- Institute of Civil Engineering, Central South University of Forestry and Technology, Changsha, China
In order to study the secondary consolidation characteristics of organic soil improved by bio-enzyme, the secondary consolidation test was carried out. First, the Gibson rheological model parameters were fitted according to the experimental results. Then, the relationship between rheological model parameters, Ca/Cc value, secondary consolidation coefficient Ca, and load was analyzed for different ratios of bio-enzyme. The results showed that: 1) the rheological model parameters were negatively correlated with the ratios of bio-enzyme and positively correlated with the load value. The rheological model parameters E1 (Kelvin elastic modulus) and η (Kelvin viscosity coefficient) reached the maximum value when the ratio of bio-enzyme was 0.01%. 2) The secondary consolidation coefficient was related to the load and showed a certain law. At 100 kPa, the secondary consolidation coefficient of samples reached the peak. When it was less than 200 kPa, the secondary consolidation coefficient changed obviously with the increase in load. When the load was greater than 200 kPa, the variation trend of the secondary consolidation coefficient tended to be gentle with the increase in load and finally tended to be constant. With the increase in load, the secondary consolidation coefficient Ca finally approached to a stable value. 3) The value of Ca/Cc of improved organic soil varied from 0.042 to 0.1 under various loads. In this article, the secondary consolidation characteristics of organic soil modified by bio-enzyme were studied. The secondary consolidation strain can be predicted by the established rheological model parameter equations.
1 Introduction
Organic matter in soil could enter the interlaminar space of the soil structure, which leads to soil expansion and reduces shear strength and permeability (Zhu et al., 2019a). Organic matter mainly consists of C, N, and other organic compounds, including monosaccharides, polysaccharides, and humus with complex structure. The main component of humus is humic acid, which has a porous spongy structure and is more hydrophilic and absorbent than clay minerals. Organic matter is widely distributed in natural soils. According to the content of organic matter in soil, soil can be divided into peat, peat soil, inorganic soil, and organic soil. The content of organic matter in organic soils is about 5%–10%.
Researchers conducted a number of experiments to study the effect of organic matter on soil. The content of organic matter in soil could significantly affect the engineering properties of soil (Canakci et al., 2015). The presence of organic matter reduced soil compactness and peak strength values. Tremblay et al. (2002) analyzed the effects of organic matter on the shear strength of soil and showed that the presence of organic matter significantly affected the strength of soil. Therefore, it can be found that the presence of organic matter has an adverse effect on the strength of soil (Nath et al., 2017).
Because of the existence of organic matter in soil, organic soil has the characteristics of low pH value, large porosity, high natural water content, high compressibility, high plasticity, and low bearing capacity (Saride et al., 2013). Therefore, scholars began to search for traditional amendments to treat organic soil. Saride et al. (2013) used lime, cement, and fly ash to improve organic soil, which could effectively improve the strength of organic soil. Zhu et al. (2019b) showed that modified magnesium oxide cement as a modifier could effectively improve organic soil. However, the aforementioned traditional methods had the disadvantages of high CO2 emission, being unfriendly to the environment, and high engineering costs. The use of traditional modifiers, such as cement and lime, leads to more environmental pollution and greenhouse gas emissions (Ramdas et al., 2020). Therefore, this article finds a new method of improvement to address the urgent need.
The bio-enzyme soil curing agent (hereinafter referred to as bio-enzyme) is a new soil improver extracted from plant fermentation. It could replace the traditional inorganic material improver to a certain range. Bio-enzyme has the characteristics of non-toxic, non-corrosive, and environmental protection (Chen et al., 2019). Soil improvement by bio-enzymes is a reasonable method to improve soil stability (Ramdas et al., 2020). Soil improvement by bio-enzymes could effectively improve and maintain soil strength and hydraulic properties (Pooni et al., 2019). Soil improvement by bio-enzymes could significantly improve the mechanical properties of soil and improve the compressive strength and shear strength of soil (Chen et al., 2019). Therefore, the technology of soil improvement by bio-enzymes has gradually matured. However, none of the aforementioned studies involved the improvement of organic soil by bio-enzymes, so in this article, the improvement of organic soil by bio-enzyme was studied.
Rheology includes creep, stress relaxation, long-term strength, and elastic after-effect. The secondary consolidation of soil was the creep of soil. The non-uniform settlement deformation and post-construction settlement deformation of buildings were related to the secondary consolidation characteristics of soil (Mesri, 1977). Therefore, the study of secondary consolidation is very important (Li et al., 2021). The traditional method of constructing rheological constitutive equations of soil is widely used. Traditional methods include the empirical method and the element method. The element method is the most commonly used rheological constitutive model construction method because of its few parameters, clear physical meaning, and simple form. A method for determining rheological model parameters proposed by Gibson in 1962 was adopted in this article. The Gibson rheological model, namely, the Merchant model, consists of a Hook body and a Kelvin body in series. In this article, the model was used to describe the secondary consolidation characteristics of organic soil modified by bio-enzyme.
To sum up, this article carried out the secondary consolidation test of organic soil improved by bio-enzyme. The Gibson rheological model parameters E0 (Hook elastic modulus), E1 (Kelvin elastic modulus), and η (Kelvin viscosity coefficient) were negatively correlated with the ratios of bio-enzyme. When the ratio of bio-enzyme was 0.01%, E1 and η reached the maximum value. At the same time, the secondary consolidation coefficient was related to the load. The secondary consolidation coefficient of samples with different ratios of bio-enzyme under different loads showed a certain rule. The Ca/Cc values ranged from 0.042 to 0.1, which is consistent with Mesri’s results (Mesri, 1977). The rheological model parameter equations can be used to describe the secondary consolidation of modified organic soil.
2 Materials and methods
2.1 Materials
2.1.1 Source of test soil
The test soil in this article was taken from Changsha at a depth of 1–1.5 m. The soil was brownish yellow and uniform. The main physical and mechanical indexes are shown in Table 1.
2.1.2 Bio-enzyme reagents
The biological enzyme soil curing agent ( referred to as bio-enzyme) used in this experiment is Terra-Zyme, developed by Nature Plus Co., Ltd. The enzyme is a brown viscous liquid product with 60% plant enzyme content and a pH value between 4.3 and 5.3. It is non-toxic and pollution-free and easily soluble in water, with a special odor and good water stability, as shown in Figure 1.
2.1.3 Preparation of soil samples
In this experiment, soil with different percentages of organic matter was artificially remolded. The researchers used sodium humate and fulvic acid as additional organic matter to reshape organic soils with varying amounts of organic matter (Xu et al., 2007). Fulvic acid was used as the additive organic material in this study. The ratios of bio-enzyme in soil were calculated as the ratio of enzyme mass to the dry mass of soil. Organic soil samples with bio-enzyme ratios of 0.00%, 0.01%, 0.02%, 0.03%, and 0.04% (plant enzyme ratios of 0.00%, 0.006%, 0.012%, 0.018%, and 0.024%, respectively) were prepared.
2.2 Test methods
The consolidation instrument used in the test was the WG single lever consolidation instrument, as shown in Figure 2. The sample height was 2 cm, and the cross-sectional area was 30 cm2. According to Highway Geotechnical Test Regulations (JTG 3430-2020), the test adopted the method of graded loading, with six grades of loads: 25, 50, 100, 200, 400, and 800 kPa. The next level of load could be added after each level of load was stabilized. Manual readings were taken to record the test data.
2.3 Test results and analysis
2.3.1 Coefficient of consolidation Cv
According to Highway Geotechnical Test Regulations (JTG 3430-2020), the consolidation coefficient Cv was calculated by the time square root method. According to Eq. 1, the consolidation coefficients under different ratios of bio-enzyme and different load grades were calculated, as shown in Figure 3.
where H is half of the average of the initial height and final height of the sample under a certain level of load and t90 is the time required for the sample consolidation degree to reach 90%.
Figure 3 shows the following relationship between the load and consolidation coefficient of organic soil improved by bio-enzyme:
(1) The consolidation coefficient decreased with the increase in load under different ratios of bio-enzyme. When the load was less than 200 kPa, the consolidation coefficient changed obviously with the load. When the load was greater than 200 kPa, the consolidation coefficient tended to be stable gradually.
(2) The consolidation coefficient decreased first and then increased with the increase in ratios of bio-enzyme under the same load.
2.3.2 Coefficient of secondary consolidation Ca
The e-lgt relation curve of the secondary consolidation test of organic soil improved by bio-enzyme is shown in Figure 4. The standard deviation of e-lgt relation curve data in Figure 4 varied from 0.0041 to 0.0061. Figure 4 shows that the abscissa of the tangent line of the curve part of the e-lgt relation curve of soil under different loads intersected the extension line of the straight segment approximately at 1,380 min. Therefore, the start time of secondary consolidation in this test was 1,380 min.
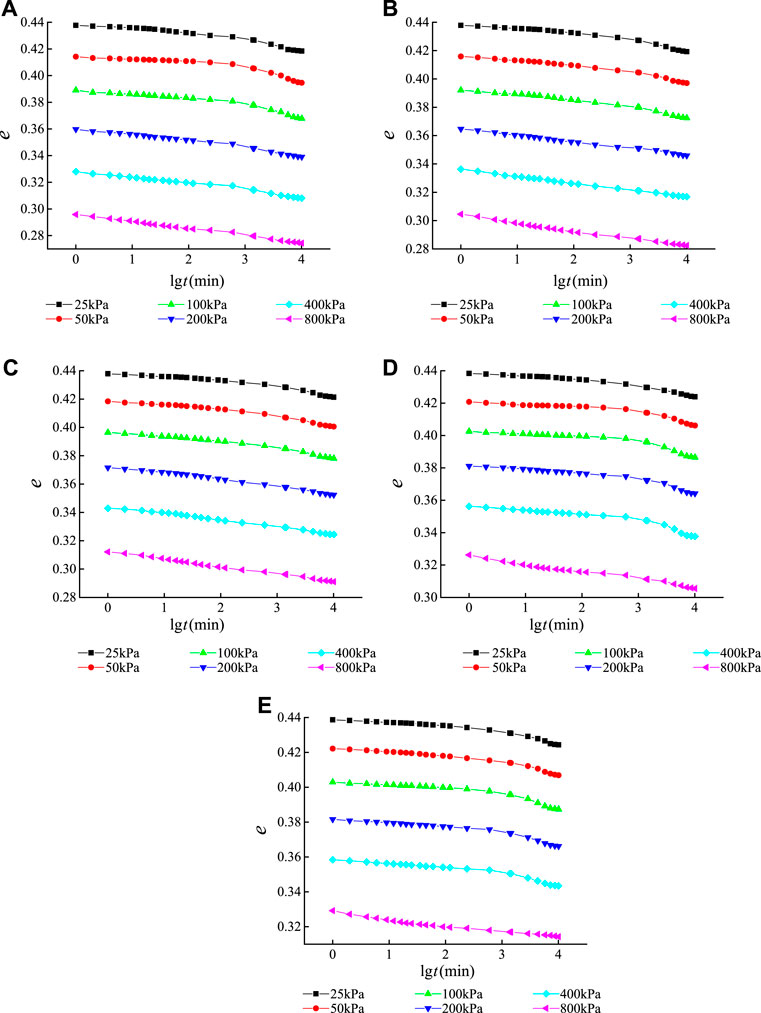
FIGURE 4. e-lgt relation curves: (A) bio-enzyme ratio of 0.00%, (B) bio-enzyme ratio of 0.01%, (C) bio-enzyme ratio of 0.02%, (D) bio-enzyme ratio of 0.03%, and (E) bio-enzyme ratio of 0.04%.
The method proposed by Buisman to determine the secondary consolidation coefficient by e-lgt relation curves is as follows:
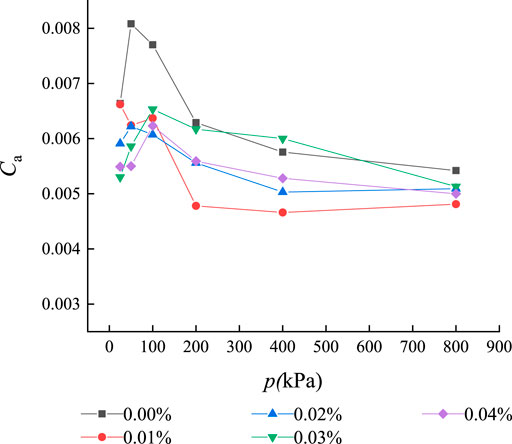
FIGURE 5. Relation curves between the secondary consolidation coefficient Ca and load with different ratios of bio-enzyme.
2.3.3 Compression index Cc
According to Highway Geotechnical Test Regulations (JTG 3430-2020), the compression index was calculated using the following method:
Walker (1969) found that Ca/Cc was about 0.025. Wang et al. (2015) showed that the Ca/Cc ratio of different clays varied between 0.02 and 0.05. Mesri (1977) summarized the secondary consolidation tests of 22 soils and concluded that Ca/Cc of the same soil had a certain value ranging from 0.025 to 0.1. This conclusion had also been recognized by most scholars. Mesri (1973) further pointed out that the Ca/Cc ratio was 0.04 ± 0.01 for most inorganic soils and 0.05 ± 0.01 for organic soils. When the ratio of bio-enzyme was 0.02%, Ca and Cc of the sample roughly met the linear relationship (as shown in Figure 7). Its slope was 0.02469, and the absolute value of the correlation coefficient R of linear fitting was 0.86825. The relationship curve between Ca/Cc and load is shown in Figure 8. According to Figure 8, the Ca/Cc values of bio-enzyme-modified organic soil varied from 0.042 to 0.1 under various loads, which was consistent with Mesri’s conclusions (Mesri 1977). Therefore, the Ca/Cc values could be used to estimate the Ca value of organic soil improved by bio-enzyme.
3 Determining the rheological model parameters
3.1 Gibson rheological model
Although the construction method of the rheological constitutive model of rock and soil has made great progress, the traditional construction method of the rheological constitutive equation of rock and soil is still widely used. The element method, as the most commonly used traditional method, is particularly prominent in geotechnical engineering numerical analysis and theoretical calculation. This article introduced a method for determining rheological model parameters proposed by Gibson in 1962. Gibson used the three-component rheological model (the Merchant model, which consists of a Hook body and a Kelvin body in series) to describe the stress–strain relationship of soil:
After the pore pressure disappears:
where t0 is the pore pressure dissipation time (secondary consolidation start time), E0 is the elastic modulus of the Hook body, E1 is the elastic modulus of the Kelvin body, η is the viscosity coefficient of the Kelvin body, and σ′ is the incremental load.
The elastic modulus E0 of the Hook body was obtained from the final settlement under a certain level of load. The elastic modulus E1 and viscosity coefficient η of the Kelvin body were determined by fitting the strain–time (y-t) relationship curve:
3.2 Fitting of rheological model parameters of organic soil modified by bio-enzyme
The secondary consolidation part of the strain–time (y-t) curve had a good linear correlation. Therefore, according to the Gibson data processing method, the secondary consolidation of the strain–time (y-t) relationship curves was linearly fitted. According to Eq. 4, the y-t curves fitting the results of samples with different ratios of bio-enzymes are shown in Tables 2–6. It can be seen from Tables 2–6 that the fitting effect is good under loading at all levels. The correlation coefficients were above 0.88.
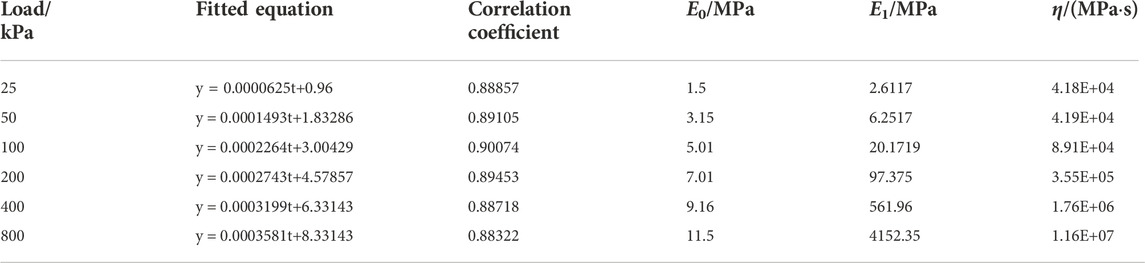
TABLE 2. Rheological model fitting results and parameters of the samples when the ratio of bio-enzyme was 0.00%.
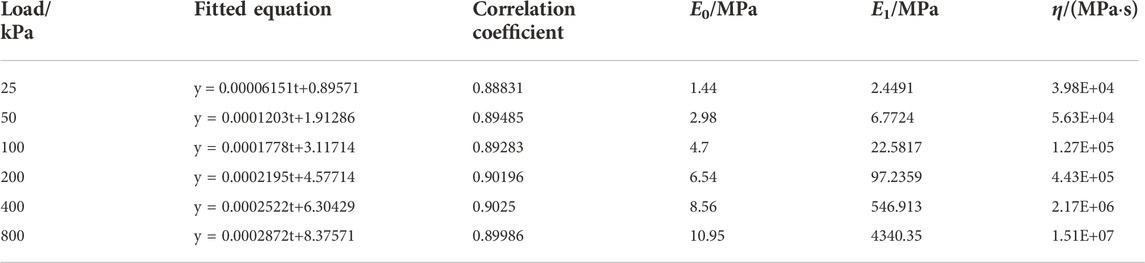
TABLE 3. Rheological model fitting results and parameters of the samples when the ratio of bio-enzyme was 0.01%.
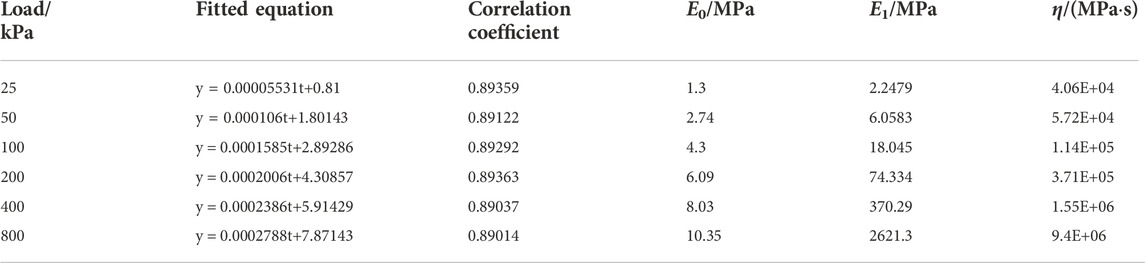
TABLE 4. Rheological model fitting results and parameters of the samples when the ratio of bio-enzyme was 0.02%.
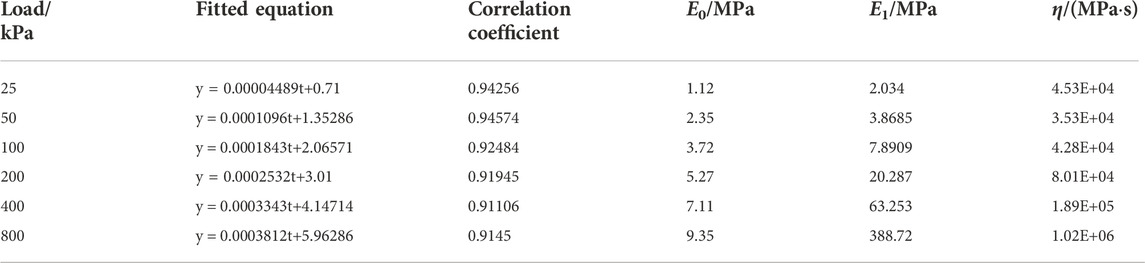
TABLE 5. Rheological model fitting results and parameters of the samples when the ratio of bio-enzyme was 0.03%.
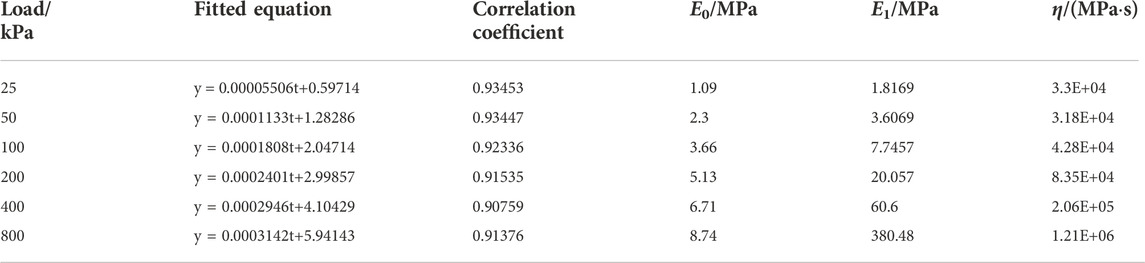
TABLE 6. Rheological model fitting results and parameters of the samples when the ratio of bio-enzyme was 0.04%.
3.3 The relationship between rheological model parameters and load
The rheological model parameters of organic soil improved by bio-enzyme are shown in Figure 9. According to Figure 9, the rheological model parameters E0, E1, and η of organic soil improved by bio-enzyme were roughly negatively correlated with the ratios of bio-enzyme. Parameters E0, E1, and η were positively correlated with loads. When the ratio of bio-enzyme was 0.01%, the parameters E1 and η reached the maximum value.
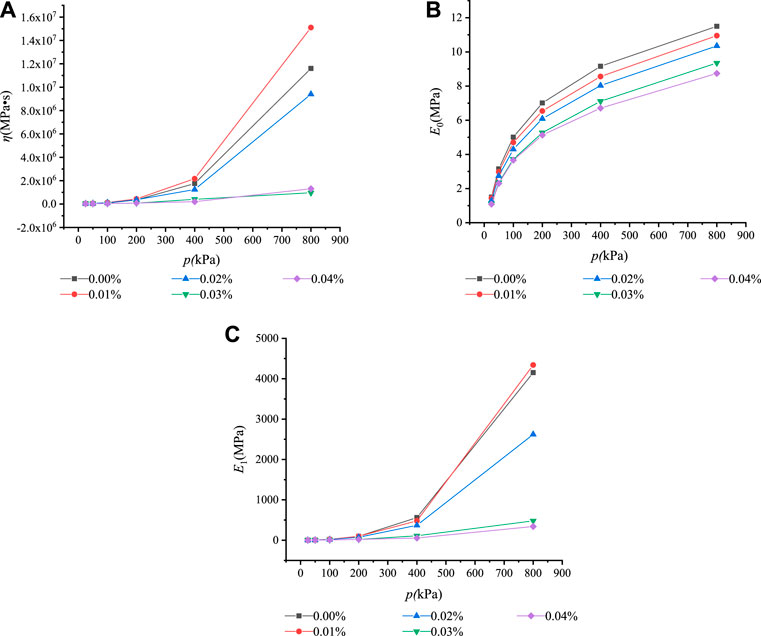
FIGURE 9. Relationship curves between rheological model parameters and load under different ratios of bio-enzyme: (A) relationship between modulus
3.4 Establishment of the rheological model with different ratios of bio-enzyme
The empirical relationship between model parameters and loads under different ratios of bio-enzyme was established as shown in Eqs 5–9 (Z is different ratios of bio-enzyme
4 Conclusion
In order to study the secondary consolidation characteristics of organic soil improved by bio-enzyme, the secondary consolidation experiment was carried out. The Gibson rheological model parameters were fitted according to the experimental results. The relationship between rheological model parameters and loads under different ratios of bio-enzyme was analyzed. The main conclusions of this article are as follows:
(1) The rheological model parameters E0, E1, and η of organic soil improved by bio-enzyme were roughly negatively correlated with the ratios of bio-enzyme. Parameters E0, E1, and η were positively correlated with loads. When the ratio of bio-enzyme is 0.01%, the parameters E1 and η reached the maximum value.
(2) The secondary consolidation coefficient reached its peak at about 100 kPa. As the load increased, the secondary consolidation coefficient tended to a stable value. When the load is less than 200 kPa, the consolidation coefficient changed obviously with the load. When the load was greater than 200 kPa, the consolidation coefficient tended to be stable gradually. The compression index increased with the increase in load. Under the same load, the compression index decreased with the increase in ratios of bio-enzyme.
(3) The secondary consolidation characteristics of organic soil modified by bio-enzyme were studied. The rheological model parameter equations can be used to describe the secondary consolidation of modified organic soil.
(4) The microscopic mechanism of the secondary consolidation characteristics of organic soil improved by bio-enzyme is worth further study in the future.
Data availability statement
The original contributions presented in the study are included in the article/Supplementary Material; further inquiries can be directed to the corresponding author.
Author contributions
YZ wrote the first draft of the manuscript. YZ contributed with the literature search, illustrations, and writing of the manuscript. YZ contributed to manuscript revision, read and approved the submitted version. CW contributed to read and approved the submitted version.
Funding
The work is supported by the National Special Program for International Scientific and Technology Cooperation (Grant No. 2014DFA53120)and the International Science and Technology Cooperation Program. The work is supported by the Science and Technology Innovation Program of Hunan Province (2020RC4049).
Conflict of interest
The authors declare that the research was conducted in the absence of any commercial or financial relationships that could be construed as a potential conflict of interest.
Publisher’s note
All claims expressed in this article are solely those of the authors and do not necessarily represent those of their affiliated organizations, or those of the publisher, the editors, and the reviewers. Any product that may be evaluated in this article, or claim that may be made by its manufacturer, is not guaranteed or endorsed by the publisher.
References
Canakci, H., Sidik, W., and Kilic, I. H. (2015). Effect of bacterial calcium carbonate precipitation on compressibility and shear strength of organic soil. Soils Found. 55 (5), 1211–1221. doi:10.1016/j.sandf.2015.09.020
Chen, Y. Q., Wen, C. P., and Fang, X. Q. (2019). Modified Yin’s double-yield-surface model for bioenzyme-treated expansive soil. Rock Soil Mech. 40 (9), 3515–3523. doi:10.16285/j.rsm.2018.1004
Li, J. H., Zhao, S. W., Wang, X., Hao, J. Q., Zhi, B., Zhang, H., et al. (2021). Experimental study on consolidation characteristics of compacted loess. Adv. Civ. Eng. 2021, 1–12. doi:10.1155/2021/6687858
Mesri, G. (1973). Coefficient of secondary compression. J. Soil Mech. Found. Div. 99 (1), 123–137. doi:10.1061/jsfeaq.0001840
Mesri, G., and Godlewski, P. M. (1977). Time and stress-compressibility interrelationship. J. Geotech. Engrg. Div. 103 (5), 417–430. doi:10.1061/ajgeb6.0000421
Nath, B. D., Molla, M. K. A., and Sarkar, G. (2017). Study on strength behavior of organic soil stabilized with fly ash. Int. Sch. Res. Notices 2017, 1–6. doi:10.1155/2017/5786541
Pooni, J., Giustozzi, F., Robert, D. J., Setunge, S., and O'Donnell, B. (2019). Durability of enzyme stabilized expansive soil in road pavements subjected to moisture degradation. Transp. Geotech. 21, 100255. doi:10.1016/j.trgeo.2019.100255
Ramdas, V. M., Mandree, P., Ramchuran, S., Lalloo, R., and Mukaratirwa, S. (2020). Review of current and future bio-based stabilisation products (enzymatic and polymeric) for road construction materials. Transp. Geotech. 27, 100458. doi:10.1016/j.trgeo.2020.100458
Saride, S., Puppala, A. J., and Chikyala, S. R. (2013). Swell-shrink and strength behaviors of lime and cement stabilized expansive organic clays. Appl. Clay Sci. 85, 39–45. doi:10.1016/j.clay.2013.09.008
Tremblay, H., Duchesne, J., Locat, J., and Leroueil, S. (2002). Influence of the nature of organic compounds on fine soil stabilization with cement. Can. Geotech. J. 39 (3), 535–546. doi:10.1139/t02-002
Walker, L. K. (1969). Undrained creep in a sensitive clay. Géotechnique 19 (4), 515–529. doi:10.1680/geot.1969.19.4.515
Wang, D. X., Abriak, N. E., and Zentar, R. (2015). One-dimensional consolidation of lime-treated dredged harbour sediments. Eur. J. Environ. Civ. Eng. 19 (2), 199–218. doi:10.1080/19648189.2014.939309
Xu, R. Q., Guo, Y., and Liu, Z. Y. (2007). Experimental study on mechanical properties of stabilized artificial organic soil. J. Zhejiang Univ. Eng. Sci. 41 (1), 109–113. doi:10.3785/j.issn.1008-973X.2007.01.020
Yu, X. J., Yin, Z. Z., and Dong, W. J. (2007). Influence of load on secondary consolidation deformation of soft soils. Chin. J. Geotechnical Eng. 29 (6), 913–916. doi:10.3321/j.issn:1000-4548.2007.06.021
Zhu, J. F., Rao, C. Y., Yu, Q. S., Liu, H. X., and Pan, B. J. (2019b). Experimental study on the properties of the organic soil solidified by the composite magnesium oxysulfate cement-curing agent. Chin. J. Rock Mech. Eng. 38 (S1), 3206–3214. doi:10.13722/j.cnki.jrme.2018.0658
Keywords: subgrade engineering, organic soil improved by bio-enzyme, Gibson three-element rheological model, secondary consolidation test, different ratios of bio-enzyme
Citation: Zhang Y and Wen C (2022) Secondary consolidation characteristics of organic soil modified by bio-enzyme based on the Gibson model. Front. Mater. 9:1002987. doi: 10.3389/fmats.2022.1002987
Received: 25 July 2022; Accepted: 02 September 2022;
Published: 16 December 2022.
Edited by:
Wen Zhong, University of Manitoba, CanadaReviewed by:
Rui Zhang, Changsha University of Science and Technology, ChinaFrancesco Todaro, Politecnico di Bari, Italy
Copyright © 2022 Zhang and Wen. This is an open-access article distributed under the terms of the Creative Commons Attribution License (CC BY). The use, distribution or reproduction in other forums is permitted, provided the original author(s) and the copyright owner(s) are credited and that the original publication in this journal is cited, in accordance with accepted academic practice. No use, distribution or reproduction is permitted which does not comply with these terms.
*Correspondence: Yixin Zhang, MTIyMzM0MzMzN0BxcS5jb20=
†ORCID: Yixin Zhang, orcid.org/0000-0001-9879-9823