microimage of PCC loaded with silver nanoparticles. Reproduced from (Azarian and Sutapun, 2022) “Tuning polymorphs of precipitated calcium carbonate from discarded eggshells: effects of polyelectrolyte and salt concentration” https://pubs.rsc.org/en/content/articlehtml/2022/ra/d2ra01673g licensed under CC BY-NC 3.0.
Similarly, Apalangya et al. (2014) have prepared calcium carbonate silver hybrid nanoparticles with wet mechanochemical milling technique using chicken eggshell and silver nitrate as starting materials. The eggshell particles with crystalline calcite structure were embedded with silver nanoparticles ranging in size from 5 to 20 nm. The hybrid eggshell/silver nanocomposite exhibited superior inhibition of E. coli growth using the Kirby–Bauer discs diffusion assay. In a related study, the aragonite calcium carbonate from cockle shell have been synthesized via oil-in-water microemulsions in high-pressure homogenizer using Tween-80 and glycerol as surfactant and co-surfactant, respectively. The ciprofloxacin drug was loaded into obtained cockle shells aragonite calcium carbonate nanoparticles (encapsulation efficiency of 95%) in suspension by mechanically stirring, and antibacterial properties were studied by disc diffusion protocol on Salmonella Typhimurium. The results indicated that ciprofloxacin/calcium carbonate nanoparticles improved the antibacterial efficacy of the antibiotic by increasing the mean diameter of the inhibition zone from 11.7 ± 0.9 mm in ciprofloxacin alone to 18.6 ± 0.5 mm in calcium carbonate delivery system (Ja, 2016).
Dayanidhi et al. (2020) have synthesized the silver nanoparticles with an average particle size of 35 nm, using microwave and Sapindus mukorossi extract as a stabilizing agent. The obtained silver nanoparticles were immobilized on the surface of grounded eggshell powder with micron size in solution by overnight mechanical stirring. A recent review paper published by Ferreira et al. (2020) also reviewed the vaterite calcium carbonate crystals obtained from precipitation technique as carriers for controlled delivery of antimicrobials.
4.6 Cement and bio-ceramics
Cement is one of the most used structural materials in constructions and buildings (Wan Mohammad et al., 2017). Cement is an expensive mixture of raw materials, including iron, calcium, silicon, and aluminium which yields carbon dioxide emissions during production, making the cement industry one of the most significant contributors of greenhouse gas (7% of global CO2 emission) (Benhelal et al., 2013). Green cement can be produced by using biogenic calcium carbonate waste instead of limestone and clay raw materials in clinker production, consequently reducing carbon dioxide emissions (Tan et al., 2018). In another study, Pliya et al. (Pliya and Cree, 2015) have studied the use of ground untreated white and brown chicken eggshell powders as potential replacements of calcium carbonate derived from limestone in cement mortars. The results indicated that the addition of untreated eggshell powders in cement reduced the compression and flexural strengths of the cement. The lower properties of calcium carbonate eggshell powders would be due to irregular calcite calcium carbonate particles with larger sizes and residual of inner organic membrane of shells as they were obtained by only grinding without any treatment or precipitation method. This indicates the importance of precipitation technique or additional organic extraction treatment to obtain purer calcium carbonate with desirable size and controllable crystal structures. In another study, Cree et al. (Cree and Rutter, 2015) have applied heat and bleaching treatments to eggshell powders in order to obtain the purified form of calcite calcium carbonate as a potential replacement of limestone in the cement industry. Although pure calcite calcium carbonate was obtained by heat and bleaching treatments, irregular calcite morphology was observed under electron microscopy. Moreover, as stated by the same authors, bleaching treatment is unsustainable, and is not eco-friendly.
Choi et al. (2016) have introduced a method to generate bio-cementation in sand using microbially induced carbonate precipitation process from waste eggshells instead of using calcium chloride. The eggshells were digested in acetic acid and used for calcium carbonate precipitation in concrete, and a comparative study was performed between sand samples treated using precipitated calcium carbonate from eggshell and sands using precipitated calcium carbonate using calcium chloride. It was found that calcium carbonate produced from eggshells is just as good as using calcium chloride solution. The recent review paper published by Chong et al. (2020), have focused on the properties of concrete with untreated eggshell powders (eggshell concrete) based on previous studies, stated clearly in detail that at about 10 wt% eggshell powders, the cement possesses various advantages in comparison to conventional cement including improved hardened properties, lower setting time, and enhanced resistance to water penetration. However, there is a lack of research regarding eggshell cement’s durability properties in different environments. Moreover, there is a lack of research regarding the usage of precipitated calcium carbonate from eggshell particles with desired size, structure and morphology in advanced cement and ceramics industries.
4.7 Cosmetic, pharmaceutical and food industry
Annually one-third of the food suitable for human consumption is wasted globally, which is also blameworthy for one-third of all greenhouse gases produced (Waheed et al., 2019). Calcium carbonate is a vital mineral ingredient which tonnes of it discarded daily plays a significant role in supporting bone and teeth health. In research by Murakami et al. (2007) physicochemical properties of calcium carbonate from eggshells have been studied and found that calcium carbonates derived from eggshell wastes are more thermally stable than the conventional calcium carbonate with similar crystallinity, indicating that eggshell calcium carbonate should be served as an alternative in pharmaceutical, food and cosmetic products. Recently, Hickman et al. (2019) have studied the composition of various calcium carbonate obtained from geological, synthetic and biological origins and found that biological calcium carbonate is not different from traditional calcium carbonate sources, plus the advantages of renewability and sustainability. However, the organic membrane of eggshells would bring out the need for some additional processing such as precipitation or treatment technique.
Furthermore, calcium carbonate can react with gastric HCl in the human body and act as an antacid. Several studies indicated that porous calcium carbonate derived from eggshell powder could be absorbed better in the human intestine than limestone calcium carbonate (Aditya et al., 2021). Up to now, there is no study available in literature dealing with the usage of calcium carbonate with different polymorphic structures obtained from treated or precipitation techniques. However, plenty of research is available in the literature that used and reported the calcium carbonate derived biogenic waste in foods, cosmetics, and pharmaceutical industry by a simple direct method, which is out of the interest of the current review paper. There is also plenty of research in the literature that used the calcium carbonate derived biogenic waste as starting materials to obtain other calcium derivatives such as calcium citrate (Palacios et al., 2021), hydroxyapatite (Alhussary et al., 2020), and calcium lactate (Daengprok et al., 2002) for pharmaceutical and food industry applications.
5 Conclusion and outlook
In this comprehensive, up-to-date review, we have summarized all the reported studies so far that applied treated or precipitated calcium carbonate derived from biogenic wastes for advanced materials application. The development of advanced material applications based on derived calcium carbonate from biogenic wastes showed significant progress due to its multiple impacts towards Sustainable Development Goals (SDGs) such as reducing environmental pollution, sustainability, and cost-effectiveness. The recently published research papers regarding the utilization of biogenic waste-derived calcium carbonate have significantly increased in different fields such as water treatment, filler, and reinforcement in polymer composites, energy storage devices, pharmaceutical, biomedical application, and drug delivery. However, most of the reported research in literature have used a direct method and employed simple mechanochemical or grinding techniques to reduce the size of calcium carbonate with only calcite crystal structures. The disadvantages of untreated or non-precipitated methods are obtaining impure irregular calcium carbonate particles with minimal control on crystal structure, size, and morphology. As calcium carbonate derived from biogenic waste gains more attention, it is essential to seek new process routes to improve and boost its further progress in the future. Therefore, it is necessary to put additional efforts into investigating, formulating, and examining new methodologies such as indirect, i.e., treated or precipitation technique to obtain an engineered reduced-sized calcium carbonate with stable polymorphic structures and desired morphologies. Calcium carbonate is an excellent inorganic material for advanced materials with improved properties. The technique development for precipitation and usage of calcium carbonate from biogenic wastes is vital due to decreasing environmental pollution and the opportunity to engineer and obtain desired calcium carbonate polymorphic structures with intended particle sizes and shapes suitable for various applications.
Author contributions
MA reviewed the literature and drafted the review while corresponding author supervised, organized and reviewed the paper.
Funding
This work was supported by Suranaree University of Technology (SUT), Thailand Science Research and Innovation (TSRI), and National Science, Research, and Innovation Fund (NSRF).
Conflict of interest
The authors declare that the research was conducted in the absence of any commercial or financial relationships that could be construed as a potential conflict of interest.
Publisher’s note
All claims expressed in this article are solely those of the authors and do not necessarily represent those of their affiliated organizations, or those of the publisher, the editors and the reviewers. Any product that may be evaluated in this article, or claim that may be made by its manufacturer, is not guaranteed or endorsed by the publisher.
References
Aditya, S., Stephen, J., and Radhakrishnan, M. (2021). Utilization of eggshell waste in calcium-fortified foods and other industrial applications: A review. Trends Food Sci. Technol. 115, 422–432. doi:10.1016/j.tifs.2021.06.047
Ahmad, M., Usman, A. R. A., Lee, S. S., Kim, S. C., Joo, J. H., Yang, J. E., et al. (2012). Eggshell and coral wastes as low cost sorbents for the removal of pb 2+, cd 2+ and cu 2+ from aqueous solutions. J. Ind. Eng. Chem. 18, 198–204. doi:10.1016/j.jiec.2011.11.013
Ahmed, T. A. E., Wu, L., Younes, M., and Hincke, M. (2021). Biotechnological applications of eggshell: Recent advances. Front. Bioeng. Biotechnol. 9, 675364–675419. doi:10.3389/fbioe.2021.675364
Alhussary, B., Taqa, A. G., and Taqa, A. (2020). Preparation and characterization of natural nano hydroxyapatite from eggshell and seashell and its effect on bone healing. J. Appl. Veterinary Sci. 5, 25–32. doi:10.21608/javs.2020.85567
Ananthu, M., Shamnadh, M., and Dileep, P. N. (2018). Experimental evaluation on mechanical properties and wear resistance in PMMA seashell bionanocomposite for medical application. Mater. Today Proc. 5, 25657–25666. doi:10.1016/j.matpr.2018.11.007
Apalangya, V., Rangari, V., Tiimob, B., Jeelani, S., and Samuel, T. (2014). Development of antimicrobial water filtration hybrid material from bio source calcium carbonate and silver nanoparticles. Appl. Surf. Sci. 295, 108–114. doi:10.1016/j.apsusc.2014.01.012
Athanasiadou, D., Jiang, W., Goldbaum, D., Saleem, A., Basu, K., Pacella, M. S., et al. (2018). Nanostructure, osteopontin, and mechanical properties of calcitic avian eggshell. Sci. Adv. 4, eaar3219–13. doi:10.1126/sciadv.aar3219
Awang, J., Awang, H., Abu Bakar, Z., Zuk, M. D., and Noordin, M. M. (2007). Mineral composition of the cockle (Anadara granosa) shells of West Coast of Peninsular Malaysia and it’s potential as biomaterial for use in bone repair. UPM institutional Repos. 6, 591–594.
Awogbemi, O., Inambao, F., and Onuh, E. I. (2020). Modification and characterization of chicken eggshell for possible catalytic applications. Heliyon 6, e05283. doi:10.1016/j.heliyon.2020.e05283
Azarian, M. H., and Sutapun, W. (2022). Tuning polymorphs of precipitated calcium carbonate from discarded eggshells: Effects of polyelectrolyte and salt concentration. RSC Adv. 12, 14729–14739. doi:10.1039/d2ra01673g
Baláž, M., Boldyreva, E. V., Rybin, D., Pavlović, S., Rodríguez-Padrón, D., Mudrinić, T., et al. (2021). State-of-the-Art of eggshell waste in materials science: Recent advances in catalysis, pharmaceutical applications, and mechanochemistry. Front. Bioeng. Biotechnol. 8, 612567. doi:10.3389/fbioe.2020.612567
Beck, R., and Andreassen, J. P. (2010). The onset of spherulitic growth in crystallization of calcium carbonate. J. Cryst. Growth 312, 2226–2238. doi:10.1016/j.jcrysgro.2010.04.037
Benhelal, E., Zahedi, G., Shamsaei, E., and Bahadori, A. (2013). Global strategies and potentials to curb CO2 emissions in cement industry. J. Clean. Prod. 51, 142–161. doi:10.1016/j.jclepro.2012.10.049
Beuvier, T., Calvignac, B., Delcroix, G. J. R., Tran, M. K., Kodjikian, S., Delorme, N., et al. (2011). Synthesis of hollow vaterite CaCO3 microspheres in supercritical carbon dioxide medium. J. Mat. Chem. 21, 9757–9761. doi:10.1039/c1jm10770d
Bharatham, H., Zakaria, M. Z. A. B., Perimal, E. K., Yusof, L. M., and Hamid, M. (2014). Mineral and physiochemical evaluation of Cockle shell (Anadara granosa) and other selected Molluscan shell as potential biomaterials. Sains Malays. 43, 1023–1029.
Borhade, A. V., and Kale, A. S. (2017). Calcined eggshell as a cost effective material for removal of dyes from aqueous solution. Appl. Water Sci. 7, 4255–4268. doi:10.1007/s13201-017-0558-9
Brečević, L., Nöthig-Laslo, V., Kralj, D., and Popović, S. (1996). Effect of divalent cations on the formation and structure of calcium carbonate polymorphs. J. Chem. Soc. Faraday Trans. 92, 1017–1022. doi:10.1039/FT9969201017
Bushuev, Y. G., Finney, A. R., and Rodger, P. M. (2015). Stability and structure of hydrated amorphous calcium carbonate. Cryst. Growth Des. 15, 5269–5279. doi:10.1021/acs.cgd.5b00771
Chakraborty, A., Parveen, S., Chanda, D. K., and Aditya, G. (2020). An insight into the structure, composition and hardness of a biological material: The shell of freshwater mussels. RSC Adv. 10, 29543–29554. doi:10.1039/d0ra04271d
Choi, S., Ph, D., Wu, S., Ph, D., Chu, J., and Ph, D. (2016). Biocementation for sand using an eggshell as calcium source. J. Geotech. Geoenviron. Eng. 142, 2–5. doi:10.1061/(ASCE)GT.1943-5606.0001534
Chong, B. W., Othman, R., Ramadhansyah, P. J., Doh, S. I., and Li, X. (2020). Properties of concrete with eggshell powder: A review. Phys. Chem. Earth Parts A/B/C 120, 102951. doi:10.1016/j.pce.2020.102951
Christy, A. G. (2017). A review of the structures of vaterite: The impossible, the possible, and the likely. Cryst. Growth Des. 17, 3567–3578. doi:10.1021/acs.cgd.7b00481
Cree, D., and Rutter, A. (2015). Sustainable bio-inspired limestone eggshell powder for potential industrialized applications. ACS Sustain. Chem. Eng. 3, 941–949. doi:10.1021/acssuschemeng.5b00035
Daengprok, W., Garnjanagoonchorn, W., and Mine, Y. (2002). Fermented pork sausage fortified with commercial or hen eggshell calcium lactate. Meat Sci. 62, 199–204. doi:10.1016/S0309-1740(01)00247-9
Dayanidhi, K., Vadivel, P., Jothi, S., and Eusuff, N. S. (2020). Facile synthesis of Silver@Eggshell nanocomposite: A heterogeneous catalyst for the removal of heavy metal ions, toxic dyes and microbial contaminants from water. J. Environ. Manage. 271, 110962. doi:10.1016/j.jenvman.2020.110962
Eggnovo (2019). Eggnovo (Spain). Available at: https://eggnovo.com/.
Essabir, H., Bensalah, M. O., Rodrigue, D., Bouhfid, R., and Qaissel kacem, A. (2017). A comparison between bio- and mineral calcium carbonate on the properties of polypropylene composites. Constr. Build. Mat. 134, 549–555. doi:10.1016/j.conbuildmat.2016.12.199
Faatz, M., Cheng, W., Wegner, G., Fytas, G., Penciu, R. S., and Economou, E. N. (2005). Mechanical strength of amorphous CaCO 3 colloidal spheres. Langmuir 21, 6666–6668. doi:10.1021/la050604m
Feng, Y., Ashok, B., Madhukar, K., Zhang, J., Zhang, J., Reddy, K. O., et al. (2014). Preparation and characterization of polypropylene carbonate bio-filler (eggshell powder) composite films. Int. J. Polym. Analysis Charact. 19, 637–647. doi:10.1080/1023666X.2014.953747
Ferreira, A. M., Vikulina, A. S., and Volodkin, D. (2020). CaCO3 crystals as versatile carriers for controlled delivery of antimicrobials. J. Control. Release 328, 470–489. doi:10.1016/j.jconrel.2020.08.061
Fombuena, V., Bernardi, L., Fenollar, O., Boronat, T., and Balart, R. (2014). Characterization of green composites from biobased epoxy matrices and bio-fillers derived from seashell wastes. Mat. Des. 57, 168–174. doi:10.1016/j.matdes.2013.12.032
Foroutan, R., Mohammadi, R., Farjadfard, S., Esmaeili, H., Ramavandi, B., and Sorial, G. A. (2019). Eggshell nano-particle potential for methyl violet and mercury ion removal: Surface study and field application. Adv. Powder Technol. 30, 2188–2199. doi:10.1016/j.apt.2019.06.034
Fu, W., Mohd Noor, M. H., Yusof, L. M., Ibrahim, T. A. T., Keong, Y. S., Jaji, A. Z., et al. (2017a). In vitro evaluation of a novel pH sensitive drug delivery system based cockle shell-derived aragonite nanoparticles against osteosarcoma. J. Exp. Nanosci. 12, 166–187. doi:10.1080/17458080.2017.1287965
Fu, X., Xu, K., Feng, X., Chang, B., Yang, F., and Huang, R. (2017b). CaCO3 nanoparticles as the tags for potentiometric detection of a cardiac biomarker using a calcium ion-selective electrode. Anal. Methods 9, 6609–6612. doi:10.1039/c7ay01949a
Habte, L., Khan, M. D., Shiferaw, N., Farooq, A., Lee, M., Jung, S., et al. (2020). Synthesis, characterization and mechanism study of green aragonite crystals fromwaste biomaterials as calcium supplement. Sustainability 12, 5062. doi:10.3390/su12125062
Hamester, M. R. R., Balzer, P. S., and Becker, D. (2012). Characterization of calcium carbonate obtained from oyster and mussel shells and incorporation in polypropylene. Mat. Res. 15, 204–208. doi:10.1590/S1516-14392012005000014
Hariharan, M., Varghese, N., Cherian, A. B., Sreenivasan, P. V., Paul, J., Benny Cherian, A., et al. (2014). Synthesis and characterisation of CaCO3 ( calcite ) nano particles from cockle shells using chitosan as precursor. Int. J. Sci. Res. Publ. 4, 1–5.
Hart, A. (2020). Mini-review of waste shell-derived materials’ applications. Waste Manag. Res. 38, 514–527. doi:10.1177/0734242X19897812
Hassan, T. A., Rangari, V. K., and Jeelani, S. (2014). Value-added biopolymer nanocomposites from waste eggshell-based CaCO 3 nanoparticles as fillers. ACS Sustain. Chem. Eng. 2, 706–717. doi:10.1021/sc400405v
Hassan, T. A., Rangari, V. K., Rana, R. K., and Jeelani, S. (2013). Sonochemical effect on size reduction of CaCO3 nanoparticles derived from waste eggshells. Ultrason. Sonochem. 20, 1308–1315. doi:10.1016/j.ultsonch.2013.01.016
Hassen, A. A., Dizbay-Onat, M., Bansal, D., Bayush, T., and Vaidya, U. (2015). Utilization of chicken eggshell waste as a bio-filler for thermoplastic polymers: Thermal and mechanical characterization of polypropylene filled with naturally derived caco3. Polym. Polym. Compos. 23, 653–662. doi:10.1177/096739111502300908
Hayeemasae, N., Song, L. W., and Ismail, H. (2019). Sustainable use of eggshell powder in the composite based on recycled polystyrene and virgin polystyrene mixture. Int. J. Polym. Analysis Charact. 24, 266–275. doi:10.1080/1023666X.2019.1567089
He, M., Forssberg, E., Wang, Y., and Han, Y. (2005). Ultrasonication-assisted synthesis of calcium carbonate nanoparticles. Chem. Eng. Commun. 192, 1468–1481. doi:10.1080/009864490896025
Hickman, G. J., Belton, D. J., Newick, R., and Perry, C. C. (2019). Barriers to adoption of biogenic carbonates in the food , pharmaceutical & supplement sectors. NFS J. 16, 1–8. doi:10.1016/j.nfs.2019.05.002
Hincke, M. T., Chien, Y. C., Gerstenfeld, L. C., and McKee, M. D. (2008). Colloidal-gold immunocytochemical localization of osteopontin in avian eggshell gland and eggshell. J. Histochem. Cytochem. 56, 467–476. doi:10.1369/jhc.2008.950576
Hincke, M. T., Nys, Y., Gautron, J., Mann, K., Rodriguez-Navarro, A. B., and McKee, M. D. (2012). The eggshell: Structure, composition and mineralization. Front. Biosci. 17, 1266–1280. doi:10.2741/3985
Hou, W., and Feng, Q. (2005). A simple method to control the polymorphs of calcium carbonate in CO 2-diffusion precipitation. J. Cryst. Growth 282, 214–219. doi:10.1016/j.jcrysgro.2005.04.102
Huang, Y. J., Ji, Y. R., Kang, Z., Li, F., Ge, S. F., Yang, D. P., et al. (2020). Integrating eggshell-derived CaCO3/MgO nanocomposites and chitosan into a biomimetic scaffold for bone regeneration. Chem. Eng. J. 395, 125098. doi:10.1016/j.cej.2020.125098
Hunton, P. (2005). Research on eggshell structure and quality: An historical overview. Rev. Bras. Cienc. Avic. 7, 67–71. doi:10.1590/s1516-635x2005000200001
Islam, K. N., Bakar, M. Z. B. A., Ali, M. E., Hussein, M. Z. B., Noordin, M. M., Loqman, M. Y., et al. (2013). A novel method for the synthesis of calcium carbonate (aragonite) nanoparticles from cockle shells. Powder Technol. 235, 70–75. doi:10.1016/j.powtec.2012.09.041
Islam, K. N., Bakar, M. Z. B. A., Noordin, M. M., HusseinBinRahman, M. Z. N. S. B. A., and Ali, M. E. (2011). Characterisation of calcium carbonate and its polymorphs from cockle shells (Anadara granosa). Powder Technol. 213, 188–191. doi:10.1016/j.powtec.2011.07.031
Islam, K. N., Zuki, A. B. Z., Ali, M. E., Bin Hussein, M. Z., Noordin, M. M., Loqman, M. Y., et al. (2012). Facile synthesis of calcium carbonate nanoparticles from cockle shells. J. Nanomater. 2012, 1–5. doi:10.1155/2012/534010
Ja, M. (2016). Antibacterial activity of ciprofloxacin-encapsulated cockle shells calcium carbonate ( aragonite ) nanoparticles and its biocompatability in. Intenational J. Mol. Sci. 17, 17050713. doi:10.3390/ijms17050713
Jaji, A. Z., Abu Bakar, M. Z. B., Mahmud, R., Loqman, M. Y., Hezmee, M. N. M., Isa, T., et al. (2017). Synthesis, characterization, and cytocompatibility of potential cockle shell aragonite nanocrystals for osteoporosis therapy and hormonal delivery. Nanotechnol. Sci. Appl. 10, 23–33. doi:10.2147/NSA.S113030
Jessop, B. M., Shiao, J. C., Iizuka, Y., and Tzeng, W. N. (2008). Prevalence and intensity of occurrence of vaterite inclusions in aragonite otoliths of American eels Anguilla rostrata. Aquat. Biol. 2, 171–178. doi:10.3354/ab00047
JustEgg Chilled Foods Ltd (2019). JustEgg chilled foods Ltd. Available at: http://justegg.co.uk/about-us/sustainability/.
Kang, D. J., Pal, K., Park, S. J., Bang, D. S., and Kim, J. K. (2010). Effect of eggshell and silk fibroin on styrene-ethylene/butylene-styrene as bio-filler. Mat. Des. 31, 2216–2219. doi:10.1016/j.matdes.2009.10.033
Lai, Y., Chen, L., Bao, W., Ren, Y., Gao, Y., Yin, Y., et al. (2015). Glycine-mediated, selective preparation of monodisperse spherical vaterite calcium carbonate in various reaction systems. Cryst. Growth Des. 15, 1194–1200. doi:10.1021/cg5015847
Lakshminarayanan, R., Loh, X. J., Gayathri, S., Sindhu, S., Banerjee, Y., Kini, R. M., et al. (2006). formation of transient amorphous calcium carbonate precursor in quail eggshell mineralization : An in vitro study. Biomacromolecules 7, 3202–3209. doi:10.1021/bm0605412
Li, H. Y., Tan, Y. Q., Zhang, L., Zhang, Y. X., Song, Y. H., Ye, Y., et al. (2012). Bio-filler from waste shellfish shell: Preparation, characterization, and its effect on the mechanical properties on polypropylene composites. J. Hazard. Mat. 217–218, 256–262. doi:10.1016/j.jhazmat.2012.03.028
Li, Y., Xin, S., Bian, Y., Xu, K., Han, C., and Dong, L. (2016). The physical properties of poly(l-lactide) and functionalized eggshell powder composites. Int. J. Biol. Macromol. 85, 63–73. doi:10.1016/j.ijbiomac.2015.12.070
Lin, P. Y., Wu, H. M., Hsieh, S. L., Li, J. S., Dong, C., Chen, C. W., et al. (2020). Preparation of vaterite calcium carbonate granules from discarded oyster shells as an adsorbent for heavy metal ions removal. Chemosphere 254, 126903. doi:10.1016/j.chemosphere.2020.126903
Liu, R., and Lian, B. (2019). Immobilisation of Cd(II) on biogenic and abiotic calcium carbonate. J. Hazard. Mat. 378, 120707. doi:10.1016/j.jhazmat.2019.05.100
Luo, X., Song, X., Cao, Y., Song, L., and Bu, X. (2020). Investigation of calcium carbonate synthesized by steamed ammonia liquid waste without use of additives. RSC Adv. 10, 7976–7986. doi:10.1039/c9ra10460g
Luo, X., Song, X., Lai, C., Wang, J., and Cao, Y. (2021). Sonochemical synthesis of vaterite-type calcium carbonate using steamed ammonia liquid waste without additives. ACS Omega 6, 23846–23854. doi:10.1021/acsomega.1c02772
Madiabu, M. J., Untung, J., Solihat, I., and Ichzan, A. M. (2021). Equilibrium and kinetic study of removal copper(II) from aqueous solution using chicken eggshells: Low-cost sorbent. molekul 16, 28–37. doi:10.20884/1.jm.2021.16.1.658
Mailafiya, M. M., Abubakar, K., Danmaigoro, A., Chiroma, S. M., Rahim, E. B. A., Moklas, M. A. M., et al. (2019). Cockle shell-derived calcium carbonate (aragonite) nanoparticles: A dynamite to nanomedicine. Appl. Sci. (Basel). 9, 2897–2925. doi:10.3390/app9142897
Matthews, S., and Asadov, A. (2020). Plasma spraying of CaCO3 coatings from oyster and mussel shell. J. Therm. Spray. Tech. 29, 1144–1171. doi:10.1007/s11666-020-01024-7
Minakshi, M., Higley, S., Baur, C., Mitchell, D. R. G., Jones, R. T., and Fichtner, M. (2019). Calcined chicken eggshell electrode for battery and supercapacitor applications. RSC Adv. 9, 26981–26995. doi:10.1039/c9ra04289j
Minakshi, M., Visbal, H., Mitchell, D. R. G., and Fichtner, M. (2018). Bio-waste chicken eggshells to store energy. Dalton Trans. 47, 16828–16834. doi:10.1039/c8dt03252a
Mony, B., Rajesh Ebenezar, A. V., Ghani, M. F., Narayanan, A., Anand, S., and Mohan, A. G. (2015). Effect of chicken egg shell powder solution on early enamel carious lesions: An invitro preliminary study. J. Clin. Diagn. Res. 9, ZC30–ZC32. doi:10.7860/JCDR/2015/11404.5656
Munusamy, Y., Sethupathi, S., and Choon, C. H. (2019). Potential use of waste cockle shell as filler for thermoplastic composite. J. Mat. Cycles Waste Manag. 21, 1063–1074. doi:10.1007/s10163-019-00867-9
Murakami, F. S., Rodrigues, P. O., De Campos, C. M. T., and Silva, M. A. S. (2007). Physicochemical study of CaCO3 from egg shells. Cienc. Tecnol. Aliment. 27, 658–662. doi:10.1590/S0101-20612007000300035
Murcia-Salvador, A., Pellicer, J. A., Rodríguez-López, M. I., Gómez-López, V. M., Núñez-Delicado, E., and Gabaldón, J. A. (2020). Egg by-products as a tool to remove direct blue 78 dye from wastewater: Kinetic, equilibrium modeling, thermodynamics and desorption properties. Mater. (Basel) 13, 1262. doi:10.3390/ma13061262
Murugan, S., Munusamy, Y., and Ismail, H. (2017). Effects of chicken eggshell filler size on the processing, mechanical and thermal properties of PVC matrix composite. Plastics Rubber Compos. 46, 42–51. doi:10.1080/14658011.2016.1260217
Owuamanam, S., and Cree, D. (2020). Progress of bio-calcium carbonate waste eggshell and seashell fillers in polymer composites: A review. J. Compos. Sci. 4, 70. doi:10.3390/jcs4020070
Palacios, C., Cormick, G., Hofmeyr, G. J., Garcia-Casal, M. N., Peña-Rosas, J. P., and Betrán, A. P. (2021). Calcium-fortified foods in public health programs: Considerations for implementation. Ann. N. Y. Acad. Sci. 1485, 3–21. doi:10.1111/nyas.14495
Pliya, P., and Cree, D. (2015). Limestone derived eggshell powder as a replacement in Portland cement mortar. Constr. Build. Mat. 95, 1–9. doi:10.1016/j.conbuildmat.2015.07.103
Portugal, S. J., Bowen, J., and Riehl, C. (2018). A rare mineral, vaterite, acts as a shock absorber in the eggshell of a communally nesting bird. Ibis Lond. 1859. 160, 173–178. doi:10.1111/ibi.12527
Putkham, A. I., Ladhan, S., and Putkham, A. (2018). Factors affecting the particle size of bio-Calcium carbonate synthesized from industrial eggshell waste. Mat. Trans. 59, 1220–1224. doi:10.2320/matertrans.MF201708
Qi, C., Zhu, Y. J., and Chen, F. (2014). Microwave hydrothermal transformation of amorphous calcium carbonate nanospheres and application in protein adsorption. ACS Appl. Mat. Interfaces 6, 4310–4320. doi:10.1021/am4060645
Qi, R. J., and Zhu, Y. J. (2006). Microwave-assisted synthesis of calcium carbonate (vaterite) of various morphologies in water - ethylene glycol mixed solvents. J. Phys. Chem. B 110, 8302–8306. doi:10.1021/jp060939s
Ramakrishna, C., Thenepalli, T., and Ahn, J. W. (2017). A brief review of aragonite precipitated calcium carbonate (PCC) synthesis methods and its applications-. Korean Chem. Eng. Res. 55, 443–455. doi:10.9713/kcer.2017.55.4.443
Rodríguez-navarro, A. B., Marie, P., Nys, Y., Hincke, M. T., and Gautron, J. (2015). Amorphous calcium carbonate controls avian eggshell mineralization : A new paradigm for understanding rapid eggshell calcification. J. Struct. Biol. X. 190, 291–303. doi:10.1016/j.jsb.2015.04.014
Santu, A., Sujith, A., Sherly, K. B., and Kurian, M. (2020). Preparation and characterization of polyvinyl alcohol and starch composite reinforced with eggshell. AIP Conf. Proc. 2263, 0018810. doi:10.1063/5.0018810
Setiawan, D., Subhan, A., and Saptari, S. A. (2017). Ca-doped LTO using waste eggshells as Ca source to improve the discharge capacity of anode material for lithium-ion battery. AIP Conf. Proc. 1862, 10–15. doi:10.1063/1.4991177
Seyahmazegi, E. N., Mohammad-Rezaei, R., and Razmi, H. (2016). Multiwall carbon nanotubes decorated on calcined eggshell waste as a novel nano-sorbent: Application for anionic dye Congo red removal. Chem. Eng. Res. Des. 109, 824–834. doi:10.1016/j.cherd.2016.04.001
Shafiu Kamba, A., Ismail, M., Tengku Ibrahim, T. A., and Zakaria, Z. A. B. (2013). A pH-sensitive, biobased calcium carbonate aragonite nanocrystal as a novel anticancer delivery system. Biomed. Res. Int. 2013, 1–10. doi:10.1155/2013/587451
Shafiur, R. G. M., Aftab, H., Shariful, I. M., Mukhlish, M. Z. B., and Ali, F. (2016). Enhanced physico-mechanical properties of polyester resin film using CaCO3 filler. Fibers Polym. 17, 59–65. doi:10.1007/s12221-016-5612-y
Sharmeeni, M., Yamuna, M., Mathialagan, M., and Ismail, H. (2016). Development of HDPE-modified eggshell composite. Polym. Compos. 18, 1630–1637. doi:10.1002/pc.24108
Shi, Y., Zhou, K., Li, D., Guyonnet, V., Hincke, M. T., and Mine, Y. (2021). Avian eggshell membrane as a novel biomaterial: A review. Foods 10, 2178–2215. doi:10.3390/foods10092178
Shuhadah, S., and Ghani, S. A. (2009). LDPE-isophthalic acid-modified egg shell powder. J. Phys. Sci. 20, 87–98.
Silvergate Plastics (2003) Silvergate plastics. Available at: https://www.silvergate.co.uk/.
Skubiszewska-Zięba, J., Charmas, B., and Waniak-Nowicka, H. (2017). Hydrothermal and mechanochemical synthesis of crystalline CaCO3. Adsorp. Sci. Technol. 35, 668–676. doi:10.1177/0263617417704298
Soldati, A. L., Jacob, D. E., Wehrmeister, U., and Hofmeister, W. (2008). Structural characterization and chemical composition of aragonite and vaterite in freshwater cultured pearls. Mineral. Mag. 72, 579–592. doi:10.1180/minmag.2008.072.2.579
Sulimai, N. H., Rani, R. A., Khusaimi, Z., Abdullah, S., Salifairus, M. J., Rusop, M., et al. (2017). One step precipitation of CaCO<inf>3</inf> nanoparticles assisted by drying and hydrothermal method. IEEE Reg. Symp. Micro Nanoelectron. One 2017, 187–190. doi:10.1109/RSM.2017.8069134
Sutapun, W., Raksakulpiwat, Y., Suppakarn, N., Jeencham, R., and Aontee, A. (2012). Characterization of precipitated calcium carbonate from eggshell powder. Adv. Mat. Res. 410, 228–231. doi:10.4028/www.scientific.net/AMR.410.228
Tan, Y. Y., Doh, S. I., and Chin, S. C. (2018). Eggshell as a partial cement replacement in concrete development. Mag. Concr. Res. 70, 662–670. doi:10.1680/jmacr.17.00003
Than, M. M., Lawanprasert, P., and Jateleela, S. (2012). Utilization of eggshell powder as excipient in fast and sustained release acetaminophen tablets. Mahidol Univ. J. Pharm. Sci. 39, 32–38.
Tiimob, B. J., Jeelani, S., and Rangari, V. K. (2016). Eggshell reinforced biocomposite - an advanced “green” alternative structural material. J. Appl. Polym. Sci. 133, 1–10. doi:10.1002/app.43124
Tiimob, B. J., Mwinyelle, G., Abdela, W., Samuel, T., Jeelani, S., and Rangari, V. K. (2017). Nanoengineered eggshell − silver tailored copolyester polymer blend film with antimicrobial properties. J. Agric. Food Chem. 65, 1967–1976. doi:10.1021/acs.jafc.7b00133
Tiles Made With Eggshell (2022). Tiles made with eggshell. Available at: https://grupoeuroatomizado.com/.
Tizo, M. S., Blanco, L. A. V., Cagas, A. C. Q., Dela Cruz, B. R. B., Encoy, J. C., Gunting, J. V., et al. (2018). Efficiency of calcium carbonate from eggshells as an adsorbent for cadmium removal in aqueous solution. Sustain. Environ. Res. 28, 326–332. doi:10.1016/j.serj.2018.09.002
Toro, P., Quijada, R., Yazdani-Pedram, M., and Arias, J. L. (2007). Eggshell, a new bio-filler for polypropylene composites. Mat. Lett. 61, 4347–4350. doi:10.1016/j.matlet.2007.01.102
Trushina, D. B., Borodina, T. N., Belyakov, S., and Antipina, M. N. (2023). Calcium carbonate vaterite particles for drug delivery : Advances and challenges. Mat. Today Adv. 14, 100214. doi:10.1016/j.mtadv.2022.100214
Trushina, D. B., Bukreeva, T. V., and Antipina, M. N. (2016). Size-controlled synthesis of vaterite calcium carbonate by the mixing method: Aiming for nanosized particles. Cryst. Growth Des. 16, 1311–1319. doi:10.1021/acs.cgd.5b01422
Tsai, W. T., Hsien, K. J., Hsu, H. C., Lin, C. M., Lin, K. Y., and Chiu, C. H. (2008). Utilization of ground eggshell waste as an adsorbent for the removal of dyes from aqueous solution. Bioresour. Technol. 99, 1623–1629. doi:10.1016/j.biortech.2007.04.010
Udrea, I., Capat, C., Olaru, E. A., Isopescu, R., Mihai, M., Mateescu, C. D., et al. (2012). Vaterite synthesis via gas-liquid route under controlled pH conditions. Ind. Eng. Chem. Res. 51, 8185–8193. doi:10.1021/ie202221m
Vandeginste, V. (2021). Food waste eggshell valorization through development of new composites: A review. Sustain. Mater. Technol. 29, e00317. doi:10.1016/j.susmat.2021.e00317
Waheed, M., Butt, M. S., Shehzad, A., Adzahan, N. M., Shabbir, M. A., Rasul Suleria, H. A., et al. (2019). Eggshell calcium: A cheap alternative to expensive supplements. Trends Food Sci. Technol. 91, 219–230. doi:10.1016/j.tifs.2019.07.021
Waheed, M., Yousaf, M., Shehzad, A., Inam-Ur-Raheem, M., Khan, M. K. I., Khan, M. R., et al. (2020). Channelling eggshell waste to valuable and utilizable products: A comprehensive review. Trends Food Sci. Technol. 106, 78–90. doi:10.1016/j.tifs.2020.10.009
Wan Mohammad, W. A. S. B., Othman, N. H., Wan Ibrahim, M. H., Rahim, M. A., Shahidan, S., and Rahman, R. A. (2017). A review on seashells ash as partial cement replacement. IOP Conf. Ser. Mat. Sci. Eng. 271, 012059. doi:10.1088/1757-899X/271/1/012059
Yang, X., and Xu, G. (2011). The influence of xanthan on the crystallization of calcium carbonate. J. Cryst. Growth 314, 231–238. doi:10.1016/j.jcrysgro.2010.11.158
Yang, Z., Peng, H., Wang, W., and Liu, T. (2011). Preparation and properties of eggshell/β-polypropylene bio-composites. J. Appl. Polym. Sci. 125, 61–66. doi:10.1002/app.34670
Yari, A. R., Majidi, G., Reshvanloo, M. T., Ansari, M., Nazari, S., Emami, M., et al. (2015). Using eggshell in acid orange 2 dye removal from aqueous solution. Iran. J. Heal. Sci. 3, 38–45.
Yoo, S., Hsieh, J. S., Zou, P., and Kokoszka, J. (2009). Utilization of calcium carbonate particles from eggshell waste as coating pigments for ink-jet printing paper. Bioresour. Technol. 100, 6416–6421. doi:10.1016/j.biortech.2009.06.112
Yu, H. D., Zhang, Z. Y., Win, K. Y., Chan, J., Teoh, S. H., and Han, M. Y. (2010). Bioinspired fabrication of 3D hierarchical porous nanomicrostructures of calcium carbonate for bone regeneration. Chem. Commun. 46, 6578–6580. doi:10.1039/c0cc01348j
Yuan, G., Chen, X., Li, X., Liang, Q., Miao, G., and Yuan, B. (2015). The synthesis of calcium carbonate microparticles with multiple morphologies through self-assembly method. Powder Technol. 284, 253–256. doi:10.1016/j.powtec.2015.06.066
Zhao, Z., Zhang, L., Dai, H., Du, Y., Meng, X., Zhang, R., et al. (2011). Surfactant-assisted solvo- or hydrothermal fabrication and characterization of high-surface-area porous calcium carbonate with multiple morphologies. Microporous Mesoporous Mat. 138, 191–199. doi:10.1016/j.micromeso.2010.09.006
Zhou, G. T., Yu, J. C., Wang, X. C., and Zhang, L. Z. (2004). Sonochemical synthesis of aragonite-type calcium carbonate with different morphologies. New J. Chem. 28, 1027–1031. doi:10.1039/b315198k
Keywords: biogenic calcium carbonate, waste seashells, waste eggshells, bio-functional materials, advanced materials
Citation: Azarian MH and Sutapun W (2022) Biogenic calcium carbonate derived from waste shells for advanced material applications: A review. Front. Mater. 9:1024977. doi: 10.3389/fmats.2022.1024977
Received: 22 August 2022; Accepted: 04 November 2022;
Published: 17 November 2022.
Edited by:
Andrea Dorigato, University of Trento, ItalyReviewed by:
Maria Harja, Gheorghe Asachi Technical University of Iași, RomaniaSuseela Alla, Technology and Research, India
Copyright © 2022 Azarian and Sutapun. This is an open-access article distributed under the terms of the Creative Commons Attribution License (CC BY). The use, distribution or reproduction in other forums is permitted, provided the original author(s) and the copyright owner(s) are credited and that the original publication in this journal is cited, in accordance with accepted academic practice. No use, distribution or reproduction is permitted which does not comply with these terms.
*Correspondence: Wimonlak Sutapun, d2ltb25sYWtAc3V0LmFjLnRo