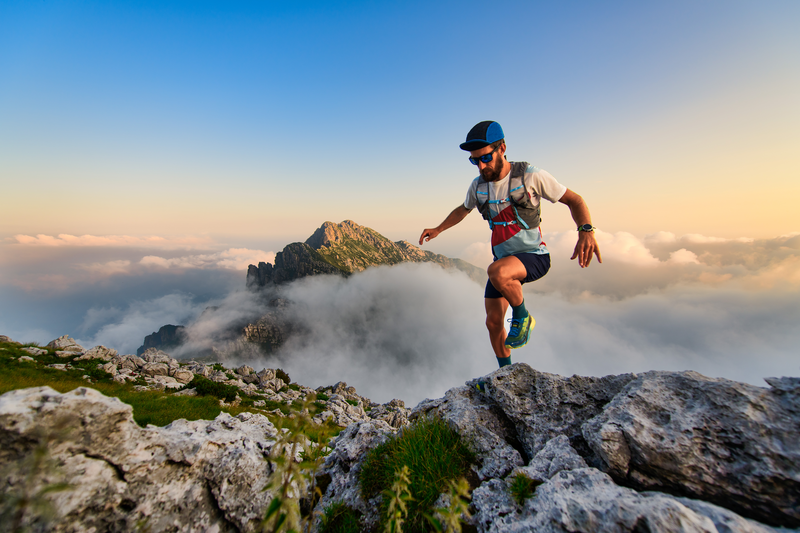
95% of researchers rate our articles as excellent or good
Learn more about the work of our research integrity team to safeguard the quality of each article we publish.
Find out more
REVIEW article
Front. Mater. , 15 December 2021
Sec. Quantum Materials
Volume 8 - 2021 | https://doi.org/10.3389/fmats.2021.783239
Nonlinear optical (NLO) materials have spanned a large area of science and technology owning to their potential applications in optoelectronics. The invention of the first Ruby laser has sparked a fresh interest in the area of nonlinear optics. The computational designing and experimental synthesis of organic and inorganic NLO materials with higher order nonlinearities come into vogue in the field of materials science. To date, several strategies including metal ligand framework, push pull mechanism, diradical character, and so on have been devised to enhance the NLO response of materials. In addition, introduction of diffuse excess electrons is an efficient approach to design noncentrosymmetric materials for nonlinear optics. The current review highlights a systematic array of different computational studies (covering the last decade of intensive research work) for the theoretical designing of NLO materials. In the present review, theoretical designing from the simplest NLO material to the complex alkali, alkaline earth, transition, and superalkali doped nanomaterials is summarized. The emergence of excess electrons strategy has played a pivotal role in enhancing the NLO properties especially hyperpolarizabilities. We expect that this review will provide a better understanding of the NLO responses of nanoclusters, paving the way for the advancement of hi-tech NLO materials to meet the real challenges in optoelectronics.
Nonlinear optics is a new and versatile branch of science that describes the light-matter interaction when induced polarization nonlinearly depends on external electric and magnetic fields (Boyd, 2020; Shen, 1984). Nonlinear optical (NLO) phenomenon arises when a material generates electromagnetic radiations of varying phase, amplitude, and frequency during its propagation on interaction with electromagnetic fields (Tessore et al., 2018). The NLO effect is produced when strong electrical fields under high intensity laser beams interact with matter. In 1960, the invention of the first Ruby laser by Maiman sparked fresh interest in the field of nonlinear optics (Maiman, 1960). Franken et al. also complemented this phenomenon by observing the second harmonic generation (SHG) effect in quartz crystals (Franken et al., 1961). The simple manifestations of nonlinear optics include intensity-dependent light refraction and absorption (Majumdar et al., 2020; Wang et al., 2018). The formation of solitons (Z. Luo et al., 2015), power restriction of light transmission (Lettow and Martin, 2010), and multiphoton fluorescence (Wostyn et al., 2001) are all possible outcomes of this phenomenon. The field of nonlinear optics has become a Frontier area in the fields of ultrafast pulse measurement (X. Liu et al., 2017), light generation and modulation (J. Chen et al., 2018), optical parametric amplification (Kumbhakar and Kobayashi, 2007; Popov and Shalaev, 2006), laser frequency conversion (D. Hou et al., 2018a), modulators (Grote et al., 2002), and optical switches (Biswal et al., 2019; W. Li et al., 2014). In addition, nonlinear optics have remarkable applications in dynamic image processing (Van Steenwinckel et al., 2001), sensing (Ray, 2010), high resolution imaging (Miller et al., 2002), optical computing (Hutchinson and Milburn, 2004), optical fiber communication (Arivuoli, 2001), optical data storage (Iliopoulos et al., 2010), and materials analysis (Y. R. Shen, 1989). The biological applications in the field of NLO microscopic imaging (Huff et al., 2008; Wang et al., 2009) or photodynamic therapy (Kachynski et al., 2014) are also of great interest. Some major applications of nonlinear optical materials are represented in Figure 1.
Bulk crystals such as potassium titanyl phosphate (KTP) (Stucky et al., 1989), beta-barium borate (β-BaB2O4) (Girisun et al., 2017), and lithium iodate (LiIO3) (Kumar et al., 2010a; Kumar et al., 2010b) are currently used as commercial nonlinear media. NLO materials have covered a vast area of science and technology and many scientists are working on their exploration in various fields of optoelectronics. The increase in the number of publications year after year, approaching almost 570 papers in 2021, is evidence of the considerable efforts in the field of nonlinear optics. A bar graph showing the number of publications for the last 5 years is shown in Figure 2. The current state of technology necessitates a strong demand for on-chip integration of photonic and optoelectronic devices.
FIGURE 2. Number of publications per year related to the theoretical studies on nonlinear optical materials: Data obtained by using keywords “Nonlinear optical, Theoretical” from Scopus as of November 2021.
The history of nonlinear optics can be traced by the work of John Kerr on carbon disulfide (CS2) crystals. He observed the alteration in the refractive index by induced quadratic electric field (E) and this phenomenon is known as the electro-optic Kerr effect (Kerr, 1879). In 1883, a similar but linear electric field effect was also observed in quartz and is now known as Pockel’s effect (Burland, 1994). These nonlinear phenomena were only used in a limited way until the laser was invented in 1960, followed by the discovery of the second harmonic generation in quartz (Maiman, 1960; Franken et al., 1961). Based on these developments, the area of nonlinear optics exploded in the 1960s, with Bloembergen’s pioneering research into the complete range of NLO responses of different materials (Armstrong et al., 1962; Ducuing and Bloembergen, 1964). A. D. Buckingham and co-workers have added a key contribution in developing understanding of NLO processes at the atomic and molecular levels (Buckingham and Orr, 1967).
The basic sources of microscopic hyperpolarizabilities were studied in depth during the 1970s. In 1973, the transmission of stationary NLO pulses in dispersive dielectric fibers was observed by Hasegawa et al. (Buckingham and Orr, 1967). The experimental methodologies for measuring these molecular nonlinearities, and fabricating novel crystals that could be useful in second-harmonic production and optoelectronic modulation have been devised (Gibbs et al., 1976; Mollenauer et al., 1980; Cirilo et al., 2010). In the early 1990s, second and third order NLO responses in atoms and small molecules were calculated and measured in the gas phase by David Shelton and Julia Rice (Shelton and Rice, 1994). David et al. worked on identification of chromophores with higher order optical nonlinearities using a range of electronic structural models and computational approaches (Kanis et al., 1992, 1994). This literature demonstrates that advanced computational methodologies on isolated atoms and molecules might help us to understand the origin of nonlinear processes and to contribute toward the designing tools for forecasting their features. Currently, a majority of the NLO work is focused on the causes, processes, and applications of NLO materials.
Nonlinear optics illustrate the light-matter nonlinear relationship in a medium under the intense light beam (laser) and electromagnetic fields (Becker, 1998). On propagation of light through a medium, the valence electrons produce a charge transfer relative to the atoms in the medium under the action of induced electric field and results in medium polarization (P). The relationship between polarization intensity of medium and electric field strength of the incident light can be expressed as in Equation 1.
Here,
Here,
NLO response is not the property of all systems. In centrosymmetric systems, the induced polarization is the same in all directions and has no NLO response. Therefore, hyperpolarizability is zero in all directions in such systems. While in the case of non-centrosymmetric systems, the restoring force is determined by the charges of displaced electrons. The direction and amount of the applied electromagnetic field will also influence the induced polarization. As a result, materials that can generate an asymmetric wave can also produce an NLO response. Non-centrosymmetry is a basic requirement for a system to show an NLO response (Fan et al., 2010; Luo et al., 2018). All kinds of non-centrosymmetric systems exhibit NLO effects, but in order to obtain their potential applications, they must have a maximum nonlinearity at low power. Therefore, the researchers have focused on designing novel materials with a higher order NLO response.
A revolution in electronics is on the horizon with the uprising of two unique domains including spintronics and molecular electronics. Single-molecule magnets in spintronics can be used to establish a basic link between these two domains (Bogani and Wernsdorfer, 2010). Molecular optics may result by intriguing development in optics since the implementation and development of NLO materials at the molecular level. The NLO molecules can be used to make macroscopical NLO materials (Cheng et al., 1991; Delaire and Nakatani, 2000). Due to the discovery and diverse applications of nonlinear optics, the researchers are focused on improving the NLO response of various inorganic and organic materials by using a variety of strategies. To date, several approaches for tuning the NLO response of materials have been established. These include electron donor to accepter push–pull mechanisms, designing octupolar molecules, introducing diffuse excess electrons, using multi-decker sandwich clusters, applying bond length alteration theory, designing metal organic frameworks (MOFs), and so forth. Some of these are listed here.
The electron push-pull strategy was first introduced by Zyss and his co-workers (Zyss and Ledoux, 1994). This approach is primarily employed for organic chromophores especially stilbenes, porphyrins, paracyclophanes (Vijayakumar et al., 2008; Orbelli Biroli et al., 2011), and so on. The structure of such organic compounds is a major contributor toward the development of NLO response. When the donor-acceptor groups are connected via π-bonding, a conjugated (D-π-A) system is formed. This system is referred to as a push-pull mechanism based system because of the intermolecular charge transfer (ICT) from donor to acceptor (linked through π-bonding), which leads to induction of polarization under the presence of light and electric field. The best example of such NLO chromophores is DANS (4-[N,N-dimethylamino]-4′-nitro stilbene) which shows an intramolecular charge transfer (ICT) (Vijayakumar et al., 2008). It has an electron donor dimethylamino group (-N(CH3)2) and nitro group (-NO2) as electron acceptor with trans-stilbene as π-conjugated bridge as shown in Figure 3. Vijayakumar et al. observed that planar conformations would result in increased NLO activity (435.41 × 10−31 esu), but a departure from planarity results in decreased NLO activity (285.16 × 10−31 esu).
Paracyclophane (pCP) is an important building block for complexes in which D-A substituents interact via a sterically restricted (π-π) stack. In 2000, Zyss et al. observed the experimental and theoretical quadratic hyperpolarizability tensor (60 × 10−30 esu) of dipolar 4-(4-dihexylaminostyryl)-16-(4-nitrostyryl)[2.2]paracyclophane for the first time. The collective electron oscillator (CEO) approach was used to confirm collective nonlinear polarization involving the entire end-to-end molecular structure (Zyss et al., 2000). Since then, paracyclophanes are serving as novel NLO candidates in various fields. Recently, the NLO response of [2.2]paracyclophane based polymers has been investigated theoretically (Ivonina et al., 2020). The NLO properties of a series of diacetylene-functionalized organic molecules (DFOM) with D-π-A stacking have been computed. These complexes were designed by structural tailoring of newly synthesized BDDA (4,4′-(buta-1,3-diyne-1,4-diyl) dianiline) chromophores. The involvement of extended conjugation and charge transfer character have been explored via different DFT methods. The enhanced NLO response (β̥ = 2.5×104 au) of the designed diacetylene-functionalized organic complexes reflected that these complexes could serve as better NLO candidates (Khalid et al., 2019). The development of novel synthetic and theoretical techniques leads to more diverse substitution patterns of porphyrin rings because it widens the potential applicability in nonlinear optics. In this regard, NLO response of a series of substituted zinc(II) porphyrinates with –N(CH3)2 as donors and –NO2 as electron acceptors has been investigated. The experimental and theoretical investigation of a series of 5,15 push−pull meso-diarylzinc(II) porphyrinates with –OCH3 or –N(CH3)2 as donors and –COOH or –COOCH3 as electron acceptors in DMF and chloroform have been explored (Orbelli Biroli et al., 2017). Their NLO response was determined using the electric-field-induced second-harmonic generation technique.
Metal organic frameworks, a fascinating class of hybrid NLO materials, have resulted by altering the central metal atom or adjusting the organic linkers (with different functional groups) as compared to traditional organic and inorganic NLO crystals. According to a recent research on ZIF-8 (zeolitic imidazolate) framework (Van Cleuvenbergen et al., 2016), it is observed that ZIF-8 has a strong SHG signal that is similar to the inorganic crystals of KH2PO4. A theoretical investigation on the structures and exploration of NLO response of experimentally synthesized Zr-based UiO-66 MOFs with BDC (1,4-benzene-dicarboxylate) linkers and its substituents (−NH2, −OH, −SH, and halogen atoms) has been carried out to reveal the effect of ligand functionalization on NLO properties (Ni et al., 2020). Recently, the NLO response of titanium-based MIL-125 MOFs (crystals) has been investigated using DFT calculations. In this MOFs, the BDC linkers have been altered either by extending the BDC ligands or by substituting various functional groups as in MIL-126 and MIL-127. It was observed that the NLO response especially SHG intensity has been improved on introducing substituent groups into the BDC linker (Ni et al., 2019). These findings are helpful for developing novel NLO materials with hi-tech performance.
Diradical character is an efficient approach for the enhancement of NLO response of open-shell singlet systems (Nakano et al., 2004, 2007; Nakano et al., 2006b). The chemical systems with intermediate diradical character exhibit greater NLO response (hyperpolarizabilities) than pure or closed shell diradical systems. The cause of this reliance has been speculated by using the VCI (valence configuration interaction) approach for H2 model (a two-site diradical system) (Nakano et al., 2006b) and a linear H4 model for tetraradical systems (Nakano et al., 2012). Several DFT calculations have been performed to investigate the relationship between hyperpolarizabilities and diradical character (y) of singlet diradical systems such as p-quinodimethane (PQM) molecule (Nakano et al., 2005), polycyclic diphenalenyl radicals (Ohta et al., 2007), graphene nanoflakes (Nakano et al., 2008), and imidazole ring based diradical systems (Nakano et al., 2006a; Qiu et al., 2008). Apart from these studies, a comparison of NLO response of cyclic/linear acenes vs. phenylenes has reflected a distinct enhancement in the tetraradical nature of cyclacenes as well as their second hyperpolarizabilities (γzzzz ≈ 104 au) (Muhammad et al., 2013). These theoretical predictions have been observed in the TPA (two-photon absorption) analysis of experimentally synthesized diphenalenyl diradical compounds (Kamada et al., 2007), hepta/octazethrenes (Li et al., 2012), and benzannulated Chichibabin’s hydrocarbons (Zeng et al., 2012), as well as in the THG (third harmonic generation) effect of BDPI-2Y (1,4-bis-(4,5-diphenylimidazole-2-ylidene)cyclohexa2,5-diene) (Kishida et al., 2010). Muhammad et al. theoretically observed the dependence of NLO response on the diradical character of various fullerenes (C20, C26, C30, C36, C40, C42, C48, C60, and C70). It was observed that C20, C60, and C70 were closed-shell systems while others were intermediate and open-shell systems. The larger second order hyperpolarizabilities were observed for fullerenes with intermediate diradical character such as C40 have shown γzzzz up to 43.44 × 103au. Similarly, the NLO responses of diradical character based various buckyferrocenes have been explored at the molecular level. The closed-shell bucky ferrocenes were (CpFe)2/5C60Me10 and (CpFe)2η5C70Me10, intermediate open-shell singlets were (CpFe)2η5C70Me8 (CpFe)2η5C30, and (CpFe)2η5C70Me4 while others were purely open shell based complexes. The largest third order NLO response (γzzzz = 130.71×104 au) was observed for (CpFe)2η5C70 buckyferrocenes with intermediate diradical character (Muhammad et al., 2015). These findings show that diradical character has enhanced the NLO response of complexes and served as a suitable approach for obtaining high performance NLO materials.
Introduction of excess electrons into various systems is a novel approach that remarkably enhances their NLO properties. The NLO response of organic and inorganic systems can be boosted by excess electrons. In 2004, Li et al. introduced a new potential class of NLO materials with diffuse excess electrons for the first time (Li et al., 2004). They investigated theoretically the nonlinear optical response of (FH)2{e}(HF) clusters with the highest hyperpolarizability of 8.1 × 106 au. Similarly, first hyperpolarizability (β̥ ≈ 107 au) of excess electron bound water trimer anion (H2O)3⎯ was extremely larger than molecular cluster of water trimer (H2O)3 itself (W. Chen et al., 2004). These results indicate that the excess electrons have a potent role in improving the nonlinear optical features. A dispersive orbital is occupied by the diffuse excess electrons, which is in a Rydberg-like state. The nonlinear optical susceptibilities of a Rydberg atom or molecule are extremely high in the gas phase, as evidenced by available experimental data and theoretical studies (Manykin, 2002). The significant impact of excess electrons on clusters’ hyperpolarizability opened new possibilities for the design and preparation of innovative NLO molecular materials. Doping of metal atoms (alkali, alkaline, transition) on complexants is an efficient approach to obtain excess electrons compounds (Ahsan and Ayub, 2020b; Munsif et al., 2018; Rad and Ayub, 2018c; Sun et al., 2017a). Electrides, alkalides, alkaline earthides, coinage metalides, and superalkalides are the various classes of excess electron compounds that show remarkable NLO response. A general representation for alkalide, alkaline earthide, and coinage metalide complexes is shown in Figure 4.
Electrides are a representative type of diffuse excess electron compounds that have a great possibility to serve as NLO materials. They are formed when valance electrons of metal atom are pushed by complexants and become excess electrons in the system. Dye et al. synthesized the first electride Cs+ (18-crown-6)2e− as an excess electron system in 1983 (Ellaboudy et al., 1983; Dawes et al., 1986). In 2005, Chen et al. theoretically investigated the NLO response of (HCN)n-Li and Li-(HCN)n (n = 1, 2, 3) electrides for the first time (W. Chen et al., 2005b). The 2s valence electrons of Li have become excess electrons by the interaction of a Li atom and (HCN)n. Since then, many alkali metal based electride complexes with larger NLO response have been investigated. Electrides include cup-like Li@calix [4]pyrrole (W. Chen et al., 2005b), M@pyrrole (M = Li, Na, and K) (Yu et al., 2011), M+(e@C20F20)− (J.-J. Wang et al., 2012), and (M3O)+(e@C20F20)- and chain-like Lin−H−(CF2−CH2)3−H (n = 1, 2) (H.-L. Xu et al., 2007), and so on. Some of these electrides are listed in Table 1.
Alkalides are novel excess electron compounds obtained when another metal atom is placed on the opposite side of electrides. Complexants' excess electrons are wrapped around a second metal atom to form a metal anion. Due to its low electron affinity, the excess electrons of anionic metal atoms are loosely bound with a dispersive nature. The first alkalide was successfully synthesized by Dye and co-workers in 1974 (Dye et al., 1974; Tehan et al., 1974). Later on, two stable alkalides K+(Me6Aza222)Na− and K+(Me6Aza222)K− were synthesized by the same group which opened up the possibility of alkalide applications (Kim et al., 1999). Theoretical studies of room temperature stable alkalides Li+(calix [4] pyrrole)M− with a greater NLO response opened a fresh approach for developing innovative alkalides (W. Chen et al., 2005a). The alkalide complexes of Li+ (calix [4]pyrrole)K− (W. Chen et al., 2006; Sun et al., 2013) and (M+@n6adz)M′- (n = 2, 3, M, M′ = Li, Na and K) (F.-F. Wang et al., 2008) with larger NLO response have been reported. Similarly, theoretical studies on smaller alkalides Li+(NH3)4M− were explored (Jing et al., 2006). It was observed that the NLO response of smaller alkalides was four times larger than larger organic Li+(calix [4]pyrrole)M− alkalides (Jing et al., 2006). A series of alkaline earth based alkalides M(NH3)6NaCl and M(NH3)6Na2− (M = Mg and Ca), with the highest hyperpolarizability up to 123,050 au have been explored theoretically (W.-M. Sun et al., 2015). Theoretical exploration of the NLO response of a new series of superalkali based alkalides Li3+(calix [4]pyrrole)M–, Li3O+(calix [4]pyrrole)M–, and M3O+(calix [4]pyrrole)K− (M = Li, Na, and K) has been carried out via DFT calculations with the highest first hyperpolarizability up to 34,718 au (W. M. Sun et al., 2014). The superalkali based alkalides are shown in Figure 5.
FIGURE 5. Superalkali based alkalides of Li3O+(calix [4]pyrrole)M− and M3O+(calix [4]pyrrole)K−complexes. Reprinted from W. M. Sun et al., 2014 with permission from Copyright (2014) American Chemical Society.
Similarly, superalkali (Li2F,Li3O, Li4N, and Li3) doped (aza222)K alkalides with enhanced NLO response have been investigated theoretically (W.-M. Sun et al., 2016b). Coinage metal (Cu, Ag, and Au) based alkalides of (M@36adz)M′ have been investigated via DFT calculations and exhibit enhanced NLO responses (W. M. Sun et al., 2017a). A theoretical investigation of Li2F superalkali based alkalides FLi2-M-Li2F (M = Li, Na, and K) has been carried out (Srivastava and Misra, 2015b). The negative charge on the sandwiched alkali metal atoms confirmed their alkalide nature. The mean polarizabilities of these stable superalkali based alkalides are up to 103 au and hence may serve as better NLO candidates. The NLO response of Li3O based alkalides OLi3-M-OLi3 (M = Li, Na, and K) have been observed theoretically for the first time (Srivastava and Misra, 2017). The sandwiched Li, Na, and K metal atoms bear a negative charge donated by excess electrons of superalkalis. The highest NLO response of 1.9 × 104 au is observed for OLi3-K-OLi3 at C2V symmetry.
Recently, a series of novel alkalides M+·1·M′– (M, M′ = Li, Na, K) of a facially polar C6H6F6 molecule (C6H6F6 = 1,all-cis-1,2,3,4,5,6-hexafluorocyclohexane) has been investigated theoretically (Sun et al., 2017b). These alkalides show remarkable NLO response with the highest hyperpolarizability of 1.45 × 106 au. These findings were a tour de force of NLO materials and paved the way to new possibilities for designing stable alkalides. The NLO response (hyperpolarizability) of some excess electron based alkalides are listed in Table 2.
TABLE 2. Nonlinear optical response (hyperpolarizability) of excess electron based alkalide complexes.
Alkaline earthides are novel excess electron compounds in which electron acceptors are alkaline earth metals (alkaline earth anion) in place of alkali metals. They show very large NLO response and have partial p-electrons participation in excess electron formation, unlike alkalides in which partial s-electrons participate. Development of negative charge on alkaline earth metals in the presence of alkali metals is more difficult due to their ns2 (closed shell) electronic configuration and their weak electron affinity. As a result, selecting the right complexant to stabilize the alkali metal cation and alkaline earth metal anion at the same time is critical. One such alkaline earthide organic system Li+ -1-M (1 = C6H6F6 (all-cis-1,2,3,4,5,6-hexafluorocyclohexane) with Be, Mg, and Ca as an electron acceptor has been reported (Hou J. et al., 2018). Sajjad et al. carried out theoretical investigation of thermally stable alkaline earthides of M+-1-M’ (1 = C6H6F6 (all-cis-1,2,3,4,5,6-hexafluorocyclohexane). These complexes have large NLO response with the highest hyperpolarizability up to 4.14 × 109 au (Sajjad et al., 2020).
Ahsan et al. theoretically investigated the NLO response of two series of a novel organic M+(26Adz)M− (M− = Be, Mg and Ca) alkaline earthides. These complexes show remarkable NLO response up to 1.0 × 106 au (Ahsan and Ayub, 2020a). Zhu et al. reported alkaline-earthides of Li(NH3)4M (M = Be, Mg, Ca) based on a cage-like complexant. These complexes have an Li+ cation near the center of the complexant and the alkaline-earth anion is present outside. These alkaline earthides exhibit larger NLO response up to 8.01×106 au and could be considered as novel NLO molecules (Zhu et al., 2019). Another example of alkaline earthide complexes based on hexaammine complexant M+(NH3)6M− (M+ = Li, Na, K; M− = Be, Mg, Ca) that show larger NLO have been investigated by Ahsan et al. (Ahsan and Ayub, 2020b). These complexes have remarkable NLO response up to 6.4 × 105 au and were thermodynamically stable. Recently, the NLO response of superalkali containing alkaline earthides M3O+-1-M’₋ (1 = C6H6F6; M’ = Be, Mg, Ca and M = Li, Na, and K) has been explored. The highest first hyperpolarizability is up to 5.2 × 106 au and shows that designed complexes would have potential applications in optoelectronics (Bano et al., 2022).
Coinage metalides are new representatives of excess electron compounds in which coinage metal atoms [copper (Cu), silver (Ag), and gold (Au)] serve as excess electron acceptors. The ns1 valence-electron configurations and higher electron affinities (EA) were main features of coinage metal atoms than alkali metal atoms. Therefore, it is highly expected that the coinage metal atoms would be used to produce a new type of stable NLO material. In this regard, designing of a series of all-cis-1,2,3,4,5,6-hexafluorocyclohexane based M+-1-M’− (1 = C6H6F6, M’ = Cu, Ag, and Au and M = Li, Na, and K) complexes have been investigated, theoretically. These complexes were thermodynamically stable due to stronger electrostatic interactions. All these coinage metalides possess remarkably enhanced NLO response up to 90,220 au (X.-H. Li et al., 2020a).
The excess electron compounds in which a superalkali bears the anionic site are termed superalkalides. The larger volume of superalkalides than alkalides results in loosely binding of excess electrons to the superalkali core. It results in a more diffuse and readily polarized electron density distribution of complexes. Therefore, superalkalides might be responsible for the enhancement of NLO response. It is more difficult to locate an acceptable complexant to synthesize superalkalides due to the more loosely bounded nature of excess electron in superalkalis. The complexant in superalkalides must be highly resistive toward reduction. In 2015, the designing of the first superalkalides M+(en)3M3’O− (en = ethylenediamine; M, M’ = Li, Na, and K) was carried out by the aid of ab initio calculations. These superalkalides were stabilized by the presence of hydrogen bonds and have remarkable NLO response (Mai et al., 2015). Similarly, designing superalkalides L-M-L-M′3O (L, C6H6F6) has been investigated theoretically (B. Li et al., 2018). It was observed that designed superalkalides L-M-L-M′3O were thermodynamically more stable than their respective alkalides. Moreover, these superalkalides possess larger NLO response up to 1.31 × 106 au for L-Li-L-Li3O complex and would be useful candidates for high performance NLO materials.
Superalkalis in combination with superhalogens result in the formation of supersalts such as Li3O, Cs2NO3, and Cs2Cl (superalkalis) in combination with BeF4, BeF3, and NO3 (superhalogens) constitute supersalts (Giri et al., 2014). Wang et al. theoretically observed superhalides of (Li3)+(SH)− (SH = LiF2, BeF3, and BF4). These superalkali-superhalogen compounds have special characteristics of low vertical ionization energy, delocalized σ bonding orbital on the Li3 ring and are electrides or alkalides in nature (F. Wang et al., 2006). The theoretically calculated bond energies of Li6B-X (X = F, LiF2, BeF3, BF4) supersalts are up to 220.6 kcal/mol with enhanced NLO response ranging from 5166.5 to 17,791 au (Li et al., 2008). Similarly, the supersalts of BF4-M (M = Li, FLi2, OLi3, NLi4) have been investigated theoretically (Yang et al., 2012). The NLO response and binding energies of these ionic compounds are also higher than pure superalkalis.
Many organic and inorganic excess electron based NLO materials have been designed theoretically. Doping is an efficient approach to enhance the NLO response of various systems including cyclacene (Xu et al., 2009), calix [4] pyrrole (W. Chen et al., 2006), organic and inorganic fullerenes (Ahsan et al., 2021; S.; Liu et al., 2019; Shakerzadeh et al., 2016a; Ullah et al., 2020b), phosphorenes, B10H14 baskets (Muhammad et al., 2009), cyclic polyamines (Li et al., 2009a), fluorocarbons (H. L. Xu et al., 2007), and so forth.
Kroto et al. invented fullerene, a third allotropic form of carbon, in 1985 and were awarded the Nobel prize in 1996 for fullerene discovery (Kroto et al., 1985; Lopez et al., 2011). Fullerenes are sp2 hybridized polyhedral carbon cages with resonating π electrons (Haymet, 1985; Haddon et al., 1986). Fullerenes exist in a variety of shapes, including hollow spheres, ellipsoids, and tubes depending on the amount of carbon atoms in them, for example, C20, C60, and C70 (Wakabayashi and Achiba, 1992; Ortíz-Saavedra et al., 1993; Fripertinger, 1996). Bucky balls, also known as spherical fullerene (C60), is a stable carbon cluster with 20 hexagons and 12 pentagons that resemble a soccer ball (Hawkins et al., 1991). It has a hollow sphere like geometry with a diameter of 7Å. It is stabilized by electron resonance and can tolerate extreme temperature and pressure conditions (Chuvilin et al., 2010). Fullerenes have attracted extensive attention in various fields including optoelectronics (Tutt and Kost, 1992), information technology (Senftleben et al., 2009), biomedical (Q. Liu et al., 2014; Markovic and Trajkovic, 2008; Stoilova et al., 2007), etc. In the area of nonlinear optics, various classes of fullerene family such as endohedral fullerenes, exohedral fullerenes, and substituted fullerenes have been explored as in Figure 6.
Exohedral fullerenes are the most versatile fullerenes. They are formed by the doping of atoms to the outside of the fullerene cage. In this regard, LaC60 has been synthesized and theoretically investigated (Rosen and Wästberg, 1989; Rosen and Waestberg, 1988). The exohedral nature of fullerenes was also observed in superconductive alkali fullerides general formula M3C60 (M = K, Rb, Cs) (Erwin and Pickett, 1991; Pauling, 1991; Sparn et al., 1991; Novikov et al., 1992). Endohedral metallofullerenes are formed by inside doping of metal atoms to a fullerene cage. Endohedral metallofullerenes were first predicted in 1985 by the encapsulation of the La+ (lanthanum ion) in C60 (Heath et al., 1985). After the macroscopic manufacture of fullerenes in 1990, rapid research of its unique features became available (Krätschmer et al., 1990a; Krätschmer et al., 1990b). Polar endohedral metallofullerenes Li@C60 have emerged as novel nanomaterials from its first synthesis reported in 1996 (Tellgmann et al., 1996). Later on, two of its salts [Li+@C60]PF6− and [Li+@C60]SbCl6− have been synthesized and were confirmed by crystallographic analysis (Okada et al., 2012). A theoretical investigation on [Li@C60]PF6 endofullerene complex has been carried out and its spectral properties match well with the experimental data (Srivastava et al., 2016a). The endohedral metallofullerene complex of Li@C60-PF6 is resulted by the interaction of Li@C60 with PF6 superhalogen and shows a remarkable NLO response up to 1500 au. Similarly, NLO response of stable Li@C60-BX4 (X = F, Cl, Br) complexes have been explored theoretically (S.-J. Wang et al., 2013). Superalkali (Li2F, Li3O, Li4N) entrapped C60 endofullerenes have been investigated theoretically and a comparison of their electronic properties with Li@C60 has been carried out (Srivastava et al., 2016). These superalkali@C60 endofullerenes are stabilized by charge transfer from superalkali toward C60 fullerenes and have large polarizabilities. Superalkali endofullerenes on interaction with superhalogens SA@C60-X (SA = Li2F, Li3O, Li4N, X = BF4, BCl4, and BBr4) form stable endofullerene complexes (Srivastava et al., 2017). The nonlinear optical response has been computed for these complexes and the highest NLO response up to 3.2 × 104 au for Li2F@C60-BBr4 has been computed. Fullerenes with encapsulated metal atoms have unique features that are not present in pristine fullerenes (A. A. Popov et al., 2013). Endohedral fullerene complexes have prospective applications in MRI contrast agents (Anilkumar et al., 2011; Zhang et al., 2007), radiotracers (Kobayashi et al., 1995), polymeric solar cells (PSCs) (Ross et al., 2009), lasers (Yakigaya et al., 2007), optoelectronics (B. Wu et al., 2015; Lee et al., 2002), and in quantum computers (Benjamin et al., 2006).
Substituted fullerenes, also known as hetero-fullerenes, have one or more carbon atom of fullerene replaced by heteroatoms. The first heterofullerene C59B was synthesized by Gou et al., in 1991 (Guo et al., 1991). Similarly, synthesis and electronic structure of five stable metallocarbohedrenes M8C12 ((M = Ti, Zr, Hf, V) have been achieved by using anion photoelectron spectroscopy (S. Li et al., 1997). Since then, a variety of hybrid or non-carbon fullerene-like structures with varied numbers of substituted metal atoms (Al12N12, B12N12, Al12P12, B12P12 etc.) has also been reported (Akasaka et al., 1999; Billas et al., 2000; Liu et al., 2019; Paul et al., 2017) and is shown in Figure 7.
The replacement of C=C double bonds with X–Y hetero bonds is a viable technique for the development of stable heterofullerenes. The geometric, stability and electronic properties of C20 analogous-heterofullerenes X 4Y4C 12 (X = B, Al, Ga, Si and Y = N, P, As, Ge) have been investigated theoretically (Koohi et al., 2018). The homonuclear non-carbon nanocages may include gold (Gao et al., 2014; Zheng et al., 2018a) and boron nanocages (Z. Wang et al., 2020) while nitrides and phosphides of group III and carbides of group IV are included in heteronuclear non-carbon nanocages (Tahmasebi et al., 2016). The relative stabilities of different sized nanocages have been the subject of several theoretical investigations. Fowler et al. theoretically investigated that B12N12 is the most stable nanocage among B12N12, B16N16, and B28N28 nanoclusters (Seifert et al., 1997). The first synthesis of B12N12 nanocluster was carried out by Oku et al. and was detected by laser desorption time-off-light mass spectrometry (Oku et al., 2004). Wu et al. explored a series of (AlN)n (n = 2–41) nanocages and concluded that Al12N12 inorganic fullerene is the most stable and served as a suitable NLO substrate (H.-S. Wu et al., 2003). Similarly, Beheshtian et al. explored the geometric and electronic properties of Al12N12, B12N12, Al12P12, and B12P12 fullerenes like structure via DFT simulations (Beheshtian et al., 2012). These nanostructures are semiconductor materials with a wide energy gap. Group III nitrides and phosphides with fullerene like properties come in a variety of sizes. The geometrical, electrical, and nonlinear optical properties of these fullerene-like structures were altered on heteroatom introduction. These nanocages can be doped by various electron-based systems as shown in Figure 8.
Introduction of diffuse excess electron is an efficient approach to improve the NLO response of various systems. Alkali metals can donate excess electrons easily due to low ionization potential (Redko et al., 2005). Fullerene like nanostructures of group II-VI and III−V (Al12N12, B12N12, Al12P12, B12P12, Be12O12, Mg12O12, Ca12O12, Si12C12, etc.) are important sources of nanoscale materials due to their potential applications as solar cells, optoelectronics quantum devices, hydrogen storage, photocatalysts, and in biomedicine (Rad and Ayub, 2018a; Zheng et al., 2018b; Ravaei et al., 2019; Zhou et al., 2019). The geometrical, electrical, and optical properties of these nanocages will be altered on the introduction of alkali metal. Niu et al. observed the alkali metal doping at various position of M@x-Al12N12 (x = b66, b64, and r6). Diffuse excess electrons of alkali metals have lowered the energy gap that led to an enhancement in NLO response of Al12N12. The highest β̥ of 8.89 ×105 au has been obtained for Li@r6-Al12N12. It reflects that designed complexes will be applicable in hi-tech NLO materials and electronic nanodevices (Niu et al., 2014). Alkali metal doping at various position (b64, and r6) of Al12N12 nanocage is shown in Figure 9.
FIGURE 9. Alkali metal doping at various positions of M@x-Al12N12 nanocage (x = b64, and r6). Reprinted with permission from Niu et al., 2014. Copyright (2014) American Chemical Society.
Alkali metal doping on Be12O12 and Mg12O12, the most viable clusters of group II-VI, has been reported. Their results show that alkali metal interaction with Be12O12 and Mg12O12 tuned up their structural and optoelectronic properties. A more pronounced NLO response was observed by exohedral doping and was larger than encapsulated ones for these metal oxide nanoclusters (Shakerzdeh et al., 2015). First synthesis of B12N12 nanocage and its detection by laser desorption mass spectrometry by Oku et al. opened new research perspectives in various fields (Oku et al., 2004). Theoretical investigation of alkali metals doped MB12N11 and MB11N12 has been carried out for their potential applications in nonlinear optics. The highest NLO response of 1.3 × 104 au for KB12N11 was obtained (Maria et al., 2016). In another work, structural stability, boundary crossing barriers, and NLO properties of alkali metals encapsulated X12Y12 nanocages (X = B, Al and Y = N, P) have been studied. A correlation existed between the size of alkali metal with adsorption energies of complexes and diameter of nanocages with the distortion energies. It was concluded that the nonlinear optical properties of alkali metal encapsulated phosphide nanocages (B12P12) were two to three times larger than those of corresponding nitride nanocages. The highest NLO response was obtained for K@ B12P12 nanocage, i.e., 5.7 × 105 au due to its very low excitation energy (0.5 eV) (Ayub, 2016). In 2016, Hou et al. studied the structural and NLO response of alkali metal atoms doped B12N12 electrides via DFT simulations. It was observed that HOMO-LUMO energy gap of designed electrides was lowered by the introduction of excess electrons of alkali metals. The highest nonlinear optical response of 18,889 au was observed in B12N12-Na isomer. The decrease in transition energy values in the range of 1.4–2.3 eV has caused the enhancement in NLO response (N. Hou et al., 2016).
Shakerzadeh and co-workers observed the electro-optical properties of Li complexation with B12N12 and B16N16 nanoclusters theoretically. It was concluded that Li complexation with boron nitride nanoclusters has significantly tuned up the nonlinear optical response with the reduction in HOMO–LUMO energy gap. Large NLO response in the range of 2975–130,837 au reflected that the Li complexed boron nitride clusters could serve as innovative electro-optical materials (Shakerzadeh et al., 2016b). The same group observed the optoelectronic effect of alkali metals (Li, Na, and K) encapsulation on B12N12, Al12N12, Ga12N12, C24, Si12C12, and Ge12C12 nanoclusters of group III nitrides and group IV carbides. Nitride nanoclusters show remarkable NLO response than that of corresponding carbides. Optoelectronic properties of B12N12 complexes especially hyperpolarizabilities were remarkably increased to 8505.49 au for Na@B12N12 and 122,503.76 au for K@B12N12 (Tahmasebi et al., 2016).
Alkali metal substituted MX12P11 and MX11P12 (X = B, Al) nanocages were also explored for geometric, electronic, and NLO properties investigation. Diffuse excess electrons of alkali metals (M = Li, Na, and K) have boosted up the NLO response of phosphide nanocages by the decrease in HOMO-LUMO energy gap (Maria et al., 2017). In the same year, Song et al. computed the optoelectronic properties of alkali metal doped C24N24 nanocage by using DFT calculations. Alkali metals were doped outside (M = Li, Na, and K) and inside (M = Na and K) the nanocage at various doping positions. Alkali metal doping altered the electronic properties and orbital energy gap have lowered in both cases in contrast to pristine C24N24. The highest first hyperpolarizabilities obtained for Mout@C24N24 and Mint@C24N24 were 1242 au and 4772 au, respectively (Song et al., 2017). Stable Si12C12 nanocage has been observed theoretically and has many applications in optical communication, optical data storage, laser devices, and optical computing (Pochet et al., 2010; Javan, 2013; Duan and Burggraf, 2015). Theoretical investigation of alkali metals (M = Li, Na, and K) doping on Si12C12 nanocage was carried out. There was a remarkable enhancement in nonlinear optical response of M@Si12C12 complexes as compared to pristine Si12C12 (Solimannejad et al., 2017).
Exploring novel NLO materials is an area of scientific investigation of our recent era. In this regard, Zn12O12 nanocage served as a stable motif with a potential for cluster materials (Reber et al., 2006). In a recent work, endohedral and exohedral doping of alkali metals on Zn12O12 clusters at all possible sites have been carried out. The DFT results manifest that most of M@Zn12O12 complexes were electride in nature and thermodynamically stable. Alkali metal doped Zn12O12 nanocages have prominent features of exothermic encapsulation superior to other inorganic nanocages with endothermic encapsulation. The energy gaps were significantly reduced with the enhancement in NLO response. The highest NLO response of 1.0 × 105 au was observed for K@r6-Zn12O12 complex. Third order NLO response was also computed for the designed M@Zn12O12 electrides (S. Khan et al., 2021b). Similarly, geometric, electronic, and NLO response of endohedral and exohedral alkali metal doped Ca₁₂O₁₂ electrides have been studied. The excess electrons of alkali metals have caused reduction in energy gaps of these complexes. The highest NLO response of the order of 1.0 × 106 au was obtained for endohedral electrides (endo-K@Ca12O12) (Ahsan et al., 2021).
Recently, a DFT investigation of bi-alkali metals (Li2, Na2, K2) doped B12P12 nanocages has proved to be an effective approach. These bi-alkali metals doped complexes were thermodynamically stable with enhanced NLO response of the order of 4.0 × 104 au. Non-covalent interactions and atoms in molecules analyses reflected their bonding phenomenon (Baloach et al., 2021). In other work, bi-alkali metal doped Al12N12 nanoclusters were explored with greater stability. A remarkable enhancement in the NLO response with the reduction in HOMO-LUMO energy gap was observed. The highest hyperpolarizability of 1.2 × 105 au for K2@Ntop-Al12N12 reflected that these bi-alkali metal doped complexes proved as potential candidates in high-tech NLO materials (Sohail et al., 2021). The highest nonlinear optical response of some alkali metal doped nanocages is listed in Table 3.
Alkaline earth metals (Be, Mg, Ca, Sr, Ba, and Ra) are also a good source of excess electrons for complexes. In this regard, alkaline earth metal oxides especially BeO and MgO are wurtzite insulators, with very high thermal conductivity and melting points (Duman et al., 2009). Nonlinear optical response of alkaline earth metals doped Be12O12 and Mg12O12 nanocluster was studied. Excess electrons of alkaline earth metals have profoundly enhanced the NLO response of Be12O12 and Mg12O12 nanocages and their HOMO-LUMO gaps were lowered. The highest hyperpolarizability of the order of 4.72 × 104 au was obtained for endo-Ca@Mg12O12. Hyperpolarizability trend was rationalized by two-level model calculations and βvec was also computed (Kosar et al., 2020a). In another work, alkaline earth metals doping on (XY)12 (X = Al, B, and Y = P) based phosphide nanoclusters of group III has been observed theoretically. Excess electrons of alkaline earth metals have lowered the HOMO-LUMO gaps of designed complexes and enhanced NLO response. The highest hyperpolarizability of the order of 7.8 × 104 au was observed in this case (Ullah et al., 2020b). Similarly alkaline earth metals doping on Al12N12 nanocages was carried out by using DFT calculations. It was observed that designed complexes were thermodynamically stable, and their energy gaps (GH-L) were significantly reduced. These designed complexes have the ability to serve as potential NLO candidates as they have large NLO response of 7769 au as compared to pristine Al12N12 (0 au) (Ullah et al., 2020c). Further exploration of NLO materials by single and multi-doping of odd number alkaline earth metals on C20 fullerenes was carried out. The stable complex Ca5@C20 has the highest hyperpolarizability of 6.5 × 107 au with the energy gap of 1.40 eV. These multi-alkaline earth metals doped complexes would be an ideal choice to obtain novel nanomaterials in optoelectronics (Tahir et al., 2020). In other work, theoretical investigation of Be, Mg, and Ca (even number = 2, 4, 6) doped C20 nanocluster have been carried out. The complexes were thermodynamically stable and the highest binding energy of −241.18 kcalmol-1 was observed for Be6@C20. Their energy gap (GH-L) was effectively reduced for all complexes with the lowest value of 0.82 eV for Ca6@C20 nanocluster. Their NLO response was also enhanced significantly but was lower than odd alkaline earth metal doped C20 (Kosar et al., 2021b). Single and multi-alkaline earth metal atoms doping on C24 fullerene have been carried out. The Be4@C24 has the highest stabilization energy of −182.87 kcal mol−1 which reflected that designed complexes are thermodynamically stable. The lowest energy gap (GH-L) of 0.83 eV for Mg4@C24 has reflected its conductive nature. Newly generated HOMO orbitals (due to excess electrons) have dramatically enhanced the NLO response up to 3.62 × 106 au in the case of Mg4@C24. These results have paved the way to synthesize highly efficient optoelectronic materials (Kosar et al., 2021c).
DFT investigation of second row transition metal atoms (zirconium (Zr), ruthenium (Ru), molybdenum (Mo), and palladium (Pd)) substituted C20 fullerene was carried out. The results manifest that charge distribution of C20 was altered by substitution. The HOMO-LUMO energy gap was lowered in the case of Zr and Mo atoms while it was increased on Ru and Pd substitution. Their nonlinear optical properties including polarizability and hyperpolarizabilities were improved. Their first hyperpolarizabilities were 2795, 2775, 1304, and 66,669 au for Zr, Mo, Ru, and Pd substituted C20 nanocluster (Rad and Ayub, 2017). Scandium (Sc) doped Be12O12, Mg12O12, and Ca12O12 nanocages were explored to study the effect of Sc doping on structure, stability, and optoelectronic properties. Reduction in energy gaps of designed complexes has enhanced conductance of Sc doped complexes. NLO response of all designed complexes was increased and the highest NLO was obtained for Sc-doped Ca12O12. TD-DFT calculations also confirmed that the highest hyperpolarizability was observed for Sc-doped Ca12O12 nanoclusters with the smallest transition energy values (ΔE). Sc-doped nanoclusters would be an ideal candidate for optoelectronic devices (Omidi et al., 2017). Exohedral doping of the first row transition metal doping at various sites (x = b64, b66, r6, and r4) of Al12N12 nanocage was carried out. The geometric, electronic, and optoelectronic properties were computed for all M@x-Al12N12 complexes and their spin state stability was also computed. Transition metal doping resulted in chemisorption and a remarkable reduction in energy gap up to 40% were observed in this case. Their NLO response was comparable to their alkali metal doped Al12N12 analogs and the highest NLO response of 1.85 × 104 au was observed for Cu@r6-Al12N12 (Arshad et al., 2018).
Gilani et al. have carried out exohedral and endohedral doping of copper on Al12N12 nanocages. It was concluded that the Cu@r6-Al12N12 (copper at the center of the six-membered ring of Al12N12) has the highest reduction in energy gap (Eg) up to 52%. Excess electrons have generated a new HOMO orbital between the original Frontier molecular orbitals as revealed from total and partial densities of states. The highest NLO response of 1.8 × 104 au was observed for Cu@r6-Al12N12 as in the case of previous studies. TD-DFT simulations were performed to obtain crucial excited states. Two level model-based verification of hyperpolarizability was also carried out. These striking results reflect that the designed copper doped complexes would have potential applications in nonlinear optics (Gilani et al., 2018). Ali et al. have explored the exohedral doping of nickel metal (Ni) on inorganic Mg12O12 nanocluster by using DFT simulations. Different energy changes including zero-point energies (ZPE), thermal enthalpies (ΔH), thermal energies (ΔE), and thermal Gibbs free energies (ΔG) were also computed and compared with interaction energies of Ni@Mg12O12 nanocluster. A remarkably enhanced nonlinear optical response on doping was observed as compared to pristine Mg12O12 (Rad and Ayub, 2018b). In another work, Rad et al. carried out substitutional doping of transition metal (Cr, Ni, and Ti) at C20 fullerene theoretically. Transition metals substitution has altered the charge distribution of C20 with the highest charge distribution for TiC19. The nonlinear optical properties were enhanced significantly and the highest hyperpolarizability 2.5 × 103 au observed in the case of TiC19 complex (Rad and Ayub, 2018c).
In 2019, the same group also performed the substitutional doping of transition metal (Sc, Fe, Cu, and Zn) at C20 fullerene via DFT simulations. Replacement of carbon by a transition metal has enhanced the optoelectronic properties and the highest hyperpolarizability of 2224.5 au was observed for Sc–C19. Furthermore, the results of reactivity descriptors reflected iron-substituted fullerene (Fe–C19) as the softest metal. These results showed that designed transition metal substituted fullerene would have promising applications in optoelectronics (Rad and Ayub, 2019). The optoelectronic effect of the first row transition metal doping C24N24 cavernous nitride fullerene has been performed via DFT simulation. Transition metal atoms were doped in the N4 cavity of the nanocage. Spin state analysis reflected that spin state stability increases to sextet up to Mn@C24N24 and then gradually decreases to singlet for Zn@C24N24 system. Transition metal atoms have decreased the HOMO-LUMO energy gap up to 63%. The NLO response was enhanced for all M@C24N24 cavernous nitride complexes and reflected that designed complexes would be better candidates for high performance NLO materials (Shakerzadeh et al., 2020a). The first experimental synthesis of novel all-boron fullerene B40 has opened new research avenues for its potential application in various fields (Zhai et al., 2014).
Optoelectronic properties of the first row transition metal decorated at hexagonal and heptagonal rings of B40 fullerene have been computed. Obtained complexes were stable with the reduced HOMO-LUMO energy gaps and work functions. The highest hyperpolarizability of 1.35 × 105 au was observed for cobalt (Co) doped B40 fullerene (Shakerzadeh et al., 2020b). Ullah et al. investigated the structural and optoelectronic effect of first row transition metals decorated Al12P12 heterofullerene. Thermally stable M@Al12P12 complexes have reduced energy gaps due to excess electrons of transition metal atoms. Nonlinear optical properties were enhanced and the highest hyperpolarizability up to 5.9 × 106 au was obtained (Ullah et al., 2021). Similarly, optoelectronic properties of transition metal doped B12P12 nanocage at different doping sites have been explored via DFT simulations. Adsorption of the first row transition metals at B12P12 have significantly enhanced the thermodynamic stability with the highest interaction energy of −74.42 kcal mol-1 for Ni@r4-B12P12 nanocluster. Their NLO response has improved remarkably up to 4.4 × 104 au due to excess electron of transition metals. Furthermore, SHG and EOPE values of 1.1 × 105 and 4.4 × 105 au for Sc@B12P12 showed that designed M@ B12P12 have a tendency to serve as NLO materials (Irshad et al., 2021). Recently, Khaliq et al. investigated the NLO properties of lanthanum metal doping on Al12N12 and Al12P12 nanoclusters. Interaction energy analysis indicated that designed complexes were stable and exohedral doping was more favorable than endohedral doping. Lanthanum doping has uplifted the NLO responses of complexes up to 4.43 × 104 au and will serve as a high-tech NLO material in optoelectronics (Khaliq et al., 2021a). The highest nonlinear optical response of some alkali metal doped nanocages is listed in Table 4.
Superalkalis have low ionization potential than alkali metals and have a better tendency to donate excess electrons toward the system (Gutsev and Boldyrev, 1982). This enables them to form a wide range of unique molecules with intriguing features while the structural integrity of superalkalis remains intact on interaction with other atoms. In this regard, superalkalis (Li2F, Li3O, and Li4N) (Rehm et al., 1992), hyperlithiated superalkali clusters LinF (n = 2–5) (Srivastava and Misra, 2015c), and hypervalent superalkalis M2X (M = Li, Na; X = F, Cl) (Srivastava and Misra, 2016c) served as potential superalkali subunits. These superalkali clusters on interaction with metal atoms resulted in the formation of hyperalkalized superalkali-metal (SA-M) species (Srivastava and Misra, 2016b). These species possess remarkable NLO response and the highest NLO up to 3.6 × 104 au is observed for NLi4-Na. The gas phase basicities of superalkali hydroxides FLinOH (n = 2–5) have been computed theoretically and are comparable to those of strong alkali hydroxides such as LiOH and some of them may act as superbases (Srivastava and Misra, 2015a). These superalkali hydroxides have several unusual and intriguing features, one of which is their enhanced NLO responses. The hydrogenated superalkalis (SAHs = FLi2H, OLi3H, and NLi4H) with enhanced NLO response have also been investigated theoretically (Srivastava and Misra, 2016a).
These superalkali clusters donate their excess electrons on interaction with nanocages to form high performance NLO materials. In 2016, Sun et al. carried out theoretical investigation of superalkali M3O (M = Li, Na, K) doping on Al12N12 nanocage for the first time (W. M. Sun et al., 2016a). Diffuse excess electrons of superalkalis have enhanced the hyperpolarizability of complexes up to 1.86 × 107 au. Moreover, NLO response of Li3O superalkali on other nanocages has been investigated systematically. These electrides have high deep ultraviolet transparency and larger NLO response. These superalkali doped nanocages would be potential precursors for high-performance NLO materials as shown in Figure 10.
FIGURE 10. Superalkali Li3O doped Al12N12 nanocage with NLO response and UV-visible spectrum. Reprinted from W. M. Sun et al., 2016a with permission of Royal Society of Chemistry.
The optoelectronic properties of superalkali MnO (M = Li, Na, K; n = 2, 3, 4) doped Mg12O12 nanocages have been investigated theoretically. Superalkali excess electrons have reduced the energy gap and remarkable increment in the NLO response was observed for M3O superalkali doped nanocluster series. These complexes were potential candidates in the field of nonlinear optics with the highest hyperpolarizability of β̥ ≈ 600,000 au for K3O@Mg12O12 complex (Hesari et al., 2016). In another work, Li3O, Na3O, and K3O doped Si12C12 nanocages have been explored via DFT simulations. It was observed that energy gap (HOMO-LUMO) was reduced monotonically with the size of superalkali subunits. Doping of superalkalis Li3O@Si12C12 (3.750 eV), Na3O@Si12C12 (2.984 eV), and K3O@Si12C12 (2.634 eV) has transformed the pristine Si12C12 nanocage (5.452 eV) to n-type semiconductors. A reduction in HOMO-LUMO gap has strikingly enhanced the NLO response with the highest hyperpolarizability of 21,695 au for K3O@Si12C12. These superalkali doped Si12C12 nanocages would have a major role in optoelectronic devices due to their semiconducting nature (Lin et al., 2017). Nonlinear optical properties of superalkali doped C20 fullerene like nanocluster have been investigated theoretically. A decrement in energy gap was observed and indicated that superalkalis have enhanced the optoelectronic properties of C20 fullerene. A large NLO response was observed with the highest hyperpolarizability for Na3O@C20 nanocluster (Noormohammadbeigi and Shamlouei, 2018). Inorganic boron-based nanosystem of all-boron fullerene B40 has also been doped with superalkali subunits (M3O)n (M = Li and K, n = 1 and 2) to investigate the geometrical, stability, and optoelectronic properties. Excess electrons of superalkalis have significantly enhanced the NLO response and narrowed the HOMO-LUMO energy gap (0.61–1.11 eV) as compared to pristine B40 (2.86 eV). The increment in the first hyperpolarizability and second hyperpolarizability was up to 2.46 × 105 and 4.85 × 108 au, respectively. These complexes were structurally stable due to formation of B-O bond and have binding energies in the range of 57.0–99.8 kcal/mol. Their fascinating results reflect that superalkali doped B40 nanocage would be beneficial for modern electronic nanodevices (Noormohammadbeigi and Shamlouei, 2018).
In 2019, Ullah et al. carried out three superalkalis doping on Li2F, Li3O, and Li4N on fullerene like B12P12 inorganic nanocage to investigate the NLO response of designed complexes. Doping at various sites resulted in different isomers with excess electrons and were thermodynamically stable. Nonlinear optical response of complexes has been enhanced with the highest hyperpolarizability of 3.48 × 105 au. These superalkali doped B12P12 nanoclusters have deep ultraviolet transparency and would have potential applications in high-performance NLO devices (Ullah et al., 2019a). In the same year, theoretical investigation of Li2F, Li3O, and Li4N doping on B12P12 nanocage have been carried out to observe their geometric, electronic, and NLO properties. All the designed complexes were electride in nature due to diffuse excess electrons of superalkali subunits. These complexes were highly stable as indicated by their interaction energies (−105.13 kcal mol−1). These stable inorganic electrides have narrowed HOMO-LUMO energy gap with an increment in NLO response up to 6.25 × 104 au for Li4N@Al12P12 (Ullah et al., 2019b). Ullah et al. also explored the superalkali (Li2F, Li3O, and Li4N) doping on Si12C12 nanocage at various positions via DFT calculations. Superalkali doping has altered the electronic properties of Si12C12 from insulator to n-type semiconductor. These complexes have static first hyperpolarizability up to 19,864 au. Furthermore, frequency dependent hyperpolarizability calculations were also performed in the region 400–1600 nm to check their practical applicability. The designed complexes were transparent in deep UV regions and would be applicable in the deep UV transparent NLO materials (Ullah et al., 2020d). In a recent work, Li3O, Na3O, and K3O superalkalis doping at various doping sites of Zn12O12 nanocage have been carried out theoretically. Excess electrons of superalkalis have enhanced the NLO response of Zn12O12 nanocluster and were UV transparent under workable laser conditions. The NLO increased monotonically and the highest NLO response of 394,217 au was observed for K3O@Zn12O12. These n-type semiconductor complexes have reduced the energy gap up to 70% as compared to pristine Zn12O12. These designed complexes will be applicable in the high performance NLO materials (Kosar et al., 2020b).
A theoretical investigation of superalkali doping on structural and optoelectronic properties of C24 fullerene has been carried out via DFT simulations. The density of states analysis was carried out to confirm the alteration in HOMO-LUMO energy gap on superalkalis doping. The excess electrons of superalkalis have remarkably enhanced the NLO response especially polarizability and hyperpolarizability of C24 fullerene-based nanostructures (Omidi et al., 2020). Li3O super alkali doping at various positions of B12N12 reflected that designed complexes were thermodynamically stable and chemisorbed. A remarkable reduction in HOMO-LUMO energy gap (0.42–3.94 eV) has transferred the designed complexes to n-type semiconductor as compared to pristine insulator nanocage (6.84 eV). Nonlinear optical response of superalkali doped B12N12 nanocluster was increased from 1045 au to 126,261 au. These complexes were transparent in the UV region as reflected by TD-DFT investigations and would have practical applications as optoelectronic materials (Raza Ayub et al., 2020). In a recent work, Li4N and Li4O doped Al12N12 nanoclusters have been explored for their NLO properties (Khaliq et al., 2021b). These Li4N and Li4O based Al12N12 nanoclusters were thermodynamically stable and have deep UV transparency. Smaller excitation energies of 1.27–2.73 eV have a major role in tuning up the NLO response of designed complexes. A remarkable enhancement in NLO response with the highest hyperpolarizability of 5.7 × 104 au was achieved for Li4N@Al12N12 isomer (Figure 11). These Li4N and Li4O based Al12N12 nanoclusters would be potential precursors in designing high-performance NLO materials.
FIGURE 11. Li4N@Al12N12 complex with the highest hyperpolarizability of 5.7 × 104 au. Reprinted from Khaliq et al., 2021b with permission from Elsevier.
Besides inorganic nanoclusters, superalkali doping on many other organic clusters has been carried out for their potential applications in nonlinear optics (X. Li and Li, 2019) (P. Khan et al., 2021a). Organic NLO materials have extended π conjugation, high laser damage thresholds, and smaller dielectric constants (Kang et al., 2019). Most of these organic NLO materials are electrides, alkalides, alkaline earthides, coinage metalides, and superalkalides and have been described in the above sections. However, some other superalkali doped organic NLO materials have attracted much attention in recent years. In this regard, the NLO response of M3O and M3S (M = Li, Na, and K), single and double superalkali M3O doped graphdiyne nanoclusters have been explored (N. Hou et al., 2021; Kosar et al., 2020b; X. Li et al., 2020b). Similarly, Shehzadi et al. observed a remarkable increase in the NLO response of superalkali doped graphdiyne nanoclusters (M2X@GDY X = F, Cl, Br and M = Li, Na, K) with the highest response (7.7 × 104 au) for Na2F@GDY (Shehzadi et al., 2019). Such novel carbon material graphynes (GYs) on combination with superalkalis results in the formation of stable complexes. These GY superalkali complexes OM3+@(GY/GDY/GTY)− possess exceptionally large NLO response of 6.5 × 105 au for Li3O@GTY (GTY = graphtrigne). The NLO properties of doping of alkali metal atoms and superalkali at pyridinic vacancy graphene (3NG) have been studied theoretically. The highest NLO response of 1.6 × 104 was observed for K3O@3NG (Song et al., 2018b). A theoretical exploration of superalkali doped boron substituted biphenalenyl diradical dimer (BC25H18) was carried out by Song et al. and the highest NLO response was for K3O@BC25H18 (Song et al., 2018a). Superalkali doping on cyclic conjugated ring systems especially cyclic oligofuran (CF) and hexathiophene ring (6CT) has been carried out theoretically to evaluate their NLO response (Sajid et al., 2020; Sajid et al., 2021). The highest NLO response of some organic complexes is listed in Table 5.
The corroboration between theoretical and experimental data is useful for designing and synthesis of novel NLO materials. In this regard, various NLO systems have been synthesized and their NLO responses have been investigated theoretically and experimentally. The linear and NLO susceptibilities of single crystals of potassium titanyl phosphate KTiOPO4 have been studied experimentally and theoretically (Reshak et al., 2010). Using DFT calculations, the theoretically predicted band gap (3.1 eV) of KTiOPO4 shows excellent agreement with the experimental data (3.2 eV) at WIEN2K code. Furthermore, the computed second harmonic generation (SHG) susceptibility coefficients are matched with single crystal SHG response. The experimental and theoretical NLO studies of synthesized LiBaB9O15 crystals have been carried out (Reshak et al., 2013). The electronic properties especially band gap energies (5.11 eV) by using DFT simulations match well with the experimental values (5.17 eV). The calculated second order NLO susceptibility especially second harmonic generation (SHG) was β333 = 0.291 × 10−30 esu at 1064 nm. This computed value correlated with the experimental one (0.467 × 10−30 esu) at 1064 nm by using Nd:YAG laser. Single crystals of LiNaB4O7 have been synthesized and confirmed by X-ray crystallography. The results of DFT calculations for electronic properties by using LDA, GGA, EVGGA, and mBJ methods demonstrate that these crystals have a substantial band gap of about 2.80, 2.91, 3.21, and 3.81 eV, respectively. These results match well with the experimental band gap of 3.88 eV. The calculated absorption spectrum of LiNaB4O7 crystals also matched well with the experimental one. The second order hyperpolarizabilities of these crystals were 0.306 × 10−30 esu (static) and 0.332 × 10−30 esu at 1064 nm (Reshak et al., 2012). The first and second hyperpolarizabilities of substituted dimesityl boranes have been explored both theoretically (AM1 semi-empirical level, MOPAC program) and experimentally (at 1907 nm under Nd-YAG laser). The theoretical results were in concurrence with the experimentally measured data for these complexes (Yuan et al., 2006). The nonlinear optical crystals of strontium borate Sr2B5O9(OH)H2O have been synthesized. The DFT calculations of SHG response, nonlinear refractive index, and birefringence computed by using CASTEP package were consistent with experimentally measured values (Zhang et al., 2015). These studies show that theoretical strategies for calculating NLO response show a good agreement with the available experimental data. Therefore, theoretically predicted high performance NLO materials could be a good starting point for the experimentalists.
The current review establishes a systematic array of different studies for the theoretical designing of NLO materials. NLO materials have covered a large area of science and technology owning to their potential applications in optoelectronics. In material research, computational design and experimental synthesis of NLO materials with higher order nonlinearities come into vogue. Several strategies including metal ligand framework, push pull mechanism, diradical character, and so on have been elaborated for the enhancement of NLO response of materials. Role of diffuse excess electron in developing noncentrosymmetric materials for nonlinear optics have been discussed. A theoretical design from the simplest NLO material to the complex alkali, alkaline earth, transition, and superalkali doped nanoclusters is summarized in the present review. Excess electrons of the nanoclusters have played a pivotal role in enhancing the NLO properties of various systems especially hyperpolarizabilities. These excess electron-based systems may help to increase the second hormonic generation (SHG) efficiency and electro-optical Pockels effect (EOPE). These insights are essential for the development of new NLO materials. These findings also provide an intriguing area for future research into potential building blocks and structure-directing constituents that may help to promote the synthesis of these theoretically designed NLO materials. It is expected that the present review will provide a better understanding of the NLO responses of nanoclusters, paving the way for the development of high-tech NLO materials.
K.A., M.G., and T.M. contributed to the design and outlines of the review. R.B. and M.A. collected the literature and wrote the first draft. R.Z. revised the first draft and corrected its language. J.I. and S.T. wrote some sections of the review. All authors contributed to manuscript revision, read, and approved the submitted version.
This project was financially supported by the Higher Education Commission, Pakistan under HEC-NRPU project (7853).
The authors declare that the research was conducted in the absence of any commercial or financial relationships that could be construed as a potential conflict of interest.
All claims expressed in this article are solely those of the authors and do not necessarily represent those of their affiliated organizations, or those of the publisher, the editors, and the reviewers. Any product that may be evaluated in this article, or claim that may be made by its manufacturer, is not guaranteed or endorsed by the publisher.
Ahsan, A., and Ayub, K. (2020a). Adamanzane Based Alkaline Earthides with Excellent Nonlinear Optical Response and Ultraviolet Transparency. Opt. Laser Tech. 129 (April), 106298. doi:10.1016/j.optlastec.2020.106298
Ahsan, A., and Ayub, K. (2020b). Extremely Large Nonlinear Optical Response and Excellent Electronic Stability of True Alkaline Earthides Based on Hexaammine Complexant. J. Mol. Liquids 297, 111899. doi:10.1016/j.molliq.2019.111899
Ahsan, A., Khan, S., Gilani, M. A., and Ayub, K. (2021). Endohedral Metallofullerene Electrides of Ca12O12 with Remarkable Nonlinear Optical Response. RSC Adv. 11 (3), 1569–1580. doi:10.1039/d0ra08571e
Akasaka, T., Okubo, S., Wakahara, T., Yamamoto, K., Kobayashi, K., Nagase, S., et al. (1999). Endohedrally Metal-Doped Heterofullerenes: La@C81N and La2@C79N. Chem. Lett. 28 (9), 945–946. doi:10.1246/cl.1999.945
Anilkumar, P., Lu, F., Cao, L., G. Luo, P. P., Liu, J.-H., Sahu, S., et al. (2011). Fullerenes for Applications in Biology and Medicine. Cmc 18 (14), 2045–2059. doi:10.2174/092986711795656225
Arivuoli, D. (2001). Fundamentals of Nonlinear Optical Materials. Pramana - J. Phys. 57 (5–6), 871–883. doi:10.1007/s12043-001-0004-1
Armstrong, J. A., Bloembergen, N., Ducuing, J., and Pershan, P. S. (1962). Interactions between Light Waves in a Nonlinear Dielectric. Phys. Rev. 127 (6), 1918–1939. doi:10.1103/physrev.127.1918
Arshad, Y., Khan, S., Hashmi, M. A., and Ayub, K. (2018). Transition Metal Doping: a New and Effective Approach for Remarkably High Nonlinear Optical Response in Aluminum Nitride Nanocages. New J. Chem. 42 (9), 6976–6989. doi:10.1039/c7nj04971d
Ayub, K. (2016). Are Phosphide Nano-Cages Better Than Nitride Nano-Cages? A Kinetic, Thermodynamic and Non-linear Optical Properties Study of Alkali Metal Encapsulated X12Y12 Nano-Cages. J. Mater. Chem. C 4 (46), 10919–10934. doi:10.1039/c6tc04456e
Baloach, R., Ayub, K., Mahmood, T., Asif, A., Tabassum, S., and Gilani, M. A. (2021). A New Strategy of Bi-alkali Metal Doping to Design Boron Phosphide Nanocages of High Nonlinear Optical Response with Better Thermodynamic Stability. J. Inorg. Organomet. Polym. 31 (7), 3062–3076. doi:10.1007/s10904-021-02000-6
Bano, R., Arshad, M., Mahmood, T., Ayub, K., Sharif, A., Perveen, S., et al. (2022). Face Specific Doping of Janus All-Cis-1,2,3,4,5,6-Hexafluorocyclohexane with Superalkalis and Alkaline Earth Metals Leads to Enhanced Static and Dynamic NLO Responses. J. Phys. Chem. Sol. 160, 110361. doi:10.1016/j.jpcs.2021.110361
Becker, P. (1998). Borate Materials in Nonlinear Optics. Adv. Mater. 10 (13), 979–992. doi:10.1002/(sici)1521-4095(199809)10:13<979:aid-adma979>3.0.co;2-n
Beheshtian, J., Bagheri, Z., Kamfiroozi, M., and Ahmadi, A. (2012). A Comparative Study on the B12N12, Al12N12, B12P12 and Al12P12 Fullerene-like Cages. J. Mol. Model. 18 (6), 2653–2658. doi:10.1007/s00894-011-1286-y
Benjamin, S. C., Ardavan, A., Briggs, G. A. D., Britz, D. A., Gunlycke, D., Jefferson, J., et al. (2006). Towards a Fullerene-Based Quantum Computer. J. Phys. Condens. Matter 18 (21), S867–S883. doi:10.1088/0953-8984/18/21/s12
Bezi Javan, M. (2013). Optical Properties of SiC Nanocages: Ab Initio Study. Appl. Phys. A. 113 (1), 105–113. doi:10.1007/s00339-013-7633-3
Billas, I. M. L., Massobrio, C., Boero, M., Parrinello, M., Branz, W., Tast, F., et al. (2000). First Principles Calculations of Iron-Doped Heterofullerenes. Comput. Mater. Sci. 17 (2–4), 191–195. doi:10.1016/s0927-0256(00)00022-7
Biswal, B. P., Valligatla, S., Wang, M., Banerjee, T., Saad, N. A., Mariserla, B. M. K., et al. (2019). Nonlinear Optical Switching in Regioregular Porphyrin Covalent Organic Frameworks. Angew. Chem. 131, 6970–6974. doi:10.1002/ange.201814412
Bogani, L., and Wernsdorfer, W. (2010). “Molecular Spintronics Using Single-Molecule Magnets,” in Nanoscience and Technology: A Collection of Reviews from Nature Journals (Singapore: World Scientific), 194–201.
Buckingham, A. D., and Orr, B. J. (1967). Molecular Hyperpolarisabilities. Q. Rev. Chem. Soc. 21 (2), 195–212. doi:10.1039/qr9672100195
Burland, D. (1994). Optical Nonlinearities in Chemistry: Introduction. Chem. Rev. 94 (1), 1–2. doi:10.1021/cr00025a600
Chen, J., Krasavin, A., Ginzburg, P., Zayats, A. V., Pullerits, T., and Karki, K. J. (2018). Evidence of High-Order Nonlinearities in Supercontinuum white-light Generation from a Gold Nanofilm. ACS Photon. 5 (5), 1927–1932. doi:10.1021/acsphotonics.7b01125
Chen, W., Li, Z.-R., Wu, D., Gu, F.-L., Hao, X.-Y., Wang, B.-Q., et al. (2004). The Static Polarizability and First Hyperpolarizability of the Water Trimer anion:Ab Initiostudy. J. Chem. Phys. 121 (21), 10489–10494. doi:10.1063/1.1811609
Chen, W., Li, Z.-R., Wu, D., Li, R.-Y., and Sun, C.-C. (2005b). Theoretical Investigation of the Large Nonlinear Optical Properties of (HCN)n Clusters with Li Atom. J. Phys. Chem. B 109 (1), 601–608. doi:10.1021/jp0480394
Chen, W., Li, Z.-R., Wu, D., Li, Y., Sun, C.-C., Gu, F. L., et al. (2006). Nonlinear Optical Properties of Alkalides Li+(calix[4]pyrrole)M-(M = Li, Na, and K): Alkali Anion Atomic Number Dependence. J. Am. Chem. Soc. 128 (4), 1072–1073. doi:10.1021/ja056314+
Chen, W., Li, Z.-R., Wu, D., Li, Y., Sun, C.-C., and Gu, F. L. (2005a). The Structure and the Large Nonlinear Optical Properties of Li@Calix[4]pyrrole. J. Am. Chem. Soc. 127 (31), 10977–10981. doi:10.1021/ja050601w
Cheng, L. T., Tam, W., Stevenson, S. H., Meredith, G. R., Rikken, G., and Marder, S. R. (1991). Experimental Investigations of Organic Molecular Nonlinear Optical Polarizabilities. 1. Methods and Results on Benzene and Stilbene Derivatives. J. Phys. Chem. 95 (26), 10631–10643. doi:10.1021/j100179a026
Chuvilin, A., Kaiser, U., Bichoutskaia, E., Besley, N. A., and Khlobystov, A. N. (2010). Direct Transformation of Graphene to Fullerene. Nat. Chem 2 (6), 450–453. doi:10.1038/nchem.644
Cirilo, E. R., Natti, P. L., Romeiro, N. M. L., Natti, E. R. T., and de Oliveira, C. F. (2010). Solitons in Ideal Optical Fibers-A Numerical Development. Semina: Ciências Exatas e Tecnológicas 31, 57–68. doi:10.5433/1679-0375.2010v31n1p57
Dawes, S. B., Ward, D. L., Huang, R. H., and Dye, J. L. (1986). First Electride crystal Structure. J. Am. Chem. Soc. 108 (12), 3534–3535. doi:10.1021/ja00272a073
Delaire, J. A., and Nakatani, K. (2000). Linear and Nonlinear Optical Properties of Photochromic Molecules and Materials. Chem. Rev. 100 (5), 1817–1846. doi:10.1021/cr980078m
Duan, X. F., and Burggraf, L. W. (2015). Theoretical Investigation of Stabilities and Optical Properties of Si12C12 Clusters. J. Chem. Phys. 142 (3), 034303. doi:10.1063/1.4905542
Ducuing, J., and Bloembergen, N. (1964). Statistical Fluctuations in Nonlinear Optical Processes. Phys. Rev. 133 (6A), A1493–A1502. doi:10.1103/physrev.133.a1493
Duman, S., Sütlü, A., Bağcı, S., Tütüncü, H. M., and Srivastava, G. P. (2009). Structural, Elastic, Electronic, and Phonon Properties of Zinc-Blende and Wurtzite BeO. J. Appl. Phys. 105 (3), 033719. doi:10.1063/1.3075814
Dye, J. L., Ceraso, J. M., Lok, M., Barnett, B. L., and Tehan, F. J. (1974). Crystalline Salt of the Sodium Anion (Na-). J. Am. Chem. Soc. 96 (2), 608–609. doi:10.1021/ja00809a060
Ellaboudy, A., Dye, J. L., and Smith, P. B. (1983). Cesium 18-crown-6 Compounds. A Crystalline Ceside and a Crystalline Electride. J. Am. Chem. Soc. 105 (21), 6490–6491. doi:10.1021/ja00359a022
Erwin, S. C., and Pickett, W. E. (1991). Theoretical Fermi-Surface Properties and Superconducting Parameters for K 3 C 60. Science 254 (5033), 842–845. doi:10.1126/science.254.5033.842
Fan, X., Pan, S., Hou, X., Tian, X., Han, J., Haag, J., et al. (2010). Growth and Properties of Single Crystals of Noncentrosymmetric Na3VO2B6O11. Cryst. Growth Des. 10 (1), 252–256. doi:10.1021/cg900877h
Franken, P. A., Hill, A. E., Peters, C. W., and Weinreich, G. (1961). Generation of Optical Harmonics. Phys. Rev. Lett. 7 (4), 118–119. doi:10.1103/physrevlett.7.118
Fripertinger, H. (1996). The Cycle index of the Symmetry Group of the Fullerene C60. MATCH Commun. Math. Comput. Chem. 33, 121–138.
Gao, Y., Dai, X., Kang, S. G., Jimenez-Cruz, C. A., Xin, M., Meng, Y., et al. (2014). Structural and Electronic Properties of Uranium-Encapsulated Au₁₄ Cage. Sci. Rep. 4 (1), 5862–5866. doi:10.1038/srep05862
Gibbs, H. M., McCall, S. L., and Venkatesan, T. N. C. (1976). Differential Gain and Bistability Using a Sodium-Filled Fabry-Perot Interferometer. Phys. Rev. Lett. 36 (19), 1135–1138. doi:10.1103/physrevlett.36.1135
Gilani, M. A., Tabassum, S., Gul, U., Mahmood, T., Alharthi, A. I., Alotaibi, M. A., et al. (2018). Copper-doped Al12N12 Nano-Cages: Potential Candidates for Nonlinear Optical Materials. Appl. Phys. A. 124 (1), 1–14. doi:10.1007/s00339-017-1425-0
Giri, S., Behera, S., and Jena, P. (2014). Superalkalis and Superhalogens as Building Blocks of Supersalts. J. Phys. Chem. A. 118, 638–645. doi:10.1021/jp4115095
Girisun, T. C. S., Somayaji, R. M., Priyadarshani, N., and Rao, S. V. (2017). Femtosecond Third Order Optical Nonlinearity and Optical Limiting Studies of (γ and β)-Barium Borate Nanostructures. Mater. Res. Bull. 87, 102–108. doi:10.1016/j.materresbull.2016.11.022
Grote, J. G., Zetts, J. S., Nelson, R. L., Diggs, D. E., Hopkins, F. K., Yaney, P. P., et al. (2002). Nonlinear Optic Polymer Electro-Optic Modulators for Space Applications. Photon. Space Environments VIII, 6–18. doi:10.1117/12.453494
Guo, T., Jin, C., and Smalley, R. E. (1991). Doping Bucky: Formation and Properties of boron-doped Buckminsterfullerene. J. Phys. Chem. 95 (13), 4948–4950. doi:10.1021/j100166a010
Gutsev, G. L., and Boldyrev, A. I. (1982). DVM Xα Calculations on the Electronic Structure of "superalkali" Cations. Chem. Phys. Lett. 92 (3), 262–266. doi:10.1016/0009-2614(82)80272-8
Haddon, R. C., Brus, L. E., and Raghavachari, K. (1986). Electronic Structure and Bonding in Icosahedral C60. Chem. Phys. Lett. 125 (5–6), 459–464. doi:10.1016/0009-2614(86)87079-8
Hawkins, J. M., Meyer, A., Lewis, T. A., Loren, S., and Hollander, F. J. (1991). Crystal Structure of Osmylated C 60 : Confirmation of the Soccer Ball Framework. Science 252 (5003), 312–313. doi:10.1126/science.252.5003.312
Haymet, A. D. J. (1985). C120 and C60: Archimedean Solids Constructed from Sp2 Hybridized Carbon Atoms. Chem. Phys. Lett. 122 (5), 421–424. doi:10.1016/0009-2614(85)87239-0
Heath, J. R., O'Brien, S. C., Zhang, Q., Liu, Y., Curl, R. F., Tittel, F. K., et al. (1985). Lanthanum Complexes of Spheroidal Carbon Shells. J. Am. Chem. Soc. 107 (25), 7779–7780. doi:10.1021/ja00311a102
Hesari, A. M., Shamlouei, H. R., and Raoof Toosi, A. (2016). Effects of the Adsorption of Alkali Metal Oxides on the Electronic, Optical, and Thermodynamic Properties of the Mg12O12nanocage: a Density Functional Theory Study. J. Mol. Model. 22 (8), 1–8. doi:10.1007/s00894-016-3044-7
Hou, D., Nissimagoudar, A. S., Bian, Q., Wu, K., Pan, S., Li, W., et al. (2018a). Prediction and Characterization of NaGaS2, a High thermal Conductivity Mid-infrared Nonlinear Optical Material for High-Power Laser Frequency Conversion. Inorg. Chem. 58 (1), 93–98. doi:10.1021/acs.inorgchem.8b01174
Hou, J., Linsheng, Zhu., Zhu, D., Jiang, D., Qin, J., and Duan, Q. (2018b). Alkaline-earthide: A New Class of Excess Electron Compounds Li-C6h6f6-M (M = Be, Mg and Ca) with Extremely Large Nonlinear Optical Responses. Chem. Phys. Lett. 711 (July), 55–59. doi:10.1016/j.cplett.2018.09.023
Hou, N., Du, F., Feng, R., Wu, H., and Li, Z. (2021). Effects of the Atomic Number of Alkali Atom and Pore Size of Graphyne on the Second‐order Nonlinear Optical Response of Superalkali Salts of Graphynes OM3+@ GYs−(M= Li, Na, and K). Int. J. Quan. Chem. 121 (4), e26477. doi:10.1002/qua.26477
Hou, N., Wu, Y.-Y., and Liu, J.-Y. (2016). Theoretical Studies on Structures and Nonlinear Optical Properties of Alkali Doped Electrides B12N12-M (M = Li, Na, K). Int. J. Quan. Chem. 116 (17), 1296–1302. doi:10.1002/qua.25177
Hou, N., Wu, Y.-Y., Wang, B.-Q., and Wu, H.-S. (2018c). Investigation on the Electronic Structures and Nonlinear Optical Properties of Alkali Metal Atom Doped All-Cis 1,2,3,4,5,6-hexafluorocyclohexane. Int. J. Quan. Chem 118 (15), e25619. doi:10.1002/qua.25619
Huff, T. B., Shi, Y., Fu, Y., Wang, H., and Cheng, J.-X. (2008). Multimodal Nonlinear Optical Microscopy and Applications to central Nervous System Imaging. IEEE J. Select. Top. Quan. Electron. 14 (1), 4–9. doi:10.1109/jstqe.2007.913419
Hutchinson, G. D., and Milburn, G. J. (2004). Nonlinear Quantum Optical Computing via Measurement. J. Mod. Opt. 51 (8), 1211–1222. doi:10.1080/09500340408230417
Iliopoulos, K., Krupka, O., Gindre, D., and Sallé, M. (2010). Reversible Two-Photon Optical Data Storage in Coumarin-Based Copolymers. J. Am. Chem. Soc. 132 (41), 14343–14345. doi:10.1021/ja1047285
Irshad, S., Ullah, F., Khan, S., Ludwig, R., Mahmood, T., and Ayub, K. (2021). First Row Transition Metals Decorated boron Phosphide Nanoclusters as Nonlinear Optical Materials with High Thermodynamic Stability and Enhanced Electronic Properties; A Detailed Quantum Chemical Study. Opt. Laser Tech. 134, 106570. doi:10.1016/j.optlastec.2020.106570
Ivonina, M. V., Orimoto, Y., and Aoki, Y. (2020). Nonlinear Optical Properties of Push-Pull Systems Containing [2.2]paracyclophane: Theoretical Study via Elongation Method. Chem. Phys. Lett. 755, 137760. doi:10.1016/j.cplett.2020.137760
Jing, Y.-Q., Li, Z.-R., Wu, D., Li, Y., Wang, B.-Q., Gu, F. L., et al. (2006). Effect of the Complexant Shape on the Large First Hyperpolarizability of Alkalides Li+(NH3)4M−. ChemPhysChem 7 (8), 1759–1763. doi:10.1002/cphc.200600157
Kachynski, A. V., Pliss, A., Kuzmin, A. N., Ohulchanskyy, T. Y., Baev, A., Qu, J., et al. (2014). Photodynamic Therapy by In Situ Nonlinear Photon Conversion. Nat. Photon 8 (6), 455–461. doi:10.1038/nphoton.2014.90
Kamada, K., Ohta, K., Kubo, T., Shimizu, A., Morita, Y., Nakasuji, K., et al. (2007). Strong Two-Photon Absorption of Singlet Diradical Hydrocarbons. Angew. Chem. Int. Ed. 46 (19), 3544–3546. doi:10.1002/anie.200605061
Kang, L., Liang, F., Jiang, X., Lin, Z., and Chen, C. (2019). First-Principles Design and Simulations Promote the Development of Nonlinear Optical Crystals. Acc. Chem. Res. 53, 209–217. doi:10.1021/acs.accounts.9b00448
Kanis, D. R., Ratner, M. A., and Marks, T. J. (1992). Calculation and Electronic Description of Quadratic Hyperpolarizabilities. Toward a Molecular Understanding of NLO Responses in Organotransition Metal Chromophores. J. Am. Chem. Soc. 114 (26), 10338–10357. doi:10.1021/ja00052a035
Kanis, D. R., Ratner, M. A., and Marks, T. J. (1994). Design and Construction of Molecular Assemblies with Large Second-Order Optical Nonlinearities. Quantum Chemical Aspects. Chem. Rev. 94 (1), 195–242. doi:10.1021/cr00025a007
Kerr, J. (1879). Electro-optic Observation on Various Liquids. Lond. Edinb. Dublin Philos. Mag. J. Sci. 8 (47), 85–102. doi:10.1080/14786447908639658
Khalid, M., Hussain, R., Hussain, A., Ali, B., Jaleel, F., Imran, M., et al. (2019). Electron Donor and Acceptor Influence on the Nonlinear Optical Response of Diacetylene-Functionalized Organic Materials (DFOMs): Density Functional Theory Calculations. Molecules 24 (11), 2096. doi:10.3390/molecules24112096
Khaliq, F., Ayub, K., Mahmood, T., Muhammad, S., Tabassum, S., and Gilani, M. A. (2021a). First Example of Lanthanum as Dopant on Al12N12 and Al12P12 Nanocages for Improved Electronic and Nonlinear Optical Properties with High Stability. Mater. Sci. Semiconductor Process. 135 (July), 106122. doi:10.1016/j.mssp.2021.106122
Khaliq, F., Mahmood, T., Ayub, K., Tabassum, S., and Gilani, M. A. (2021b). Exploring Li4N and Li4O Superalkalis as Efficient Dopants for the Al12N12 Nanocage to Design High Performance Nonlinear Optical Materials with High Thermodynamic Stability. Polyhedron 200, 115145. doi:10.1016/j.poly.2021.115145
Khan, P., Mahmood, T., Ayub, K., Tabassum, S., and Amjad Gilani, M. (2021a). Turning Diamondoids into Nonlinear Optical Materials by Alkali Metal Substitution: A DFT Investigation. Opt. Laser Tech. 142 (April), 107231. doi:10.1016/j.optlastec.2021.107231
Khan, S., Gilani, M. A., Munsif, S., Muhammad, S., Ludwig, R., and Ayub, K. (2021b). Inorganic Electrides of Alkali Metal Doped Zn12O12 Nanocage with Excellent Nonlinear Optical Response. J. Mol. Graphics Model. 106 (May), 107935. doi:10.1016/j.jmgm.2021.107935
Kim, J., Ichimura, A. S., Huang, R. H., Redko, M., Phillips, R. C., Jackson, J. E., et al. (1999). Crystalline Salts of Na- and K- (Alkalides) that Are Stable at Room Temperature. J. Am. Chem. Soc. 121 (45), 10666–10667. doi:10.1021/ja992667v
Kishida, H., Hibino, K., Nakamura, A., Kato, D., and Abe, J. (2010). Third-order Nonlinear Optical Properties of a π-conjugated Biradical Molecule Investigated by Third-Harmonic Generation Spectroscopy. Thin Solid Films 519 (3), 1028–1030. doi:10.1016/j.tsf.2010.08.037
Kobayashi, K., Kuwano, M., Sueki, K., Kikuchi, K., Achiba, Y., Nakahara, H., et al. (1995). Activation and Tracer Techniques for Study of Metallofullerenes. J. Radioanal. Nucl. Chem. Articles 192 (1), 81–89. doi:10.1007/bf02037739
Koohi, M., Soleimani-Amiri, S., and Shariati, M. (2018). Novel X- and Y-Substituted Heterofullerenes X4Y4C12 Developed from the Nanocage C20, where X = B, Al, Ga, Si and Y = N, P, as, Ge: a Comparative Investigation on Their Structural, Stability, and Electronic Properties at DFT. Struct. Chem. 29 (3), 909–920. doi:10.1007/s11224-017-1071-3
Kosar, N., Ayub, K., and Mahmood, T. (2021a). Surface Functionalization of Twisted Graphene C32H15 and C104H52 Derivatives with Alkalis and Superalkalis for NLO Response; a DFT Study. J. Mol. Graphics Model. 102, 107794. doi:10.1016/j.jmgm.2020.107794
Kosar, N., Gul, S., Ayub, K., Bahader, A., Gilani, M. A., Arshad, M., et al. (2020a). Significant Nonlinear Optical Response of Alkaline Earth Metals Doped Beryllium and Magnesium Oxide Nanocages. Mater. Chem. Phys. 242, 122507. doi:10.1016/j.matchemphys.2019.122507
Kosar, N., Shehzadi, K., Ayub, K., and Mahmood, T. (2020b). Theoretical Study on Novel Superalkali Doped Graphdiyne Complexes: Unique Approach for the Enhancement of Electronic and Nonlinear Optical Response. J. Mol. Graphics Model. 97, 107573. doi:10.1016/j.jmgm.2020.107573
Kosar, N., Tahir, H., Ayub, K., Gilani, M. A., Arshad, M., and Mahmood, T. (2021b). Impact of Even Number of Alkaline Earth Metal Doping on the NLO Response of C20 Nanocluster; a DFT Outcome. Comput. Theor. Chem. 1204, 113386. doi:10.1016/j.comptc.2021.113386
Kosar, N., Tahir, H., Ayub, K., Gilani, M. A., and Mahmood, T. (2021c). Theoretical Modification of C24 Fullerene with Single and Multiple Alkaline Earth Metal Atoms for Their Potential Use as NLO Materials. J. Phys. Chem. Sol. 152, 109972. doi:10.1016/j.jpcs.2021.109972
Krätschmer, W., Fostiropoulos, K., and Huffman, D. R. (1990a). The Infrared and Ultraviolet Absorption Spectra of Laboratory-Produced Carbon Dust: Evidence for the Presence of the C60 Molecule. Chem. Phys. Lett. 170 (2–3), 167–170. doi:10.1016/0009-2614(90)87109-5
Krätschmer, W., Lamb, L. D., Fostiropoulos, K., and Huffman, D. R. (1990b). Solid C 60: a New Form of Carbon. Nature 347 (6291), 354–358. doi:10.1038/347354a0
Kroto, H. W., Heath, J. R., O’Brien, S. C., Curl, R. F., and Smalley, R. E. (1985). C60: Buckminsterfullerene. Nature 318 (6042), 162–163. doi:10.1038/318162a0
Kumar, R. A., Vizhi, R. E., Vijayan, N., and Babu, D. R. (2010a). Growth, Optical and Mechanical Properties of Nonlinear Optical Alpha-Lithium Iodate Single crystal. Sch. Res. Lib. 2 (5), 247–254.
Kumar, R. A., Vizhi, R. E., Vijayan, N., Bhagavannarayana, G., and Babu, D. R. (2010b). Growth, Crystalline Perfection and Z-Scan Studies of Nonlinear Optical Alpha-Lithium Iodate Single crystal. J. Pure Appl. Ind. Phys. 1 (1), 1–106.
Kumbhakar, P., and Kobayashi, T. (2007). Ultra-broadband Optical Parametric Amplification in KBe2BO3F2 crystal. Opt. Commun. 277 (1), 205–208. doi:10.1016/j.optcom.2007.04.061
Lee, J., Kim, H., Kahng, S.-J., Kim, G., Son, Y.-W., Ihm, J., et al. (2002). Bandgap Modulation of Carbon Nanotubes by Encapsulated Metallofullerenes. Nature 415 (6875), 1005–1008. doi:10.1038/4151005a
Lettow, J. S., and Martin, C. (2010). Graphene in NLO Devices for High Laser Energy Protection. Jessup, MD: VORBECK MATERIALS CORP.
Li, B., Peng, D., Gu, F. L., and Zhu, C. (2018). Facially Polarized Molecule for Alkalides and Superalkalides with Considerable Nonlinear Optical Response. ChemistrySelect 3, 12782–12790. doi:10.1002/slct.201802769
Li, S., Wu, H., and Wang, L.-S. (1997). Probing the Electronic Structure of Metallocarbohedrenes: M8C12 (M = Ti, V, Cr, Zr, Nb). J. Am. Chem. Soc. 119 (32), 7417–7422. doi:10.1021/ja9710159
Li, W., Chen, B., Meng, C., Fang, W., Xiao, Y., Li, X., et al. (2014). Ultrafast All-Optical Graphene Modulator. Nano Lett. 14 (2), 955–959. doi:10.1021/nl404356t
Li, X.-H., Zhang, X.-L., Chen, Q.-H., Zhang, L., Chen, J.-H., Wu, D., et al. (2020a). Coinage Metalides: a New Class of Excess Electron Compounds with High Stability and Large Nonlinear Optical Responses. Phys. Chem. Chem. Phys. 22 (16), 8476–8484. doi:10.1039/c9cp06894e
Li, X., and Li, S. (2019). Investigations of Electronic and Nonlinear Optical Properties of Single Alkali Metal Adsorbed Graphene, Graphyne and Graphdiyne Systems by First-Principles Calculations. J. Mater. Chem. C 7 (6), 1630–1640. doi:10.1039/c8tc05392h
Li, X., Zhang, Y., and Lu, J. (2020b). Remarkably Enhanced First Hyperpolarizability and Nonlinear Refractive index of Novel Graphdiyne-Based Materials for Promising Optoelectronic Applications: A First-Principles Study. Appl. Surf. Sci. 512 (December 2019), 145544. doi:10.1016/j.apsusc.2020.145544
Li, Y., Heng, W.-K., Lee, B. S., Aratani, N., Zafra, J. L., Bao, N., et al. (2012). Kinetically Blocked Stable Heptazethrene and Octazethrene: Closed-Shell or Open-Shell in the Ground State? J. Am. Chem. Soc. 134 (36), 14913–14922. doi:10.1021/ja304618v
Li, Y., Li, Z.-R., Wu, D., Li, R.-Y., Hao, X.-Y., and Sun, C.-C. (2004). An Ab Initio Prediction of the Extraordinary Static First Hyperpolarizability for the Electron-Solvated Cluster (FH)2{e}(HF). J. Phys. Chem. B 108 (10), 3145–3148. doi:10.1021/jp036808y
Li, Y., Wu, D., and Li, Z.-R. (2008). Compounds of Superatom Clusters: Preferred Structures and Significant Nonlinear Optical Properties of the BLi6-X (X = F, LiF2, BeF3, BF4) Motifs. Inorg. Chem. 47 (21), 9773–9778. doi:10.1021/ic800184z
Li, Z.-J., Li, Z.-R., Wang, F.-F., Luo, C., Ma, F., Wu, D., et al. (2009a). A Dependence on the Petal Number of the Static and Dynamic First Hyperpolarizability for Electride Molecules: Many-petal-shaped Li-Doped Cyclic Polyamines. J. Phys. Chem. A. 113 (12), 2961–2966. doi:10.1021/jp8109012
Li, Z.-J., Wang, F.-F., Li, Z.-R., Xu, H.-L., Huang, X.-R., Wu, D., et al. (2009b). Large Static First and Second Hyperpolarizabilities Dominated by Excess Electron Transition for Radical Ion Pair Salts M2˙+TCNQ˙−(M = Li, Na, K). Phys. Chem. Chem. Phys. 11 (2), 402–408. doi:10.1039/b809161g
Lin, Z., Lu, T., and Ding, X.-L. (2017). A Theoretical Investigation on Doping Superalkali for Triggering Considerable Nonlinear Optical Properties of Si12 C12 Nanostructure. J. Comput. Chem. 38 (18), 1574–1582. doi:10.1002/jcc.24796
Liu, Q., Cui, Q., Li, X. J., and Jin, L. (2014). The Applications of Buckminsterfullerene C60and Derivatives in Orthopaedic Research. Connect. Tissue Res. 55 (2), 71–79. doi:10.3109/03008207.2013.877894
Liu, S., Gao, F.-W., Xu, H.-L., and Su, Z.-M. (2019). Transition Metals Doped Fullerenes: Structures - NLO Property Relationships. Mol. Phys. 117 (6), 705–711. doi:10.1080/00268976.2018.1538540
Liu, X., Guo, Q., and Qiu, J. (2017). Emerging Low-Dimensional Materials for Nonlinear Optics and Ultrafast Photonics. Adv. Mater. 29 (14), 1605886. doi:10.1002/adma.201605886
Luo, M., Liang, F., Song, Y., Zhao, D., Xu, F., Ye, N., et al. (2018). M2B10O14F6 (M = Ca, Sr): Two Noncentrosymmetric Alkaline Earth Fluorooxoborates as Promising Next-Generation Deep-Ultraviolet Nonlinear Optical Materials. J. Am. Chem. Soc. 140 (11), 3884–3887. doi:10.1021/jacs.8b01263
Luo, Z., Li, Y., Zhong, M., Huang, Y., Wan, X., Peng, J., et al. (2015). Nonlinear Optical Absorption of Few-Layer Molybdenum Diselenide (MoSe_2) for Passively Mode-Locked Soliton Fiber Laser [Invited]. Photon. Res. 3 (3), A79–A86. doi:10.1364/PRJ.3.000A79
Mai, J., Gong, S., Li, N., Luo, Q., and Li, Z. (2015). A novel class of compounds-superalkalides: M+(en)3M3′O− (M, M′ = Li, Na, and K; en = ethylenediamine)-with excellent nonlinear optical properties and high stabilities. Phys. Chem. Chem. Phys. 17 (43), 28754–28764. doi:10.1039/c5cp03635f
Maiman, T. H. (1960). Stimulated Optical Radiation in Ruby. Nature 187 (4736), 493–494. doi:10.1038/187493a0
Majumdar, S., Singha, T., Dhibar, S., Mandal, A., Datta, P. K., and Dey, B. (2020). Protein-Based Self-Healing Cu(II)-Metallohydrogels: Efficient Third-Order Nonlinear Optical Materials in Terms of an Intensity-dependent Refractive Index and Two-Photon Absorption. ACS Appl. Electron. Mater. 2 (11), 3678–3685. doi:10.1021/acsaelm.0c00728
Manykin, E. A. (2002). Multiphoton Processes in Systems of Rydberg Atoms. Moscow: Russian Research Center Moscow Kurchatov Inst.
Maria, ., Iqbal, J., and Ayub, K. (2016). Enhanced Electronic and Non-linear Optical Properties of Alkali Metal (Li, Na, K) Doped boron Nitride Nano-Cages. J. Alloys Comp. 687, 976–983. doi:10.1016/j.jallcom.2016.06.121
Maria, ., Iqbal, J., Ludwig, R., and Ayub, K. (2017). Phosphides or Nitrides for Better NLO Properties? A Detailed Comparative Study of Alkali Metal Doped Nano-Cages. Mater. Res. Bull. 92, 113–122. doi:10.1016/j.materresbull.2017.03.065
Markovic, Z., and Trajkovic, V. (2008). Biomedical Potential of the Reactive Oxygen Species Generation and Quenching by Fullerenes (C60). Biomaterials 29 (26), 3561–3573. doi:10.1016/j.biomaterials.2008.05.005
Miller, M. J., Wei, S. H., Parker, I., and Cahalan, M. D. (2002). Two-photon Imaging of Lymphocyte Motility and Antigen Response in Intact Lymph Node. Science 296 (5574), 1869–1873. doi:10.1126/science.1070051
Mollenauer, L. F., Stolen, R. H., and Gordon, J. P. (1980). Experimental Observation of Picosecond Pulse Narrowing and Solitons in Optical Fibers. Phys. Rev. Lett. 45 (13), 1095–1098. doi:10.1103/physrevlett.45.1095
Montellano López, A., Mateo-Alonso, A., and Prato, M. (2011). Materials Chemistry of Fullerene C60derivatives. J. Mater. Chem. 21 (5), 1305–1318. doi:10.1039/c0jm02386h
Muhammad, S., Ito, S., Nakano, M., Kishi, R., Yoneda, K., Kitagawa, Y., et al. (2015). Diradical Character and Nonlinear Optical Properties of Buckyferrocenes: Focusing on the Use of Suitably Modified Fullerene Fragments. Phys. Chem. Chem. Phys. 17 (8), 5805–5816. doi:10.1039/c4cp05175k
Muhammad, S., Minami, T., Fukui, H., Yoneda, K., Minamide, S., Kishi, R., et al. (2013). Comparative Study of Diradical Characters and Third-Order Nonlinear Optical Properties of Linear/cyclic Acenes versus Phenylenes. Int. J. Quan. Chem. 113 (4), 592–598. doi:10.1002/qua.24032
Muhammad, S., Xu, H., Liao, Y., Kan, Y., and Su, Z. (2009). Quantum Mechanical Design and Structure of the Li@B10H14 Basket with a Remarkably Enhanced Electro-Optical Response. J. Am. Chem. Soc. 131 (33), 11833–11840. doi:10.1021/ja9032023
Munsif, S., Maria, ., Khan, S., Ali, A., Gilani, M. A., Iqbal, J., et al. (2018). Remarkable Nonlinear Optical Response of Alkali Metal Doped Aluminum Phosphide and boron Phosphide Nanoclusters. J. Mol. Liquids 271, 51–64. doi:10.1016/j.molliq.2018.08.121
Nakano, M., Kishi, R., Ohta, S., Takebe, A., Takahashi, H., Furukawa, S., et al. (2006b). Origin of the Enhancement of the Second Hyperpolarizability of Singlet Diradical Systems with Intermediate Diradical Character. J. Chem. Phys. 125 (7), 074113. doi:10.1063/1.2213974
Nakano, M., Minami, T., Fukui, H., Kishi, R., Shigeta, Y., and Champagne, B. (2012). Full Configuration Interaction Calculations of the Second Hyperpolarizabilities of the H4 Model Compound: Summation-Over-States Analysis and Interplay with Diradical Characters. J. Chem. Phys. 136 (2), 024315. doi:10.1063/1.3675684
Nakano, M., Kishi, R., Nakagawa, N., Ohta, S., Takahashi, H., Furukawa, S.-i., et al. (2006a). Second Hyperpolarizabilities (γ) of Bisimidazole and Bistriazole Benzenes: Diradical Character, Charged State, and Spin State Dependences. J. Phys. Chem. A. 110 (12), 4238–4243. doi:10.1021/jp056672z
Nakano, M., Kishi, R., Nitta, T., Kubo, T., Nakasuji, K., Kamada, K., et al. (2005). Second Hyperpolarizability (γ) of Singlet Diradical System: Dependence of γ on the Diradical Character. J. Phys. Chem. A. 109 (5), 885–891. doi:10.1021/jp046322x
Nakano, M., Kishi, R., Ohta, S., Takahashi, H., Kubo, T., Kamada, K., et al. (2007). Relationship between Third-Order Nonlinear Optical Properties and Magnetic Interactions in Open-Shell Systems: a New Paradigm for Nonlinear Optics. Phys. Rev. Lett. 99 (3), 33001. doi:10.1103/physrevlett.99.033001
Nakano, M., Nagai, H., Fukui, H., Yoneda, K., Kishi, R., Takahashi, H., et al. (2008). Theoretical Study of Third-Order Nonlinear Optical Properties in Square Nanographenes with Open-Shell Singlet Ground States. Chem. Phys. Lett. 467 (1–3), 120–125. doi:10.1016/j.cplett.2008.10.084
Nakano, M., Nitta, T., Yamaguchi, K., Champagne, B., and Botek, E. (2004). Spin Multiplicity Effects on the Second Hyperpolarizability of an Open-Shell Neutral π-Conjugated System. J. Phys. Chem. A. 108 (18), 4105–4111. doi:10.1021/jp049637l
Ni, B., Cai, X., Lin, J., Li, Y., Huang, S., Li, Z., et al. (2019). Tailoring the Linear and Second-Order Nonlinear Optical Responses of the Titanium-MIL-125 Metal-Organic Framework through Ligand Functionalization: A First Principles Study. J. Phys. Chem. C 123 (1), 653–664. doi:10.1021/acs.jpcc.8b08008
Ni, B., Sun, W., Kang, J., and Zhang, Y. (2020). Understanding the Linear and Second-Order Nonlinear Optical Properties of UiO-66-Derived Metal-Organic Frameworks: A Comprehensive DFT Study. J. Phys. Chem. C 124 (21), 11595–11608. doi:10.1021/acs.jpcc.0c01580
Niu, M., Yu, G., Yang, G., Chen, W., Zhao, X., and Huang, X. (2014). Doping the Alkali Atom: An Effective Strategy to Improve the Electronic and Nonlinear Optical Properties of the Inorganic Al12N12 Nanocage. Inorg. Chem. 53 (1), 349–358. doi:10.1021/ic4022917
Noormohammadbeigi, M., and Shamlouei, H. R. (2018). The Effect of Superalkali M3O (M = Li, Na and K) on Structure, Electrical and Nonlinear Optical Properties of C20 Fullerene Nanocluster. J. Inorg. Organomet. Polym. 28 (1), 110–120. doi:10.1007/s10904-017-0730-6
Novikov, D. L., Gubanov, V. A., and Freeman, A. J. (1992). Electronic Structure, Electron-Phonon Interaction and Superconductivity in K3C60, Rb3C60 and Cs3C60. Physica C: Superconductivity 191 (3–4), 399–408. doi:10.1016/0921-4534(92)90936-7
Ohta, S., Nakano, M., Kubo, T., Kamada, K., Ohta, K., Kishi, R., et al. (2007). Theoretical Study on the Second Hyperpolarizabilities of Phenalenyl Radical Systems Involving Acetylene and Vinylene Linkers: Diradical Character and Spin Multiplicity Dependences. J. Phys. Chem. A. 111 (18), 3633–3641. doi:10.1021/jp0713662
Okada, H., Komuro, T., Sakai, T., Matsuo, Y., Ono, Y., Omote, K., et al. (2012). Preparation of Endohedral Fullerene Containing Lithium (Li@C60) and Isolation as Pure Hexafluorophosphate Salt ([Li+@C60][PF6−]). RSC Adv. 2 (28), 10624–10631. doi:10.1039/c2ra21244g
Oku, T., Nishiwaki, A., and Narita, I. (2004). Formation and Atomic Structure of B12N12nanocage Clusters Studied by Mass Spectrometry and Cluster Calculation. Sci. Tech. Adv. Mater. 5 (5–6), 635–638. doi:10.1016/j.stam.2004.03.017
Omidi, M., Sabzehzari, M., and Shamlouei, H. R. (2020). Influence of Superalkali Oxides on Structural, Electrical and Optical Properties of C24 Fullerene Nanocluster: A Theoretical Study. Chin. J. Phys. 65, 567–578. doi:10.1016/j.cjph.2020.02.032
Omidi, M., Shamlouei, H. R., and Noormohammadbeigi, M. (2017). The Influence of Sc Doping on Structural, Electronic and Optical Properties of Be12O12, Mg12O12 and Ca12O12 Nanocages: a DFT Study. J. Mol. Model. 23 (3), 82. doi:10.1007/s00894-017-3243-x
Orbelli Biroli, A., Tessore, F., Pizzotti, M., Biaggi, C., Ugo, R., Caramori, S., et al. (2011). A Multitechnique Physicochemical Investigation of Various Factors Controlling the Photoaction Spectra and of Some Aspects of the Electron Transfer for a Series of Push-Pull Zn(II) Porphyrins Acting as Dyes in DSSCs. J. Phys. Chem. C 115 (46), 23170–23182. doi:10.1021/jp2030363
Orbelli Biroli, A., Tessore, F., Righetto, S., Forni, A., Macchioni, A., Rocchigiani, L., et al. (2017). Intriguing Influence of −COOH-Driven Intermolecular Aggregation and Acid-Base Interactions with N,N-Dimethylformamide on the Second-Order Nonlinear-Optical Response of 5,15 Push-Pull Diarylzinc(II) Porphyrinates. Inorg. Chem. 56 (11), 6438–6450. doi:10.1021/acs.inorgchem.7b00510
Ortíz-Saavedra, J., Aguilera-Granja, F., Dorantes-Dávila, J., and Morán-López, J. L. (1993). The S and P Character of the Electronic Structure of C20, C60 and C70. Solid State. Commun. 85 (9), 767–771. doi:10.1016/0038-1098(93)90668-D
Paul, D., Deb, J., Bhattacharya, B., and Sarkar, U. (2017). Density Functional Theory Study of Pristine and Transition Metal Doped Fullerene. AIP Conf. Proc. 1832 (1), 50107. doi:10.1063/1.4980340
Pauling, L. (1991). The Structure of K3C60 and the Mechanism of Superconductivity. Proc. Natl. Acad. Sci. 88 (20), 9208–9209. doi:10.1073/pnas.88.20.9208
Pochet, P., Genovese, L., Caliste, D., Rousseau, I., Goedecker, S., and Deutsch, T. (2010). First-principles Prediction of Stable SiC Cage Structures and Their Synthesis Pathways. Phys. Rev. B 82 (3), 35431. doi:10.1103/physrevb.82.035431
Popov, A. A., Yang, S., and Dunsch, L. (2013). Endohedral Fullerenes. Chem. Rev. 113 (8), 5989–6113. doi:10.1021/cr300297r
Popov, A. K., and Shalaev, V. M. (2006). Compensating Losses in Negative-index Metamaterials by Optical Parametric Amplification. Opt. Lett. 31 (14), 2169–2171. doi:10.1364/ol.31.002169
Qiu, Y.-Q., Fan, H.-L., Sun, S.-L., Liu, C.-G., and Su, Z.-M. (2008). Theoretical Study on the Relationship between Spin Multiplicity Effects and Nonlinear Optical Properties of the Pyrrole Radical (C4H4N·). J. Phys. Chem. A. 112 (1), 83–88. doi:10.1021/jp073907t
Rad, A. S., and Ayub, K. (2017). Substitutional Doping of Zirconium-, Molybdenum-, Ruthenium-, and Palladium: an Effective Method to Improve Nonlinear Optical and Electronic Property of C20 Fullerene. Comput. Theor. Chem. 1121, 68–75. doi:10.1016/j.comptc.2017.10.015
Rad, A. S., and Ayub, K. (2019). Change in the Electronic and Nonlinear Optical Properties of Fullerene through its Incorporation with Sc-, Fe-, Cu-, and Zn Transition Metals. Appl. Phys. A 125 (6), 1–9. doi:10.1007/s00339-019-2721-7
Rad, A. S., and Ayub, K. (2018a). How Can Nickel Decoration Affect H 2 Adsorption on B 12 P 12 Nano-Heterostructures? J. Mol. Liquids 255, 168–175. doi:10.1016/j.molliq.2018.01.149
Rad, A. S., and Ayub, K. (2018c). Nonlinear Optical and Electronic Properties of Cr-, Ni-, and Ti- Substituted C 20 Fullerenes: A Quantum-Chemical Study. Mater. Res. Bull. 97 (September 2017), 399–404. doi:10.1016/j.materresbull.2017.09.036
Rad, A. S., and Ayub, K. (2018b). Nonlinear Optical, IR and Orbital Properties of Ni Doped MgO Nanoclusters: A DFT Investigation. Comput. Theor. Chem. 1138, 39–47. doi:10.1016/j.comptc.2018.06.003
Ravaei, I., Haghighat, M., and Azami, S. M. (2019). A DFT, AIM and NBO Study of Isoniazid Drug Delivery by MgO Nanocage. Appl. Surf. Sci. 469 (November 2018), 103–112. doi:10.1016/j.apsusc.2018.11.005
Ray, P. C. (2010). Size and Shape Dependent Second Order Nonlinear Optical Properties of Nanomaterials and Their Application in Biological and Chemical Sensing. Chem. Rev. 110 (9), 5332–5365. doi:10.1021/cr900335q
Raza Ayub, A., Aqil Shehzad, R., Alarfaji, S. S., and Iqbal, J. (2020). Super Alkali (OLi3) Doped boron Nitride with Enhanced Nonlinear Optical Behavior. J. Nonlinear Optic. Phys. Mat. 29 (01n02), 2050004. doi:10.1142/s0218863520500046
Reber, A. C., Khanna, S. N., Hunjan, J. S., and Beltrán, M. R. (2006). Cobalt Doped Rings and Cages of ZnO Clusters: Motifs for Magnetic Cluster-Assembled Materials. Chem. Phys. Lett. 428 (4–6), 376–380. doi:10.1016/j.cplett.2006.07.045
Redko, M. Y., Jackson, J. E., Huang, R. H., and Dye, J. L. (2005). Design and Synthesis of a Thermally Stable Organic Electride. J. Am. Chem. Soc. 127 (35), 12416–12422. doi:10.1021/ja053216f
Rehm, E., Boldyrev, A. I., and Schleyer, P. v. R. (1992). Ab Initio study of Superalkalis. First Ionization Potentials and Thermodynamic Stability. Inorg. Chem. 31 (23), 4834–4842. doi:10.1021/ic00049a022
Reshak, A. H., Chen, X., Auluck, S., and Kamarudin, H. (2012). Linear and Nonlinear Optical Susceptibilities and Hyperpolarizability of Borate LiNaB4O7 Single Crystals: Theory and experiment. J. Appl. Phys. 112 (5), 53526. doi:10.1063/1.4749409
Reshak, A. H., Chen, X., Auluck, S., Kamarudin, H., Chyský, J., Wojciechowski, A., et al. (2013). Linear and Nonlinear Optical Susceptibilities and the Hyperpolarizability of Borate LiBaB9O15 single-crystal: Theory and experiment. J. Phys. Chem. B 117 (45), 14141–14150. doi:10.1021/jp4077905
Reshak, A. H., Kityk, I. V., and Auluck, S. (2010). Investigation of the Linear and Nonlinear Optical Susceptibilities of KTiOPO4 Single Crystals: Theory and experiment. J. Phys. Chem. B 114 (50), 16705–16712. doi:10.1021/jp1072878
Rosen, A., and Waestberg, B. (1988). First-principle Calculations of the Ionization Potentials and Electron Affinities of the Spheroidal Molecules Carbon (C60) and Lanthanum Carbude (LaC60). J. Am. Chem. Soc. 110 (26), 8701–8703. doi:10.1021/ja00234a024
Rosén, A., and Wästberg, B. (1989). Electronic Structure of Spheroidal Metal Containing Carbon Shells: Study of the LaC60 and C60 Clusters and Their Ions within the Local Density Approximation. Z. Für Physik D Atoms, Mol. Clusters 12 (1), 387–390. doi:10.1007/978-3-642-74913-1_86
Ross, R. B., Cardona, C. M., Swain, F. B., Guldi, D. M., Sankaranarayanan, S. G., Van Keuren, E., et al. (2009). Tuning Conversion Efficiency in Metallo Endohedral Fullerene-Based Organic Photovoltaic Devices. Adv. Funct. Mater. 19 (14), 2332–2337. doi:10.1002/adfm.200900214
Sajid, H., Ayub, K., and Mahmood, T. (2020). Exceptionally High NLO Response and Deep Ultraviolet Transparency of Superalkali Doped Macrocyclic Oligofuran Rings. New J. Chem. 44, 2609–2618. doi:10.1039/c9nj05065e
Sajid, H., Ullah, F., Khan, S., Ayub, K., Arshad, M., and Mahmood, T. (2021). Remarkable Static and Dynamic NLO Response of Alkali and Superalkali Doped Macrocyclic [hexa-]thiophene Complexes; a DFT Approach. RSC Adv. 11 (7), 4118–4128. doi:10.1039/d0ra08099c
Sajjad, S., Ali, A., Mahmood, T., and Ayub, K. (2020). Janus Alkaline Earthides with Excellent NLO Response from Sodium and Potassium as Source of Excess Electrons; a First Principles Study. J. Mol. Graphics Model. 100, 107668. doi:10.1016/j.jmgm.2020.107668
Seifert, G., Fowler, P. W., Mitchell, D., Porezag, D., and Frauenheim, T. (1997). Boron-nitrogen Analogues of the Fullerenes: Electronic and Structural Properties. Chem. Phys. Lett. 268 (5–6), 352–358. doi:10.1016/s0009-2614(97)00214-5
Senftleben, O., Stimpel-Lindner, T., Iskra, P., Eisele, J., and Baumgärtner, H. (2009). C60Nanostructures for Applications in Information Technology. Adv. Eng. Mater. 11 (4), 278–284. doi:10.1002/adem.200800293
Shakerzadeh, E., Biglari, Z., and Tahmasebi, E. (2016a). M@B40 (M = Li, Na, K) Serving as a Potential Promising Novel NLO Nanomaterial. Chem. Phys. Lett. 654, 76–80. doi:10.1016/j.cplett.2016.05.014
Shakerzadeh, E., Mashak Shabavi, Z., and Anota, E. C. (2020a). Enhanced Electronic and Nonlinear Optical Responses of C24N24 Cavernous Nitride Fullerene by Decoration with First Row Transition Metals; A Computational Investigation. Appl. Organomet. Chem. 34 (8), e5694. doi:10.1002/aoc.5694
Shakerzadeh, E., Tahmasebi, E., and Biglari, Z. (2016b). A Quantum Chemical Study on the Remarkable Nonlinear Optical and Electronic Characteristics of boron Nitride Nanoclusters by Complexation via Lithium Atom. J. Mol. Liquids 221, 443–451. doi:10.1016/j.molliq.2016.05.090
Shakerzadeh, E., Yousefizadeh, M., and Bamdad, M. (2020b). Electronic and Nonlinear Optical Features of First Row Transition Metals-Decorated All-boron B40 Fullerene: A Promising Route to Remarkable Electro-Optical Response. Inorg. Chem. Commun. 112 (November 2019), 107692. doi:10.1016/j.inoche.2019.107692
Shakerzdeh, E., Tahmasebi, E., and Shamlouei, H. R. (2015). The Influence of Alkali Metals (Li, Na and K) Interaction with Be12O12 and Mg12O12 Nanoclusters on Their Structural, Electronic and Nonlinear Optical Properties: A Theoretical Study. Synth. Met. 204, 17–24. doi:10.1016/j.synthmet.2015.03.008
Shehzadi, K., Ayub, K., and Mahmood, T. (2019). Theoretical Study on Design of Novel Superalkalis Doped Graphdiyne: A New Donor-Acceptor (D-π-A) Strategy for Enhancing NLO Response. Appl. Surf. Sci. 492, 255–263. doi:10.1016/j.apsusc.2019.06.221
Shelton, D. P., and Rice, J. E. (1994). Measurements and Calculations of the Hyperpolarizabilities of Atoms and Small Molecules in the Gas Phase. Chem. Rev. 94 (1), 3–29. doi:10.1021/cr00025a001
Shen, Y. R. (1989). Surface Properties Probed by Second-Harmonic and Sum-Frequency Generation. Nature 337 (6207), 519–525. doi:10.1038/337519a0
Sohail, M., Khaliq, F., Mahmood, T., Ayub, K., Tabassum, S., and Gilani, M. A. (2021). Influence of Bi-alkali Metals Doping over Al12N12 Nanocage on Stability and Optoelectronic Properties: A DFT Investigation. Radiat. Phys. Chem. 184 (November 2020), 109457. doi:10.1016/j.radphyschem.2021.109457
Solimannejad, M., Rahimi, R., and Kamalinahad, S. (2017). Nonlinear Optical (NLO) Response of Si12C12 Nanocage Decorated with Alkali Metals (M = Li, Na and K): A Theoretical Study. J. Inorg. Organomet. Polym. 27 (5), 1234–1242. doi:10.1007/s10904-017-0570-4
Song, Y.-D., Wang, L., and Wang, Q.-T. (2018a). Effect of (Super) Alkali Doping and boron Substitution on the Nonlinear Optical Property of Biphenalenyl Diradical π Dimer: A Theoretical Study. Optik 165, 319–331. doi:10.1016/j.ijleo.2018.03.124
Song, Y.-D., Wang, L., and Wang, Q.-T. (2018b). Structures and Nonlinear Optical Properties of Alkali Atom/superalkali Doped Pyridinic Vacancy Graphene. Optik 154, 411–420. doi:10.1016/j.ijleo.2017.10.100
Song, Y.-D., Wang, L., and Wu, L.-M. (2017). How the Alkali Metal Atoms Affect Electronic Structure and the Nonlinear Optical Properties of C24N24 Nanocage. Optik 135, 139–152. doi:10.1016/j.ijleo.2017.01.096
Sparn, G., Thompson, J. D., Huang, S.-M., Kaner, R. B., Diederich, F., Whetten, R. L., et al. (1991). Pressure Dependence of Superconductivity in Single-phase K 3 C 60. Science 252 (5014), 1829–1831. doi:10.1126/science.252.5014.1829
Srivastava, A. K. (2020). Enormously High Second-Order Nonlinear Optical Response of Single Alkali Atom Decorated Hexalithiobenzene. J. Mol. Liquids 298, 112187. doi:10.1016/j.molliq.2019.112187
Srivastava, A. K., Kumar, A., and Misra, N. (2016a). Structure and Properties of Li@C 60 -PF 6 Endofullerene Complex. Physica E: Low-Dimensional Syst. Nanostructures 84, 524–529. doi:10.1016/j.physe.2016.06.021
Srivastava, A. K., Kumar, A., and Misra, N. (2017). Superalkali@C60−superhalogen: Structure and Nonlinear Optical Properties of a New Class of Endofullerene Complexes. Chem. Phys. Lett. 682, 20–25. doi:10.1016/j.cplett.2017.05.070
Srivastava, A. K., and Misra, N. (2015a). Ab Initio investigations on the Gas Phase Basicity and Nonlinear Optical Properties of FLinOH Species (N = 2-5). RSC Adv. 5 (91), 74206–74211. doi:10.1039/c5ra14735b
Srivastava, A. K., and Misra, N. (2015b). Ab Initio prediction of Novel Alkalides FLi2-M-Li2F (M = Li, Na and K). Chem. Phys. Lett. 639, 307–309. doi:10.1016/j.cplett.2015.09.046
Srivastava, A. K., and Misra, N. (2017). Competition between Alkalide Characteristics and Nonlinear Optical Properties in OLi3⌉M⌉Li3O (M = Li, Na, and K) Complexes ⌉ M⌉ Li3O (M= Li, Na, and K) Complexes. Int. J. Quan. Chem. 117 (3), 208–212. doi:10.1002/qua.25313
Srivastava, A. K., and Misra, N. (2016a). Hydrogenated Superalkalis and Their Possible Applications. J. Mol. Model. 22 (6), 122. doi:10.1007/s00894-016-2994-0
Srivastava, A. K., and Misra, N. (2016c). M2X (M= Li, Na; X= F, Cl): the Smallest Superalkali Clusters with Significant NLO Responses and Electride Characteristics. Mol. Simulation 42 (12), 981–985. doi:10.1080/08927022.2015.1132840
Srivastava, A. K., and Misra, N. (2015c). Nonlinear Optical Behavior of Li N F (N = 2-5) Superalkali Clusters. J. Mol. Model. 21 (12), 305. doi:10.1007/s00894-015-2849-0
Srivastava, A. K., and Misra, N. (2016b). Remarkable NLO Responses of Hyperalkalized Species: the Size Effect and Atomic Number Dependence. New J. Chem. 40 (6), 5467–5472. doi:10.1039/c6nj00584e
Srivastava, A. K., Pandey, S. K., and Misra, N. (2016b). Prediction of superalkali@C60 Endofullerenes, Their Enhanced Stability and Interesting Properties. Chem. Phys. Lett. 655-656, 71–75. doi:10.1016/j.cplett.2016.05.039
Stoilova, O., Jérôme, C., Detrembleur, C., Mouithys-Mickalad, A., Manolova, N., Rashkov, I., et al. (2007). C60-containing Nanostructured Polymeric Materials with Potential Biomedical Applications. Polymer 48 (7), 1835–1843. doi:10.1016/j.polymer.2007.02.026
Stucky, G. D., Phillips, M. L. F., and Gier, T. E. (1989). The Potassium Titanyl Phosphate Structure Field: a Model for New Nonlinear Optical Materials. Chem. Mater. 1 (5), 492–509. doi:10.1021/cm00005a008
Sun, W.-M., Fan, L.-T., Li, Y., Liu, J.-Y., Wu, D., and Li, Z.-R. (2014). On the Potential Application of Superalkali Clusters in Designing Novel Alkalides with Large Nonlinear Optical Properties. Inorg. Chem. 53 (12), 6170–6178. doi:10.1021/ic500655s
Sun, W.-M., Li, X.-H., Wu, D., Li, Y., He, H.-M., Li, Z.-R., et al. (2016b). A Theoretical Study on Superalkali-Doped Nanocages: Unique Inorganic Electrides with High Stability, Deep-Ultraviolet Transparency, and a Considerable Nonlinear Optical Response. Dalton Trans. 45 (17), 7500–7509. doi:10.1039/c6dt00342g
Sun, W.-M., Li, X.-H., Wu, J., Lan, J.-M., Li, C.-Y., Wu, D., et al. (2017a). Can Coinage Metal Atoms Be Capable of Serving as an Excess Electron Source of Alkalides with Considerable Nonlinear Optical Responses? Inorg. Chem. 56 (8), 4594–4600. doi:10.1021/acs.inorgchem.7b00183
Sun, W.-M., Li, Y., Li, X.-H., Wu, D., He, H.-M., Li, C.-Y., et al. (2016a). Stability and Nonlinear Optical Response of Alkalides that Contain a Completely Encapsulated Superalkali Cluster. ChemPhysChem 17 (17), 2672–2678. doi:10.1002/cphc.201600389
Sun, W.-M., Ni, B.-L., Wu, D., Lan, J.-M., Li, C.-Y., Li, Y., et al. (2017b). Designing Alkalides with Considerable Nonlinear Optical Responses and High Stability Based on the Facially Polarized Janus All-Cis-1,2,3,4,5,6-Hexafluorocyclohexane. Organometallics 36 (17), 3352–3359. doi:10.1021/acs.organomet.7b00491
Sun, W.-M., Wu, D., Li, Y., and Li, Z.-R. (2013). Substituent Effects on the Structural Features and Nonlinear Optical Properties of the Organic Alkalide Li+(calix[4]pyrrole)Li−. ChemPhysChem 14 (2), 408–416. doi:10.1002/cphc.201200805
Sun, W.-M., Wu, D., Li, Y., Liu, J.-Y., He, H.-M., and Li, Z.-R. (2015). A Theoretical Study on Novel Alkaline Earth-Based Excess Electron Compounds: Unique Alkalides with Considerable Nonlinear Optical Responses. Phys. Chem. Chem. Phys. 17 (6), 4524–4532. doi:10.1039/c4cp04951a
Tahir, H., Kosar, N., Ayub, K., and Mahmood, T. (2020). Outstanding NLO Response of Thermodynamically Stable Single and Multiple Alkaline Earth Metals Doped C20 Fullerene. J. Mol. Liquids 305, 112875. doi:10.1016/j.molliq.2020.112875
Tahmasebi, E., Shakerzadeh, E., and Biglari, Z. (2016). Theoretical Assessment of the Electro-Optical Features of the Group III Nitrides (B12N12, Al12N12 and Ga12N12) and Group IV Carbides (C24, Si12C12 and Ge12C12) Nanoclusters Encapsulated with Alkali Metals (Li, Na and K). Appl. Surf. Sci. 363, 197–208. doi:10.1016/j.apsusc.2015.12.001
Tehan, F. J., Barnett, B. L., and Dye, J. L. (1974). Alkali Anions. Preparation and crystal Structure of a Compound Which Contains the Cryptated Sodium Cation and the Sodium Anion. J. Am. Chem. Soc. 96 (23), 7203–7208. doi:10.1021/ja00830a005
Tellgmann, R., Krawez, N., Lin, S.-H., Hertel, I. V., and Campbell, E. E. B. (1996). Endohedral Fullerene Production. Nature 382 (6590), 407–408. doi:10.1038/382407a0
Tessore, F., Biroli, A. O., Di Carlo, G., and Pizzotti, M. (2018). Porphyrins for Second Order Nonlinear Optics (NLO): An Intriguing History. Inorganics 6 (3), 81. doi:10.3390/inorganics6030081
Tutt, L. W., and Kost, A. (1992). Optical Limiting Performance of C60 and C70 Solutions. Nature 356 (6366), 225–226. doi:10.1038/356225a0
Ullah, F., Ayub, K., and Mahmood, T. (2020a). Remarkable Second and Third Order Nonlinear Optical Properties of Organometallic C6Li6-M3o Electrides. New J. Chem. 44 (23), 9822–9829. doi:10.1039/d0nj01670e
Ullah, F., Irshad, S., Khan, S., Hashmi, M. A., Ludwig, R., Mahmood, T., et al. (2021). Nonlinear Optical Response of First-Row Transition Metal Doped Al12P12 Nanoclusters; a First-Principles Study. J. Phys. Chem. Sol. 151, 109914. doi:10.1016/j.jpcs.2020.109914
Ullah, F., Kosar, N., Ali, A., Maria, T., Mahmood, T., and Ayub, K. (2020b). Design of Novel Inorganic Alkaline Earth Metal Doped Aluminum Nitride Complexes (AEM@Al12N12) with High Chemical Stability, Improved Electronic Properties and Large Nonlinear Optical Response. Optik 207, 163792. doi:10.1016/j.ijleo.2019.163792
Ullah, F., Kosar, N., Ali, A., MariaMahmood, T., Mahmood, T., and Ayub, K. (2020c). Alkaline Earth Metal Decorated Phosphide Nanoclusters for Potential Applications as High Performance NLO Materials; A First Principle Study. Physica E: Low-Dimensional Syst. Nanostructures 118 (October 2019), 113906. doi:10.1016/j.physe.2019.113906
Ullah, F., Kosar, N., Arshad, M. N., Gilani, M. A., Ayub, K., and Mahmood, T. (2020d). Design of Novel Superalkali Doped Silicon Carbide Nanocages with Giant Nonlinear Optical Response. Opt. Laser Tech. 122, 105855. doi:10.1016/j.optlastec.2019.105855
Ullah, F., Kosar, N., Ayub, K., Gilani, M. A., and Mahmood, T. (2019a). Theoretical Study on a boron Phosphide Nanocage Doped with Superalkalis: Novel Electrides Having Significant Nonlinear Optical Response. New J. Chem. 43 (15), 5727–5736. doi:10.1039/C9NJ00225A
Ullah, F., Kosar, N., Ayub, K., and Mahmood, T. (2019b). Superalkalis as a Source of Diffuse Excess Electrons in Newly Designed Inorganic Electrides with Remarkable Nonlinear Response and Deep Ultraviolet Transparency: A DFT Study. Appl. Surf. Sci. 483, 1118–1128. doi:10.1016/j.apsusc.2019.04.042
Van Cleuvenbergen, S., Stassen, I., Gobechiya, E., Zhang, Y., Markey, K., De Vos, D. E., et al. (2016). ZIF-8 as Nonlinear Optical Material: Influence of Structure and Synthesis. Chem. Mater. 28 (9), 3203–3209. doi:10.1021/acs.chemmater.6b01087
Van Steenwinckel, D., Hendrickx, E., Persoons, A., and Samyn, C. (2001). Large Dynamic Ranges in Photorefractive NLO Polymers and NLO-Polymer-Dispersed Liquid Crystals Using a Bifunctional Chromophore as a Charge Transporter. Chem. Mater. 13 (4), 1230–1237. doi:10.1021/cm001159d
Vijayakumar, T., Hubert Joe, I., Reghunadhan Nair, C. P., and Jayakumar, V. S. (2008). Efficient π Electrons Delocalization in Prospective Push-Pull Non-linear Optical Chromophore 4-[N,N-dimethylamino]-4′-nitro Stilbene (DANS): A Vibrational Spectroscopic Study. Chem. Phys. 343 (1), 83–99. doi:10.1016/j.chemphys.2007.10.033
Wakabayashi, T., and Achiba, Y. (1992). A Model for the C60 and C70 Growth Mechanism. Chem. Phys. Lett. 190 (5), 465–468. doi:10.1016/0009-2614(92)85174-9
Wang, F.-F., Li, Z.-R., Wu, D., Sun, X.-Y., Chen, W., Li, Y., et al. (2006). Novel Superalkali Superhalogen Compounds (Li3)+(SH)− (SH=LiF2, BeF3, and BF4) with Aromaticity: New Electrides and Alkalides. ChemPhysChem 7 (5), 1136–1141. doi:10.1002/cphc.200600014
Wang, F.-F., Li, Z.-R., Wu, D., Wang, B.-Q., Li, Y., Li, Z.-J., et al. (2008). Structures and Considerable Static First Hyperpolarizabilities: New Organic Alkalides (M+@n6adz)M'- (M, M' = Li, Na, K; N = 2, 3) with Cation inside and Anion outside of the Cage Complexants. J. Phys. Chem. B 112 (4), 1090–1094. doi:10.1021/jp076790h
Wang, G., Higgins, S., Wang, K., Bennett, D., Milosavljevic, N., Magan, J. J., et al. (2018). Intensity-dependent Nonlinear Refraction of Antimonene Dispersions in the Visible and Near-Infrared Region. Appl. Opt. 57 (22), E147–E153. doi:10.1364/ao.57.00e147
Wang, H.-W., Langohr, I. M., Sturek, M., and Cheng, J.-X. (2009). Imaging and Quantitative Analysis of Atherosclerotic Lesions by CARS-Based Multimodal Nonlinear Optical Microscopy. Atvb 29 (9), 1342–1348. doi:10.1161/atvbaha.109.189316
Wang, J.-J., Zhou, Z.-J., Bai, Y., Liu, Z.-B., Li, Y., Wu, D., et al. (2012). The Interaction between Superalkalis (M3O, M = Na, K) and a C20F20 Cage Forming Superalkali Electride Salt Molecules with Excess Electrons inside the C20F20 Cage: Dramatic Superalkali Effect on the Nonlinear Optical Property. J. Mater. Chem. 22 (19), 9652–9657. doi:10.1039/c2jm15405f
Wang, S.-J., Li, Y., Wang, Y.-F., Wu, D., and Li, Z.-R. (2013). Structures and Nonlinear Optical Properties of the Endohedral Metallofullerene-Superhalogen Compounds Li@C60-BX4 (X = F, Cl, Br). Phys. Chem. Chem. Phys. 15 (31), 12903–12910. doi:10.1039/c3cp51443a
Wang, Y.-F., Huang, J., Jia, L., and Zhou, G. (2014). Theoretical Investigation of the Structures, Stabilities, and NLO Responses of Calcium-Doped Pyridazine: Alkaline-Earth-Based Alkaline Salt Electrides. J. Mol. Graphics Model. 47, 77–82. doi:10.1016/j.jmgm.2013.11.003
Wang, Z., Li, Y., Sheng-Jiang, G., Jing-Hui, L., Mei, X., and Rastegar, S. F. (2020). Quasi-planar B36 boron Cluster: a New Potential Basis for Ammonia Detection. J. Mol. Model. 26 (10), 263–268. doi:10.1007/s00894-020-04486-2
Wostyn, K., Binnemans, K., Clays, K., and Persoons, A. (2001). Hyper-Rayleigh Scattering in the Fourier Domain for Higher Precision: Correcting for Multiphoton Fluorescence with Demodulation and Phase Data. Rev. Scientific Instr. 72 (8), 3215–3220. doi:10.1063/1.1384429
Wu, B., Hu, J., Cui, P., Jiang, L., Chen, Z., Zhang, Q., et al. (2015). Visible-light Photoexcited Electron Dynamics of Scandium Endohedral Metallofullerenes: the Cage Symmetry and Substituent Effects. J. Am. Chem. Soc. 137 (27), 8769–8774. doi:10.1021/jacs.5b03612
Wu, H.-S., Zhang, F.-Q., Xu, X.-H., Zhang, C.-J., and Jiao, H. (2003). Geometric and Energetic Aspects of Aluminum Nitride Cages. J. Phys. Chem. A. 107 (1), 204–209. doi:10.1021/jp027300i
Xu, H.-L., Li, Z.-R., Wu, D., Ma, F., Li, Z.-J., and Gu, F. L. (2009). Lithiation and Li-Doped Effects of [5]cyclacene on the Static First Hyperpolarizability. J. Phys. Chem. C 113 (12), 4984–4986. doi:10.1021/jp806864w
Xu, H.-L., Li, Z.-R., Wu, D., Wang, B.-Q., Li, Y., Gu, F. L., et al. (2007). Structures and Large NLO Responses of New Electrides: Li-Doped Fluorocarbon Chain. J. Am. Chem. Soc. 129 (10), 2967–2970. doi:10.1021/ja068038k
Xu, H.-L., Sun, S.-L., Muhammad, S., and Su, Z.-M. (2011). Three-propeller-blade-shaped Electride: Remarkable Alkali-Metal-Doped Effect on the First Hyperpolarizability. Theor. Chem. Acc. 128 (2), 241–248. doi:10.1007/s00214-010-0837-0
Yakigaya, K., Takeda, A., Yokoyama, Y., Ito, S., Miyazaki, T., Suetsuna, T., et al. (2007). Superconductivity of Doped Ar@C60. New J. Chem. 31 (6), 973–979. doi:10.1039/b700726d
Yang, H., Li, Y., Wu, D., and Li, Z.-r. (2012). Structural Properties and Nonlinear Optical Responses of Superatom Compounds BF4 -M (M = Li, FLi2 , OLi3 , NLi4 ). Int. J. Quan. Chem. 112 (3), 770–778. doi:10.1002/qua.23053
Yu, G., Huang, X.-R., Chen, W., and Sun, C.-C. (2011). Alkali Metal Atom-Aromatic Ring: A Novel Interaction Mode Realizes Large First Hyperpolarizabilities of M@AR (M = Li, Na, and K, AR = Pyrrole, Indole, Thiophene, and Benzene). J. Comput. Chem. 32 (9), 2005–2011. doi:10.1002/jcc.21789
Yuan, Z., Entwistle, C. D., Collings, J. C., Albesa-Jové, D., Batsanov, A. S., Howard, J. A. K., et al. (2006). Synthesis, Crystal Structures, Linear and Nonlinear Optical Properties, and Theoretical Studies of (P-R-Phenyl)-, (P-R-Phenylethynyl)-, and (E)-[2-(p-R-Phenyl)ethenyl]dimesitylboranes and Related Compounds. Chem. Eur. J. 12 (10), 2758–2771. doi:10.1002/chem.200501096
Zeng, Z., Sung, Y. M., Bao, N., Tan, D., Lee, R., Zafra, J. L., et al. (2012). Stable Tetrabenzo-Chichibabin's Hydrocarbons: Tunable Ground State and Unusual Transition between Their Closed-Shell and Open-Shell Resonance Forms. J. Am. Chem. Soc. 134 (35), 14513–14525. doi:10.1021/ja3050579
Zhai, H.-J., Zhao, Y.-F., Li, W.-L., Chen, Q., Bai, H., Hu, H.-S., et al. (2014). Observation of an All-boron Fullerene. Nat. Chem 6 (8), 727–731. doi:10.1038/nchem.1999
Zhang, F., Zhang, F., Qun, J., Pan, S., Yang, Z., and Jia, D. (2015). Synthesis, Characterization and Theoretical Studies of Nonlinear Optical crystal Sr2B5O9(OH)·H2O. Phys. Chem. Chem. Phys. 17 (16), 10489–10496. doi:10.1039/c5cp00864f
Zhang, J., Liu, K., Xing, G., Ren, T., and Wang, S. (2007). Synthesis and In Vivo Study of Metallofullerene Based MRI Contrast Agent. J. Radioanal. Nucl. Chem. 272 (3), 605–609. doi:10.1007/s10967-007-0632-0
Zheng, C., Huang, J., Lei, L., Chen, W., Wang, H., and Li, W. (2018a). Nanosecond Nonlinear Optical and Optical Limiting Properties of Hollow Gold Nanocages. Appl. Phys. B 124 (1), 17. doi:10.1007/s00340-017-6888-3
Zheng, C., Huang, J., Lei, L., Chen, W., Wang, H., and Li, W. (2018b). Nanosecond Nonlinear Optical and Optical Limiting Properties of Hollow Gold Nanocages. Appl. Phys. B 124 (1), 1–8. doi:10.1007/s00340-017-6888-3
Zhou, S., Yang, X., Shen, Y., King, R. B., and Zhao, J. (2019). Dual Transition Metal Doped Germanium Clusters for Catalysis of CO Oxidation. J. Alloys Comp. 806, 698–704. doi:10.1016/j.jallcom.2019.07.297
Zhu, L., Xue, K., and Hou, J. (2019). A Theoretical Study of Alkaline-Earthides Li(NH3)4M (M = Be, Mg, Ca) with Large First Hyperpolarizability. J. Mol. Model. 25 (6), 1–10. doi:10.1007/s00894-019-4042-3
Zyss, J., and Ledoux, I. (1994). Nonlinear Optics in Multipolar media: Theory and Experiments. Chem. Rev. 94 (1), 77–105. doi:10.1021/cr00025a003
Keywords: nonlinear optical materials (NLO), fullerenes, hyperpolarizability, electrides, alkalides
Citation: Bano R, Asghar M, Ayub K, Mahmood T, Iqbal J, Tabassum S, Zakaria R and Gilani MA (2021) A Theoretical Perspective on Strategies for Modeling High Performance Nonlinear Optical Materials. Front. Mater. 8:783239. doi: 10.3389/fmats.2021.783239
Received: 25 September 2021; Accepted: 15 November 2021;
Published: 15 December 2021.
Edited by:
Mingliang Tian, Hefei Institutes of Physical Science (CAS), ChinaReviewed by:
Ambrish Kumar Srivastava, Deen Dayal Upadhyay Gorakhpur University, IndiaCopyright © 2021 Bano, Asghar, Ayub, Mahmood, Iqbal, Tabassum, Zakaria and Gilani. This is an open-access article distributed under the terms of the Creative Commons Attribution License (CC BY). The use, distribution or reproduction in other forums is permitted, provided the original author(s) and the copyright owner(s) are credited and that the original publication in this journal is cited, in accordance with accepted academic practice. No use, distribution or reproduction is permitted which does not comply with these terms.
*Correspondence: Mazhar Amjad Gilani, bWF6aGFyZ2lsYW5pQGN1aWxhaG9yZS5lZHUucGs=
Disclaimer: All claims expressed in this article are solely those of the authors and do not necessarily represent those of their affiliated organizations, or those of the publisher, the editors and the reviewers. Any product that may be evaluated in this article or claim that may be made by its manufacturer is not guaranteed or endorsed by the publisher.
Research integrity at Frontiers
Learn more about the work of our research integrity team to safeguard the quality of each article we publish.