- 1Space Science Centre (ANGKASA), Universiti Kebangsaan Malaysia, UKM, Bangi, Malaysia
- 2Institute of Radiation and Polymer Technology, Bangladesh Atomic Energy Commission, Dhaka, Bangladesh
- 3Centre for Applied Physics and Radiation Technologies, Sunway University, Bandar Sunway, Petaling Jaya, Malaysia
- 4Department of Radiological Sciences, College of Applied Medical Sciences, Taif University, Taif, Saudi Arabia
- 5Hydrocolloids Research Centre, University of Chester, Chester, United Kingdom
To estimate the molecular weight (Mw) and gelling properties, a total of 26 alginate samples consisting of control (n = 13) and 15 kGy γ-irradiated (n = 13) samples were characterized through viscometric and gel permeation chromatography (GPC-MALLS) methods. Based on the observations, a remarkable decrease in the intrinsic viscosity of all samples of alginates was evident due to the effects of radiation, with a linear relationship between viscosity and concentration in 0.01 M NaCl solution. The correlation among the Mw, percentage mass recovery, radii of gyration (Rz/Rg), and percentage reduction of Mw assessed by GPC was significant. The Mw decreased dramatically (from 3.1 × 105 to 0.49 × 105 mole/g in sample no. 12) by the effect of radiation with momentous relation to the % reduction of the molecular weight. The highest molecular weight reduction (84%), which is the most sensitive to γ-radiation, and the average reduction rate was ≥50%. The mass recovery was 100% obtained from samples no. 1,3,4,5,7,12, and 13, while the rest of the samples’ recovery rate was significantly higher. The reduction rate of mass molecular weight (Mw) is higher than the average molecular weight (Mv), but they showed a sensitivity towards radiation, consequently their performance are different from each other. The stability test was performed as a critical behaviour in the control, recurrently same as in the irradiated samples. Thus, the sterilization dose of 15 kGy for the Mw distribution, and subsequently for the characterization, was significantly effective.
Introduction
Alginates are unbranched copolymers consisting of (1–4)-linked α-L-guluronic acid (G) and β-D-mannuronic acid (M), where these monomers are responsible for the formation of sequential block structures of M-, G-, MG-, and GM- (Artiga-Artigas et al., 2017; Feng et al., 2017; Ammar et al., 2018; GhorbaniGorji et al., 2018). Since the M and G residues are in the 1C4 and 4C1 conformation, the types of glycosidic linkages identified include diaxial (GG), diequatorial (MM), and equatorial-axial (MG). However, the stiffness and chain extension of the G-block is more dominant compared to the M-block due to the hindered rotation around the glycosidic linkage (Jiao et al., 2019).
Polymers have been utilized in various fields such as agriculture, environmental, and medicine. Previous literature reported the use of polymers in biomaterials (soft and hard tissue scaffolds, cartilage, etc.) to aerospace materials (Bashir and Rajendran, 2018; Song et al., 2018) and agriculture (Milani et al., 2017). As such, natural polymers revolutionized the agricultural, horticultural, healthcare, and industrial sectors including food factories over the past 2 decades. Due to these technological advancements, rapid disease detection in the agricultural sector has become feasible where cellular unicellular healing treatment amplifies the ability of plants to grow in harsh conditions and yield quality products (Malerba and Cerana, 2019). With such technological advancements, a great surge in the demand for organic and fresh agricultural products was observed.
Scholars across the world are now investigating to develop new natural products that render plant growth promotion potential, subdue post-harvest losses from pathogenic diseases, enhance plant yields, and eventually avert negative effects on the human body. Among the types of polymers widely accepted for the above-mentioned purposes include Na-alginate, chitosan, and carrageenan. These compounds are non-toxic, biodegradable, bioactive polymers, and are appreciated worldwide for their bio-fertilizer activities, fungicidal effects, and whole plant elicitors (Uddin et al., 2020).
Alginate is widely accepted in various fields due to its ability to form gels through easy binding to cations. It only requires a divalent cation to preferentially bind and has the affinity towards alginate to convert a soluble alginate solution to gel. When the gel is formed, the cations yield a higher affinity towards the G-block rather than the M-block (Szekalska et al., 2016; Urbanova et al., 2019; Abasalizadeh et al., 2020). Hence, the monomering composition and sequence (i.e., blockiness) affect the gel-forming of alginate. During the cationic chelation gel formation by Ca2+, G-enriched alginate samples render rigid and frail gels, while the M-enriched samples produce pliable and flexible gels (Sehgal et al., 2019). Moreover, the gel formation phenomenon of the alginate is widely termed the “egg-box” model (Chan et al., 2017; Gawkowska et al., 2018; Zhang et al., 2019; Cao et al., 2020). Since molecular degradation is a very important property of polymers, degradation studies are widely performed to elucidate the stature of polymers and produce/prepare potentially low Mw compounds such as oligomers and dimers from polymers (La Mantia et al., 2017; Pathak and Navneet, 2017).
Among the different techniques available, radiation processing technology is fairly novel and promising for the investigation of degradation. As such, the application of ionizing radiation is actively used in numerous fields including the production of viscose, flesh, paper, pharmaceuticals, food preservation, and natural bio sensitive agents. That said, the polysaccharides and derivatives of the polysaccharides that are degraded and exposed to high-energy quantum beam radiation have long been termed degradable polymers (Tamada and Kudo, 2018; Ashfaq et al., 2020).
This study aims to acquire more knowledge on the impacts of chemical composition and sequential arrangement of alginate using radiation technology in pharmaceutical, industrial, and agricultural applications, together with the characterization of alginate. Hence, the study investigates the reduction rate after irradiation, particularly focusing on a radiation dose of 15 kGy, which has not the same effect on their characterization, with diverse correlations among the commercial samples of this experiment. The experimental results are expected to open a research avenue for future studies in polymer characterizations.
Experimental Methods and Techniques
Materials
Alginate samples were extracted from the brown algae (Phaeophyceae), a common source of all samples with varying initial Mw. Analytical grade reagents including NaCl, CaCl2, and NaN3 were also utilized in this study (purchased from Fisher Scientific, UK).
Methods
The irradiation breaks down the Mw and the alginates into smaller units of molecules (M-/G-blocks). Hence, the degradation produces polymers with low Mw, manifesting the critical conditions of low and high doses of γ-radiation. A single dose of 15 kGy was applied to the commercial alginate samples. A correlation of experimental results following the different methods is stated below.
Measurement of Concentration-dependent pH
Alginate samples at different concentrations, 10 ml (w/w) in 0.01 M NaCl were prepared to allow a continuous shaker to obtain a clear solution. The pH buffer series (4.0, 7.0, and 9.2) were prepared for calibration of the pH meter, followed by pH measurement using a Benchtop pH meter (Orion 4 Star, pH-ISE, Thermo Electron Corporation).
Determination of Intrinsic Viscosity
The changes in the relative viscosity of the prepared alginate solutions were assessed using an Ubbelohde-type (Cannon Ubbelohde Semi-Micro Calib 75) capillary viscometer during binding with 0.01 M NaCl at 25°C ± 1°C (Sariyerli et al., 2018).
The 1 L 0.01 M NaCl solvent was prepared and filtered using a 0.8 microns cellulose acetate membrane (Na-glene, 25 mm) vacuum filter, followed by the addition of 0.005% sodium azide (NaN3) to protect the bacterial growth. The different concentrations of alginate samples 10 ml (w/w) were dissolved in the solvent and left overnight on a roller to allow continuous shaking for clear solutions. Following homogenization, the time flow (in seconds) of the solvent between the two marks of a calibrated Ubbelohde viscometer was measured. This procedure was also repeated with 2 ml alginate solution. The solution was also filtered using a 0.8 microns cellulose membrane to remove insoluble materials. The time to flow between the two marks of the viscometer was measured twice. If the difference error was less than 2 s, both the readings were averaged, but if the error was more than 2 s, a third measurement was recorded before averaging the time. However, if the readings were significantly different a fresh batch of samples was prepared. If the time recorded for the alginate emulsion was between 2 and 3 times that of the solvent, the sample was diluted, and a longer time recorded required the sample to be diluted again before measurement, whereas a shorter time recorded required the preparation of a more concentrated solution. Next, 0.4 ml of the filtered solvent (0.01 M NaCl) was added to the viscometer to measure the viscosity. The final measurement (time of flow) was repeated by successively adding 0.6, 1, 2, and 4 ml of solvent.
Determination of Mw Based on GPC-MALLS
The Gel Permeation Chromatography coupled with a multi-angle laser light scattering (Dawn DSP, Wyatt Technology Corporation) was employed to assess the Mw distribution and polymeric system parameters (Feng et al., 2017; Hadar et al., 2019). The 0.1 M NaCl solvent was prepared and filtered using Cellulose Nitrate membrane (pore size: 0.22 µm, dia: 0.47, Thermo Scientific, DS0200-4020, made in the United States). Approximately 50–100 ml of the solvent was used to rinse the vacuum flask gently before filtering the rest of the amount. Then, 0.005% NaN3 was added to prevent bacterial growth. The 10 ml (w/w) sample (1 mg/ml concentration) prepared under considering the following moisture content calculation: Exact Sample = sample (g) × (1- moisture content/100). The RI and UV detectors were set to auto-zero before injecting samples into the system. Afterword, 1 mg sample was injected into the system through the filter (membrane 200, MF 1.2) at a pump flow rate of 0.45 ml/min, pressure of the whole system was 1.99–2.2 Mpa, using 11 detectors in UV scattering, light scattering wavelength was 280 nm, 35°C, and 0.2 μW. At the end of the run, the Mw, radii of gyration, and percentage mass recovery (calculated mass/injected mass×100) were recorded.
Determination of Mark-Houwink Constant
To determine the Mark-Houwink constant, a plot was plotted taking the logarithm of intrinsic viscosity against the logarithm of Mw (M) obtained from GPC-MALLS, using the equation:
The slope of a straight line represents the ɑ-value, while the intercept represents the (Mark-Houwink-Equation) k value is l. The values of the constants K and ɑ were 8.1 × 10–3 ml/g and 0.92, respectively (El-Mohdy, 2017; Masimov et al., 2019).
Stability Test of the Samples
To measure the solubility, the gel was prepared following the “procedure”, then filtered using an MF 200, size 70 mm (pore size 1.2 mm) glass microfibers and weighted as W1. The weight (W) of the glass fiber membrane was measured after drying it in an oven at 105°C for 1 h, before subsequently chilling it in the desiccator with silica gel. The method that was approved by the Joint Expert Committee on Food Additives (JECFA) for hydrocolloids, was adopted by changing the solvent from mild alkaline to water. To determine the gel fraction, test materials depending on mesh size likely a 20-mesh steel screen (1,041 µm) can be used (H. Gulrez et al., 2011). The gel strength was determined using two steps namely in 50 ml of water and by adding 1 ml of 0.5 M CaCl2 gelling solution dropwise into 50 ml of water as a binding agent (Artiga-Artigas et al., 2017). Described procedure was followed for 50 ml in water solution and 50 ml in water by adding the gelling agent. The filter paper dried in an oven at 105°C until it cooled and reached a constant weight. The percentage gel strength was calculated using:
The % Gel Strength increased = % Gel strength with CaCl2 - % Gel strength in water.
“Procedure”
Step 1: The 0.5% alginate (100 ml) solution was prepared by adding 0.5 g of alginate into 100 ml warm distilled water while stirring (using magnetic flee) continuously for 15 min at 60°C. Subsequently, 0.5 M CaCl2 (50 ml) solution was prepared and kept separately. A little amount was taken in a universal vial then picked up a 1 ml syringe and kept it in a safe place.
Step 2: Ensured the sample is fully dissolved. If the sample is not fully dissolved, stirring was continued until no visible insoluble materials. Once fully dissolved, 50 ml of the exact solution (0.5% stock) was transferred into a beaker and kept aside.
Step 3: The remaining solution was filtered (while hot) according to the following method. The weight of an MF 200, size 47 µm glass microfiber was determined (W1) before filtering the remaining 0.5% alginate solution. Introduced a small portion of the solution to the filter then started pump for filtering gently. Then put the full sample to be filtered accurately. The beaker should be rinsed with exact 5 ml distilled water and the solution filtered again. The same process was repeated for all samples, with and without gel solution.
Step 4: Following filtrations, the filter paper was gently removed from the vacuum filter system and dried in a dryer overnight (with labels on aluminum foil).
Step 5: A 1 ml volume of 0.5 M CaCl2 solution was added dropwise into a beaker with the solution from Step 2 while stirring for 5 min. After stirring, Step 3 and Step 4 were repeated with a 1 ml syringe. The filter paper was transferred to aluminum foil and dried overnight at 105°C.
Step 6: The next day, the filter papers from the dryer were immediately transferred into a desiccator before the filter papers were measured. The pre- and post-weight were measured to determine the gel strength following the equation.
Degree of Polymerisation
The DP is defined as the number of monomers in a macromolecule or polymer or oligomer molecule (Anshuman, 2018). The atomic mass/Mw of the monomeric per unit of alginates samples has taken 200 and calculated the DP followed by DP = Total Mw/atomic weight of the monomeric per unit (200).
Statistical Analysis
The experimental data is made up of the results obtained from the mean averages of three replications of the samples. A sample is taken and run through the system three times, and the mean average is considered for the data presented. The data were analyzed using the M-STAT program, where the covariance was accepted at 0.01 ≥ 0.05 level of significance.
Results and Discussion
Effects of Radiation on the pH
In the irradiated samples, the dissociated monomeric units with small molecules are capable of absorbing water through inter- and intra-molecular pores. Therefore, the moisture content significantly increased for all samples following irradiation. Based on Table 1, the pH values were recorded in the range of 5.2/5.3 (sample 7) to 7.2/7.0 (sample 3) in the control/irradiated sample. Although the pH values were not significantly different in terms of radiation, a small variation was observed among the samples. The pH values indicated a slight dissociation of the carboxylic acid groups. Meanwhile, in an aqueous alginate solution, two types of forces were identified, the repulsive forces among the ionized carboxylic -COO- groups and the H-bonding formed between ionized carboxyl groups and carboxylic acid groups. These two forces are opposite to each other. At a high pH values exceeding the pKa value (3.7) of the uronic acid groups, the mutual repulsive forces between the ionized carboxyl groups because the network structure to loosen. Whereas, at a low pH, the electrostatic interactions are weakened promoting the intermolecular H-bonds leading to possible entanglements (Tally and Atassi, 2015; Rizwan et al., 2017; Ghobashy and Bassioni, 2018; Olad et al., 2018; Shivakumara and Demappa, 2019).
Effect of Irradiation on Intrinsic Viscosity
The determination of the viscosity of alginate solutions in water is not suited for characterizing alginate samples (data not presented). The alginate solutions presented electrolytic behavior in a water solvent. There was no significant linear relationship observed between the viscosity and concentration of the alginate aqueous solutions in water. Although the findings are under review, the effects of salts were most pronounced at low concentrations of alginate. When the concentration of alginate was increased, the ionic strength increased. Moreover, at a sufficiently high alginate concentration (approximately 3%), an increase in ionic strength was also observed through the addition of inorganic salt to the solution that produced very little or no effect on the viscosity (Wu et al., 2018). Generally, polyelectrolytes possess intrinsic viscosity that is reciprocally linear to the square root of ionic strength (Figure 1). It is well known that the viscosity of alginates is ionic strength dependent strongly. However, the relationship to the ionic strength has not yet been established (Chen et al., 2019).
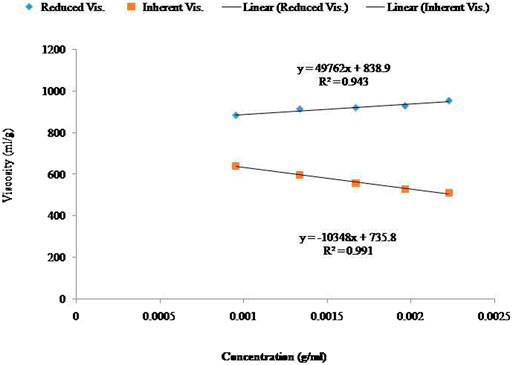
FIGURE 1. Linear relationship between the concentration and viscosity of specific control samples in solvent (0.01 M NaCl). Effects of average Mw (Mv) (Viscometric method).
To determine the Mv of the alginates, the Mark-Houwink equation was used:
Where [η] is the intrinsic viscosity, k and a are values of constants, and M is the Mv. A plot of log η vs Mw yielded the α value from the slope of the straight line and log k value from the axis intercept. The control constants α and k were calculated as 0.94 and 7.25 × 10–5, respectively, while the constants of the irradiated samples were calculated as 0.91 and 8.78 × 10–5, respectively (Figure 2; Figure 3).
The Mark-Houwink’s parameter α for the control and irradiated samples ranged from 0.91 to 0.94 and from 7.254 × 10–5 to 8.78 × 10–5 for K at different ionic strengths of the alginate samples. Polymer or bio-polymer samples with linear chain stature had no branches and yielded α values of 0.6–0.8, whereas branched sampled yielded lower α values, and stiff chain with stretched samples yielded higher values (Mudgil et al., 2018). Therefore, the α values were calculated in this experiment to confirm the open and stiff structure of the samples, because Table 1 listed lower values for the irradiated samples.
Effects of Mw Based on GPC-MALLS
The GPC was used to determine the molecular distribution of all samples. Table 1 presents the Mw, percentage mass recovery, radii of gyration (Rz/Rg), and percentage reduction of Mw. The Mw and radii of gyration decreased due to the effects of γ-radiation. The Mv of intrinsic viscosity is usually higher than the Mw. The viscosity average molecular weight depends on intrinsic viscosity which is reliant on ionic strength of the solution and the interaction between per unit helical residues, and it varies on the effect of Mark-Houwink’s K and α value. The relationships between the initial Mv and Mw with irradiated Mv and Mw are depicted in Figure 4; Figure 5, respectively.
Correlation Between Mw and Percentage Reduction of Initial and Irradiated Samples
The initial Mw of the samples was dramatically decreased by the irradiation on all alginate samples. The highest reduction was observed in sample 12 (84% reduction) and the lowest reduction was observed in sample 13 (33% reduction). Samples 2, 4, 5, 7, 8, and 10 yielded approximately 50% reduction due to the effects of irradiation. On the other hand, samples 6 and 9 showed an approximately 60% reduction. In short, all the samples are sensitive towards irradiation (percentage reduction of Mw), but this was also dependant on the dose of radiation. The graphical correlations of molecular and % reduction between the initial and irradiated samples are presented in Figure 6.
Effects of Radiation on DP
According to the calculated DP presented in Table 1, some alginate samples were dramatically affected by the radiation. The results revealed that some samples were sensitive towards a radiation dose of 15 kGy, while several samples degraded comparatively slowly depending on the block of G- and M-units of the alginate samples.
Effects of Radiation on Solubility (Gel-Strength/Hydrogel Content)
The hydrogel content is observed as acted as a critical nature with the CaCl2 salt (Figure 7). Among the 26 samples, both in control and irradiated samples showed decreases along with increases, and some are the same in nature in terms of hydrogel content for the binding behavior of alginate to CaCl2. Based on the established isothermal process, viscosity, and Ca2+ selective electrode measurements (Fang et al., 2007), the binding of Ca2+ to alginate involved three distinct steps upon increasing the concentration of CaCl2. The findings were delineated into three distinct and successive steps in the binding of calcium to alginate with increasing concentrations of Ca2+: 1) interaction of Ca2+ with a single guluronate unit forming monocomplexes; 2) propagation and creation of egg-box dimmers via the pairing of these monocomplexes; and 3) lateral association of the egg-box dimmers, generating multimers. The final step created different association modes based on the varied Mw of the alginate samples.
The interchain association along with the boundaries between these steps were reasonably critical, which closely correlated with the Ca/guluronate stoichiometry outcome for the egg-box dimmers and multimers with 2/1 helical association of the chains. The configuration of the egg-box dimmers and their consequent association thermodynamically corresponded that was fitted by a model of self-regulating binding sites. The relationship of percentage gel content of the initial and irradiated samples is illustrated in Figure 8. The percentage gel contents of the samples in case of gel strength are showed critical behavior in nature. The gel content of samples 2, 3, 5, 6, 9, and 10 decreased after irradiation, while samples 1, 4, 7, 12, and 13 increased, and samples 8 and 11 remained unchanged (initial and irradiated).
On the other hand, the gel strength increased for some samples because more G residues were released in irradiated samples. However, at high doses of irradiation, the alginate samples degraded into smaller units with the existing mechanism (Mollah et al., 2009). Another proposed phenomenon explained that some samples became more sensitive to M residues after irradiation. The M content comparatively decreased, whereas increased G residues quickly reacted with Ca2+ to produce egg-box dimmer and increased the percentage gel strength (Figure 7) (Fang et al., 2007). The results also revealed that the isotherm technique which involves three steps of binding phenomena occurred depending on the (Ca/G = R) stoichiometry (step I; R = 0.25, step II; R = 0.55, step III; R = 0.75). Based on the stoichiometry (Figure 8), the samples were laterally associated with egg-box multimers composed of 2/1 helical chains. The egg-box dimmers are formed to laterally aggregate with the inter-cluster association which is related to a reduction in molecular size.
Moreover, the G-break dependent on gel strength was calculated (G-value = gel strength/0.25). The results indicated that the peak in sample 7 (control) was similar to sample 6, while the lowest was identified in sample 13 (control). The G-break was proportional to the gel strength, in accordance with the literature. Hence, it can be inferred that the gel strength depends on the G-value and that it increases in irradiated samples.
Conclusion
This study investigated the degradation of natural polymer alginate via γ-radiation. The viscometry and GPC were utilized to assess the Mw distribution of the control and 15 kGy irradiated samples. In aqueous solutions of the alginate, samples indicated a significant linear relationship between viscosity and concentration, whereas a desired linear relationship was obtained in 0.01 M NaCl solution for both the control and irradiated samples. Irradiation produced a reducing trend in the intrinsic viscosity. While the GPC results for the irradiated samples were significant in terms of Mw, percentage mass recovery, radii of gyration (Rz/Rg), and percentage reduction of Mw. Also, the Mv was irregular and higher compared to the Mw. The Mw demonstrated a drastic downward change due to radiation. The most sensitive sample towards degradation by radiation yielded 84% Mw reduction (highest), whereas the rest of the samples yielded a reduction rate of ≥50%, based on the dose of radiation as it directly breaks the chain causing interchain associations to become smaller and free from the bond. In conclusion, the effects of radiation on the degradation of natural polymer alginate were proven.
Data Availability Statement
The raw data supporting the conclusions of this article will be made available by the authors, without undue reservation.
Author Contributions
Conceptualization; MM, SA-A, Methodology; MM, SA-A, Writing-original draft preparation, MM, MR; Writing-review and editing, MM, MF, MK; Supervision, SA-A and MF; Project administration, MF; All authors have read and agreed to the published version of the manuscript.
Conflict of Interest
The authors declare that the research was conducted in the absence of any commercial or financial relationships that could be construed as a potential conflict of interest.
Publisher’s Note
All claims expressed in this article are solely those of the authors and do not necessarily represent those of their affiliated organizations, or those of the publisher, the editors and the reviewers. Any product that may be evaluated in this article, orclaim that may be made by its manufacturer, is not guaranteed or endorsed by the publisher.
Acknowledgments
MM is thankful to SA-A, a host professor in the United Kingdom who allowed us to do research in his laboratory. The authors would like to extend their sincere thanks to Taif University researchers supporting project number (TURSP-2020/287), Taif, Saudi Arabia for its funding and support of APC.
Supplementary Material
The Supplementary Material for this article can be found online at: https://www.frontiersin.org/articles/10.3389/fmats.2021.761995/full#supplementary-material
References
Abasalizadeh, F., Moghaddam, S. V., Alizadeh, E., akbari, E., Kashani, E., Fazljou, S. M. B., et al. (2020). Alginate-based Hydrogels as Drug Delivery Vehicles in Cancer Treatment and Their Applications in Wound Dressing and 3D Bioprinting. J. Biol. Eng. 14 (1). doi:10.1186/s13036-020-0227-7
Ammar, H. H., Lajili, S., Sakly, N., Cherif, D., Rihouey, C., Le Cerf, D., et al. (2018). Influence of the Uronic Acid Composition on the Gastroprotective Activity of Alginates from Three Different Genus of Tunisian Brown Algae. Food Chem. 239, 165–171. doi:10.1016/j.foodchem.2017.06.108
Anshuman, S. (2018). Plastics Design Library, Introduction to Plastics Engineering. William Andrew Publishing, 17–48. ISBN 9780323395007. doi:10.1016/B978-0-323-39500-7.00002-2
Artiga-Artigas, M., Acevedo-Fani, A., and Martín-Belloso, O. (2017). Effect of Sodium Alginate Incorporation Procedure on the Physicochemical Properties of Nanoemulsions. Food Hydrocolloids 70, 191–200. doi:10.1016/j.foodhyd.2017.04.006
Ashfaq, A., Clochard, M.-C., Coqueret, X., Dispenza, C., Driscoll, M. S., Ulański, P., et al. (2020). Polymerization Reactions and Modifications of Polymers by Ionizing Radiation. Polymers 12 (12), 2877. doi:10.3390/polym12122877
Bashir, M., and Rajendran, P. (2018). A Review on Electroactive Polymers Development for Aerospace Applications. J. Intell. Mater. Syst. Structures 29 (19), 3681–3695. doi:10.1177/1045389x18798951
Cao, L., Lu, W., Mata, A., Nishinari, K., and Fang, Y. (2020). Egg-box Model-Based Gelation of Alginate and Pectin: A Review. Carbohydr. Polym. 242, 116389. doi:10.1016/j.carbpol.2020.116389
Chan, S. Y., Choo, W. S., Young, D. J., and Loh, X. J. (2017). Pectin as a Rheology Modifier: Origin, Structure, Commercial Production and Rheology. Carbohydr. Polym. 161, 118–139. doi:10.1016/j.carbpol.2016.12.033
Chen, T., Fu, X., Zhang, L., and Zhang, Y. (2019). Viscosity Behavior of P(DAC-AM) with Serial Cationicity and Intrinsic Viscosity in Inorganic Salt Solutions. Polymers 11 (12), 1944. doi:10.3390/polym11121944
El-Mohdy, H. L. A. (2017). Radiation-induced Degradation of Sodium Alginate and its Plant Growth Promotion Effect. Arabian J. Chem. 10, S431–S438. doi:10.1016/j.arabjc.2012.10.003
Fang, Y., Al-Assaf, S., Phillips, G. O., Nishinari, K., Funami, T., Williams, P. A., et al. (2007). Multiple Steps and Critical Behaviors of the Binding of Calcium to Alginate. J. Phys. Chem. B 111, 2456–2462. doi:10.1021/jp0689870
Feng, L., Cao, Y., Xu, D., Wang, S., and Zhang, J. (2017). Molecular Weight Distribution, Rheological Property and Structural Changes of Sodium Alginate Induced by Ultrasound. Ultrason. Sonochem. 34, 609–615. doi:10.1016/j.ultsonch.2016.06.038
Gawkowska, D., Cybulska, J., and Zdunek, A. (2018). Structure-Related Gelling of Pectins and Linking with Other Natural Compounds: A Review. Polymers 10 (7), 762. doi:10.3390/polym10070762
Ghobashy, M. M., and Bassioni, G. (2018). pH Stimuli-Responsive Poly(acrylamide-Co -sodium Alginate) Hydrogels Prepared by γ-radiation for Improved Compressive Strength of concrete. Adv. Polym. Technol. 37, 2123–2133. doi:10.1002/adv.21870Citations:9
Ghorbani Gorji, E., Waheed, A., Ludwig, R., Toca-Herrera, J. L., Schleining, G., and Ghorbani Gorji, S. (2018). Complex Coacervation of Milk Proteins with Sodium Alginate. J. Agric. Food Chem. 66, 3210–3220. doi:10.1021/acs.jafc.7b03915
H. Gulrez, S. K., Al-Assaf, S., and O, G. (2011). Hydrogels: Methods of Preparation, Characterisation and Applications. Prog. Mol. Environ., 117–150. doi:10.5772/24553
Hadar, J., Skidmore, S., Garner, J., Park, H., Park, K., Wang, Y., et al. (2019). Characterization of Branched Poly(lactide-Co-Glycolide) Polymers Used in Injectable, Long-Acting Formulations. J. Controlled Release 304, 75–89. doi:10.1016/j.jconrel.2019.04.039
Jiao, W., Chen, W., Mei, Y., Yun, Y., Wang, B., Zhong, Q., et al. (2019). Effects of Molecular Weight and Guluronic Acid/Mannuronic Acid Ratio on the Rheological Behavior and Stabilizing Property of Sodium Alginate. Molecules 24 (23), 4374. doi:10.3390/molecules24234374
La Mantia, F. P., Morreale, M., Botta, L., Mistretta, M. C., Ceraulo, M., and Scaffaro, R. (2017). Degradation of Polymer Blends: A Brief Review. Polym. Degrad. Stab. 145, 79–92. doi:10.1016/j.polymdegradstab.2017.07.011
Malerba, M., and Cerana, R. (2019). Recent Applications of Chitin- and Chitosan-Based Polymers in Plants. Polymers 11 (5), 839. PMID: 31072059. doi:10.3390/polym11050839
Masimov, E. A., Pashayev, B. G., Gasanov&Gadzhieva, G. S. Sh. N., and Gadzhieva, S. N. (2019). Viscometric Determination of the Conformations and Sizes of Polyethylene Glycol Macromolecules in Aqueous Solutions. Russ. J. Phys. Chem. 93, 1054–1058. doi:10.1134/S0036024419060207
Milani, P., França, D., Balieiro, A. G., and Faez, R. (2017). Polymers and its Applications in Agriculture. Polímeros 27 (3), 256–266. doi:10.1590/0104-1428.09316
Mollah, M. Z. I., Khan, M. A., and Khan, R. A. (2009). Effect of Gamma Irradiated Sodium Alginate on Red Amaranth (Amaranthus Cruentus L.) as Growth Promoter. Radiat. Phys. Chem. 78 (1), 61–64. doi:10.1016/j.radphyschem.2008.08.002
Mudgil, D., Barak, S., Patel, A., and Shah, N. (2018). Partially Hydrolyzed Guar Gum as a Potential Prebiotic Source. Int. J. Biol. Macromolecules 112, 207–210. ISSN 0141-8130. doi:10.1016/j.ijbiomac.2018.01.164
Olad, A., Pourkhiyabi, M., Gharekhani, H., and Doustdar, F. (2018). Semi-IPN Superabsorbent Nanocomposite Based on Sodium Alginate and Montmorillonite: Reaction Parameters and Swelling Characteristics. Carbohydr. Polym. 190, 295–306. ISSN 0144-8617. doi:10.1016/j.carbpol.2018.02.088
Pathak, V., and Navneet, M. (2017). Review on the Current Status of Polymer Degradation: a Microbial Approach. Bioresour. Bioproc. 4, 15. doi:10.1186/s40643-017-0145-9
Rizwan, M., Yahya, R., Hassan, A., Yar, M., Azzahari, A., Selvanathan, V., et al. (2017). pH Sensitive Hydrogels in Drug Delivery: Brief History, Properties, Swelling, and Release Mechanism, Material Selection and Applications. Polymers 9 (4), 137. doi:10.3390/polym9040137
Sariyerli, G. S., Sakarya, O., and Akcadag, U. Y. (2018). Comparison Tests for the Determination of the Viscosity Values of Reference Liquids by Capillary Viscometers and Stabinger Viscometer SVM 3001. Int. J. Metrol. Qual. Eng. 9, 7. doi:10.1051/ijmqe/2018004
Sehgal, R., Mehta, A., and Gupta, R. (2019). “Alginates: General Introduction and Properties,” in Alginates: Applications in the Biomedical and Food Industries. Editor S Ahmed, 3, 1–20. ISBN: 978-1-119-48791-3. doi:10.1002/9781119487999.ch1
Shivakumara, L. R., and Demappa, T. (2019). Synthesis and Swelling Behavior of Sodium Alginate/Poly(vinyl Alcohol) Hydrogels. tjps 16 (3), 252–260. doi:10.4274/tjps.galenos.2018.92408
Song, R., Murphy, M., Li, C., Ting, K., Soo, C., and Zheng, Z. (2018). Current Development of Biodegradable Polymeric Materials for Biomedical Applications. Dddt 12, 3117–3145. doi:10.2147/DDDT.S165440
Szekalska, M., Puciłowska, A., Szymańska, E., Ciosek, P., and Winnicka, K. (2016). Alginate: Current Use and Future Perspectives in Pharmaceutical and Biomedical Applications. Int. J. Polym. Sci. 2016, 1–17. Hindawi Limited. doi:10.1155/2016/7697031
Tally, M., and Atassi, Y. (2015). Optimized Synthesis and Swelling Properties of a pH-Sensitive Semi-IPN Superabsorbent Polymer Based on Sodium Alginate-G-Poly(acrylic Acid-Co-Acrylamide) and Polyvinylpyrrolidone and Obtained via Microwave Irradiation. J. Polym. Res. 22, 181. doi:10.1007/s10965-015-0822-3
Tamada, M. (2018). “Radiation Processing of Polymers and its Applications,” in Radiation Applications. An Advanced Course in Nuclear Engineering. Editor H Kudo (Singapore: Springer), 07, 63–80. doi:10.1007/978-981-10-7350-2_8
Uddin, I., Islam, J. M. M., Haque, A., Zubair, A., Barua, R., Rahaman, S., et al. (2020). Significant Influence of Gamma-Radiation-Treated Chitosan and Alginate on Increased Productivity as Well as Improved Taste and Flavor of Pineapple. Int. J. Fruit Sci. 20, 455–469. doi:10.1080/15538362.2020.1740909
Urbanova, M., Pavelkova, M., Czernek, J., Kubova, K., Vyslouzil, J., Pechova, A., et al. (2019). Interaction Pathways and Structure-Chemical Transformations of Alginate Gels in Physiological Environments. Biomacromolecules 20 (11), 4158–4170. doi:10.1021/acs.biomac.9b01052
Wu, Z., Wu, J., Zhang, R., Yuan, S., Lu, Q., and Yu, Y. (2018). Colloid Properties of Hydrophobic Modified Alginate: Surface Tension, ζ-potential, Viscosity and Emulsification. Carbohydr. Polym. 181, 56–62. ISSN 0144-8617. doi:10.1016/j.carbpol.2017.10.052
Keywords: alginate (ALG), irradiation, molecular weight (Mw), reduction, sterilization, characterization
Citation: Mollah MZI, Rahaman MS, Faruque MRI, Khandaker MU, Osman H, Alamri S and Al-Assaf S (2021) Effects of Radiation sterilization Dose on the Molecular Weight and Gelling Properties of Commercial Alginate Samples. Front. Mater. 8:761995. doi: 10.3389/fmats.2021.761995
Received: 20 August 2021; Accepted: 12 November 2021;
Published: 20 December 2021.
Edited by:
Ravin Narain, University of Alberta, CanadaReviewed by:
Weifeng Zhao, Sichuan University, ChinaKunyu Zhang, Johns Hopkins University, United States
Copyright © 2021 Mollah, Rahaman, Faruque, Khandaker, Osman, Alamri and Al-Assaf. This is an open-access article distributed under the terms of the Creative Commons Attribution License (CC BY). The use, distribution or reproduction in other forums is permitted, provided the original author(s) and the copyright owner(s) are credited and that the original publication in this journal is cited, in accordance with accepted academic practice. No use, distribution or reproduction is permitted which does not comply with these terms.
*Correspondence: M. Z. I. Mollah, emFoaXJ1bDE5NzNAeWFob28uY29t