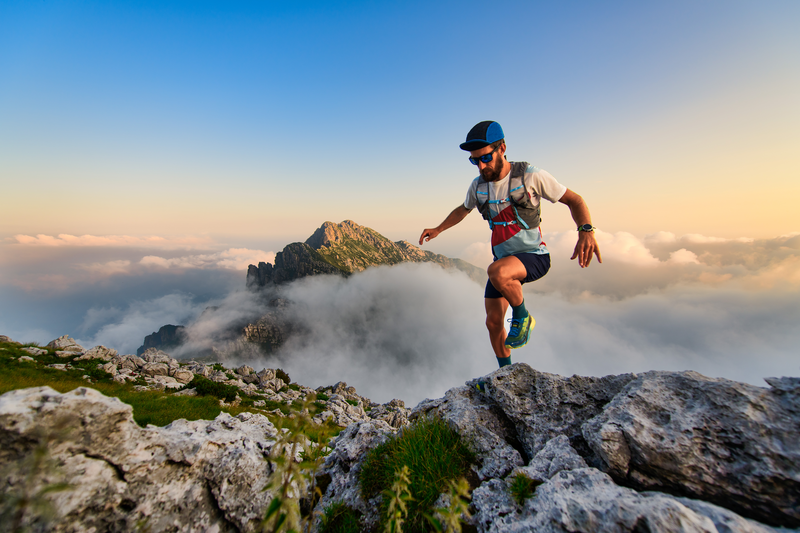
95% of researchers rate our articles as excellent or good
Learn more about the work of our research integrity team to safeguard the quality of each article we publish.
Find out more
ORIGINAL RESEARCH article
Front. Mater. , 15 November 2021
Sec. Structural Materials
Volume 8 - 2021 | https://doi.org/10.3389/fmats.2021.792561
This article is part of the Research Topic Non-BF Slag-based Green Cementitious Materials View all 20 articles
The strength characteristics of cemented paste backfill (CPB) in mining areas are key control factors for the safety assessment of overlying strata. A series of experiments about uniaxial compression and triaxial compression were carried out to study the influence of cement content of filling slurry, curing age, and curing temperature on strength behavior of CPB specimens. The failure mechanism and damage feature of CPB have been investigated. The results show the following: (1) The uniaxial compressive strength of CPB specimens exhibits an upward trend with the increase of cement content and curing age. When the cement content is high, the uniaxial compressive strength increases sharply with increasing curing age. (2) The cohesion of CPB specimens increases with the increase of cement content of filling slurry, curing age, and curing temperature. The cohesion of CPB specimens with curing age 7 days and 14 days increases linearly with increasing cement content. At the later stage of curing age, the strength growth of high cement content backfill is significantly greater than that of low cement content. The internal friction angle of the filling increases slightly with increasing filling cement content, curing age, and curing temperature. (3) The shear strength of CPB specimens at curing age 7 days exhibits an upward trend with the increase of confining pressure, while the shear strength at 14 days and 28 days curing age decreases slightly as the confining pressure increases. (4) With the increase of cement content in backfill, the brittleness increases significantly when the backfill is damaged. The failure mode of CPB specimens changes from monoclinic section shear failure to X-type failure with increasing curing age, and the failure process is divided into four stages: pore compaction, linear elastic deformation, plastic deformation, and post-peak failure.
With the increase of mining depth, geological disasters, such as underground rock burst and roof caving, occur frequently. At same time, there are potential risks, for instance, dam failure and environmental pollution in the tailing reservoir, formed by tailings accumulation (Cai, 2009; Yang et al., 2018). Tailings cemented filling mining method can effectively use mine tailings and prepare cemented filling slurry to backfill the goaf, so as to control the ground pressure, support the stope, reduce the surface subsidence, and improve ore recovery (Zhao and Hu, 2008; Lu et al., 2008). Therefore, the tailings cemented filling mining method is widely adopted by many mines (Ghirian and Fall, 2014; Jiang et al., 2019).
The mechanical properties of cemented paste backfill (CPB) are the key to the safe application of backfill mining method, and it is also the main controlling factor for safety assessment of overlying strata in the mine goaf. In order to better utilize the mechanical properties of the CPB to serve the mining operation, many scholars have conducted a lot of research work on compressive tests. Fall et al. (2005), Guo and Li, 2017, and Hou et al. (2021) explored the relationship between uniaxial compressive strength and material ratio of CPB. Li et al. (2005), Wang et al. (2015), and Zhang et al. (2020) optimized the filling material ratio to meet the actual demand of project through orthogonal test. Cao et al. (2016, 2019) studied the influence of CPB stratification on strength by simulating the interval between actual filling groups. Nie et al. (2016) tested the strength of CPB with different water contents and determined the influence of water content on the strength of CPB. Meanwhile, the microscopic damage evolution law of CPB during compression has also been widely concerned. Xie et al. (2015), Li, 2019, and Zhao et al. (2020) tested the acoustic emission parameters of specimens under compression, and analyzed the influence of curing temperature and cement content on the development of microcracks in CPB specimens. Yi et al., 2020 investigated the internal response of CPB during compression by rock CT scanner. Wei et al. (2020) explored the crack propagation process using crack camera observation system. The relationship between fracture area and tensile strength of cement paste with supplementary cementitious materials and the influence of new SCM on the long-term durability of concrete have been analyzed (Li and Li, 2015; Juenger et al., 2019). Richardson (2008) reviewed the model of calcium silicate hydrates. Li et al. (2020a) measured the intrinsic nanomechanical properties of C-S-H under different Ca/Si conditions by high-pressure X-ray diffraction method and made fibrillar C-S-H seeds from hydrated tricalcium silicate lower cement demand. The effects of curing time, cement content, and saturation state on mode-I fracture toughness of CPB have been studied (Libos and Cui, 2020). In addition, Zhou et al., 2019 and Wang, 2014 carried out the numerical simulation of uniaxial compression process of CPB. In triaxial compression test, Wu et al. (2019, 2021) studied the influence of cement content and inclined interface angle on the strength characteristics and failure mode of CPB. Yang et al. (2018) and Xu et al. (2019) analyzed the failure mode and energy dissipation characteristics of CPB. Fall et al. (2007), Rankine and Sivakugan, 2007, and Xu et al., 2014 revealed the deformation characteristics of CPB in compression. Those studies are valuable in stope engineering, yet it is worth noting that most research focus on uniaxial compression, which does not agree with reality, the actual three-dimensional stress state (Liu et al., 2019). Furthermore, the occurrence environment of backfill is complex, which indicates that the mechanical property of CPB could be affected by various factors.
The objective of this study is to determine the influence of cement content, curing age, and curing temperature on uniaxial compressive and triaxial shear strength of CPB. A series of uniaxial compression tests and triaxial compression tests are carried out to analyze the strength and failure characteristics of the CPB. The quantitative relationship between uniaxial compressive strength and triaxial shear strength has been obtained. This study can provide a valuable reference for the efficient application of CPB.
The tailings utilized in this test were obtained from a copper mine, located in Anhui Province of China. The basic physical properties of the tailings were determined according to Rock Test Regulations for Water Conservancy and Hydropower Engineering (SL/T 264-2020). The natural density of the tailings is 1.424 g/ cm3, the dry density is 1.420 g/ cm3, and the moisture content is 0.977%. The particle size distribution (PSD) is shown in Figure 1.
It can be seen from Figure 1 that the cumulative pass rate of the tailings with particle size less than 20 microns reaches 44% (more than 35%), which belongs to the finer-grained tailings. This particle gradation can effectively reduce the layering and segregation in the slurry mixing process and make the internal aggregate distribution of the specimens more uniform, which makes the mechanical properties more stable.
With full tailings as aggregate and P. O 42.5 Portland cement as cementing material, multiple cylindrical tailings CPB specimens (∅ 50 mm × 100 mm) were prepared. The mass concentration of filling specimen was 72%, and the cement contents were 7, 10, and 25% (mass ratio). Afterwards, the CPB specimens were cured at room temperature 20 ± 0.5°C and humidity 95 ± 5% for 7, 14, and 28 days, respectively. These specimens were used to conduct experiments investigating the influence of cement content and curing age of CPB strength.
A series of uniaxial and triaxial compression tests of CPB specimens were performed by TruePath automatic stress path triaxial apparatus. The loading rate is 1 mm/ min, and the confining pressures of triaxial compression test are set to 100, 200, 300, and 400 kPa, respectively. The influence of three factors, namely, cement content, curing age, and curing temperature, on strength was discussed in this section. The specific test scheme is shown in Table 1.
The uniaxial compression test of CPB specimens focuses on the influence of slurry cement content and curing age on the uniaxial compressive strength. The experimental results are shown in Figure 2. The uniaxial compressive strength exhibits an upward trend with the increase of cement content and curing age. It is evident that the compressive strength increases slowly and maintains constant amplitude when the cement content is less than 10%, yet the compressive strength of specimens increases sharply as the cement content is greater than 10% (25% this test). It is worth noting that the strength value reaches 3.4 MPa at 28 days curing age (25% cement content), which is 1.7 times higher than that of 7 days (2.0 MPa/7% cement content) under the same working condition. The trends of relationship curve are obviously different with various cement content and curing age, even for the same specimens. The results of specimens with curing age ( days) and cement content (25%) are the most prominent. So, it can be concluded that the curing age has a more significant effect on the strength increase of high cement content specimens.
It can be seen from the results of Figure 2 that when the cement content is higher (25%) and the curing age is longer (28 days), the compressive strengths of CPB specimens are significantly higher than those under other conditions. Increasing cement content can efficiently enhance the cementing effect of CPB, reduce the internal pores and gaps of the specimen, and cause increase of the uniaxial compressive strength. Meanwhile, prolonging the curing age is beneficial to promote the internal hydration reaction, so that the hydration reaction of a large number of gelling materials inside CPB specimens is more fully developed, and more calcium silicate hydrate is generated, so as to significantly increase the uniaxial compressive strength (Hou et al., 2021). When the cement content is higher (25%) and the curing period is longer (28 days), the compressive strength value of CPB is significantly higher than the strength of the samples under other working conditions, which indicates when the curing time is longer and the cement content is higher, the hydration reaction of a large amount of cementitious material inside the CPB will react more fully, which can significantly improve the uniaxial compressive strength of CPB specimens. However, for the specimens with low cement content and short curing age, due to the insufficient progress of the cement hydration reaction and insufficient hydration products, there are many pores and micro-cracks inside the specimens and the original defects are serious. The corresponding compressive strength is lower and damage is more likely to occur.
A total of 60 sets of triaxial compression tests with different cement contents and curing ages were carried out in this study. By drawing the molar stress circles of filling bodies with different cement contents under different curing ages and confining pressures, the Mohr–Coulomb criterion was adopted. The shear failure line of each CPB specimen is fitted, and the cohesion and internal friction angle of the corresponding specimen are obtained. The fitting results are shown in Figure 3.
The relationship curves between the cohesion, internal friction angle, and curing age of the CPB specimens with different cement contents are shown in the following figures:
As shown in Figure 4; Figure 5, the cohesion of CPB specimens increases with the increase of cement content and curing age, and the greater the cement content, the more obvious the increase. The values of specimens range from 190 to 790 kPa. When the cement content is 25%, the cohesion with curing age (28 days) reaches 790 kPa, which is 390 kPa higher than that (400 kPa) at 7 days. The main reason is that the CPB specimens with higher cement content will generate more binging phases (calcium silicate hydrate), which makes the bonding between tailings particles and cement tighter, and the bonding effect is better. It can be seen that, to achieve a cohesive strength of about 400 kPa, from the perspective of saving filling costs, a design scheme with low cement content and high curing age can be preferred. The friction angle increases slowly with increasing curing age and cement content. However, comparing the internal friction angle values of the specimens, there is a little difference between the friction angles of the CPB specimens with different curing ages and cement contents. The values of internal friction angle range from 26.6° to 29.7°; the change is only about 3.1°. This result shows that the influence of cement hydration on the friction angle of the CPB is relatively small.
In order to study the influence of curing temperature on shear strength, a series of triaxial tests were carried out for the specimens with cement 10% and curing age 7 days at seven curing temperatures (20°C, 25°C, 30°C, 35°C, 40°C, 45°C, and 50°C). The relationship curves between shear strength indexes and curing temperature are shown as Figure 6.
With the increase of curing temperature, the cohesive forces of CPB specimens increase rapidly and significantly, from 243 kPa corresponding to 25°C to 430 kPa corresponding to 50°C, which nearly doubled. This is because the higher curing temperature accelerates the hydration of the binder, and the hydration products increase with the increasing curing temperature, and the cement hydration product (C-S-H) is the main binding phase. The friction angle increases slightly with the increase of curing temperature, and the change value is only about 2.4°. Therefore, the increase of curing temperature can significantly increase the cohesive strength, but the effect of increasing the friction strength is not obvious.
Under a confining pressure of 300 kPa, the failure conditions of CPB specimens in the triaxial compression test are shown in Figure 7. When the specimen with a curing age 7 days and cement content 25% is broken, a penetrating and inclined tensile-shear failure surface appears, and there is a slight surface peeling. However, the shape of two other cement content tests is still relatively complete without obvious cracks, but the height reduction and lateral expansion appear, and there are some wrinkles and small cracks on the surface. This indicates that as the cement content decreases, the ductility of CPB specimens gradually increases when it is broken. This is due to the fact that when the cement content is relatively low, enough hydration products are not generated inside the CPB to cement and fill the pores, and there is still compressible space inside, so it has a strong deformability. The surface wrinkles decrease with the increase of the cement content during the curing age of 14 days and 28 days, and there is no obvious sign of damage. The reason is that with the increase of curing age, the amount of C-S-H generated inside the specimens increases and tends to be stable gradually, so that the brittleness of CPB specimens improves when it is damaged. As the curing age increases to 28 days, the failure form of the CPB changes from a single oblique cross-section shear failure to an X-shaped failure, and there is a local fall-off phenomenon in the specimen with a cement content of 25%, and the damage becomes more serious and there exist lumps. In the actual engineering, the design of cement content and curing age of CPB should not only consider the strength, but also take the deformation characteristics into account, so that it can better provide support for the safety of surrounding rock.
Taking the stress–strain curve of backfill under confining pressure of 300 kPa, 25% cement content after 28 days of curing as an example (curing temperature 20 ± 0.5°C), the compression failure process of specimen has been analyzed. The result is shown in Figure 8.
It can be concluded that the compression deformation and failure of the CPB can be roughly divided into the following four stages:
1) Pore compaction stage (section OA). In the initial stage of compression, the internal pores and cracks are gradually compacted and closed, so that the strength of the specimen is improved.
2) Linear elastic deformation stage (section AB), the internal cracks gradually develop and penetrate in the end. At this stage, the stress–strain curve of the CPB is close to a straight line, and the stress growth rate gradually slows down.
3) Plastic deformation stage (section BC). As the load further increases, new cracks continue to be generated and intertwined with the original cracks, gradually extending and converging in the direction of stress action, and then the specimen enters the stage of yield failure.
4) Post-peak failure stage (section CD). Plastic deformation further develops, the load-bearing capacity of the CPB decreases rapidly, and visible macroscopic cracks are formed on the surface. The bearing capacity of specimen continues to decrease rapidly until it is completely destroyed.
Taking the CPB specimens with curing age 7, 14, and 28 days as an example (curing temperature 20 ± 0.5°C), the relationships between the maximum principal stress and the confining pressure under uniaxial compression and triaxial compression test conditions are compared and analyzed, as shown in Figure 9.
It can be found from Figure 9 that the maximum principal stress of the CPB under the axial force state is significantly lower than that of three-direction force state. Furthermore, the maximum principal stress increases nearly linearly with the increase of the confining pressure. Taking the confining pressure 400 kPa and curing age 28 days as an example, the differences between confining pressure 400 kPa and confining pressure-free (cement content 7, 10, and 25%) are 1.5, 1.7, and 0.4 MPa, respectively. The reason is that under the three-way stress state, the confining pressure limits the material deformation caused by the axial force, thereby improving the compressive resistance of the specimens.
In this study, the results of an experimental investigation on the influence of cement content, curing age, and curing temperature on the compression behavior of CPB specimens are presented. The following conclusions were drawn:
1) The uniaxial compressive strength of CPB increases with the increase of cement content and curing age. When the cement content is high, the influence of curing age on the strength of CPB is more obvious.
2) The cement content, curing age, and curing temperature have an important influence on strength and cohesion. In the curing temperature range 25°C–50°C, increasing the curing temperature can significantly increase the cohesion. As the cement content is relatively low, after 14 days of curing age, the compressive strength and cohesion slow down.
3) The internal friction angle increases gradually with the increase of the cement content, curing age, and curing temperature, but the degree of influence is small.
4) With the increase of the curing age, the failure form of the CPB changes from the single oblique section shear failure to the X-shaped failure. The failure process is divided into four stages: pore compaction, linear elastic deformation, plastic deformation, and post-peak failure.
The raw data supporting the conclusions of this article will be made available by the authors, without undue reservation.
ZZ contributed to design of the study and wrote the draft of the manuscript. JL carried out the experiment and performed the data analysis. All authors contributed to manuscript revision, read, and approved the submitted version.
This research was supported by the Key Program of the National Natural Science Foundation of China (No. 52130905).
The authors declare that the research was conducted in the absence of any commercial or financial relationships that could be construed as a potential conflict of interest.
All claims expressed in this article are solely those of the authors and do not necessarily represent those of their affiliated organizations, or those of the publisher, the editors and the reviewers. Any product that may be evaluated in this article, or claim that may be made by its manufacturer, is not guaranteed or endorsed by the publisher.
Cao, S., Song, W.-d., Xue, G.-l., Ma, R.-w., and Zhu, P.-r. (2016). Variation Law of Mechanical Properties and Failure Mode of Layered Tailings Cemented CPB. J. China Univ. Min. Technol. 45, 717–722.
Cao, S., Yilmaz, E., Song, W. D., and Xue, G. L. (2019). Assessment of Acoustic Emission and Triaxial Mechanical Properties of Rock-Cemented Tailings Matrix Composites. Adv. Mater. Sci. Eng. 2019, 6742392. doi:10.1155/2019/6742392
Fall, M., Belem, T., Samb, S., and Benzaazoua, M. (2007). Experimental Characterization of the Stress-Strain Behaviour of Cemented Paste Backfill in Compression. J. Mater. Sci. 42, 3914–3922. doi:10.1007/s10853-006-0403-2
Fall, M., Benzaazoua, M., and Ouellet, S. (2005). Experimental Characterization of the Influence of Tailings Fineness and Density on the Quality of Cemented Paste Backfill. Minerals Eng. 18, 41–44. doi:10.1016/j.mineng.2004.05.012
Ghirian, A., and Fall, M. (2014). Coupled Thermo-Hydro-Mechanical-Chemical Behaviour of Cemented Paste Backfill in Column Experiments. Eng. Geology. 170, 11–23. doi:10.1016/j.enggeo.2013.12.004
Guo, T.-r., and Li, Z.-j. (2017). Experimental Study on Reasonable Proportion of Cemented CPB for Iron Ore Tailings. China Met. Bull. 9, 112–113.
Hou, Y.-q., Yin, S.-h., Cao, Y., Yang, S.-x., Zhang, M.-z., Kou, P.-f., et al. (2021). Uniaxial Compressive Stress-Strain Relationship and Damage Constitutive Model of Tailings Cemented Backfill. Mater. Rep. 16, 1–15.
Jiang, H., Fall, M., Li, Y., and Han, J. (2019). An Experimental Study on Compressive Behaviour of Cemented Rockfill. Construction Building Mater. 213, 10–19. doi:10.1016/j.conbuildmat.2019.04.061
Juenger, M. C. G., Snellings, R., and Bernal, S. A. (2019). Supplementary Cementitious Materials: New Sources, Characterization, and Performance Insights. Cement Concrete Res. 122, 257–73. doi:10.1016/j.cemconres.2019.05.008
Li, J., Zhang, W., and Monteiro, P. J. M. (2020a). Structure and Intrinsic Mechanical Properties of Nanocrystalline Calcium Silicate Hydrate. ACS Sustainable Chem. Eng. 8, 12453–61. doi:10.1021/acssuschemeng.0c03230
Li, J., Zhang, W., Xu, K., and Monteiro, P. J. M. (2020b). Fibrillar Calcium Silicate Hydrate Seeds from Hydrated Tricalcium Silicate Lower Cement Demand. Cement Concrete Res. 137, 106195. doi:10.1016/j.cemconres.2020.106195
Li, K.-b. (2019). The Mechanical and Acoustic Emission Characteristics of CPB under Uniaxial Compression under Temperature Influence. China: Wuhan University of Technology.
Li, Y.-f., Zhang, J.-m., Deng, F., and Bai, S.-w. (2005). Experimental Study on Strength Characteristics of CPB in Deep Goaf. Rock. Soil Mech. 26, 865–8. doi:10.3969/j.issn.1000-7598.2005.06.00
Li, Y., and Li, J. (2015). Relationship between Fracture Area and Tensile Strength of Cement Paste with Supplementary Cementitious Materials. Construction Building Mater. 79, 223–8. doi:10.1016/j.conbuildmat.2015.01.052
Libos, I. L. S., and Cui, L. (2020). Effects of Curing Time, Cement Content, and Saturation State on Mode-I Fracture Toughness of Cemented Paste Backfill. Eng. Fracture Mech. 235, 107174. doi:10.1016/j.engfracmech.2020.107174
Liu, G.-s., Yang, X.-c., and Guo, L.-j. (2019). Three-Dimensional Arch Stress and Strength Demand Model of Subsequent CPB in Stage Open Field. J. China Coal Soc. 44, 1391–1403. doi:10.13225/j.cnki.jccs.2019.6025
Lu, S.-b., Li, X., and Liao, Q.-l. (2008). Application Prospect and Environmental Protection of Filling Mining Method. Nonferrous Met. (Mining Section). doi:10.3969/j.issn.1001-1277.2008.01.006
Nie, Y.-l., Wang, X,-j., Huang, G.-l., Chao, L,-s., Feng, X., and Cao, S.-r. (2016). Strength and Damage Model Analysis of CPB with Different Moisture Content. Bull. Chin. Ceram. Soc. 37, 2008–2013. doi:10.16552/j.cnki.issn1001-1625.2018.06.035
Rankine, R. M., and Sivakugan, N. (2007). Geotechnical Properties of Cemented Paste Backfill from Cannington Mine, Australia. Geotech. Geol. Eng. 25, 383–393. doi:10.1007/s10706-006-9104-5
Richardson, I. G. (2008). The Calcium Silicate Hydrates. Cement Concrete Res. 38, 137–58. doi:10.1016/j.cemconres.2007.11.005
Wang, X.-m., Hu, Y.-b., Wang, S., Liu, J.-x., Chen, Y., and Bian, J.-w. (2015). Orthogonal Experimental Study on Optimization of Filling Ratio of Superfine Tailings. Gold. Sci. Technol. 3, 45–49. doi:10.11872/j.issn.1005-2518.2015.03.045
Wang, Y.-p. (2014). Study on Cemented Waste Rock Filling Process and Numerical Simulation of Meso-Level Mechanical Properties of CPB. China: Central South University.
Wei, X.-m., Guo, L.-j., Zhou, X.-l., Li, C.-h., and Zhang, L.-x. (2020). Research on Full Time Sequence Stress Evolution Law and Prediction Model of High Stage Cemented. CPB. Rock. Soil Mech. 41, 3613–3620. doi:10.16285/j.rsm.2020.0585
Wu, W.-l., Liu, G.-s., Guo, L.-j., Fan, C., Zhang, Z.-h., and Zhang, X-m. (2019). Study on Mechanical Properties of Interface between Cemented CPB and Rock Based on Direct Shear Test. China Min. Mag. 28, 116–122. doi:10.12075/j.issn.1004-4051.2019.12.008
Wu, W.-l., Xu, W.-b., and Zuo, J.-p. (2021). Effect of Inclined Interface Angle on Shear Strength and Deformation Response of CPB-Rock under Triaxial Compression. Constr. Build. Mater. 279, 122478. doi:10.1016/j.conbuildmat.2021.122478
Xie, Y., He, W., Zhu, Z.-c., Liu, J.-x., Liu, H., and Xie, T. (2015). Acoustic Emission Characteristics and Damage Evolution of CPB under Uniaxial Compression. Chin. J. Appl. Mech. 32, 670–676. doi:10.11776/cjam.32.04.D034
Xu, W.-b., Song, W.-d., Dong, X., and Ma, Y. (2019). Analysis of Triaxial Compression Deformation Failure and Energy Dissipation Characteristics of Cemented. CPB. Rock. Soil Mech. 35, 3421–3429. doi:10.16285/j.rsm.2014.12.025
Xu, W.-b., Song, W.-d., Wang, D.-x., and Ma, Y. (2014). Characteristic Analysis of Deformation Failure and Energy Dissipation of Cemented CPB Body under Triaxial Compression. Rock. Soil Mech. 35, 3421–3429. doi:10.16285/j.rsm.2014.12.025
Yang, S.-x., Fu, Y.-h., Peng, J.-h., and Yang, M.-s. (2018). Analysis of Failure Mode and Energy Dissipation Characteristics of Full Tailings CPB under Triaxial Compression. Ind. Miner. Process. 47, 30–33. doi:10.16283/cnki.hgkwyjg.0.2018-06-008
Yang, X., and Guo, L.-j. (2018). Comprehensive Utilization Technology of Tailings and Waste Rock. Beijing: Chemical Industry Press.
Yi, X.-F., Liu, C.-K., and Wang, Y. (2020). In Situ CT Scanning Experimental Study on Fracture Evolution Process of Metal Tailings Cemented CPB. Rock. Soil Mech. 41, 3365–3373. doi:10.16285/j.rsm.2019.1962
Zhang, L., Guo, L.-j., and Li, W.-c. (2020). Experimental Study on Preparation of Filling Cementitious Materials Based on Copper-Nickel Smelting Slag. Gold. Sci. Technol. 28, 669–677. doi:10.11872/j.issn.1005-2518.2020.05.106
Zhao, C.-q., and Hu, N.-l. (2008). Development and Application of Filling Cementitious Materials. Gold 29, 25–29. doi:10.3969/j.issn.1001-1277.2008.01.006
Zhao, K., Yu, X., Zhu, S., Yan, Y., and Huang, M. (2020). Acoustic Emission Fractal Characteristics and Mechanical Damage Mechanism of CPB Prepared with Tantalum Niobium Mine Tailings. Constr. Build. Mater. 258, 1–11. doi:10.1016/j.conbuildmat.2020.119720
Keywords: cemented paste backfill, uniaxial compression, triaxial compression, shear strength, failure mode
Citation: Zhang Z and Li J (2021) Experimental Investigation on Strength and Failure Characteristics of Cemented Paste Backfill. Front. Mater. 8:792561. doi: 10.3389/fmats.2021.792561
Received: 10 October 2021; Accepted: 19 October 2021;
Published: 15 November 2021.
Edited by:
Lijie Guo, Beijing General Research Institute of Mining and Metallurgy, ChinaReviewed by:
Jiaqi Li, University of California, Berkeley, United StatesCopyright © 2021 Zhang and Li. This is an open-access article distributed under the terms of the Creative Commons Attribution License (CC BY). The use, distribution or reproduction in other forums is permitted, provided the original author(s) and the copyright owner(s) are credited and that the original publication in this journal is cited, in accordance with accepted academic practice. No use, distribution or reproduction is permitted which does not comply with these terms.
*Correspondence: Zhihong Zhang, emhhbmd6aDIwMDJAMTI2LmNvbQ==
Disclaimer: All claims expressed in this article are solely those of the authors and do not necessarily represent those of their affiliated organizations, or those of the publisher, the editors and the reviewers. Any product that may be evaluated in this article or claim that may be made by its manufacturer is not guaranteed or endorsed by the publisher.
Research integrity at Frontiers
Learn more about the work of our research integrity team to safeguard the quality of each article we publish.