- 1Beijing Key Laboratory of Traffic Engineering, College of Metropolitan Transportation, Faculty of Architecture, Civil and Transportation Engineering, Beijing University of Technology, Beijing, China
- 2School of Civil Engineering and Transportation, Beijing University of Civil Engineering and Architecture, Beijing, China
- 3Department of Civil and Environmental Engineering, Michigan Technological University, Houghton, MI, United States
Lignin is the second-largest plant polymer on Earth after cellulose. About 98% of lignin produced in the papermaking and pulping industry is used for combustion heating or power generation. Less than 2% of lignin is used in more valuable fields, mainly in the formulation of dispersants, adhesives, and surfactants. Asphalt is one of the most important materials in pavement engineering. It is a dark brown complex mixture composed of hydrocarbons with different molecular weights and their non-metallic derivatives. Because the chemical structure of lignin is similar to that of asphalt, it is a carbon-based hydrocarbon material. More researchers studied the application of lignin in pavement engineering. In this paper, the structure, application, and extraction technology of lignin were summarized. This is a review article describing the different applications of lignin in pavement engineering and exploring the prospects of the application. There are three main types of pavement materials that can be used for lignin in pavement engineering, which are asphalt, asphalt mixture, and roadbed soil. In asphalt, lignin can be used as a modifier, extender, emulsifier, antioxidant, and coupling agent. In asphalt mixtures, lignin can be used as an additive. In road base soils, lignin can be used as a soil stabilizer. Furthermore, the article analyzed the application effects of lignin from the life cycle assessment. The conclusions suggest that lignin-modified asphalt exhibits more viscosity and hardness, and its high-temperature resistance and rutting resistance can be significantly improved compared with conventional asphalt. In addition, some lignin-modified asphalt binders exhibit reduced low-temperature crack resistance and fatigue resistance, which can be adjusted and selected according to the climate change in different regions. The performance of lignin as an asphalt mixture additive and asphalt extender has been proved to be feasible. Lignin can also produce good mechanical properties as well as environmental benefits as a soil stabilizer. In summary, lignin plays an important role in asphalt pavement and roadbed soil, and it is likely to be a development trend in the future due to its environmental friendliness and low cost. More research is needed to generalize the application of lignin in pavement engineering.
1 Introduction
The name lignin originated from the Latin word lignum, which means wood. Lignin is widely found in plants and is the second-largest plant polymer on Earth after cellulose. It accounts for 30% of all-natural non-fossil organic carbon, and it is the natural raw material with the highest aromatic content. The papermaking and pulping industry are the main sources of lignin. Global pulping and papermaking facilities produce about 50–70 million tons of lignin every year, most of which are kraft lignin. In addition, the production of second-generation biofuels also produces organic solvent lignin, mainly hydrolyzed lignin. Lignin is an important part of the plant cell wall, as it enables plants to maintain sufficient stiffness and hardness, as shown in Figure 1. Chemically speaking, lignin is a cross-linked phenolic polymer. Specifically, it is a complex phenolic polymer formed by three alcohol monomers (p-coumaroyl alcohol, coniferyl alcohol, and sinapyl alcohol). Lignin has different functional groups such as hydroxyl, methoxyl, carbonyl, and carboxyl. More refined lignin products, such as binders and surfactants, can be obtained through degradation. Degradation of lignin can be done by chemical, thermal, or enzymatic pathways (Cao et al., 2018). The phenolic structure of lignin is a benzene ring with hydroxyl. A benzene ring is six carbon structures, each of which shares one or two covalent bonds with another carbon. In phenols, one or more hydroxyls can be attached to the benzene ring. Phenols, as antioxidants, can neutralize free radicals in the electrical group by providing protons and electrons. Lignin contains a large number of phenolic groups and can serve as an excellent and potent antioxidant. The different biological sources of lignin determine the differences in its antioxidant capacity. In addition, the antioxidant capacity of extracted lignin varies among different extraction methods, such as lignin extracted from plants through chemicals such as ethanol, acetone, acetic acid, methanol, and propanol.
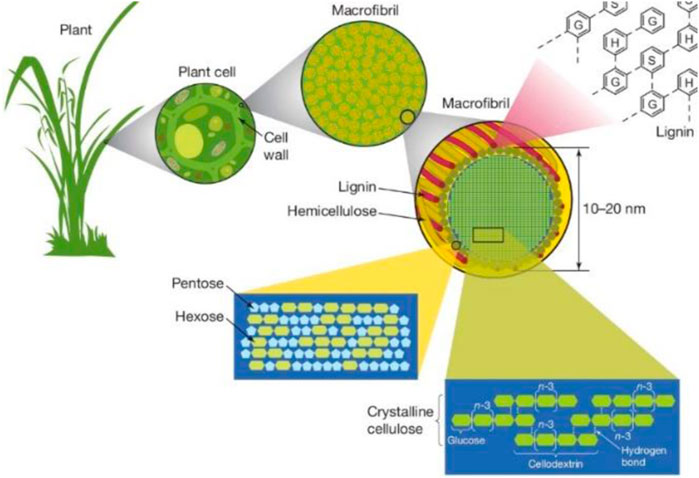
FIGURE 1. Lignin structure in the plant cell wall (Wu et al., 2017).
The paper and pulp industry is a major source of lignin, with current annual pulp and paper facilities producing approximately 50–70 million tons of lignin, mostly kraft lignin, worldwide. In addition, the production of second-generation biofuels also produces the organic solvent lignin, mainly hydrolysis lignin. About 98% of lignin produced by the paper and pulp industries is used for combustion heating or power generation, which is low in value, with less than 2% lignin used in more valuable areas, mainly in formulations of dispersants, binders, and surfactants. In addition to its application to industrially-based chemicals, polymers, and biofuels, lignin can be exploited for the development of nanomaterials for drug delivery (Figueiredo et al., 2018).
The lignin is not only used in chemical engineering but also can be utilized in pavement engineering materials and achieved remarkable effects. Asphalt is one of the most important materials in pavement engineering, and it is a black-brown complex mixture that is composed of hydrocarbons with different molecular weights and non-metallic derivatives. Asphalt is widely used in the construction of road infrastructures such as pavement, airport runways, and parking lots. Whether it is a rural road, urban trunk road, or expressway, it must involve the use of asphalt. Usually, asphalt is mixed with aggregate to form an asphalt mixture, which is used for the laying of subgrade and pavement. In addition, asphalt is also a widely used material in road maintenance, such as repairing cracks, pits, ruts, etc. However, asphalt comes from petroleum, coal coke, and other materials, which increases greenhouse gas emissions and causes damage to the environment when paving the road. In order to reduce greenhouse gas emissions, researchers have begun to pay attention to pavement construction methods involving energy conservation and emission reduction, recycling asphalt mixture, looking for alternative materials for asphalt, or improving the service performance of the pavement.
Because the chemical structure of lignin is similar to that of asphalt and because lignin is a carbon-based hydrocarbon material, more and more people have begun to study the application of lignin in asphalt pavement in recent years. The most prominent application of lignin in pavement engineering was to add asphalt as an asphalt modifier, which can significantly improve the aging resistance of asphalt and had different effects on the high-temperature rheological property, low-temperature crack resistance, and fatigue resistance of asphalt (Xu et al., 2017; Luo et al., 2019; Gao et al., 2020; Norgbey et al., 2020; Yu et al., 2021). In general, the modified asphalt has two or more modifiers to improve the performance of asphalt. Lignin can be used as a modifier coupling agent to enhance the combination of modifier and asphalt (Wu et al., 2021). In addition, the amination of lignin also allows the preparation of cationic emulsifiers for asphalt emulsion (Yuliestyan et al., 2017). Lignin can also be used as an asphalt filler (Pérez et al., 2019; Zhang et al., 2020a; Wu et al., 2021). It can reduce the consumption of asphalt and achieve the effect of energy conservation, emission reduction, and cost reduction. In 2010, a bike path paved with lignin-modified bio-asphalt was tested in Iowa, United States, with good results (Bourzac, 2015). In 2012, the mechanism of lignin-modified asphalt binder was analyzed (Pan, 2012). In 2015, the Dutch government built a pavement with lignin polymer for asphalt (Bourzac, 2015). In 2016, lignin was discussed as a soil stabilizer in Australia (Chen and Indraratna, 2015). In 2017, in the United States, the potential of lignin as a partial replacement and improver for asphalt binders was evaluated (Xu et al., 2017). In 2018 in Brazil, the performance of lignin applications for asphalt binders was analyzed (Batista et al., 2018). In 2019, the effect of lignin on the properties of asphalt and asphalt mixtures was studied in the United States (Arafat et al., 2019). In the same year, lignin was also studied as a soil stabilizer (Zheng et al., 2019). In 2020, lignin was researched as a bio additive for asphalt modification in China (Gao et al., 2020). In 2020, another study investigated the effect of lignin on the properties of asphalt binders in China (Norgbey et al., 2020). Besides, in the same year, the effect of lignin fibers on the Open-Graded Friction Course (OGFC) mixture was also studied (Zhang et al., 2020a). In 2021, a life cycle assessment of lignin fibers in asphalt mixtures was investigated (Khater et al., 2021). In Netherlands, a life cycle assessment of asphalt using kraft lignin was conducted (Moretti et al., 2022). In the Netherlands and China, molecular dynamics analysis on lignin modified asphalt was analyzed (Ren et al., 2021). Obviously, in the last 2 years, more and more studies have been conducted for lignin in asphalt pavements and road soil bases (Li et al., 2020a; Wu et al., 2021; Yu et al., 2021), and this paper provides a specific review of the application of lignin in them.
In recent years, more than 6,000 tons of asphalt per year was used in China, 80% of which is used for road construction and maintenance. Figure 2 shows the price and consumption of asphalt in China in recent years. In China, the price of lignin is about RMB 1500 per ton, while the price of asphalt is RMB 3500 per ton. From an economic point of view, the use of lignin in the asphalt binder industry can save costs, and several studies have shown that lignin can improve the performance of the asphalt pavement, making it an excellent material for prospective applications.
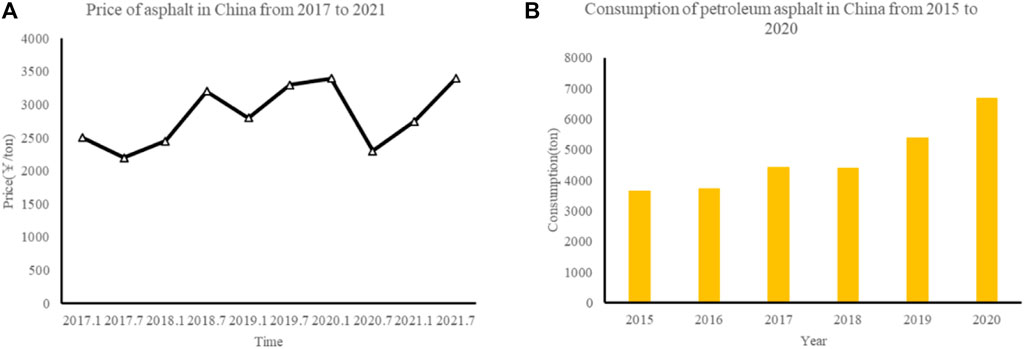
FIGURE 2. (A) Price of asphalt in China from 2017 to 2021 (B) Consumption of petroleum asphalt in China from 2015 to 2020.
This literature review is a review article on lignin and its application in pavement engineering. The first chapter is an introduction. Section 2 describes the basic overview of lignin, reviewing the structure, properties, applications, and extraction processes, respectively. The Section 3 summarizes the application of lignin in pavement engineering, which is divided into three main areas, lignin in asphalt, lignin in asphalt mixtures, and lignin in soil bases. The Section 4 is the conclusion.
Based on the literature review, the general situation and status of the lignin are briefly reviewed and explored. These characteristics of lignin were summarized including the structure, physicochemical properties, engineering applications, and manufacturing technologies. The applications of lignin in pavement engineering are also introduced and discussed from asphalt modifiers, asphalt fillers, asphalt coupling agents, soil stabilizers, life cycle assessment of lignin in the flexible pavement as well as the current and future development trend.
2 Lignin
2.1 Structure
Lignin is a renewable organic resource with aromatic properties, which widely exists in plants and is second only to cellulose in quantity. Lignin is mainly sourced from the paper and pulp industries, with a worldwide annual production of over 50 million tons, but the majority of lignin is used in fuel combustion for low-value electricity generation and heating, and only 2% of lignin is sourced to produce higher utilization chemical products. Lignin is a kind of complex high molecular organic polymer that is mainly composed of carbon, hydrogen, and oxygen. Its structural unit is the amorphous polymer network compound of phenylpropane. There are three types of structural units: p-coumaryl alcohol, coniferyl alcohol, and sinapyl alcohol. Because of their different monomers, the lignin compositions can be classified into three types: syringyl lignin, guaiacyl lignin, and para-hydroxy-phenyl lignin. Figure 3 shows the above three alcohol monomers and corresponding units of lignin.
2.2 Physical and Chemical Properties
The physical and chemical properties of lignin are mainly divided into the following four aspects: Molecular Weight, Dispersity Index, Solubility, and Thermal Properties (Calvo-Flores, 2020). Due to the diversity of lignin in plants and the difficulty of preparation, the molecular weight of lignin is still controversial. The molecular weight range of isolated lignin is about 103–105. Dispersion index is a parameter used to describe polymers and to measure molecular weight distribution. Soluble lignin and its derivatives exhibit significant dispersion, and their values may vary from 2.5–3.0 to 10–11 depending on the source of lignin. Naive lignin is insoluble in water and organic solvents because its structure is an aggregate with many hydroxyl groups present, and because lignin molecules contain many hydrogen bonds, there is strong intramolecular energy within lignin, leading to lignin being very difficult to dissolve.
Like other polymers, lignin can be characterized by parameters such as glass transition temperature (TG). Glass transition temperature is a parameter used when amorphous or semi-crystalline polymers change from a glass state to a viscous and rubbery state. At present, the TG value of lignin is known to be between 200 and 500°C.
2.3 Application
For many years, lignin’s primary use has been in fuel combustion heating or power generation, and this application is not economic. Since lignin is an abundant and renewable aromatic compound feedstock with the potential to synthesize aromatic chemical products, in recent years researchers have focused on the extraction and utilization of lignin to convert it into aromatic platform chemicals and chemical products to make more efficient and environmentally friendly use of plant polymers abundant in nature. Lignin is biocompatible, eco-friendly, low toxic, and sensitive to enzymatic degradation (Meng et al., 2019). Therefore, it can produce many high-value functional materials, such as asphalt emulsifier, fuel dispersant, resin adhesive, soil amelioration agent, rubber reinforcing agent, water coal slurry additive, water reducing agent for concrete, grinding agent for cement, viscosity reducer for heavy oil, a surface-active agent for oil extraction, etc., (Cao et al., 2018). Figure 4 shows the lignin products and applications that can be produced by different extraction methods or different types of lignin.
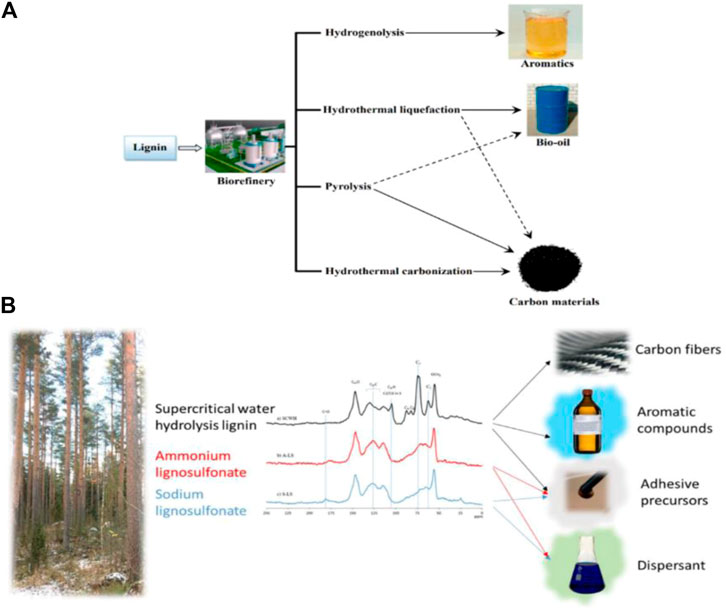
FIGURE 4. (A) Biorefinery process of lignin (Cao et al., 2018) (B) Application of different lignin (Hemmilä et al., 2019).
Lignin can be isolated by physical, chemical, and biological methods (Cao et al., 2018). The physical method is to obtain high purity lignin by breaking the link between lignin and cellulose or hemicellulose by steam explosion or mechanical grinding under high temperature and high pressure. The chemical approach is to depolymerize lignin using technology to produce aromatic compounds or other compounds such as aromatics or ethylbenzene. It has been found that the embedding of nickel nanoparticles in lignin-derived carbon can improve the stability of lignin hydrolysis catalyst and the bond-breaking ability of lignin (Wang et al., 2019). Figure 5 shows the schematic representation of embedding nickel nanoparticles into lignin-derived carbon. The biological method is to break the chemical link between lignin and carbohydrates using enzymes under mild conditions.
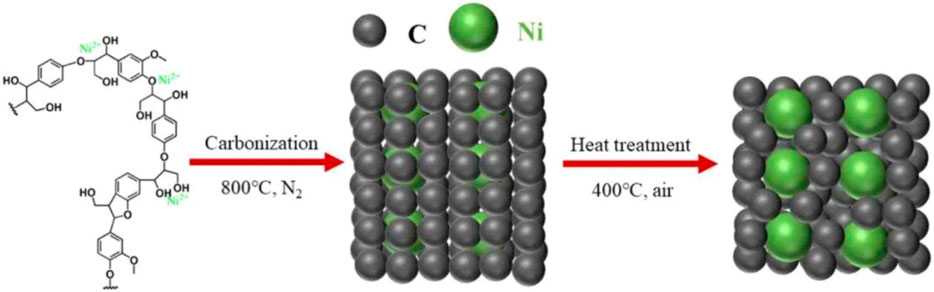
FIGURE 5. Schematic illustration of the construction of an “inlaid type” catalyst (Wang et al., 2019).
Lignin pyrolysis can decompose to produce a large number of chemicals, including char, synthases, hydrogen, and aromatics, which can serve as alternatives to conventional fuels. In industry, lignin can undergo chemical reactions to produce different chemicals with different properties that can give rise to a wide variety of uses (Mahmood et al., 2016; Cao et al., 2018; Figueiredo et al., 2018; Bajwa et al., 2019; Tribot et al., 2019). Lignosulfonate, a byproduct of the paper industry, has strong water absorption, as well as the effect of strong chelating agents that trap metal ions and form complexes. Lignosulfonate can also be used as concrete admixtures, feed binders, soil stabilizers, dispersants, dye modifiers, air absorbents in mortar, expanders, and asphalt emulsifiers.
There are structures such as hydroxyl groups and aromatic groups in lignin, which can react chemically with other polymers, leading to depolymerization products of lignin that can synthesize bio-based epoxy resins, polyesters, etc. Some studies have found that epoxy resins can improve the anti-aging properties of asphalt, which is also one of the applications of lignin in road construction. An additional depolymerization product of lignin is polyurethanes, and in comparison to those traditionally derived from treatments utilizing diisocyanates and dolichols, the polyurethanes prepared from lignin are more environmentally friendly, have better performance, and can be widely used in the shipbuilding industry, automotive industry, and furniture packaging industry.
Additionally, some studies have shown that lignin phenolic resin has been effectively used as a binder, and it can also be utilized as insulation foam, achieving good economic and environmental benefits. Because lignin has a high carbon content, lignin can also serve as a raw material for carbon fibers. Carbon fibers are initially produced from polyacrylonitrile and are costly, but lignin is expected to save the cost of carbon fiber production. Figure 6 shows the molecular model of lignin and carbon fibers, which indirectly shows that lignin can be used as the raw material of carbon fiber.
In addition, lignin has some other uses such as improving the performance of sodium-ion batteries, and lignin and silicon nanoparticle mixing can be used as a composite electrode for electrochemical reactions. Lignin has been successfully used to produce different nanomaterials such as nanoparticles, nanotubes, nanofibers, and hydrogels for different applications. Lignin-based nanoparticles have found applications in functional surface coatings, as well as in biomedical applications such as medicine, gene delivery, and tissue engineering. Lignin is a good flame retardant. The presence of methoxy and hydroxyl groups within lignin, which has a complex aromatic profile and can act as an antioxidant, has applications in biomedical fields, food engineering, and cosmetics manufacturing.
2.4 Types of Lignin and Extraction Process
According to the descriptions in the literature (Bajwa et al., 2019; Calvo-Flores, 2020), the most common lignins are kraft lignin, sulfite lignin, soda lignin, organic solvent lignin, hydrolyzed lignin, and steam-exploded lignin. Kraft pulping is one of the mainstream pulping processes, in which sulfide is used to decompose lignin into low molecular units to obtain kraft lignin in an alkaline environment at 170 degrees. Sulfated lignin can be used in fertilizers, pesticides, carbon fibers, adhesives, and resins. Figure 7 shows the models of four different types of lignin.
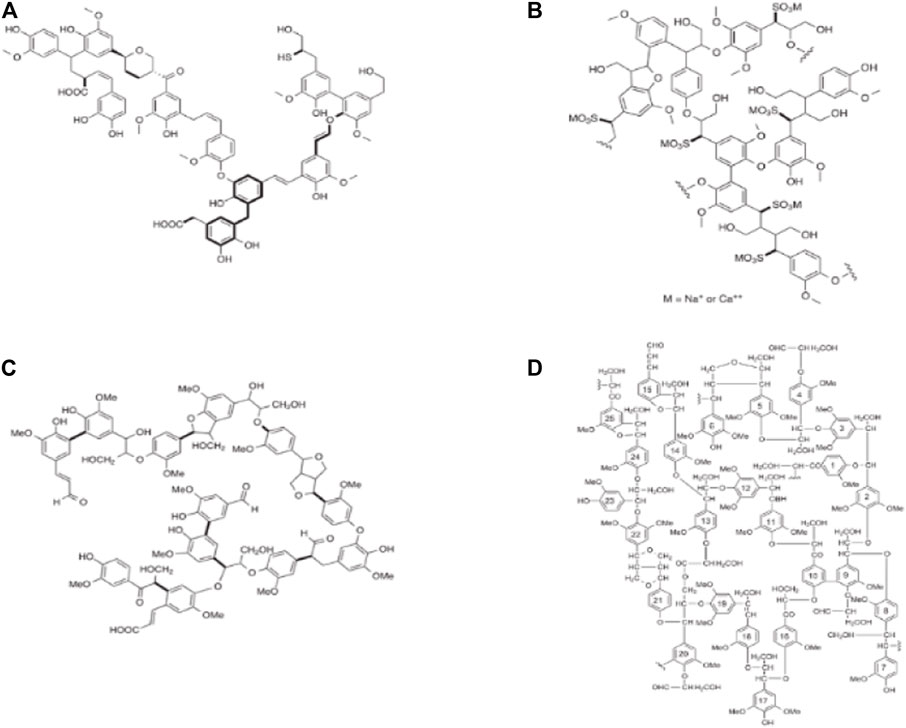
FIGURE 7. Models of different lignins (Calvo-Flores, 2020) (A) Kraft lignin; (B) Sulfite lignin; (C) Lignin for hardwood species; (D) Lignin for softwood species.
Lignin is processed with a solution containing sulfite or bisulfite in an acidic environment from 50 to 160 degrees to break the ether bonds of lignin to yield sulfite lignin. Sulfite lignin can produce different types of products such as colloidal suspensions, stabilizers, dispersants, adhesives, detergents, feed ingredients, particleboards, and cement additives. Soda lignin is a product obtained from the digestion of non-wood materials (rice straw, sugarcane bagasse, flax) with aqueous sodium hydroxide at a temperature of 160 degrees. Soda lignin can be used for the production of phenolics, animal nutrition, dispersants, and polymer synthesis. Organic solvent lignin is obtained by direct extraction from biomass of polymers using organic solvents, of which the most widely utilized organic solvent is alcohol. Side products like hydrolyzed lignin are also produced during the production process of lignocellulose, and hydrolyzed lignin is also produced by hydrolyzing and dissolving carbohydrates from cellulases. Steam-exploded lignin can be obtained when utilizing steam to treat wood in a high-temperature and high-pressure environment, based on principles similar to the production of hydrolyzed lignin. It is worth noting that lignin can be classified according to whether or not it contains sulfur. Sulfated lignin and sulfite lignin are sulfur-containing lignin, while soda lignin, organic solvent lignin, hydrolytic lignin, and steam explosion lignin are sulfur-free lignin.
3 Application of Lignin in Pavement Engineering
Lignin is a high molecular polymer composed of carbon, hydrogen, and oxygen elements, and it has abundant active groups such as aromatic ring structures, aliphatic and aromatic hydroxyl groups, and quinone groups. Its structure is similar to asphalt, as shown in Figure 8. Therefore, the vast majority of lignin applications in the field of pavement engineering are associated with asphalt. The second use of lignin is in asphalt mixtures, where it is usually added in the form of fibers. Another important application of lignin in the field of pavement engineering is as a soil stabilizer. In this section, the application of lignin in pavement engineering is presented from three perspectives: asphalt binder, asphalt mixture, and soil stabilizer.
3.1 Lignin in Asphalt
Asphalt is a complex black-brown mixture composed of hydrocarbons with different molecular weights and their non-metallic derivatives. It is a kind of high-viscous organic liquid. It exists mostly in liquid or semi-solid petroleum form with a black surface and is soluble in carbon disulfide and carbon tetrachloride. In pavement engineering, asphalt is used as the cementitious material for pavement structure, and the asphalt mixture can be obtained by proportionally matching different mineral ratios. Lignin can be used as an asphalt modifier, additive, antioxidant, coupling agent, and emulsifier.
3.1.1 Lignin as an Asphalt Modifier
As an asphalt modifier, lignin has attracted more and more attention because of its wide source, low cost, environmental friendliness, and potential to improve the performance of asphalt pavement. This section summarizes the performance of lignin-modified asphalt in recent years.
A lignin-modified asphalt (Yu et al., 2021) was developed by adding soda lignin powder in different proportions to asphalt. A series of tests were conducted, such as the viscosity test, rutting test, fatigue test, and cracking test, to verify the performance of the modified asphalt. The results show that the addition of lignin helps to reduce the temperature sensitivity, increase the hardness, reduce the ductility, reduce the low-temperature cracking performance, and increase the viscosity of base asphalt. In addition, the rheological test results showed that the addition of soda lignin significantly improved the rutting resistance and fatigue properties at all addition doses.
Lignin powder was added to the asphalt binder (Xu et al., 2017), and the road performance of the modified asphalt was tested through rheological, fatigue cracking, rutting, and other tests. The results show that the viscosity of lignin-modified asphalt binder is higher than that of basic binder, and lignin significantly improves the rutting resistance of asphalt binder at high temperatures. However, lignin may have a negative impact on the fatigue resistance of the asphalt binder. The spectral test revealed that lignin can prevent the formation of carbonyl functional groups in the aging process of asphalt binder, which shows that lignin can be used as an antioxidant modifier and has good environmental and economic benefits.
The effect of waste lignin on the performance of asphalt binder was analyzed (Norgbey et al., 2020), and tests were conducted on the characteristics, morphology, decomposition behavior, low and high-temperature behavior, fatigue resistance, storage stability, and adhesion to an aggregate of the composite binder according to the standard. The results show that the increase of lignin content improved the cohesion and hardenability of the adhesive. The low-temperature flow characteristics of the binder decreased with the increase of wood content. The rutting resistance of lignin-modified asphalt was stronger at a higher temperature. The presence of lignin significantly increased the viscosity of the asphalt binder. The adhesion between lignin-modified asphalt and aggregate was better.
The effects of lignin and glass fiber as modifiers of asphalt mixture were reviewed (Luo et al., 2019). Different from the above articles, lignin and glass fibers were used as modifiers in this study. After a series of performance tests, the results showed that the double mixing technology has a good effect on improving the overall performance of the asphalt mixture. Lignin fiber improves the low-temperature crack resistance of the asphalt mixture, while glass fiber plays a great role in improving the high-temperature performance of the asphalt mixture. Through the research, it was concluded that the optimal dosage of lignin fiber is 0.2–0.4% of asphalt mixture, and the optimal dosage of glass fiber is about 0.2–0.6% of asphalt mixture.
Different concentrations of lignin were added to asphalt as biological additives to form lignin-modified asphalt (Gao et al., 2020). The results show that the addition of lignin improves the viscosity of the original asphalt. Modified asphalt has better deformation resistance because lignin increases the elastic components in asphalt and makes the base asphalt binder harder. Adding lignin to asphalt can delay the aging of asphalt. However, adding lignin may reduce the fatigue life of the asphalt.
In addition, lignin derivatives can also be used as asphalt modifiers. In one study, lignin-based polyurethane (Peng et al., 2020) was prepared with lignin and toluene diisocyanate and was added to the matrix asphalt binder. The lignin-based polyurethane modified asphalt binder was tested, and the bonding performance of the asphalt binder was evaluated. The results show that the adhesion performance of this modified asphalt was better than that of matrix asphalt. The reason is that the anhydride group and lignin benzene ring in lignin-based polyurethane modified asphalt binder can increase the polarity, which is very important to the adhesion performance of asphalt binder.
The asphalt binder modification test of five fiber types (two polyesters, one polyacrylonitrile, one lignin, and one asbestos) was designed (Chen and Xu, 2010), and it was found that fiber can effectively improve the rutting resistance, fluidity resistance, and dynamic shear modulus of asphalt binder, while lignin fiber has better asphalt absorption function.
3.1.2 Lignin as Asphalt Extender
When more than 10%wt lignin is added to asphalt, it is usually regarded as an extender of asphalt. In this case, more than 10%wt of asphalt can be replaced by lignin so as to reduce the use of non-renewable asphalt, which would not only save cost but also protect the environment. The possibility of lignin as an asphalt filler (Wu et al., 2021) was investigated. Two materials, SK (an asphalt with a penetration grade of 70) and LMB (lignin modified bitumen) were used for a control test. The thin-film-oven-test (TFOT) was carried out on each of the two materials. Finally, the raw materials were compared with the tested materials, and three-dimensional coordinate diagrams of ductility, softening point, and penetration of four materials (SK, LMB, SK-TFOT, LMB-TFOT) were drawn (Figure 9) Finally, the penetration index (PI) was calculated. The results preliminarily proved that adding lignin to asphalt can significantly reduce the temperature sensitivity. The researchers fixed the mass ratio of lignin to asphalt at 1:4. Various properties of asphalt were evaluated by viscosity, rheology, and aging resistance tests. The results show that the hydroxyl and carbonyl groups in lignin show obvious chemical activities, which significantly affect the properties of asphalt from different angles. After adding lignin, the hardness of asphalt increases, which shows that the permeability and ductility decrease, the softening point and composite modulus increase, and the temperature sensitivity of asphalt decreases. At the same time, the mixing and paving temperature of pavement construction increased by more than 10°C. The presence of abundant associative hydroxyl in lignin is the main factor to change the aging resistance of asphalt. Therefore, we believe that lignin as a sustainable asphalt filler is promising and brings environmental benefits.
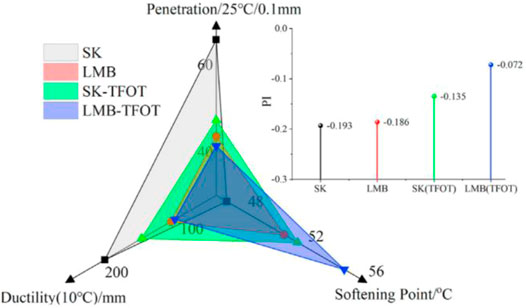
FIGURE 9. Comparison of physical properties for virgin and aged bitumen (Wu et al., 2021).
The use of liquid waste containing lignin (generated from the production of wooden cardboard) as asphalt filler (Pérez et al., 2019) in the production of asphalt mixture was investigated. Additions of 0, 5, 10, 20, and 40% industrial wastes were added into the asphalt mixture, and the adhesion, water damage resistance, elastic modulus, permanent deformation resistance, and thermal sensitivity of asphalt aggregate were analyzed. Finally, it was determined that the effect of 20% industrial waste is the best, as the mixture of lignin and asphalt mixture has high fatigue resistance at low temperature and high permanent deformation resistance at high temperature. On the basis of ensuring functionality, it has a good economic benefit.
3.1.3 Other Applications in Asphalt
The above three uses are the most mainstream applications of lignin in pavement engineering. In addition, lignin can also be used as an asphalt emulsifier, antioxidant, and coupling agent.
The application of kraft lignin as a cationic emulsifier (Yuliestyan et al., 2017) in the preparation of asphalt emulsion was studied. An emulsifier is an amphiphilic substance with a hydrophilic head and lipophilic coil. It is located at the interface between asphalt droplets and the water phase, plays the role of stabilizer, reduces the interfacial tension between two phases, and promotes the emulsification process. The cationic emulsifier can adhere to electronegative siliceous solid aggregate to achieve the effect of emulsification. The cationic emulsifier of asphalt emulsion was successfully prepared by the reaction of kraft lignin with four ethylene, five amines, and formaldehyde in an alkaline medium.
In another study (Xu et al., 2021), the α-cinnamon group as the lipophilic group and diethylenetriamine as the hydrophilic group were introduced into alkaline lignin to construct lignin-based amphiphilic cationic surfactants. After a series of tests, the results showed that the amphiphilic cationic surfactant modified by alkali lignin had a cationic form and good stability in pH = 3 aqueous solutions and can be used as an asphalt emulsifier. Lignin was modified by amination as a cationic emulsifier and added to asphalt binders to form asphalt emulsions stabilized by modified lignin, which were combined with reclaimed asphalt pavements as aggregates to create asphalt mixes with minimal environmental impact and high recycling efficiency (Yuliestyan et al., 2018).
The aging of asphalt is divided into two stages. The initial stage is the rapid oxidation stage. After a certain time, the speed slows down and enters the second stage, with slow oxidation and hardening speed. The oxidation of asphalt and the antioxidant mechanism of coniferous lignin (Pan, 2012) were simulated, and the composition and structure of chemicals were analyzed, and it was found that the previous stage was caused by the high chain-breaking trend of asphalt and the high reactivity of oxygen. After the rapid formation of light molecular weight alkanes, the oxidation reaction of asphalt slowed down gradually. Lignin can be used as an asphalt oxidant because it contains a large number of saturated hydrocarbons, which can replace asphalt chain breaking, so as to play an antioxidant effect. It should be noted that when lignin is added to asphalt, the mixing temperature needs to be controlled to prevent lignin oxidation.
Lignin can also be used as a coupling agent. Sometimes two or more materials are used as the modifier of asphalt to improve the performance of different aspects. Lignin can be used as the coupling agent of these materials to better integrate with asphalt and achieve the best effect of modified asphalt.
3.2 Lignin in Asphalt Mixtures
In addition to adding lignin into the asphalt binder to prepare modified asphalt, lignin can also be directly added to the asphalt mixture as an additive. Asphalt mixtures are typical elastic, viscous, and plastic complexes that are close to linear elastomers in low temperature and small deformation range, close to visco-plastics in high temperature and large deformation range, and close to visco-elastomers in the general temperature transition range. To evaluate the performance of asphalt pavement is to evaluate the material properties of asphalt mixtures, which include high-temperature stability, low-temperature crack resistance, moisture susceptibility, fatigue resistance, and aging resistance. Research on lignin fibers as additives in asphalt mixtures is popular, with emphasis on evaluating the performance of asphalt mixtures with the addition of lignin fibers, and several experiments can be done to evaluate the different properties of the above-mentioned asphalt mixtures.
The addition of four typical fibers as additives to the open-graded friction layer mixture was evaluated (Zhang et al., 2020a). The drainage performance, raveling resistance, rutting resistance, stiffness, and fatigue life of the mixtures were evaluated through various tests. The results show that the fiber improves the overall performance of the mixture, and the lignin fiber density is small, which has the best effect on the drainage performance of the mixture. However, due to the low tensile strength of lignin fiber, it has the least improvement on the mechanical properties of the asphalt mixture. It was concluded that the optimum content of lignin fiber is 0.3%. Figure 10 shows the lignin fibers and their SEM microstructures. Another study (Wang et al., 2012) on the incorporation of lignin fibers into an open-graded friction course (OGFC) showed that the tensile strength, water susceptibility, and abrasion of OGFC were improved and moisture susceptibility was reduced. What’s more, the addition of lignin fibers to OGFC also reduced abrasion loss and maintenance requirements.
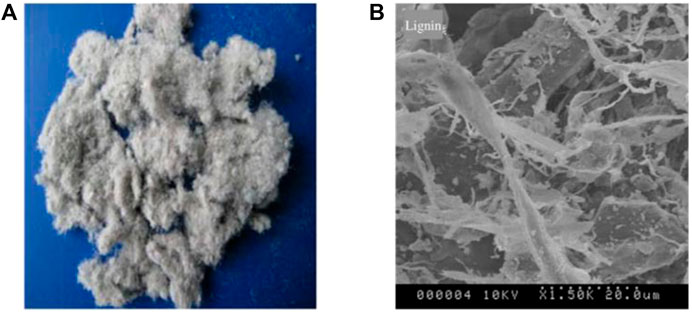
FIGURE 10. (A) Lignin fibers (Chen et al., 2009); (B) Scanning Electronic Modules (SEM) microstructure of lignin fibers (Chen and Xu, 2010).
Bagasse fiber (Li et al., 2020b) was added to the asphalt mixture, and the performance of the lignin fiber asphalt mixture was compared. The results show that adding fiber into the asphalt mixture can form a three-dimensional network, enhance the skeleton structure of the asphalt mixture, and reduce the fluidity of the asphalt mixture. Bagasse fibers can also absorb saturated hydrocarbons and aromatics on the surface to enhance the interfacial adhesion between asphalt mastic and aggregate. In this way, the high-temperature stability and low-temperature crack resistance of the mixture were significantly improved. Therefore, we believe that bagasse and lignin fiber can play the same role and are good additives for asphalt mixtures.
Similar to the asphalt binder, two or more admixtures can be used to enhance the different properties of the asphalt mixtures. After adding the anti-rutting agent to the asphalt mixtures (Fu et al., 2016), the high-temperature performance of the asphalt mixture was significantly improved, but the low-temperature performance was not improved. In contrast, adding only lignin fiber to the mixture can significantly improve the low-temperature performance but not the high-temperature performance. The researchers added two admixtures at the same time, which could improve the high and low-temperature performance and moisture susceptibility at the same time. The test results show that the optimum content of double admixtures in AC-13 and AC-16 asphalt mixtures is 0.4% anti-rutting agent and 0.36% lignin fiber, which can greatly improve the high- and low-temperature performance and moisture susceptibility of asphalt mixtures.
The volume properties, mechanical properties, and design methods of fiber-reinforced asphalt mixture (Chen et al., 2009) were studied. Four different fibers were used, and the Marshall test and performance test were performed. The results showed that after adding fiber into the asphalt mixture, the optimum asphalt content, void ratio, mineral aggregate void ratio, and Marshall stability of the asphalt mixture were improved, while the volume-specific gravity is reduced. Two different forms of lignin products added to Stone Mastic Asphalt (SMA) were studied (Zhang et al., 2020b). Lignin powders and lignin fibers were incorporated into SMA respectively, and the raveling, rutting, thermal and fatigue cracking resistance, and moisture susceptibility of the two lignin-modified asphalt mixtures were evaluated. The results show that both forms of lignin can improve the rutting resistance and thermal cracking resistance of asphalt mixtures. However, lignin powders improved the abrasion resistance, fatigue performance, and moisture susceptibility of asphalt mixtures, while lignin fibers reduced these properties. It can be seen that different forms of lignin have different effects on the performance of asphalt mixtures.
3.3 Lignin in Soil for Road Bases
Another major use of lignin in pavement engineering is as a soil stabilizer for road subgrade soils. The roadbed soil is the foundation for building roads and railroads, and the stability of the roadbed soil is the basis for ensuring the stable service of the road. Materials, such as lime, Portland cement, and fly ash, are usually used as stabilizers for roadbed soils, but these traditional materials have some disadvantages in terms of environmental protection. Lignin is an environmentally friendly, low-energy, low-cost soil stabilizer that can bind soil particles together tightly, fill pores, and form a more stable soil structure. Many studies have been conducted on the incorporation of lignin soil stabilizers for different types of soils.
The use of lignin for the stabilization of silty soils has been studied (Zhang et al., 2018a; Zhang et al., 2018b). Lignin-stabilized silty soils exhibit good strength and bearing capacity, and the lignin stabilizer has a low heavy metal content and is an environmentally friendly chemical mixture. A study (Cai et al., 2014) of lignin treatment of silt suggested a stabilization mechanism in which positively charged lignin polymers acted as a cementing agent and encapsulated individual soil particles together, subsequently forming particle clusters or aggregates that make the soil structure more stable. In Jiangsu Province, China, a study (Zhang et al., 2016) on lignin-stabilized silty soils has come to the same conclusion, showing that the lignin industrial by-product had great potential to improve the engineering properties of some poor soils. The study (Chen and Indraratna, 2015) of sandy silt showed that the use of lignosulfonate as stabilizers can increase the life of the soil bases after cyclic loading. Similarly, a study (Singh and Sahoo, 2021) on clay soils subjected to cyclic loading and came to a similar conclusion that the addition of lignosulfonate to clay soils increased the service life of the soil matrix. In particular, the addition of lignin polymer compounds to loess soils in northwestern China (Liu et al., 2019) not only increased soil strength but most importantly, reduced the environmental impact. Alkali lignin (Zheng et al., 2019) was used as a soil stabilizer and the study showed that heat-curing can increase the strength of the soil by more than 40%, which may be caused by the water content in the soil. Therefore, the interaction between lignin, soil, and water can be studied in more depth in subsequent studies to obtain the best benefits. Lignin can also be coupled with other materials as a soil stabilizer (Ijaz et al., 2020), and good results have been achieved by coupling lignosulphonate with hydrated lime as an expansive soil stabilizer. In terms of performance, lignin soil stabilizers can improve the compressive strength, freeze-thaw durability, moisture susceptibility, and shear strength of soil bases (Ceylan et al., 2010; Kim et al., 2012; Uzer, 2016; Yang et al., 2018). Economically, lignin is produced in many industrial waste products, such as by-products of the bioethanol industry (Liu et al., 2020a), so using these materials as soil stabilizers is a choice that combines performance and economy. Lignin is a material with great potential to be used even in reinforcing dams (Koohpeyma et al., 2013). Therefore, more research should be conducted in the future to investigate the application of lignin.
3.4 Life Cycle Assessment About Using Lignin in Flexible Pavement
The addition of polymers to asphalt pavements in road construction reduces energy consumption and contributes to the good technical properties of the pavement. It is necessary to include both economic and social effects of the use of renewable materials as variables in the life cycle assessment (Balaguera et al., 2018). The use of recycled materials in asphalt layers can reduce gas emissions during the construction phase (AzariJafari et al., 2016). Lignin has good economic and social effects as a renewable polymer material.
Several studies have performed a life cycle analysis of lignin in asphalt. Based on the LCA (Life cycle analysis) methodology, kraft lignin was used to modify a variety of asphalts (stone matrix asphalt, asphalt concrete, and porous asphalt) and compared with the conventional asphalt (Moretti et al., 2022). The results show that the use of lignin in asphalt can substantially reduce the climate change impact of the top-layer asphalt product over its life cycle. How additional process heat is produced in the pulp mill during the production of the material determines the overall environmental impact. Furthermore, the results of the road performance of different lignin-modified asphalts show that the maximum substitution of bitumen with lignin should be targeted to improve environmental benefits and that the substitution of weak fillers also needs to be limited.
Substitution of poor quality asphalt with lignin is beneficial for climate change and may lead to worse overall environmental performance than conventional asphalt. The environmental impact of asphalt mixture additives based on the LCA method was evaluated and analyzed in the study of pavement wearing layer construction (Khater et al., 2021). The results show that lignin-modified asphalt mixture had a slightly negative impact, compared to glass modified and composite mixtures. Lignin-modified asphalt had the best environmental impact on most of the other impact categories, except for the two indicators of freshwater aquatic ecotoxicity and marine aquatic ecotoxicity. Definitely, the use of lignin in asphalt can reduce emissions and climate impact.
The price of asphalt has been rising rapidly in recent years because of the increasing and unstable price of crude oil. In 2018, the cost of bitumen in Australia (Tokede et al., 2020) has been close to $470 per tonne, while commercial lignin cannot be specified at a specific price because its cost assessment needs to involve factors such as extraction technology, process, and paper mill equipment costs, and the cost of lignin is estimated by other studies (Dessbesell et al., 2018) to be about $700 per tonne. Therefore, at present, lignin replaces part of the asphalt in cost loss, but with the further increase in the cost of asphalt, in the future, if the demand for lignin increases, driving down the cost of lignin extraction, lignin is expected to become a popular asphalt replacement material.
3.5 Effect of Lignin in Pavement Engineering
Based on the above application of lignin in asphalt, lignin is a good asphalt substitute. It can not only reduce the amount of asphalt in pavement engineering but also improve the road performance of the asphalt mixture.
The most common application of lignin in pavement engineering is as an asphalt modifier. Research on lignin or lignocellulose as an asphalt modifier is becoming more and more prevalent. Some new environmentally friendly asphalt modifiers often used lignin-modified asphalt as the control (Calabi-Floody and Thenoux, 2012; Li et al., 2020c; Aljubory et al., 2021). Lignin is used as an asphalt modifier because of its wide source, low cost, and environmental benefits. The most important benefit is that it can improve the performance of asphalt pavement. In the literature, alkali lignin powder, lignin powder, waste lignin, and lignin fiber have been used as asphalt modifiers (Chen and Xu, 2010; Xu et al., 2017; Luo et al., 2019; Gao et al., 2020; Norgbey et al., 2020; Yu et al., 2021). Compared with original base asphalt, it is generally found that lignin-modified asphalt has strong viscosity, hardness, and high-temperature rutting resistance while slightly reducing the low-temperature crack resistance and fatigue resistance of modified asphalt. From the perspective of molecular dynamics (Ren et al., 2021), lignin can improve the density, cohesive energy density, shear viscosity, modulus, and bond strength of asphalt. However, with the increase of lignin content, the free volume, diffusion coefficient, and self-healing ability of lignin-modified asphalt decrease. This can also explain the difference in road performance of lignin-modified asphalt. In addition, lignin derivatives can also be used as asphalt modifiers, such as epoxy resin (Li et al., 2020a; Xiang and Xiao, 2020), phenolic resin, etc. Epoxy asphalt prepared with lignin has excellent performance and good rutting resistance, but the low-temperature crack resistance is defective, so it can be used only in high-temperature regions.
When the lignin content exceeds a certain proportion, lignin is used as an asphalt filler to reduce the proportion of pavement asphalt and achieve the benefits of environmental protection and cost-saving. Based on current research, too much lignin may lead to excessive hardness of asphalt pavement, reduced permeability and ductility, and poor pavement drainage and rutting resistance. However, some research results show that lignin as an asphalt filler can make the asphalt pavement reach the required strength and enhance the oxidation resistance of asphalt pavement. It may be a sustainable asphalt filler in the future. Further research is needed to determine the benefits brought by different dosages and maximize the benefits of cost and pavement strength.
Lignin can act as an antioxidant because lignin is a polyphenylene propane macromolecule with an aromatic monomer, it can interrupt the chain in the oxidation process to slow down the oxidation of asphalt. Grape residue produced in the wine industry was used as an asphalt antioxidant (Calabi-Floody and Thenoux, 2012), and its principle was similar to that of lignin as an antioxidant. In addition, lignin was used as an asphalt emulsifier (da Silva et al., 2020) and asphalt coupling agent. In the construction field, lignin was used as a stabilizer to strengthen the soil for civil engineering construction (Liu et al., 2020b).
Another major use of lignin is to directly add it to the asphalt mixture as an additive. It improved its pavement performance after adding different kinds of additives, such as lignin fibers, polyester fibers, bamboo fibers, rubber powders, and nano clay (Xu et al., 2010; Su et al., 2018; Xing et al., 2020; Liu et al., 2021). Generally, lignin improved the drainage performance, high-temperature stability, and low-temperature crack resistance of asphalt mixtures. Sometimes, more than two admixtures need to be added to improve the different properties of the asphalt mixtures (Fu et al., 2016; Fu et al., 2017). A further application of lignin is as a soil stabilizer, which can increase soil properties such as strength, shear resistance, and moisture sustainability. Most importantly, lignin has superior environmentally friendly properties compared to traditional soil stabilizers, such as lime, gypsum, and fly ash. Therefore, lignin is also a good material to be used in soil bases.
Overall, lignin has significant benefits in pavement engineering. The life cycle assessment on the use of lignin/bio-oil in asphalt was carried out (Zhang et al., 2020c; Tokede et al., 2020). Using lignin to replace part of crude oil asphalt in asphalt production can reduce carbon dioxide emissions. The performance evaluation of bio-oil modified asphalt is good, showing that it can improve the low-temperature crack resistance and fatigue resistance of asphalt. From the perspective of lignin materials themselves, a detailed life cycle assessment of lignin and its derivatives was performed (Moretti et al., 2021). In the future, environmentally friendly materials, such as lignin, may gradually replace fossil fuels and play a more important role in a short time, but lignin and its derivatives industry need to be further standardized. The same is true in the field of pavement engineering. The design method, road performance, and applicable environment of lignin modified asphalt should be gradually standardized to achieve the purpose of standardized utilization of lignin. Therefore, lignin has broad application prospects in pavement engineering. Table 1 summarized the effect of lignin application in different pavement construction materials. The mainstream applications of lignin are the asphalt modifier, asphalt mixture additive, asphalt extender, and soil stabilizer. The life cycle assessment of lignin-modified asphalt needs to be further deepened to investigate the balance between cost/pavement performance and the benefit of lignin-modified asphalt.
4 Conclusion
Lignin is a kind of aromatic polymer, which is a renewable organic resource. About 50–70 million tons of lignin are produced from papermaking and pulping industries every year. Common industrial lignin includes kraft lignin, sulfite lignin, soda lignin, organic solvent lignin, hydrolyzed lignin, and steam explosion lignin. Due to its complex chemical properties and high output, lignin is widely used in various fields. Different industrial products can be produced through processing technology, such as concrete admixtures, feed adhesives, soil stabilizers, dispersants and dye rheology, air absorbents, expansion agents, or asphalt emulsifiers in mortar, as well as macromolecular organics such as epoxy resin, polyurethane, and phenolic resin.
Lignin is mainly composed of carbon, hydrogen, and oxygen elements, which is similar to asphalt components. Therefore, in pavement engineering, lignin is mainly combined with asphalt and can be used as an asphalt modifier, asphalt extender, asphalt mixture additive, asphalt emulsifier, asphalt antioxidant, asphalt coupling agent, or soil stabilizer.
Through the literature review, the influence of lignin on asphalt or asphalt mixture is analyzed, and the main conclusions are shown as follows:
1) Lignin as an asphalt modifier can improve the viscosity, hardness, and high-temperature stability (rutting resistance) of modified asphalt, but it may reduce the low-temperature crack resistance and fatigue resistance of asphalt binders.
2) Lignin can improve the drainage performance, high-temperature stability and low temperature crack resistance of asphalt mixtures.
3) As an asphalt filler, lignin can reduce the proportion of asphalt and realize the benefits of environmental protection and cost-saving. However, much lignin may reduce the hardness, permeability, and ductility of the asphalt pavement and affect the overall pavement performance.
4) Lignin can also be used as a soil stabilizer for road base stabilization, which enhances the strength, moisture sustainability and may have better environmentally friendly properties compared to traditional soil stabilizers (lime, gypsum, fly ash).
In general, lignin has a wide application prospect in pavement engineering, which is manifested in the following advantages. Lignin can improve the viscosity, hardness, and high-temperature stability of modified asphalt. Lignin has different degrees of improvement on the drainage performance, high-temperature stability, and low-temperature crack resistance of asphalt mixtures. From the perspective of LCA, the use of lignin in asphalt can reduce the emissions and climate impact of road construction. However, there are some disadvantages of lignin that lead to its widespread application in road projects that need further research and investigation. For example, lignin can reduce the low-temperature cracking resistance and fatigue resistance of asphalt binders. The addition of much lignin to asphalt mixtures may result in reduced hardness, permeability, and ductility of asphalt pavements. From the LCA perspective, lignin-modified asphalt may negatively affect the environment. In terms of life cycle cost analysis, lignin is more costly than asphalt, but as the price of asphalt increases, it creates the possibility for this material to be widely used.
In conclusion, lignin can not only reduce the proportion of asphalt in asphalt pavement but also enhance the performance of asphalt pavement. It is an environmentally friendly and sustainable material. It has broad application prospects for the future. In future research, it is necessary to further explore the role and pavement performance of lignin in asphalt, establish industry standards, and make more effective use of this environmentally friendly material.
Author Contributions
HY and YW wrote the manuscript. JL, MX, and PM help draft the manuscript. JJ and ZY help revise the manuscript.
Conflict of Interest
This study received funding from Hunan Expressway Group Co. Ltd., Beijing Municipal Commission of Transport of China, Beijing University of Technology and National Natural Science Foundation of China. The funder was not involved in the study design, collection, analysis, interpretation of data, the writing of this article or the decision to submit it for publication. All authors declare no other competing interests.
The remaining authors declare that the research was conducted in the absence of any commercial or financial relationships that could be construed as a potential conflict of interest.
Publisher’s Note
All claims expressed in this article are solely those of the authors and do not necessarily represent those of their affiliated organizations, or those of the publisher, the editors and the reviewers. Any product that may be evaluated in this article, or claim that may be made by its manufacturer, is not guaranteed or endorsed by the publisher.
Funding
The authors appreciate the financial support of the Beijing Key Laboratory of Traffic Engineering (Beijing University of Technology, China) under grant number: 2018BJUT-JTJD007 and Beijing Municipal Commission of Transport of China under grant number: 2018-kjc-01-213. The authors also appreciate the funding support from Hunan Expressway Group Co. Ltd. and National Natural Science Foundation of China (No. 51778038). Any opinion, finding, and conclusion expressed in this paper are those of the authors and do not necessarily represent the view of any organization.
References
Aljubory, A., Teama, Z. T., Salman, H. T., and Abd Alkareem, H. M. (2021). Effects of Cellulose Fibers on the Properties of Asphalt Mixtures. Mater. Today Proc. 42, 2941–2947. doi:10.1016/j.matpr.2020.12.772
Arafat, S., Kumar, N., Wasiuddin, N. M., Owhe, E. O., and Lynam, J. G. (2019). Sustainable Lignin to Enhance Asphalt Binder Oxidative Aging Properties and Mix Properties. J. Clean. Prod. 217, 456–468. doi:10.1016/j.jclepro.2019.01.238
AzariJafari, H., Yahia, A., and Ben Amor, M. (2016). Life Cycle Assessment of Pavements: Reviewing Research Challenges and Opportunities. J. Clean. Prod. 112, 2187–2197. doi:10.1016/j.jclepro.2015.09.080
Bajwa, D. S., Pourhashem, G., Ullah, A. H., and Bajwa, S. G. (2019). A Concise Review of Current Lignin Production, Applications, Products and Their Environmental Impact. Ind. Crops Prod. 139, 111526. doi:10.1016/j.indcrop.2019.111526
Balaguera, A., Carvajal, G. I., Albertí, J., and Fullana-i-Palmer, P. (2018). Life Cycle Assessment of Road Construction Alternative Materials: A Literature Review. Resour. Conservation Recycling 132, 37–48. doi:10.1016/j.resconrec.2018.01.003
Batista, K. B., Padilha, R. P. L., Castro, T. O., Silva, C. F. S. C., Araújo, M. F. A. S., Leite, L. F. M., et al. (2018). High-Temperature, Low-Temperature and Weathering Aging Performance of Lignin Modified Asphalt Binders. Ind. Crops Prod. 111, 107–116. doi:10.1016/j.indcrop.2017.10.010
Bourzac, K. (2015). Inner Workings: Paving with Plants. Proc. Natl. Acad. Sci. USA 112 (38), 11743–11744. doi:10.1073/pnas.1509010112
Cai, G., Zhang, T., Liu, S., Li, J., and Jie, D. (2014). Stabilization Mechanism and Effect Evaluation of Stabilized Silt with Lignin Based on Laboratory Data. Mar. Georesources Geotechnology 34 (4), 331–340. doi:10.1080/1064119x.2014.966217
Calabi-Floody, A., and Thenoux, G. (2012). Controlling Asphalt Aging by Inclusion of Byproducts from Red Wine Industry. Construction Building Mater. 28 (1), 616–623. doi:10.1016/j.conbuildmat.2011.08.092
Calvo-Flores, F. G. (2020). Lignin: A Renewable Raw Material, Encyclopedia of Renewable and Sustainable Materials. Netherlands: Elsevier, 102–118.
Cao, L., Yu, I. K. M., Liu, Y., Ruan, X., Tsang, D. C. W., Hunt, A. J., et al. (2018). Lignin Valorization for the Production of Renewable Chemicals: State-Of-The-Art Review and Future Prospects. Bioresour. Technol. 269, 465–475. doi:10.1016/j.biortech.2018.08.065
Ceylan, H., Gopalakrishnan, K., and Kim, S. (2010). Soil Stabilization with Bioenergy Coproduct. Transportation Res. Rec. 2186 (1), 130–137. doi:10.3141/2186-14
Chen, Q., and Indraratna, B. (2015). Deformation Behavior of Lignosulfonate-Treated Sandy Silt under Cyclic Loading. J. Geotechnical Geoenvironmental Eng. 141 (1), 06014015. doi:10.1061/(asce)gt.1943-5606.0001210
Chen, H., and Xu, Q. (2010). Experimental Study of Fibers in Stabilizing and Reinforcing Asphalt Binder. Fuel 89 (7), 1616–1622. doi:10.1016/j.fuel.2009.08.020
Chen, H., Xu, Q., Chen, S., and Zhang, Z. (2009). Evaluation and Design of Fiber-Reinforced Asphalt Mixtures. Mater. Des. 30 (7), 2595–2603. doi:10.1016/j.matdes.2008.09.030
da Silva, M., Sad, C. M. S., Pereira, L. B., Corona, R. R. B., dos Santos, F. D., Kuster, R. M., et al. (2020). Study of the Effect of Eucalyptus Globulus Lignin and Schinus Terebinthifolius Tannin Extract on Water in Oil Emulsions of Heavy Oil. Fuel 264, 116816. doi:10.1016/j.fuel.2019.116816
Dessbesell, L., Yuan, Z., Leitch, M., Paleologou, M., Pulkki, R., and Xu, C. C. (2018). Capacity Design of a Kraft Lignin Biorefinery for Production of Biophenol via a Proprietary Low-Temperature/Low-Pressure Lignin Depolymerization Process. ACS Sustain. Chem. Eng. 6 (7), 9293–9303. doi:10.1021/acssuschemeng.8b01582
Figueiredo, P., Lintinen, K., Hirvonen, J. T., Kostiainen, M. A., and Santos, H. A. (2018). Properties and Chemical Modifications of Lignin: Towards Lignin-Based Nanomaterials for Biomedical Applications. Prog. Mater. Sci. 93, 233–269. doi:10.1016/j.pmatsci.2017.12.001
Fu, Z., Dang, Y., Guo, B., and Huang, Y. (2016). Laboratory Investigation on the Properties of Asphalt Mixtures Modified with Double-Adding Admixtures and Sensitivity Analysis. J. Traffic Transportation Eng. (English Edition) 3 (5), 412–426. doi:10.1016/j.jtte.2016.09.002
Fu, Z., Shen, W., Huang, Y., Hang, G., and Li, X. (2017). Laboratory Evaluation of Pavement Performance Using Modified Asphalt Mixture with a New Composite Reinforcing Material. Int. J. Pavement Res. Technol. 10 (6), 507–516. doi:10.1016/j.ijprt.2017.04.001
Gao, J., Wang, H., Liu, C., Ge, D., You, Z., and Yu, M. (2020). High-Temperature Rheological Behavior and Fatigue Performance of Lignin Modified Asphalt Binder. Construction Building Mater. 230, 117063. doi:10.1016/j.conbuildmat.2019.117063
Hemmilä, V., Hosseinpourpia, R., Adamopoulos, S., and Eceiza, A. (2019). Characterization of Wood-Based Industrial Biorefinery Lignosulfonates and Supercritical Water Hydrolysis Lignin. Waste Biomass Valor. 11 (11), 5835–5845. doi:10.1007/s12649-019-00878-5
Ijaz, N., Dai, F., Meng, L., Rehman, Z. U., and Zhang, H. (2020). Integrating Lignosulphonate and Hydrated Lime for the Amelioration of Expansive Soil: A Sustainable Waste Solution. J. Clean. Prod. 254, 119985. doi:10.1016/j.jclepro.2020.119985
Khater, A., Luo, D., Abdelsalam, M., Ma, J., and Ghazy, M. (2021). Comparative Life Cycle Assessment of Asphalt Mixtures Using Composite Admixtures of Lignin and Glass Fibers. Materials (Basel) 14 (21), 6589. doi:10.3390/ma14216589
Kim, S., Gopalakrishnan, K., and Ceylan, H. (2012). Moisture Susceptibility of Subgrade Soils Stabilized by Lignin-Based Renewable Energy Coproduct. J. Transp. Eng. 138 (11), 1283–1290. doi:10.1061/(asce)te.1943-5436.0000097
Koohpeyma, H. R., Vakili, A. H., Moayedi, H., Panjsetooni, A., and Nazir, R. (2013). Investigating the Effect of Lignosulfonate on Erosion Rate of the Embankments Constructed with Clayey Sand. ScientificWorldJournal 2013, 587462. doi:10.1155/2013/587462
Li, R., Bahadori, A., Xin, J., Zhang, K., Muhunthan, B., and Zhang, J. (2020). Characteristics of Bioepoxy Based on Waste Cooking Oil and Lignin and its Effects on Asphalt Binder. Construction Building Mater. 251, 118926. doi:10.1016/j.conbuildmat.2020.118926
Li, Z., Zhang, X., Fa, C., Zhang, Y., Xiong, J., and Chen, H. (2020). Investigation on Characteristics and Properties of Bagasse Fibers: Performances of Asphalt Mixtures with Bagasse Fibers. Construction Building Mater. 248, 118648. doi:10.1016/j.conbuildmat.2020.118648
Li, C., Ping, Q., Shi, H., Li, N., Zhang, J., and Wang, C. (2020). A Rapid and Quantitative Method for Assessing the Whiteness of Whitened Lignin Based on an In-Depth Analysis of Reported Methods. Int. J. Biol. Macromolecules 156, 1483–1490. doi:10.1016/j.ijbiomac.2019.11.195
Liu, W., Wang, J., Lin, G., Wen, L., and Wang, Q. (2019). Microscopic Mechanism Affecting Shear Strength in Lignin-Treated Loess Samples. Adv. Mater. Sci. Eng. 2019, 1–12. doi:10.1155/2019/7126040
Liu, Y., Chang, M., Wang, Q., Wang, Y., Liu, J., Cao, C., et al. (2020). Use of Sulfur-Free Lignin as a Novel Soil Additive: A Multi-Scale Experimental Investigation. Eng. Geology. 269, 105551. doi:10.1016/j.enggeo.2020.105551
Liu, Y., Zheng, W., Wang, Q., Cao, C., Chang, M., and Rocchi, I. (2020). Evaluating Sulfur-Free Lignin as a Sustainable Additive for Soil Improvement against Frost Resistance. J. Clean. Prod. 251, 119504. doi:10.1016/j.jclepro.2019.119504
Liu, K., Li, T., Wu, C., Jiang, K., and Shi, X. (2021). Bamboo Fiber Has Engineering Properties and Performance Suitable as Reinforcement for Asphalt Mixture. Construction Building Mater. 290, 123240. doi:10.1016/j.conbuildmat.2021.123240
Luo, D., Khater, A., Yue, Y., Abdelsalam, M., Zhang, Z., Li, Y., et al. (2019). The Performance of Asphalt Mixtures Modified with Lignin Fiber and Glass Fiber: A Review. Construction Building Mater. 209, 377–387. doi:10.1016/j.conbuildmat.2019.03.126
Mahmood, N., Yuan, Z., Schmidt, J., and Xu, C. (2016). Depolymerization of Lignins and Their Applications for the Preparation of Polyols and Rigid Polyurethane Foams: A Review. Renew. Sustain. Energ. Rev. 60, 317–329. doi:10.1016/j.rser.2016.01.037
Meng, Y., Lu, J., Cheng, Y., Li, Q., and Wang, H. (2019). Lignin-Based Hydrogels: A Review of Preparation, Properties, and Application. Int. J. Biol. Macromolecules 135, 1006–1019. doi:10.1016/j.ijbiomac.2019.05.198
Moretti, C., Corona, B., Hoefnagels, R., Vural-Gürsel, I., Gosselink, R., and Junginger, M. (2021). Review of Life Cycle Assessments of Lignin and Derived Products: Lessons Learned. Sci. Total Environ. 770, 144656. doi:10.1016/j.scitotenv.2020.144656
Moretti, C., Corona, B., Hoefnagels, R., van Veen, M., Vural-Gürsel, I., Strating, T., et al. (2022). Kraft Lignin as a Bio-Based Ingredient for Dutch Asphalts: An Attributional LCA. Sci. Total Environ. 806 (Pt 1), 150316. doi:10.1016/j.scitotenv.2021.150316
Norgbey, E., Huang, J., Hirsch, V., Liu, W. J., Wang, M., Ripke, O., et al. (2020). Unravelling the Efficient Use of Waste Lignin as a Bitumen Modifier for Sustainable Roads. Construction Building Mater. 230, 116957. doi:10.1016/j.conbuildmat.2019.116957
Pan, T. (2012). A First-Principles Based Chemophysical Environment for Studying Lignins as an Asphalt Antioxidant. Construction Building Mater. 36, 654–664. doi:10.1016/j.conbuildmat.2012.06.012
Peng, C., Huang, S., You, Z., Xu, F., You, L., Ouyang, H., et al. (2020). Effect of a Lignin-Based Polyurethane on Adhesion Properties of Asphalt Binder during UV Aging Process. Construction Building Mater. 247, 118547. doi:10.1016/j.conbuildmat.2020.118547
Pérez, I. P., Rodríguez Pasandín, A. M., Pais, J. C., and Alves Pereira, P. A. (2019). Use of Lignin Biopolymer from Industrial Waste as Bitumen Extender for Asphalt Mixtures. J. Clean. Prod. 220, 87–98. doi:10.1016/j.jclepro.2019.02.082
Ren, S., Liu, X., Zhang, Y., Lin, P., Apostolidis, P., Erkens, S., et al. (2021). Multi-Scale Characterization of Lignin Modified Bitumen Using Experimental and Molecular Dynamics Simulation Methods. Construction Building Mater. 287, 123058. doi:10.1016/j.conbuildmat.2021.123058
Singh, A. K., and Sahoo, J. P. (2021). A Study of the Performance of Lignosulfonate-Treated High Plastic Clay under Static and Cyclic Loading. Bull. Eng. Geol. Environ. 80 (10), 8265–8278. doi:10.1007/s10064-021-02444-7
Su, N., Xiao, F., Wang, J., Cong, L., and Amirkhanian, S. (2018). Productions and Applications of Bio-Asphalts - A Review. Construction Building Mater. 183, 578–591. doi:10.1016/j.conbuildmat.2018.06.118
Tokede, O. O., Whittaker, A., Mankaa, R., and Traverso, M. (2020). Life Cycle Assessment of Asphalt Variants in Infrastructures: The Case of Lignin in Australian Road Pavements. Structures 25, 190–199. doi:10.1016/j.istruc.2020.02.026
Tribot, A., Amer, G., Abdou Alio, M., de Baynast, H., Delattre, C., Pons, A., et al. (2019). Wood-Lignin: Supply, Extraction Processes and Use as Bio-Based Material. Eur. Polym. J. 112, 228–240. doi:10.1016/j.eurpolymj.2019.01.007
Uzer, A. U. (2016). Evaluation of Freezing-Thawing Cycles for Foundation Soil Stabilization. Soil Mech. Found. Eng. 53 (3), 202–209. doi:10.1007/s11204-016-9386-4
Wang, H., Liu, L. P., and Sun, L. J. (2012). Characterization of OGFC Mixtures Containing Lignin Fibers. Appl. Mech. Mater. 174-177, 775–781. doi:10.4028/www.scientific.net/amm.174-177.775
Wang, D., Li, G., Zhang, C., Wang, Z., and Li, X. (2019). Nickel Nanoparticles Inlaid in Lignin-Derived Carbon as High Effective Catalyst for Lignin Depolymerization. Bioresour. Technol. 289, 121629. doi:10.1016/j.biortech.2019.121629
Wu, W., Dutta, T., Varman, A. M., Eudes, A., Manalansan, B., Loqué, D., et al. (2017). New Routes to Renewables, Progressive charlestown. Available at: http://www.progressive-charlestown.com/ (Accessed January 11, 2021).
Wu, J., Liu, Q., Wang, C., Wu, W., and Han, W. (2021). Investigation of Lignin as an Alternative Extender of Bitumen for Asphalt Pavements. J. Clean. Prod. 283, 124663. doi:10.1016/j.jclepro.2020.124663
Xiang, Q., and Xiao, F. (2020). Applications of Epoxy Materials in Pavement Engineering. Construction Building Mater. 235, 117529. doi:10.1016/j.conbuildmat.2019.117529
Xing, X., Chen, S., Li, Y., Pei, J., Zhang, J., Wen, Y., et al. (2020). Effect of Different Fibers on the Properties of Asphalt Mastics. Construction Building Mater. 262 (8), 120005. doi:10.1016/j.conbuildmat.2020.120005
Xu, Q., Chen, H., and Prozzi, J. A. (2010). Performance of Fiber Reinforced Asphalt concrete under Environmental Temperature and Water Effects. Construction Building Mater. 24 (10), 2003–2010. doi:10.1016/j.conbuildmat.2010.03.012
Xu, G., Wang, H., and Zhu, H. (2017). Rheological Properties and Anti-aging Performance of Asphalt Binder Modified with wood Lignin. Construction Building Mater. 151, 801–808. doi:10.1016/j.conbuildmat.2017.06.151
Xu, Q., Bai, Y., Zhao, X., Ren, M., Wang, S., and Kong, F. (2021). Synthesis and Characterization of an Amphiphilic Lignin-Based Cationic Surfactant. Ind. Crops Prod. 164, 113376. doi:10.1016/j.indcrop.2021.113376
Yang, B., Zhang, Y., Ceylan, H., Kim, S., and Gopalakrishnan, K. (2018). Assessment of Soils Stabilized with Lignin-Based Byproducts. Transportation Geotechnics 17, 122–132. doi:10.1016/j.trgeo.2018.10.005
Yu, J., Vaidya, M., Su, G., Adhikari, S., Korolev, E., and Shekhovtsova, S. (2021). Experimental Study of Soda Lignin Powder as an Asphalt Modifier for a Sustainable Pavement Material. Construction Building Mater. 298, 123884. doi:10.1016/j.conbuildmat.2021.123884
Yuliestyan, A., García-Morales, M., Moreno, E., Carrera, V., and Partal, P. (2017). Assessment of Modified Lignin Cationic Emulsifier for Bitumen Emulsions Used in Road Paving. Mater. Des. 131, 242–251. doi:10.1016/j.matdes.2017.06.024
Yuliestyan, A., Gabet, T., Marsac, P., García-Morales, M., and Partal, P. (2018). Sustainable Asphalt Mixes Manufactured with Reclaimed Asphalt and Modified-Lignin-Stabilized Bitumen Emulsions. Construction Building Mater. 173, 662–671. doi:10.1016/j.conbuildmat.2018.04.044
Zhang, T., Cai, G., Liu, S., and Puppala, A. J. (2016). Engineering Properties and Microstructural Characteristics of Foundation silt Stabilized by Lignin-Based Industrial By-Product. KSCE J. Civ Eng. 20 (7), 2725–2736. doi:10.1007/s12205-016-1325-4
Zhang, T., Cai, G., and Liu, S. (2018a). Reclaimed Lignin-Stabilized Silty Soil: Undrained Shear Strength, Atterberg Limits, and Microstructure Characteristics. J. Mater. Civil Eng. 30 (11), 1–9. doi:10.1061/(asce)mt.1943-5533.0002492
Zhang, T., Cai, G., and Liu, S. (2018b). Application of Lignin-Stabilized Silty Soil in Highway Subgrade: A Macroscale Laboratory Study. J. Mater. Civil Eng. 30 (4), 1–16. doi:10.1061/(asce)mt.1943-5533.0002203
Zhang, J., Huang, W., Zhang, Y., Lv, Q., and Yan, C. (2020). Evaluating Four Typical Fibers Used for OGFC Mixture Modification Regarding Drainage, Raveling, Rutting and Fatigue Resistance. Construction Building Mater. 253, 119131. doi:10.1016/j.conbuildmat.2020.119131
Zhang, Y., Wang, X., Ji, G., Fan, Z., Guo, Y., Gao, W., et al. (2020). Mechanical Performance Characterization of Lignin-Modified Asphalt Mixture. Appl. Sci. 10 (9), 3324. doi:10.3390/app10093324
Zhang, X., Zhang, K., Wu, C., Liu, K., and Jiang, K. (2020). Preparation of Bio-Oil and its Application in Asphalt Modification and Rejuvenation: A Review of the Properties, Practical Application and Life Cycle Assessment. Construction Building Mater. 262, 120528. doi:10.1016/j.conbuildmat.2020.120528
Keywords: pavement engineering, lignin, asphalt binder, asphalt mixture, modified asphalt, polymer
Citation: Yao H, Wang Y, Liu J, Xu M, Ma P, Ji J and You Z (2022) Review on Applications of Lignin in Pavement Engineering: A Recent Survey. Front. Mater. 8:803524. doi: 10.3389/fmats.2021.803524
Received: 28 October 2021; Accepted: 22 December 2021;
Published: 14 January 2022.
Edited by:
Antonio Caggiano, Darmstadt University of Technology, GermanyReviewed by:
Pouria Hajikarimi, Amirkabir University of Technology, IranBabak Golchin, University of Mohaghegh Ardabili, Iran
Ali Behnood, Purdue University, United States
Copyright © 2022 Yao, Wang, Liu, Xu, Ma, Ji and You. This is an open-access article distributed under the terms of the Creative Commons Attribution License (CC BY). The use, distribution or reproduction in other forums is permitted, provided the original author(s) and the copyright owner(s) are credited and that the original publication in this journal is cited, in accordance with accepted academic practice. No use, distribution or reproduction is permitted which does not comply with these terms.
*Correspondence: Hui Yao, aHVpeWFvQG10dS5lZHU=