- 1State Key Laboratory of Oral Diseases, National Clinical Research Center for Oral Diseases, West China Hospital of Stomatology, Sichuan University, Chengdu, China
- 2Division for Globalization Initiative, Liaison Center for Innovative Dentistry, Graduate School of Dentistry, Tohoku University, Sendai, Japan
- 3Department of Prosthodontics, Faculty of Dental Medicine, Airlangga University, Surabaya, Indonesia
Applying hyaluronic acid, a biopolymer material, in the treatment of interdental papilla reconstruction has become a trend. The main objective of this research is to investigate the histologic effect of hyaluronic acid on interdental papilla over time. Deficient interdental papilla models were surgically created in sixty-two Sprague-Dawley (SD) rats and were randomly treated with the injection of hyaluronic acid (HA group) or phosphate-buffered saline (sham control group) or left untreated (control group). After 2, 4, and 8 weeks, the rats were sacrificed in batches to observe the histological changes. A fluorochrome label was used to monitor bone formation in 8 weeks. Immunohistochemical analyses were performed to evaluate the expression of potentially relevant cytokines, vascular endothelial growth factor (VEGF), bone morphogenetic protein-2 (BMP-2), and Wnt-induced secreted protein 1 (WISP1) in the gingival tissue in 8 weeks. A preliminary study of HA degradation after 24 weeks was performed in two rats. Following the HA injection, no inflammation or granulomatous foreign body reaction was observed. HA was able to promote collagen fiber and alveolar bone regular formation in the reconstruction site. HA also enhanced VEGF, BMP-2, and WISP-1 expression in gingival tissue (p<0.05). After 24 weeks, there was no HA filler observed in the interdental papilla. In conclusion, our study suggested that HA is an effective way to reconstruct deficient interdental papilla.
Introduction
The interdental papilla is part of the gingiva that occupies the space of the two adjacent teeth. Interdental papilla plays a vital role in one’s appearance and oral health (Joshi et al.,2017; Hochman et al.,2019). A deficiency in the interdental papilla causes open embrasures, leading to many problems, such as aesthetic appearance defects and food impaction, phonic problems (Zhang et al.,2020). A study showed that 98% of lay people considered that the presence of the interdental papilla would make a smile more attractive compared to their absence (Kolte et al.,2014; Hochman et al.,2019). Interdental papilla deficiency also gives rise to food impaction. This problem can easily be found among periodontal patients. In addition, with the interdental papilla missing, open embrasures give way to air or saliva, which is the main cause of phonic problems (Carnio and Carnio, 2018). There are several reasons for the interdental papilla recession. Common reasons for this case are anatomic structure, aging, and bad oral health habits, to name a few (de Santana et al.,2017; Kolte et al.,2018). Among these causes, the most representative and direct reason is the distance between the interproximal contact and the alveolar bone crest. When the distance is more than 5 mm, there is more likely the appearance of deficient interdental papillae (Tarnow et al.,1992; Martegani et al.,2007).
The reconstruction of the deficient papilla is one of the most difficult and the least unpredictable problems to address because multifactorial etiology is involved. Many treatment options are devoted to reconstructing deficient papilla. They can be divided into nonsurgical and surgical approaches (Zhang et al.,2020). Surgical approaches aim to reconstruct or preserve the gingival tissue as well as the alveolar bone below it. This kind of method is applied to a single site of a large area of deficiency. However, such an approach may cause gingival recession during the healing period, and it may also cause an insufficient blood supply inhibiting gingival tissue reconstruction. Nonsurgical approaches have recently been more widely used in the clinic, including restorative techniques, orthodontic movement, and biopolymer material injections such as hyaluronic acid to reshape and to diminish open embrasures (Ziahosseini et al.,2014).
Hyaluronic acid (HA) is a linear biopolymer, which is naturally presented in human connective tissues and synovial fluid (Pereira et al.,2018). As HA is already widely used as an effective filler to improve the facial volume (Landau and Fagien, 2015), it has also been successfully applied to dental papilla reconstruction [Sadat Mansouri et al.,2013; Awartani and Tatakis, 2016; Lee et al.,2016a; Lee et al.,2016b]. In 2010, A pilot study (Becker et al.,2010) explored employing HA in the interdental papilla reconstruction, and ever since then, several clinical reports have been proposed successfully. By interrupting injections, interdental papilla obtained different levels of recovery that lasted for 6–25 months (Sadat Mansouri et al.,2013; Tanwar and Hungund, 2016; Lee et al.,2016a; Ni et al.,2019).
Although HA has been proved as a nontoxic, biodegradable, and biocompatible biopolymer in cosmic beauty products, its side effects should not be ignored. Most of the adverse effects appeared within 1 week postinjection (Pi et al.,2017; Kim et al.,2020). Some of them will last over 3 weeks (Philipp-Dormston et al.,2017). It is worth noting that the two adverse reaction cases reported until now appeared 4 weeks after the initial injection. The patients experienced swelling, burning pain, extreme tenderness, and skin discoloration next to the injection site. It was speculated that the HA filler absorbed the water as time went on and squeezed the surrounding blood vessel (Gabitov, 2016). However, the gingival tissue reactions to the HA filler lasting for a week or more remained unclear.
Until now, various brands of HA products were used in the procedure of interdental papilla reconstruction. Among them, the most frequently used one is Restylane, a biphasic HA filler with a concentration of HA of 20 mg/ml (Wu et al., 2016). The present study used a domestic commercially available product with a similar concentration of HA to conduct the injection procedure. This HA filler uses a highly effective cross-linking (HEC) technique and has an advantage in price. Last but not least, HA is widely used in wound healing and tissue regeneration based on its multiple biological activities, such as bacteriostatic, osteoinductive, and angiogenesis activities (Pirnazar et al., 1999; Kim et al., 2016; Abatangelo et al., 2020; Graca et al., 2020; Zhai et al., 2020; Zhao et al., 2016). To further explore the mechanism that may be involved in interdental papilla regeneration or repair, a few representative gene markers were targeted in this study. Therefore, the first aim of this study was to verify the histological changes of interdental papilla tissue caused by HA injection for a certain time. The second aim was to explore the potential of HA to promote interdental papilla reconstruction. Meanwhile, a preliminary study with a small sample size was performed to explore the degradation of the HA filler. We hypothesized that HA might have a mechanical and reconstructive effect on the surrounding tissue after injection for a certain time.
Materials and methods
Material
The material used in this study is a commercial HA filler (Biohyalux, Bloomage Hyinc Technology Co., Ltd., Beijing, China) which contains 18 mg/ml modified HA and 2 mg/ml unmodified HA of nonanimal origin. The molecular weight of HA is 1,000–2,000 kDa, and the cross-link degree is less than 1%. The filler appears as a viscous suspension of transparent or translucent particles. The gel particle sizes were smaller than 1 mm, concentrated at 0.2–0.6 mm. The pH value of the buffered physiologic saline solution in the filler is 6.8–7.5.
Animals and model establishment
A total of sixty-two female Sprague Dawley (SD) rats weighing 220 ± 10 g were obtained from the experimental animal center at Sichuan University (Chengdu, China). The animals were raised under an ambient temperature of 24 ± 2°C, humidity of 55 ± 10%, and light/dark cycle of 12/12 h, and with water and food ad libitum. The study was performed after the rats were allowed to acclimate for 1 week.
A rat model of deficient interdental papilla was established following the interproximal periodontal defect model (Jung et al., 2011). After anesthesia, gingival crevicular incisions surrounded the mandibular incisors to expose the interproximal alveolar bone crest. The gingival tissue and alveolar bone above the interdental papilla base were removed by a 0.6-cm fissure bur with copious saline irrigation (Figure 1). After the operation, 7–0 suture material was used to close the flap. All the studies, including the experimental animals, were approved by Research Ethics Committee of West China Hospital of Stomatology.
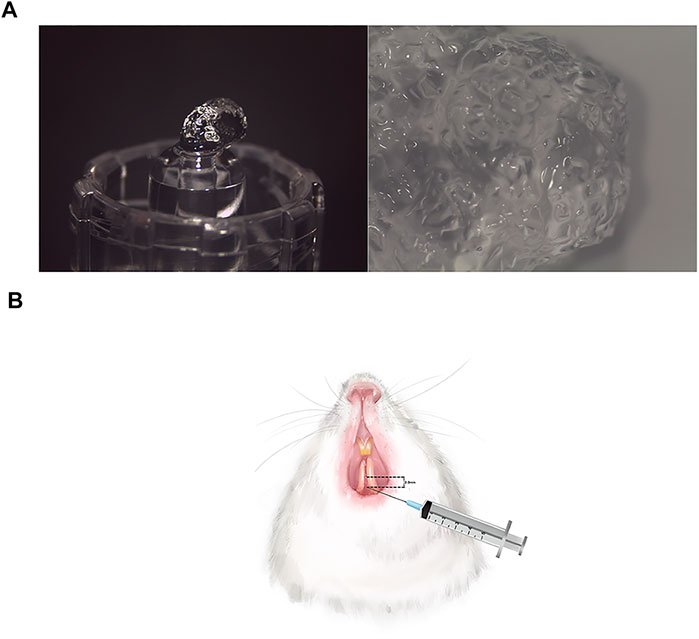
FIGURE 1. The appearance of HA filler and injection procedure. (A) The pictures of HA filler at ×8 magnification and ×12.5 magnification, respectively. The HA filler contains 18 mg/ml modified HA with pH values 6.8–7.5 and particle sizes 0.2–0.6 mm. (B) A total of 0.08 ml HA was injected at approximately 2–3 mm apically below the interdental papilla tip.
Micro-CT examination
All the animals received the micro-CT scanning of preoperation and at 1 week postoperation under anesthesia to observe the interdental papilla defects. The area of mandibular incisors was scanned (microCT, PerkinElmer, Quantum GX, Waltham, MA, USA) at 90.0 kV and 88 mA. To identify the interdental papilla tip in the image, calcium hydroxide paste was used as a radiopaque material and placed on the top of the tip. Three-dimensional images were obtained to assess the interdental papilla tip location and the alveolar crest under it.
Injection procedure
One week after the surgical procedure, sixty-two of the rats were randomly divided into three groups, namely, the control group (n = 20), the sham control group (n = 20), and the HA group (n = 22). Referring to the literature, in the HA group, a total of 0.08 ml of the HA filler (Biohyalux, Beijing, China) which contains 18 mg/ml modified HA was constantly injected by 4 times into the interdental papilla tip due to the concern that the HA might backflow due to the tissue pressure (Lee et al., 2016a) (Figure 1). The pH value of the HA was 6.8–7.5, and the gel particle sizes were concentrated at 0.2–0.6 mm. An injection-assisting device (CelTick®, Hyundae Meditech, Wonju, Korea) was employed to control the injection volume. A 30-G needle was inserted at approximately 2–3 mm apically below the interdental papilla tip (Figure 2). The same dose of phosphate-buffered saline (PBS) was injected into the same site as in the sham control group, whereas the other rats of the control group were not given any injection therapy.
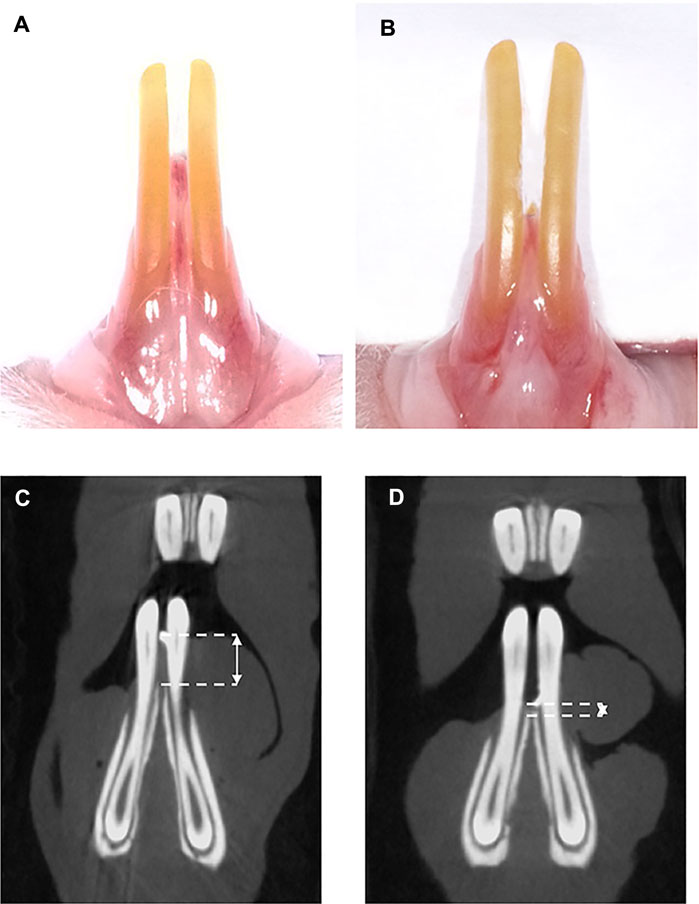
FIGURE 2. Clinical observations and micro-CT analysis of rat model. (A) Preoperation, (B) 1 week postoperation, (C) micro-CT scanning preoperation, and (D) micro-CT scanning 1 week postoperation. The double-headed arrow shows the distance between the interdental papilla tip and alveolar bone crest.
Histology
After 2, 4, and 8 weeks of the injection procedure, 15 rats of each group were sacrificed for the histological study (n = 5). Moreover, after 24 weeks, the last two rats in the HA group were sacrificed for the same study. The tissue surrounding the mandibular incisors of each group was fixed with 4% paraformaldehyde (Biosharp, Hefei City, China). Following the fixation, the samples were decalcified, dehydrated, and embedded in paraffin. Sections measuring 5-μm sections were obtained in the mesio-distal direction. Each specimen has prepared 50 sections for the following analysis. The second of every ten sections was stained with hematoxylin–eosin, and the third ones were stained with Masson’s Trichrome. The slides were scanned and analyzed by the Department of Pathology in West China Hospital. All the slides were scanned by a Digital Pathology Scanner PRECICE 500 (UNIC, Beijing, China), and the pictures were taken with NDP.view2 software (Hamamatsu Photonics, Hamamatsu, Japan).
Dynamic labeling
In order to observe alveolar bone formation, calcium-binding fluorescent dye was used to mark the mineralization (Abduo and Elseyoufi, 2018). One week after the surgery, fifteen rats were given an initial intraperitoneal injection of calcein green (Sigma-Aldrich, St. Louis, MO, USA) at 5 mg/kg of body weight. At the same time, different interventions were given to the three groups (n = 5). The second fluorochrome injection was given in 3 days before they were sacrificed. After dehydration, infiltration, and polymerization, the samples were embedded with poly-methyl methacrylate and made into 40-μm sections in the mesio-distal direction. Every specimen has prepared ten sections, and three of them were picked randomly for scanning. Fluorescence analysis was performed using a confocal microscope (A1RMP, Nikon, Japan).
Immunohistochemistry
To further investigate the growth factor expression level associated with interdental papilla reconstruction, immunohistochemical staining was performed according to the streptavidin–peroxidase (S–P) method. Three slides of every specimen were randomly picked from the 50 sections which were previously prepared in the histological research. The sections was deparaffinized and treated with trypsin for antigen retrieval. After being washed in PBS, the samples were blocked with 3% H2O2 in methanol for 30 min to quench the endogenous peroxidase activity, followed by three rinses with PBS. The nonspecific binding areas were blocked with the goat serum at 37°C for 30 min. The samples were incubated overnight at 4°C with the antibodies of vascular endothelial growth factor, VEGF (1:200; ImmunoWay, Plano, TX, USA, YM3681); bone morphogenetic protein, BMP-2 (1:200; ImmunoWay, Plano, TX, USA, YT5651); and Wnt-induced secreted protein 1, WISP1 (1:200; Abcam, Cambridge, UK, ab178547). Five pictures of each slide were captured to assess the target protein positive expression under fluorescent microscopy at ×400 magnification (Zeiss, Jena, Germany). Image-Plus Pro 6.0 (Media Cybernetics, Bethesda, MD, USA) was applied to detect the mean optical density (MOD) of the positive protein expression, which was determined according to the brown staining within the cell cytoplasm. Measurement data are described by mean ± standard and were analyzed with SPSS software (version 23.0, SPSS, Chicago, IL, USA). The data fitted, or basically fitted, the normal distribution. Repeated-measure ANOVAs were used to compare the data among the three groups. If the data were spherically symmetrically distributed, then the comparison among different time points employed one-way ANOVA; if the data were not spherically symmetrically distributed, the Green-House method was used for data rectification, and the comparison between groups was analyzed using the Bonferroni method. Statistical significance was set at p < 0.05. All measurements and analyses were performed in triplicate by three team colleagues, who were blinded to the treatment allocation.
Results
Clinical observations and micro-CT analysis of the rat model
One week after the surgical procedure, the wound healing among the three groups was uneventful. No inflammation or gingival hyperplasia was observed. Guided by the radiopaque material, which showed a hyperdense spot on the tip of the interdental papilla, the distance between the interdental papilla tip and the alveolar bone crest was measured (Figure 2). The distance before the operation was 2.24 ± 0.29 mm, and the distance postoperation was 0.77 ± 0.20 mm, which indicated that the rat model was successfully established.
Histologic observations
In the HA group, the HA filler could be seen in all the samples at 2, 4, and 8 weeks, and in the meantime, the samples appeared as pockets of extracellular pale grayish-blue material in the modified HE-stained slides, enclosed by a fibrous capsule (Figure 3). Due to the differences in hardness between the tooth and gingival tissue, some of the HA filler was squeezed out when preparing the tissue slices, leaving an empty fibrous capsule. There was no inflammatory response or granulomatous foreign body in the gingival tissue, nor was there any blood vessel squeeze or obstacle caused by the HA filler. No needle tract, inflammation, or infection was observed in the sham control group at three time points. The HA filler did not survive after the Masson Trichrome staining process (Figure 4). Collagen fibers were presented as a collection of dense bluish colors with the Masson Trichrome stain. The HA group showed more prominent dense and regular masses of blue positive collagen fibers between the epithelium and alveolar crest at 4 and 8 weeks postinjection. The preliminary study showed that no HA filler remained, and the mature collagen formed in the interdental papilla after 24 weeks.
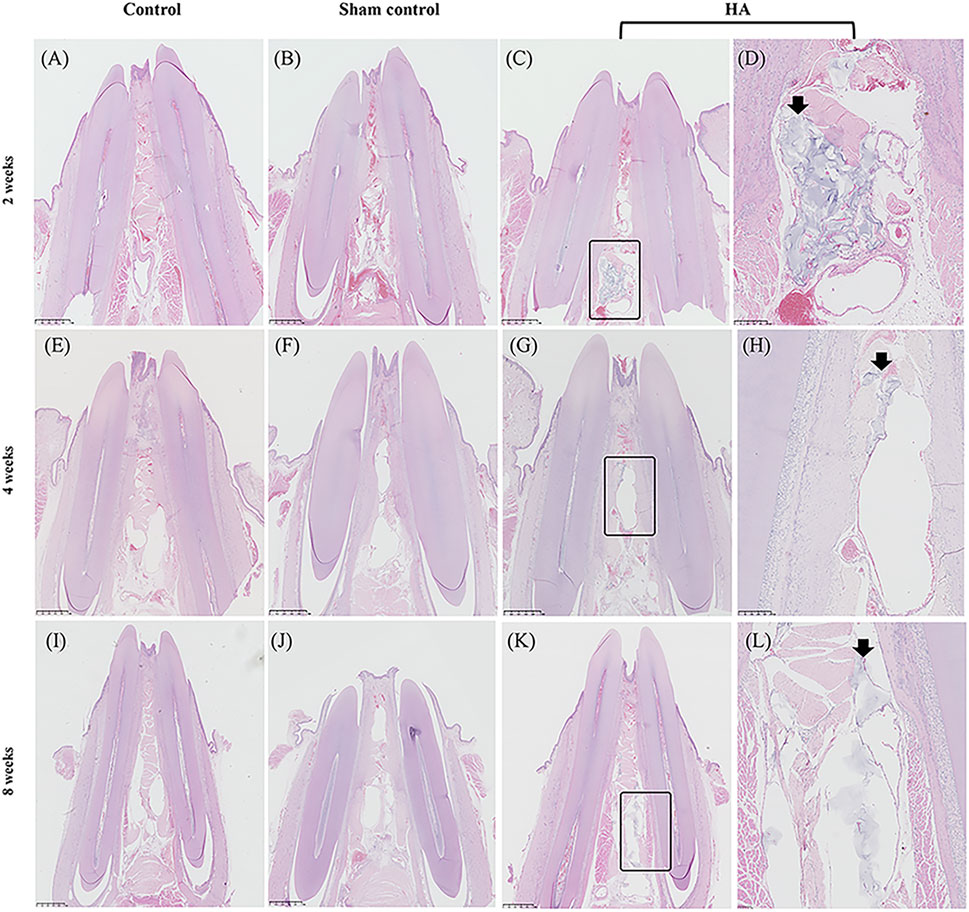
FIGURE 3. Photo-scanning of HE staining showing the histologic changes in the interdental papilla. Control group at 2 (A), 4 (E), and 8 weeks (I). Sham control group at 2 (B), 4 (F), and 8 weeks (J). HA group at 2 (C), 4 (G), and 8 weeks (K), n = 5. Figure (D), (H), (L) are the magnification of the black rectangle areas in Figure (C), (G), (K). The black arrows show the HA filler in the interdental papilla surviving from HE staining. Scale bars = 1 mm.
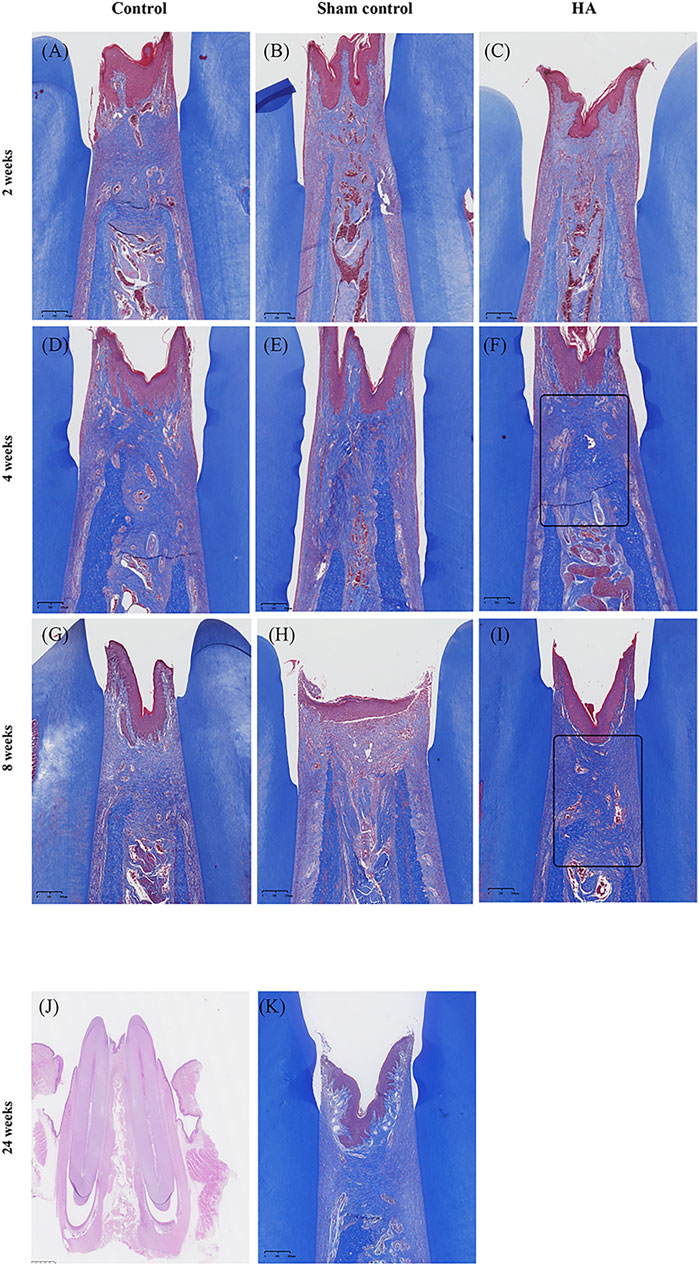
FIGURE 4. Photo-scanning of Masson Trichrome staining showing the collagen fiber formation in the interdental papilla. Control group at 2 (A), 4 (D), and 8 weeks (G). Sham control group at 2 (B), 4 (E), and 8 weeks (H). HA group at 2 (C), 4 (F), and 8- weeks (I), n = 5. Collagen fibers are presented as a collection of dense bluish colors with the Masson Trichrome stain. They are more prominent at 4 and 8 weeks postinjection in the HA group (black triangle). No HA filler remained at 24 weeks after injection (J), scale bar = 1 mm and mature collagen fibers formed in the interdental papilla (K), n = 2. Scale bars = 200 μm.
Dynamic labeling analysis
The bone formation could be seen on the alveolar bone crest by the presence of the fluorochrome markers. The pristine bone labeled by calcein showed a green signal (Figure 5). There were two lines on the top of the alveolar crest; the inner one showed the new bone formation after the first fluorochrome injection, and the outer one suggested the second injection before the animal was sacrificed. The area between the two lines came to show the remodeling of the alveolar bone in 8 weeks. According to the result, the HA group did not generate more bone tissue than the other groups, but the fluorescence signals were more regularly formed in the interdental papilla.
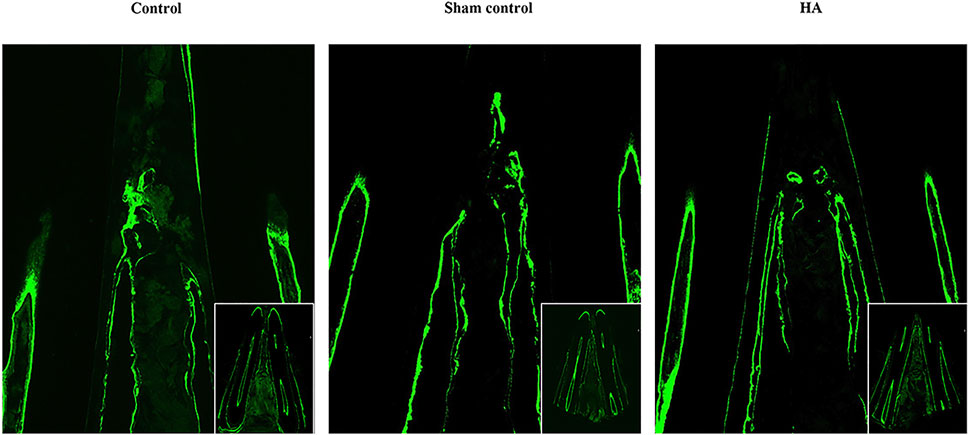
FIGURE 5. Dynamic labeling of the pristine alveolar bone. Dynamic labeling alveolar bone regeneration in representative tissue sections 8 weeks postinjection of the control group (A), sham control group (B), and HA group (C), n = 5.
Immunohistochemical analysis
The results showed no significant difference at 2 weeks postinjection among the three groups (Figure 6 and Tables 1–3). The expressions of BMP-2 and WISP1 were significantly increased at 4 weeks compared to the control and sham control groups (p < 0.05). At the same time point, the expression of VEGF was also increased significantly compared to the control group (p < 0.05). However, it had no significant change compared to the sham control group (p > 0.05). The expression of VEGF and WISP1 returned to the baseline and showed no significant difference at 8 weeks among the three groups (p > 0.05). The expression of BMP-2 was significantly increased at 8 weeks in the HA group compared to the control group and the sham control group (p < 0.05). The expression of VEGF and WISP1 groups in the sham control had no significant change among three time points compared to the control group (p > 0.05). However, the expression of BMP-2 in the sham control group was significantly increased compared to the control group at 4 and 8 weeks (p < 0.05).
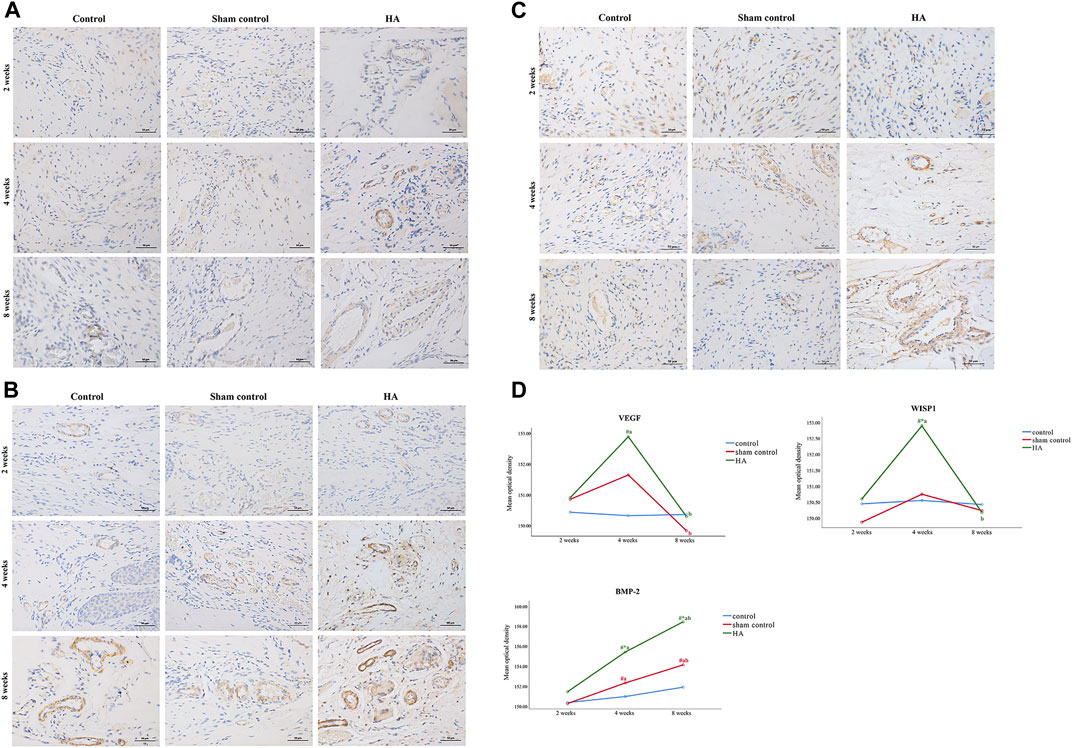
FIGURE 6. The expression of VEGF, BMP-2, and WISP1 at different time points in the interdental papilla. Representative photomicrograph of VEGF (A), BMP-2 (B), and WISP1 (C) immunohistochemistry staining in the interdental papillae. Scale bars = 50 μm. (D) Quantitative analysis of gene expression at different time points. Data represent means ± SD with group sizes of n = 5. (#P < 0.05, HA, sham control group compared to the control group; *P < 0.05, HA group, control group compared to the sham control group; a P < 0.05, compared to 2 weeks; bP < 0.05, compared to 4 weeks).
Discussion
Interdental papillae deficiency is always a complex problem to be solved. The deficiency is not always huge, but it can be difficult to repair due to the anatomy and the limited blood supply. As HA could reconstruct deficient interdental papilla efficiently and with minimal invasiveness, more and more clinical reports have increasingly sprung up to verify these features. The current study focused on the histological changes and the reconstructive potential caused by HA injection over time.
A commercial biphasic HA filler consists of modified HA, unmodified HA, and buffer solution. The aesthetic outcome of an injection procedure, together with the targeted anatomic location and tissue mobility, mostly depends on the concentration and particle size of the HA. In commercial HA filler, the concentration of HA is clustered in 20 mg/ml (Lee et al.,2020). Consistent with the literature, the HA filler employed in the present study contains 20 mg/ml HA (18 mg/ml modified HA and 2 mg/ml unmodified HA). Due to the absence of submucosa, the interdental papilla lacks mobility for accommodating the big particle size of the HA filler. What is more, although a bigger particle size of the HA filler would prolong the filling effect, the pushback force and pain will also increase according to the viscoelasticity of the filler (da Costa et al.,2017). Thus, the HA filler in the present study has a relatively small size which is commercially used in lip augmentation.
Most of the side effects caused by the HA filler appeared within 1 week postinjection, including redness, swelling, hypersensitivity, and vascular obliteration (Philipp-Dormston et al.,2017). Some of them will last over 3 weeks. In some cases, granuloma foreign bodies will last more than 2 months. No granulomatous inflammatory reactions, avascular necrosis, or squashed blood vessels were observed in the gingival tissue in the present study. Although the literature showed that the filling effect in some cases could last 6–25 months (Becker et al.,2010), our study demonstrated that the HA could remain in the gingival tissue in all individuals until 8 weeks and degraded after 24 weeks. The possible explanation could be that HA degradation speed has individual differences or the interdental papilla tissue may regenerate by applying this therapy.
Regarding anatomy, the interdental papilla is a part of the gingival tissue that contains many collagen fibers and maintains its height by supporting the alveolar bone (Martegani et al.,2007; Smith et al.,2019). HA was initially applied in soft tissue augmentation and plastic surgery due to its water retention features, biodegradability, and unique chemical–physical properties (Fallacara et al.,2017). By absorbing the water from the surrounding tissue, HA may induce the mechanical stretching of the fibroblasts, which may cause collagen synthesis (Landau and Fagien, 2015; Cheng et al.,2018). In addition, it has been proved that HA could activate collagen and elastic fiber formation through the TGF-β/Smad pathway (Bertossi et al.,2013; Bukhari et al.,2018; Fan et al.,2019). In the study of Pi et al.(2017), HA generated fibrous tissue in the interdental papilla within 7 days. To observe the morphology of collagen formation, we performed the Masson Trichrome staining. Consistent with the literature, the results found that HA stimulated denser and well-organized collagen starting at 4 weeks postinjection. A sham control was set up in the present study to eliminate the possibility of injury caused by the injection itself. At 2, 4, and 8 weeks, the sham control group had no inflammation, infection, or more collagen synthesis compared to the control group or HA group. Therefore, the injection procedure did not have a side effect on the gingival tissue.
When the distance between the contact point and the alveolar bone crest is more than 5 mm, there is more likely the appearance of deficient interdental papillae (Tarnow et al.,1992; Martegani et al.,2007). Interdental papilla can obtain a better shape if the underlying bone gives sound support. In the previous study, HA has been used to accelerate the healing of extraction sockets by promoting bone formation and wound healing (Kim et al.,2016). HA played the role of an extracellular matrix (ECM) in directing cell fate during bone regeneration (Fujioka-Kobayashi et al.,2017; Maturavongsadit et al.,2017). The presence of HA accelerates bone deposition and remodeling activities (Baldini et al.,2010). HA has also been well known to serve as a scaffold to enhance the osteogenic potential of other bioactive materials (Abatangelo et al.,2020; Zhai et al.,2020). In this study, we used calcein-labeled bone to observe the effect of HA on bone morphology under the interdental papilla. The results showed that after 8 weeks, the pristine bone was formed in a better shape in the HA group as compared to the other groups. This indicates that HA might have the potential to facilitate bone remodeling.
HA can promote cell proliferation and migratory and thus facilitate healing procedure or tissue regeneration. (Asparuhova et al., 2019; Xing et al., 2020). Vascularization is vital to tissue regeneration. HA increased a pro-angiogenic effect by upregulating the expression of VEGF and fibroblast growth factor-2 (FGF-2) (Ciccone et al., 2019). A previous study showed that HA injection stimulated micro-vascularization formed surrounding the fillers in the interdental papilla (Pi et al., 2017). Consistent with the literature, the present study showed that the expression of VEGF was upregulated at 4 weeks after the HA injection, which may help the new tissue vascularize in the interdental papilla. Similarly, HA promotes bone regeneration by influencing cell proliferation and osteogenic differentiation. In a review of the literature, it was found that HA could be positive for the expression of BMP-2 or other osteoprogenitor and osteogenic markers as it provides an ideal environment by virtue of its physicochemical properties (Nickel and Mueller, 2017; Matheus et al., 2020; Cabreira et al., 2021). In this study, the expression of BMP-2 was upregulated at 4 and 8 weeks. This may connect with the remodeling of the alveolar crest underlying the interdental papilla. Meanwhile, the expression of BMP-2 was also increased significantly in the sham control group compared to the control group at the same time points. This may be due to the injection itself which can also lead to tissue repair (Kim et al., 2017; Cho et al., 2019; Chun et al., 2019). WISP1 was also chosen to focus on this study, as it has multiple cellular functions that include skeletal system development, vascular repair, cellular survival, wound healing, and tooth development by being a transducer of the canonical Wnt/β-catenin pathway (Sarkar and Sharpe, 1999; Chen et al., 2009; Liu and Millar, 2010; Kim et al., 2013; Maiese, 2014; Maeda et al., 2015; Jin et al., 2017). A study showed that WISP1 is a downstream target of HA binding with its receptor, CD44, and could be stimulated by stretch-induced activation (Heise et al., 2011). In the present study, WISP1 was highly expressed 4 weeks after the HA injection. This result indicated that a possible cellular pathway may be activated by HA injection in the progress of the interdental papilla reconstruction.
Apart from the biological effect that HA may have on the gingival tissue mentioned above, we speculated that the HA filler makes the mechanical force to the surrounding tissue. When injected for soft tissue augmentation, HA draws water from the surrounding tissue and creates a swelling pressure to withstand compressive forces (Philipp-Dormston et al.,2017). One in vivo study showed that the HA filler increased in volume by 1.8 times of the original size at 1 week with a subsequent decrease in size over 16 weeks (Kim et al.,2015). The mechanical stretching applied by the swelling HA filler on the surrounding ECM initiated a healing and regenerative mechanism. We found that compared to collagen and bone formation persisting to the eighth week, the expression of VEGF and WISP1 returned to the baseline at last. This may be due to fiber synthesis and osteogenesis in gingival tissue being more sensitive to the mechanism of stretching. The cause–effect link between collagen formation and morphological stretching of fibroblasts following injection of exogenous cross-linked HA has been verified by previous studies (Wang et al.,2007; Cui et al.,2021). The gingival tissue and alveolar bone are constantly exposed to physical forces under physiological and pathological conditions. Mechanical stimuli promote gingival tissue and bone tissue remodeling by activating the cell viability or affecting ECM (Krishnan and Davidovitch, 2009; Nan et al.,2019). In the present study, the mechanism forces might reach a high level at 4 weeks post injection and then declined. This also fits with the onset of adverse effects reported previously (Gabitov, 2016).
To the best of our knowledge, this is the first study observing the effect of HA on interdental papillae over 1 week. However, several questions remain unanswered at present. A larger sample size of the long-term study is needed to detect the longevity of the HA filler in vivo. Based on the recognition of the filling effect depending on the modified HA concentration, viscoelasticity, and particle sizes, the comparison of the therapeutic effect among different compositions or brands of HA fillers should be verified in future work. What is more, we only detected a few cytokines’ expressions, which may be related to gingival papilla regeneration. To better understand the mechanism of HA on interdental papillae reconstruction, further research is encouraged to explore more possibilities.
Conclusion
Notwithstanding its limitations, this study verified that deficient interdental papillae can be reconstructed by the HA filler without inducing inflammation or a granulomatous foreign body reaction for at least 8 weeks. HA also has the potential of facilitating interdental papillae repair by promoting collagen and bone remodeling.
Data Availability Statement
The original contributions presented in the study are included in the article/Supplementary Material; further inquiries can be directed to the corresponding author.
Ethics Statement
The animal study was reviewed and approved by Research Ethics Committee of West China Hospital of Stomatology (No. WCHSIRB-D-2018-157).
Author Contributions
Conceptualization, YdZ and HW; methodology, YdZ and YfZ; performing of the experiments, YdZ and YfZ; collection and analysis of the data, YdZ and YfZ; writing—original draft preparation, YdZ; writing—review and editing, GH; funding acquisition, HW. All authors have read and agreed to the published version of the article.
Funding
This research was funded by the Sichuan Cadres Health Care Scientific Research Project (Key Project) Grant no. ZH 2021-901.
Conflict of Interest
The authors declare that the research was conducted in the absence of any commercial or financial relationships that could be construed as a potential conflict of interest.
Publisher’s Note
All claims expressed in this article are solely those of the authors and do not necessarily represent those of their affiliated organizations, or those of the publisher, the editors and the reviewers. Any product that may be evaluated in this article, or claim that may be made by its manufacturer, is not guaranteed or endorsed by the publisher.
Acknowledgments
We thank Runhe Liu, Qisi Lian, and Yuxiao Li for their invaluable contributions to part of the data collection and analysis for this work.
Supplementary Material
The Supplementary Material for this article can be found online at: https://www.frontiersin.org/articles/10.3389/fmats.2021.798391/full#supplementary-material
References
Abatangelo, G., Vindigni, V., Avruscio, G., Pandis, L., and Brun, P. (2020). Hyaluronic Acid: Redefining its Role. Cells 9, 1743. doi:10.3390/cells9071743
Abduo, J., and Elseyoufi, M. (2018). Accuracy of Intraoral Scanners: A Systematic Review of Influencing Factors. Eur. J. Prosthodont. Restor Dent 26, 101–121. doi:10.1922/EJPRD_01752Abduo21
Asparuhova, M. B., Kiryak, D., Eliezer, M., Mihov, D., and Sculean, A. (2019). Activity of Two Hyaluronan Preparations on Primary Human Oral Fibroblasts. J. Periodont Res. 54, 33–45. doi:10.1111/jre.12602
Awartani, F. A., and Tatakis, D. N. (2016). Interdental Papilla Loss: Treatment by Hyaluronic Acid Gel Injection: a Case Series. Clin. Oral Invest. 20, 1775–1780. doi:10.1007/s00784-015-1677-z
Baldini, A., Zaffe, D., and Nicolini, G. (2010). Bone-defects Healing by High-Molecular Hyaluronic Acid: Preliminary Results. Ann. Stomatol (Roma) 1, 2–7.
Becker, W., Gabitov, I., Stepanov, M., Kois, J., Smidt, A., and Becker, B. E. (2010). Minimally Invasive Treatment for Papillae Deficiencies in the Esthetic Zone: a Pilot Study. Clin. Implant Dent Relat. Res. 12, 1–8. doi:10.1111/j.1708-8208.2009.00247.x
Bertossi, D., Sbarbati, A., Cerini, R., Barillari, M., Favero, V., Picozzi, V., et al. (2013). Hyaluronic Acid: In Vitro and In Vivo Analysis, Biochemical Properties and Histological and Morphological Evaluation of Injected Filler. Eur. J. Dermatol. 23, 449–455. doi:10.1684/ejd.2013.2059
Bertl, K., Gotfredsen, K., Jensen, S. S., Bruckmann, C., and Stavropoulos, A. (2016). Adverse Reaction after Hyaluronan Injection for Minimally Invasive Papilla Volume Augmentation. A Report on Two Cases. Clin. Oral Impl. Res. 28, 871–876. doi:10.1111/clr.12892
Bukhari, S. N. A., Roswandi, N. L., Waqas, M., Habib, H., Hussain, F., Khan, S., et al. (2018). Hyaluronic Acid, a Promising Skin Rejuvenating Biomedicine: A Review of Recent Updates and Pre-clinical and Clinical Investigations on Cosmetic and Nutricosmetic Effects. Int. J. Biol. Macromolecules 120, 1682–1695. doi:10.1016/j.ijbiomac.2018.09.188
Cabreira, C. L., Fulginiti, R. L., Sesterheim, P., Shinkai, R. S. A., and Teixeira, E. R. (2021). Effect of Hyaluronic Acid on Paracrine Signaling of Osteoblasts from Mesenchymal Stromal Cells: Potential Impact on Bone Regeneration. Clin. Oral Invest. 25, 4571–4578. doi:10.1007/s00784-020-03771-x
Carnio, J., and Carnio, A. T. (2018). Papilla Reconstruction: Interdisciplinary Consideration for Clinical success. J. Esthet Restor Dent 30, 484–491. doi:10.1111/jerd.12411
Chen, J., Lan, Y., Baek, J.-A., Gao, Y., and Jiang, R. (2009). Wnt/beta-catenin Signaling Plays an Essential Role in Activation of Odontogenic Mesenchyme during Early Tooth Development. Developmental Biol. 334, 174–185. doi:10.1016/j.ydbio.2009.07.015
Cheng, H.-y., Chen, Y.-x., Wang, M.-f., Zhao, J.-y., and Li, L.-f. (2018). Evaluation of Changes in Skin Biophysical Parameters and Appearance after Pneumatic Injections of Non-cross-linked Hyaluronic Acid in the Face. J. Cosmet. Laser Ther. 20, 454–461. doi:10.1080/14764172.2018.1427868
Cho, S. B., Zheng, Z., Yoo, K. H., Kim, H. J., and Kim, H. (2019). Split‐face Comparison Study of Transcutaneous Pneumatic Injection Therapy with Isotonic and Hypertonic Glucose Solutions. J. Cosmet. Dermatol. 18, 487–494. doi:10.1111/jocd.12766
Chun, S. H., Kim, B. Y., Natari, S., and Kim, I. H. (2019). Needle‐free Pneumatic Injection Device; Histologic Assessment Using a Rat Model and Parameter Comparison in Predicting Collagen Synthesis Degree. Lasers Surg. Med. 51, 278–285. doi:10.1002/lsm.23046
Ciccone, V., Zazzetta, M., and Morbidelli, L. (2019). Comparison of the Effect of Two Hyaluronic Acid Preparations on Fibroblast and Endothelial Cell Functions Related to Angiogenesis. Cells 8, 1479. doi:10.3390/cells8121479
Cui, Y., Wang, F., Voorhees, J. J., and Fisher, G. J. (2021). Rejuvenation of Aged Human Skin by Injection of Cross-Linked Hyaluronic Acid. Plast. Reconstr. Surg. 147, 43S–49S. doi:10.1097/PRS.0000000000007620
da Costa, A., Biccigo, D. G. Z., de Souza Weimann, E. T., Mercadante, L. M., Oliveira, P. R. G., Prebianchi, S. B., et al. (2017). Durability of Three Different Types of Hyaluronic Acid Fillers in Skin: Are There Differences Among Biphasic, Monophasic Monodensified, and Monophasic Polydensified Products? Aesthet. Surg. J. 37, sjw161–581. doi:10.1093/asj/sjw161
de Santana, R. B., de Miranda, J. L. C., and de Santana, C. M. M. (2017). The Relationship between Open versus normal Contact point and Inter-proximal Papilla Dimensions in Periodontally Healthy Young Adults: A Controlled Clinical Trial. J. Clin. Periodontol. 44, 1164–1171. doi:10.1111/jcpe.12795
Fallacara, A., Durini, E., Vertuani, S., and Manfredini, S. (2017). Hyaluronic Acid Fillers in Soft Tissue Regeneration. Facial Plast. Surg. 33, 087–096. doi:10.1055/s-0036-1597685
Fan, Y., Choi, T.-H., Chung, J.-H., Jeon, Y.-K., and Kim, S. (2019). Hyaluronic Acid-Cross-Linked Filler Stimulates Collagen Type 1 and Elastic Fiber Synthesis in Skin through the TGF-β/Smad Signaling Pathway in a Nude Mouse Model. J. Plast. Reconstr. Aesthet. Surg. 72, 1355–1362. doi:10.1016/j.bjps.2019.03.032
Fujioka-Kobayashi, M., Müller, H.-D., Mueller, A., Lussi, A., Sculean, A., Schmidlin, P. R., et al. (2017). In Vitro effects of Hyaluronic Acid on Human Periodontal Ligament Cells. BMC Oral Health 17, 44. doi:10.1186/s12903-017-0341-1
Graça, M. F. P., Miguel, S. P., Cabral, C. S. D., and Correia, I. J. (2020). Hyaluronic Acid-Based Wound Dressings: A Review. Carbohydr. Polym. 241, 116364. doi:10.1016/j.carbpol.2020.116364
Heise, R. L., Stober, V., Cheluvaraju, C., Hollingsworth, J. W., and Garantziotis, S. (2011). Mechanical Stretch Induces Epithelial-Mesenchymal Transition in Alveolar Epithelia via Hyaluronan Activation of Innate Immunity. J. Biol. Chem. 286, 17435–17444. doi:10.1074/jbc.M110.137273
Hochman, M. N., Chu, S. J., da Silva, B. P., and Tarnow, D. P. (2019). Layperson's Esthetic Preference to the Presence or Absence of the Interdental Papillae in the Low Smile Line: A Web‐based Study. J. Esthet Restor Dent 31, 113–117. doi:10.1111/jerd.12478
Jin, L., Cao, Y., Yu, G., Wang, J., Lin, X., Ge, L., et al. (2017). SFRP2 Enhances the Osteogenic Differentiation of Apical Papilla Stem Cells by Antagonizing the Canonical WNT Pathway. Cell Mol Biol Lett 22. doi:10.1186/s11658-017-0044-2
Joshi, K., Baiju, C. S., Khashu, H., Bansal, S., and Maheswari, I. B. (2017). Clinical Assessment of Interdental Papilla Competency Parameters in the Esthetic Zone. J. Esthet Restor Dent 29, 270–275. doi:10.1111/jerd.12307
Jung, U.-W., Chang, Y.-Y., Um, Y.-J., Kim, C.-S., Cho, K.-S., and Choi, S.-H. (2011). Interproximal Periodontal Defect Model in Dogs: a Pilot Study. Oral Dis. 17, 26–32. doi:10.1111/j.1601-0825.2010.01694.x
Kim, H., Yoo, K. H., Zheng, Z., and Cho, S. B. (2017). Pressure- and Dose-Controlled Transcutaneous Pneumatic Injection of Hypertonic Glucose Solution for the Treatment of Atrophic Skin Disorders. J. Cosmet. Laser Ther. 19, 479–484. doi:10.1080/14764172.2017.1343950
Kim, J.-J., Song, H. Y., Ben Amara, H., Kyung-Rim, K., and Koo, K.-T. (2016). Hyaluronic Acid Improves Bone Formation in Extraction Sockets with Chronic Pathology: A Pilot Study in Dogs. J. Periodontol. 87, 790–795. doi:10.1902/jop.2016.150707
Kim, S.-B., Cho, J., Jue, S.-S., Park, J. H., and Kim, J. Y. (2020). Effect of Hyaluronic Acid Filler Injection on the Interdental Papilla in a Mouse Model of Open Gingival Embrasure. Ijerph 17, 4956. doi:10.3390/ijerph17144956
Kim, T. H., Bae, C. H., Lee, J. C., Ko, S. O., Yang, X., Jiang, R., et al. (2013). β-Catenin Is Required in Odontoblasts for Tooth Root Formation. J. Dent Res. 92, 215–221. doi:10.1177/0022034512470137
Kim, Z.-H., Lee, Y., Kim, S.-M., Kim, H., Yun, C.-K., and Choi, Y.-S. (2015). A Composite Dermal Filler Comprising Cross-Linked Hyaluronic Acid and Human Collagen for Tissue Reconstruction. J. Microbiol. Biotechnol. 25, 399–406. doi:10.4014/jmb.1411.11029
Kolte, A. P., Kolte, R. A., and Bawankar, P. (2018). Proximal Contact Areas of Maxillary Anterior Teeth and Their Influence on Interdental Papilla. Saudi Dental J. 30, 324–329. doi:10.1016/j.sdentj.2018.05.007
Kolte, A. P., Kolte, R. A., and Mishra, P. R. (2014). Dimensional Influence of Interproximal Areas on Existence of Interdental Papillae. J. Periodontol. 85, 795–801. doi:10.1902/jop.2013.130361
Krishnan, V., and Davidovitch, Z. (2009). On a Path to Unfolding the Biological Mechanisms of Orthodontic Tooth Movement. J. Dent Res. 88, 597–608. doi:10.1177/0022034509338914
Landau, M., and Fagien, S. (2015). Science of Hyaluronic Acid beyond Filling. Plast. Reconstr. Surg. 136, 188S–195S. doi:10.1097/prs.0000000000001823
Lee, W.-P., Kim, H.-J., Yu, S.-J., and Kim, B.-O. (2016a). Six Month Clinical Evaluation of Interdental Papilla Reconstruction with Injectable Hyaluronic Acid Gel Using an Image Analysis System. J. Esthet Restor Dent 28, 221–230. doi:10.1111/jerd.12216
Lee, W.-P., Seo, Y.-S., Kim, H.-J., Yu, S.-J., and Kim, B.-O. (2016b). The Association between Radiographic Embrasure Morphology and Interdental Papilla Reconstruction Using Injectable Hyaluronic Acid Gel. J. Periodontal Implant Sci. 46, 277–287. doi:10.5051/jpis.2016.46.4.277
Lee, W., Hwang, S.-G., Oh, W., Kim, C.-Y., Lee, J.-L., and Yang, E.-J. (2020). Practical Guidelines for Hyaluronic Acid Soft-Tissue Filler Use in Facial Rejuvenation. Ds 46, 41–49. doi:10.1097/DSS.0000000000001858
Liu, F., and Millar, S. E. (2010). Wnt/β-catenin Signaling in Oral Tissue Development and Disease. J. Dent Res. 89, 318–330. doi:10.1177/0022034510363373
Maeda, A., Ono, M., Holmbeck, K., Li, L., Kilts, T. M., Kram, V., et al. (2015). WNT1-induced Secreted Protein-1 (WISP1), a Novel Regulator of Bone Turnover and Wnt Signaling. J. Biol. Chem. 290, 14004–14018. doi:10.1074/jbc.M114.628818
Maiese, K. (2014). WISP1: Clinical Insights for a Proliferative and Restorative Member of the CCN Family. Cnr 11, 378–389. doi:10.2174/1567202611666140912115107
Martegani, P., Silvestri, M., Mascarello, F., Scipioni, T., Ghezzi, C., Rota, C., et al. (2007). Morphometric Study of the Interproximal Unit in the Esthetic Region to Correlate Anatomic Variables Affecting the Aspect of Soft Tissue Embrasure Space. J. Periodontol. 78, 2260–2265. doi:10.1902/jop.2007.060517
Matheus, H. R., Ervolino, E., Gusman, D. J. R., Alves, B. E. S., Fiorin, L. G., Pereira, P. A., et al. (2020). Association of Hyaluronic Acid with a Deproteinized Bovine Graft Improves Bone Repair and Increases Bone Formation in Critical‐size Bone Defects. J. Periodontol. doi:10.1002/jper.20-0613
Maturavongsadit, P., Bi, X., Metavarayuth, K., Luckanagul, J. A., and Wang, Q. (2017). Influence of Cross-Linkers on the In Vitro Chondrogenesis of Mesenchymal Stem Cells in Hyaluronic Acid Hydrogels. ACS Appl. Mater. Inter. 9, 3318–3329. doi:10.1021/acsami.6b12437
Nan, L., Zheng, Y., Liao, N., Li, S., Wang, Y., Chen, Z., et al. (2019). Mechanical Force Promotes the Proliferation and Extracellular Matrix Synthesis of Human Gingival Fibroblasts Cultured on 3D PLGA Scaffolds via TGF-β E-xpression. Mol. Med. Rep. 19, 2107–2114. doi:10.3892/mmr.2019.9882
Ni, J., Shu, R., and Li, C. (2019). Efficacy Evaluation of Hyaluronic Acid Gel for the Restoration of Gingival Interdental Papilla Defects. J. Oral Maxillofacial Surg. 77, 2467–2474. doi:10.1016/j.joms.2019.06.190
Nickel, J., and Mueller, T. D. (2019). Specification of BMP Signaling. Cells 8, 1579. doi:10.3390/cells8121579
Pereira, H., Sousa, D. A., Cunha, A., Andrade, R., Espregueira-Mendes, J., Oliveira, J. M., et al. (2018). Hyaluronic Acid. Adv. Exp. Med. Biol. 1059, 137–153. doi:10.1007/978-3-319-76735-2_6
Philipp-Dormston, W. G., Bergfeld, D., Sommer, B. M., Sattler, G., Cotofana, S., Snozzi, P., et al. (2017). Consensus Statement on Prevention and Management of Adverse Effects Following Rejuvenation Procedures with Hyaluronic Acid-Based Fillers. J. Eur. Acad. Dermatol. Venereol. 31, 1088–1095. doi:10.1111/jdv.14295
Pi, S., Choi, Y. J., Hwang, S., Lee, D.-W., Yook, J. I., Kim, K.-H., et al. (2017). Local Injection of Hyaluronic Acid Filler Improves Open Gingival Embrasure: Validation through a Rat Model. J. Periodontol. 88, 1221–1230. doi:10.1902/jop.2017.170101
Pirnazar, P., Wolinsky, L., Nachnani, S., Haake, S., Pilloni, A., and Bernard, G. W. (1999). Bacteriostatic Effects of Hyaluronic Acid. J. Periodontol. 70 (4), 370–374. doi:10.1902/jop.1999.70.4.370
Sadat Mansouri, S., Ghasemi, M., Salmani, Z., and Shams, N. (2013). Clinical Application of Hyaluronic Acid Gel for Reconstruction of Interdental Papilla at the Esthetic Zone. J. Islamic Dental Assoc. IRAN (Jidai) 25, 208–214.
Sarkar, L., and Sharpe, P. T. (1999). Expression of Wnt Signalling Pathway Genes during Tooth Development. Mech. Dev. 85, 197–200. doi:10.1016/s0925-4773(99)00095-7
Smith, P. C., Martínez, C., Martínez, J., and McCulloch, C. A. (2019). Role of Fibroblast Populations in Periodontal Wound Healing and Tissue Remodeling. Front. Physiol. 10, 270. doi:10.3389/fphys.2019.00270
Tanwar, J., and Hungund, S. (2016). Hyaluronic Acid: Hope of Light to Black Triangles. J. Int. Soc. Prevent Communit Dent 6, 497–500. doi:10.4103/2231-0762.192948
Tarnow, D. P., Magner, A. W., and Fletcher, P. (1992). The Effect of the Distance from the Contact point to the Crest of Bone on the Presence or Absence of the Interproximal Dental Papilla. J. Periodontol. 63, 995–996. doi:10.1902/jop.1992.63.12.995
Wang, F., Garza, L. A., Kang, S., Varani, J., Orringer, J. S., Fisher, G. J., et al. (2007). In Vivo stimulation of De Novo Collagen Production Caused by Cross-Linked Hyaluronic Acid Dermal Filler Injections in Photodamaged Human Skin. Arch. Dermatol. 143, 155–163. doi:10.1001/archderm.143.2.155
Wu, Y., Sun, N., Xu, Y., Liu, H., Zhong, S., Chen, L., et al. (2016). Clinical Comparison Between Two Hyaluronic Acid-Derived Fillers in the Treatment of Nasolabial Folds in Chinese Subjects: BioHyalux Versus Restylane. Arch. Dermatol. 308 (3), 145–151. doi:10.1007/s00403-016-1630-2
Xing, F., Zhou, C., Hui, D., Du, C., Wu, L., Wang, L., et al. (2020). Hyaluronic Acid as a Bioactive Component for Bone Tissue Regeneration: Fabrication, Modification, Properties, and Biological Functions. Nanotechnology Rev. 9, 1059–1079. doi:10.1515/ntrev-2020-0084
Zhao, N., Wang, X., Qin, L., Zhai, M., Yuan, J., and Chen, J. (2016). Effect of Hyaluronic Acid in Bone Formation and Its Applications in Dentistry. J. Biomed. Mater. Res. A. 104 (6), 1560–1569. doi:10.1002/jbm.a.35681
Zhai, P., Peng, X., Li, B., Liu, Y., Sun, H., and Li, X. (2020). The Application of Hyaluronic Acid in Bone Regeneration. Int. J. Biol. Macromolecules 151, 1224–1239. doi:10.1016/j.ijbiomac.2019.10.169
Zhang, Y., Hong, G., Zhang, Y., Sasaki, K., and Wu, H. (2020). Minimally Invasive Procedures for Deficient Interdental Papillae: A Review. J. Esthet Restor Dent 32, 463–471. doi:10.1111/jerd.12608
Keywords: hyaluronic acid, interdental papilla, biopolymer materials, animal study, biocompatibility
Citation: Zhang Y, Zhang Y, Wu H and Hong G (2021) Application of Hyaluronic Acid as a Biopolymer Material in Reconstruction of Interdental Papilla in Rats. Front. Mater. 8:798391. doi: 10.3389/fmats.2021.798391
Received: 20 October 2021; Accepted: 12 November 2021;
Published: 23 December 2021.
Edited by:
Francesco Baino, Politecnico di Torino, ItalyReviewed by:
Ziyad S. Haidar, University of the Andes, ChileGloria Huerta-Angeles, Contipro Inc., Czechia
Copyright © 2021 Zhang, Zhang, Wu and Hong. This is an open-access article distributed under the terms of the Creative Commons Attribution License (CC BY). The use, distribution or reproduction in other forums is permitted, provided the original author(s) and the copyright owner(s) are credited and that the original publication in this journal is cited, in accordance with accepted academic practice. No use, distribution or reproduction is permitted which does not comply with these terms.
*Correspondence: Hongkun Wu, d3Voa3NjdUAxMjYuY29t