- Faculty of Dentistry, Oral Biology Department, King Abdulaziz University, Jeddah, Saudi Arabia
Magnesium (Mg) is an essential trace element that has a significant role in the human body through its effects on bone metabolism. It has various applications in orthodpaedics and dentistry and the interest of this systematic review lies in its potential role as a dental implant surface coating. The dental implants can fail at different stages starting with its osseointegration phase to the restorative stage in the oral cavity. The biological loss of bone integration to the implant surface has been classified as one of the primary reasons for dental implant failure. There have been numerous strategies that have been shown to compensate this reason for implant failure, among which are the dental implant surface coatings. These coatings have been shown to improve the enhance the adhesion as well as the process of osseointegration. There are numerous studies in the existing literature that have analyzed the effects of Mg-based coatings on cellular as well as biological processes in bone-implant integration. A systematic search of various databases yielded 175 articles, of which 14 in vitro and experimental animal studies that analyzed the effect of Mg-based coatings and compared it to other coatings or no surface coatings were included in this systematic review. The main outcomes of this systematic review have been cellular behavior, osseointegration, and osteogenic markers and the effects of Mg-based coatings in these parameters have been highlighted in this review.
Introduction
Titanium (Ti) and its alloys have become the mainstay biomaterials for dental implants for their mechanical properties and excellent biocompatibility. But, these metals can get corroded in a biological environment and toxic reactions may occur (Tschernitschek et al., 2005; Niinomi, 2008). Zirconia (Zir) implants were extensively used for their increased corrosion resistance, good biocompatibility, favorable esthetic and good mechanical properties. Even though both materials have load-bearing applications with their unique properties, the main challenge in implant dentistry remains to be osseointegration. Dental implants of both metal and ceramic materials are prone to failure due to insufficient integration to bone. It may result in the formation of a fibrous tissue or weak bone formation that may cause infections or unstable implants prone to surgical removal (Best et al., 2008; Liang et al., 2007; Brohede et al., 2009).
In present day implantology, osseointegration is well-researched and predictable but it is also reliant on the case selection and the surgical procedure or loading protocol based on the stage of failure despite the successful clinical outcomes of dental implants (Albrektsson, 1998). The literature suggests that there are two mechanisms by which surface modifications on implants can influence osseointegration and they include: 1) biomechanical interlocking where there is bone growth into the rough surface of the implant and 2) biochemical interaction and bonding at the tissue-implant junction (Albrektsson and Wennerberg, 2004). For enhanced osseointegration, three factors have to be considered and they include 1) Improving macro-retentive features in dental implants such as screw/thread design or solid body press fit, and sintered bead technologies, 2) Improving micro-retentive features such as “surface roughness” of the implant to favor cellular and molecular mechanisms for bone growth along the implant surface, and 3) Surface modification that results in a topography favorable for the differentiation of cells that enable an osseous interface on the surface of implants (Stanford, 2008).
Surface coatings on titanium and zirconia dental implants offer the best approach to improving the rate of implant-bone integration and enhancing adhesion to other materials in restorations. It offers dual benefits with improved micromechanical retention and alteration of the surface chemistry for activation of biological processes that favor bone growth (Ying Kei Lung, 2017). There are various techniques that can be used to generate a surface coating on dental implants and they include plasma spraying, sol-gel processing, grit blasting, sand blasting with acid etching, laser etching, ion implantation and sputtering. There is consistent research with both plasma spraying and acid etching techniques (Jemat et al., 2015). Some of the materials that can be coated on the implant surface include hydroxyapatite (HA), ceramics, calcium phosphate, bioactive glass, and fluoride. Grit blasting and sol-gel techniques promote adhesion to restoration whereas materials like bioactive glass and HA promote osseointegration (Lung and Matinlinna, 2012; CattaniLorente et al., 2010; Hench, 2002; Darimont et al., 2002). The downside of materials like bioactive glass and HA include poor mechanical strength, brittleness, and bacterial infections around implants, so the quest for newer biomaterials is on the rise with research targets on materials having antibacterial activity, improving osseointegration outcomes that in turn can translate into long-term clinical success (Ying Kei Lung, 2017).
The alteration of implant surface chemical characteristics comprises a more significant approach in improving the biological activity of dental implants and trace elements like calcium, zinc, silicon, magnesium, and strontium have been commonly used as surface coatings for dental implants (Sawada et al., 2013; Hass et al., 2012; Yu et al., 2013; Park et al., 2010; Shi et al., 2012). Various studies have shown their positive influence on osteoblastic activity and enhance bone growth along with improved bone healing (Boanini et al., 2010; Castellani et al., 2011; Hoppe et al., 2011). Magnesium (Mg) is the fourth most abundant cation in the human body and with the total physiologic Mg, half of it being stored in mineralized bone tissue (Staiger et al., 2006). Magnesium has been shown to play a critical role in bone metabolism and it can interact with integrins on the surface of osteoblasts, thereby promoting its cell stability and adhesion properties (Zreiqat et al., 2002; Yamasaki et al., 2002).
There are numerous studies in the existing literature that have shown the effects of magnesium coatings on titanium or zirconia implants but there is a lack of a systematic approach towards the reporting of its properties with respect to cell bioactivity, osseointegration, or bone regeneration. Thus, the aim of this systematic review was to collate the evidence on magnesium coatings on titanium or zirconia implants, comparisons with conventional or no surface coatings, and to analyze its effects on outcomes like cell behavior, osseointegration, and markers of osteogenesis.
Materials and Methods
According to the Preferred Reporting Items for Systematic Reviews and Meta Analyses (PRISMA) guidelines, the focused question was constructed in the Population Intervention Control Outcomes (PICO) format and it was What is the effect of magnesium-based coatings in Ti or Zir implants on osseointegration, bioactivity, and markers of osteogenesis.
Literature Search
A combination of keywords like “dental implant”, “magnesium”, “implant coatings, and “osseointegration” were used in an electronic search in databases like Pubmed/Medline, Embase, Google Scholar, and Cochrane library. The search was performed using the PICO format and it was performed individually for the keywords and combined with “and” and “or” terms.
Pubmed/Medline: Search: (dental implant) or (titanium implant) or (zirconia implant) or (Ti dental implant) or (dental implant surface coating) or (surface coating) and (magnesium) or (magnesium oxide) or (magnesium carbonate) or (magnesium fluoride) or (magnesium based surface coating) and (ceramics) or (bioactive glass) or (fluoride) or (ceramics) or (hydroxyapatite) or (calcium phosphate) and (cell adhesion) or (cell proliferation) or (cell differentiation) or (bioactivity) or (osteoblast) or (fibroblast) or (osseointegration)
A manual search was performed to include articles that were relevant to the topic of the systematic review. The bibliographies from the full-texts of the articles were manually searched for relevant articles to be included in this review. The search timeline included articles where the focus question was addressed and it was between the years 2000 and 2019.
Eligibility Criteria
This systematic review aimed to analyze the effects of magnesium-based implant coatings on osseointegration and bone regeneration at cellular level, so animal and in vitro studies were included in the search along with studies that were conducted in humans that had observed the desired outcomes. Only articles published in English language were included in this review. The exclusion criteria included articles which had no relevance to dentistry and only to orthopedic applications, reviews, and letters to the editor. Also, articles that observed the outcome measures in non-titanium materials like magnesium implants and the coatings were excluded from this review. The focused question was used to analyze the included studies and the relevant information on outcome measures were extracted. A flowchart has been depicted on the search methodology employed in this systematic review (Figure 1).
Data Extraction
Two independent reviewers (XX, YY) conducted the literature search employing the keywords and MeSh terms, following which the articles were analyzed based on the eligibility criteria. If there was disagreement on the inclusion of certain articles, a discussion was held to resolve it. In instances where a study did not report raw data relevant to the outcome measures, the data was extracted from either the tables or graphical representations in the study. The corresponding authors were contacted to solve doubts or provide missing information relevant to the systematic review. The data extraction tables have been attached (Supplementary file).
Risk of Bias Assessment of Included Studies
The quality assessment for each animal study was carried out using the SYRCLE’s RoB tool that has 10 items and two independent authors (XX, YY) performed risk assessment across the following categories: Sequence generation, allocation concealment, blinding of investigators and outcome assessors, random housing for animals, selective reporting of outcome measures, addressing incomplete outcome, and other potential risks for bias. The risk was categorized either as “high-risk”, “low-risk” or “unclear risk” (Hooijmans et al., 2014). A third independent author (ZZ) resolved any disagreements on risk assessment with a discussion. In the existing literature, there are no tools or indices for the validation of in vitro studies and the risk of bias is quite low due to its position in the hierarchy of evidence-based dentistry (Richards, 2009).
Results
Search Results
The systematic search of the literature yielded a total of 175 articles of which 22 articles were identified to be satisfying the eligibility criteria. The manual search of relevant journals and bibliography yielded two articles that were included in this systematic review. Some articles were excluded after full text assessment and a total of 14 articles were included for evaluation in this review. Among the included articles, nine were in vitro studies (Gorrieri et al., 2006; Jiang et al., 2014; Mihailescu et al., 2016; Onder et al., 2018; Pardun et al., 2015; (Park et al., 2013; Won et al., 2017; Yu et al., 2017; Xie et al., 2009), four experimental animal models (Sul et al., 2006; Cho et al., 2010; Li et al., 2014; Tao et al., 2016) and one study was part animal model and part in vitro design (Zhao et al., 2013). The characteristics and the main outcome measures of the included studies have been shown in (Table 1).
In vitro Studies
The in vitro studies included in this systematic review observed the effects of various magnesium-based coating like calcium magnesium carbonate, magnesium fluoride (MgF2), magnesium oxide (MgO), magnesium silicate, HA incorporated with Mg or MgO, zinc and magnesium co-implanted titanium surface, along with effects of varying concentrations of Mg on osseointegration or bone regeneration. The cell lines used in the in vitro studies include human, canine, and rat bone marrow mesenchymal stem cells (hBM-MSCs, cBMSCs, rBMSCs), MG-63 osteoblast-like cells, MC3T3-E1, Hep-2 epithelial cells, and human umblical vein endothelial cells (HUVECs) (Gorrieri et al., 2006; Jiang et al., 2014; Mihailescu et al., 2016; Onder et al., 2018; Pardun et al., 2015; (Park et al., 2013; Won et al., 2017; Yu et al., 2017; Xie et al., 2009; Zhao et al., 2013). The results of the in vitro studies have been summarized in (Table 2) and analysis of individual outcomes have been explained below.
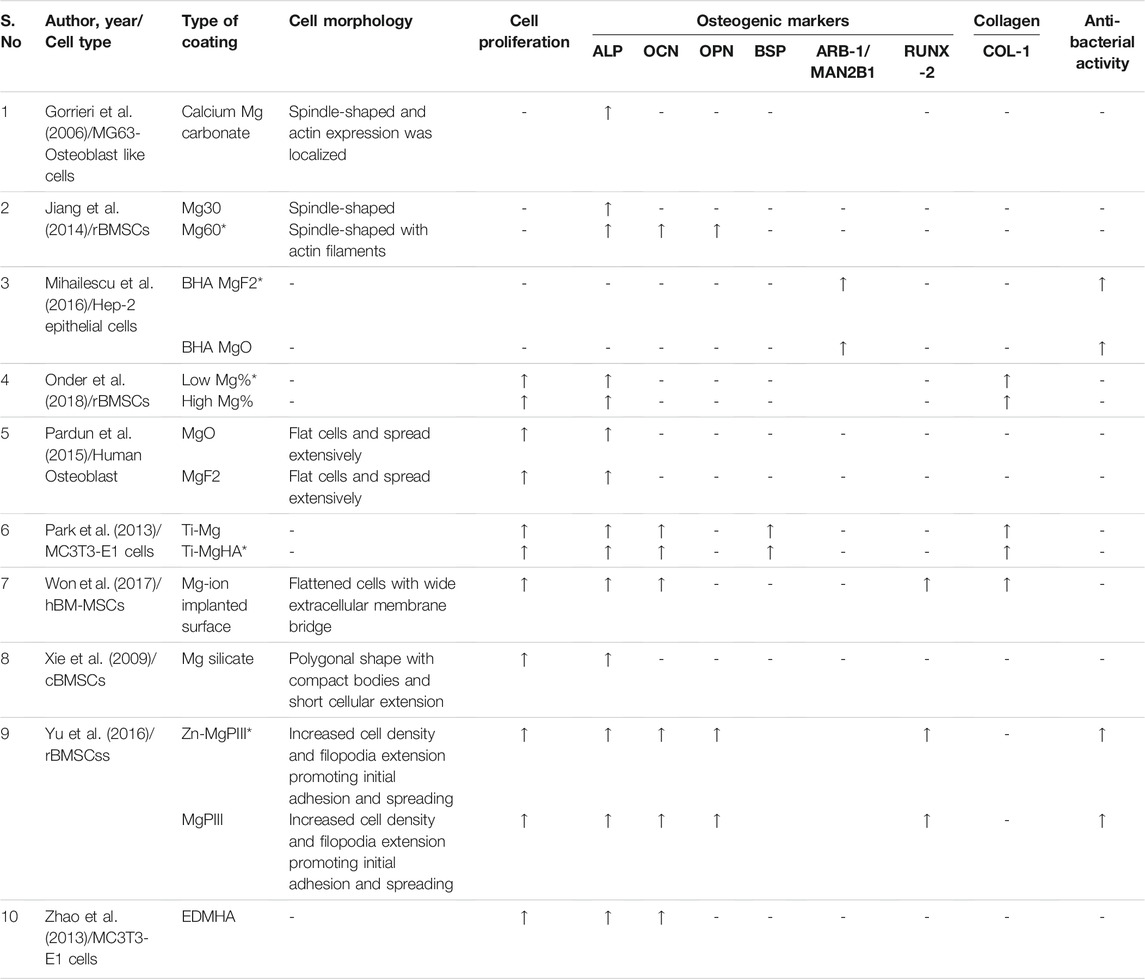
TABLE 2. Summary of results of in vitro studies observed for main outcome measures in Magnesium-based coatings.
Cellular Morphology
A total of six out of ten included in vitro studies observed the effect of Mg-based coatings on the cellular morphology of either pre-osteoblasts or bone marrow mesenchymal stem cells. The cells were either spindle-shaped or flattened in morphology with filopodia extensions and actin localization for the promotion of initial adhesion and spreading (Gorrieri et al., 2006; Jiang et al., 2014; Pardun et al., 2015; Won et al., 2017; Yu et al., 2017). An exception was the study conducted by Xie et al., where the observed cells were polygonal in shape with compact bodies and short cellular extensions (Xie et al., 2009).
Cellular Proliferation
In this systematic review, a total of seven out of ten included studies observed the effect of Mg-based coatings on cellular proliferation and found that there was a substantial increase in proliferation in the studied cell-lines. The surface coatings include MgO, MgF2, MgHA, Mg-ions, Zn-Mg co-implanted, and magnesium silicate. There was a relatively higher increase in surface coatings that include MgHA, Low Mg concentration, and Zn-Mg co-implanted surfaces when compared to their respective control groups (Onder et al., 2018; Pardun et al., 2015; Park et al., 2013; Won et al., 2017; Yu et al., 2017; Xie et al., 2009; Zhao et al., 2013).
Osteogenic Markers
All the included studies in the systematic review observed the expression of osteogenic markers using polymerase chain reaction and the markers include: 1) alkaline phosphatase (ALP), osteocalcin (OCN), Osteopontin (OPN), bone sialoprotein (BSP), arrestin beta-1 (ARB-1), mannosidase alpha class-2B (MAN2B-1), and runt-related transcription factor-2 (RUNX-2). The observed osteogenic markers were significantly elevated with the Mg-based coatings analyzed in the respective studies (Gorrieri et al., 2006; Jiang et al., 2014; Mihailescu et al., 2016; Onder et al., 2018; Pardun et al., 2015; Park et al., 2013; Won et al., 2017; Yu et al., 2017; Xie et al., 2009; Zhao et al., 2013).
Collagen Deposition
In three in vitro studies, the deposition of collagen type-I was significantly increased with Ti-Mg, MgHA, Mg-ion implanted surfaces with varying concentrations. There was significant increase with Low Mg% (9.24%) when compared to High Mg%, and also in Mg-HA group on comparison with Ti-Mg group (Onder et al., 2018; Park et al., 2013; Won et al., 2017).
Anti-Bacterial Activity
Two in vitro studies observed the anti-bacterial effect of Mg-based coatings with one study examining bovine-derived HA (BHA) with MgO or MgF2 and the other study observing Mg and Zn-Mg co-implanted Ti surfaces. Yu et al., showed that Zn-Mg co-implanted surfaces had the highest antibacterial effect against microbes like Porphyromonas gingivalis, Fusobacterium nucleatum, and Streptococcus mutans when compared to only Mg-incorporated surfaces (Yu et al., 2017). Mihailescu et al., observed that both BHA:MgO and BHA:MgF2 had 4 times higher inhibitory activity against strains of Enterococcus sp, Micrococcus sp, and Candida albicans (Mihailescu et al., 2016).
Animal Experiments
In the animal-model experimental study conducted by Sul et al., on NZ white rabbits, the osseointegration speed was compared between Mg-ion incorporated oxidized implants and non-coated machine turned implants. There was highly significant difference in the speeds between the test and Mg-ion groups at 3 and 6 weeks with p-value<0.005 and also notable differences in the strengths favoring the Mg-ion group during the same follow-up intervals (Sul et al., 2006). Cho et al., observed the effects of differential concentrations of Mg-ions as surface coating and observed that Mg-1 implants (Mg concentration-9.24%) had the highest removal torque values (RTQ) when compared to the other groups as well as the highest bone-implant contact (BIC) values, bone fill area, and new bone formation when compared to the other groups in the study (Cho et al., 2010).
The other animal studies (n = 3) observed the incorporation of Mg to hydroxyapatite (HA) on titanium surfaces either using sol-gel-dip coating method or electrochemical deposition (Li et al., 2014); (Tao et al., 2016); (Zhao et al., 2013). In all the studies, the control group was pure HA coating whereas one study compared Mg-based coating with HA incorporated with other elements like Zinc (Zn) and Strontium (Sr) (Tao et al., 2016). The analyzed results observed that bone area ratio and BIC values were significantly higher for Mg-HA coating when compared to pure HA titanium surface (Li et al., 2014); (Tao et al., 2016); (Zhao et al., 2013). A micro-CT analysis also observed that trabecular bone structure and osseointegration was significantly improved with Mg-HA coating Li et al., 2014; Tao et al., 2016; Zhao et al., 2013). Li et al., observed during biomechanical testing that Mg-HA coating increased the interfacial shear strength and maximum push-out force when compared to HA coatings (Li et al., 2014). Tao et al., observed some slightly improved results with Sr-HA when compared to Mg-HA in terms of BIC values, bone volume, and bone area ratio (Tao et al., 2016). The summary of results from the animal studies have been tabulated in (Table 3).
Risk of Bias Assessment
In this systematic review, all experimental animal studies were subjected to SYRCLE’s RoB tool for assessing the risk of bias. For each domain in the risk assessment tool, the risk of bias for each study has been summarized in (Figure 2). The 10 risks of bias items belonging to the risk assessment tool have been presented as percentages for all the included experimental studies (Figure 3). The studies presented with relatively higher risk of bias for random sequence generation, allocation concealment and blinding whereas the randomization and blinding of outcome assessments, selective reporting of outcome data, and other potential bias were poorly described in the studies. A significantly lower risk of bias was observed for reporting of baseline characteristics and reporting of incomplete outcome data among the included studies.
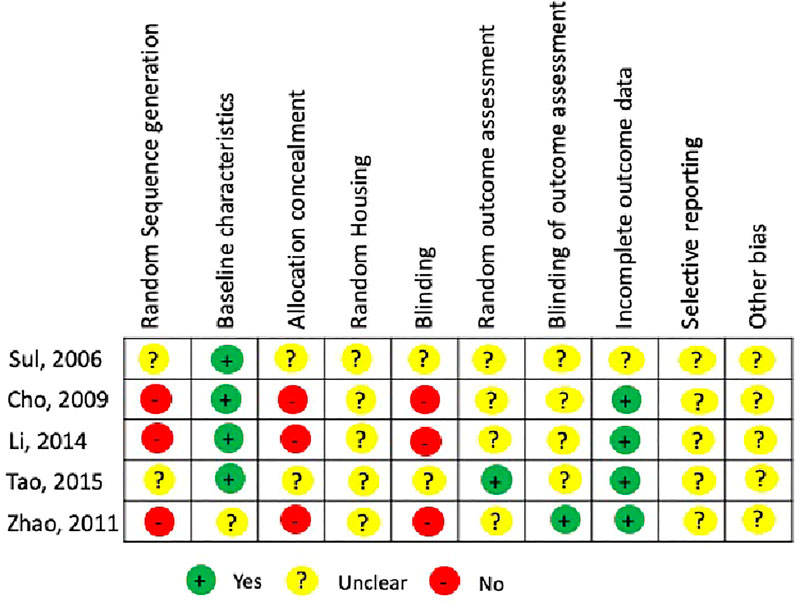
FIGURE 2. Risk assessment using SYRCLE RoB tool for individual animal experimental studies included in the systematic review.
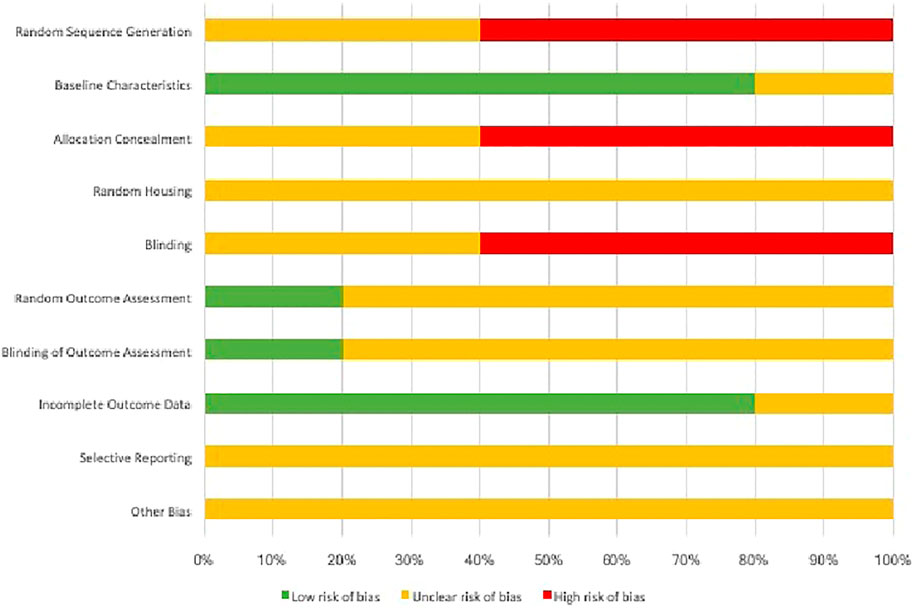
FIGURE 3. Cumulative risk assessment of the included studies in the systematic review using individual items in SYRCLE RoB tool.
Discussion
One of the essential trace elements for both animals and humans is Mg due to its influence on physiologic activities and bone metabolism. The deficiency of Mg in the diet can cause reduced bone mass and deranges the mineral and bone metabolic pathways in rats (Rude et al., 2006). In the ideal scenario, biomaterials must influence the proliferation and differentiation of the targeted cell types to stimulate formation of functional tissue (Sader et al., 2009). In the existing literature, there have been studies that show the positive effect of Mg incorporation into Ti implants with stimulated bone formation and osseointegration (Revell et al., 2004; Pang et al., 2014). There are some lacunae in research that can show the cumulative effects of Mg as surface coating on dental implants. Thus, in this systematic review, the focused question was the effect of various Mg-based coatings on Ti or Zir implants in terms of cell behavior, osteogenic markers, and on the process of osseointegration.
A total of 14 studies of both in vitro and experimental animal model design were included in this review based on the eligibility criteria. The results from the animal models were based on histologic or micro-CT analysis and observations were made in relation to bone-related parameters and osseointegration. It was shown that Mg-based coatings significantly increased the BIC values, bone area ratio, and bone volumes as well as improved speed of osseointegration and higher RTQ values (Sul et al., 2006; Cho et al., 2010; Li et al., 2014; Tao et al., 2016; Zhao et al., 2013). The process of osseointegration is reflected based on the evolution of bone growth and integration in the peri-implant tissues (Yu et al., 2016). It is well known that bone formation and integration are quantitatively measured in terms of BIC and bone area and BIC values are known to have a strong association with the strength of the bone-implant surface (Yu et al., 2016; Zhou et al., 2008). It is pivotal to note the superior bone contact observed in Mg-based coatings on Ti/Zir surfaces when compared to other observed surface coatings such as Resorbable Blasting Materials (RBM-HA, Beta-tricalcium phosphate), HA, ZnHA or groups with no implant surface coating (Sul et al., 2006; Cho et al., 2010; Li et al., 2014; Tao et al., 2016; Zhao et al., 2013).
The role of BMSCs (osteoblast precursor) and osteoblast cells cannot be emphasized more as their initial adhesion and subsequent proliferation can have a direct effect on cellular functionality and the process of osseointegration (Jiang et al., 2013). From the in vitro studies, it was observed that the cells were flattened or spindle-shaped with cellular extensions that facilitate adhesion and spreading on Mg-containing surfaces (Gorrieri et al., 2006; Jiang et al., 2014; Pardun et al., 2015; Won et al., 2017; Yu et al., 2017). The presence of actin filaments in spindle-shaped cells was suggestive of the migratory attitude of the osteoblasts. The polygonal shape with cytoplasmic processes enabled the cells to spread to reach larger sizes on the coating and they were found to be closely adherent to the coating (Gorrieri et al., 2006; Xie et al., 2009). Also, it was observed that there was increased cellular proliferation and cell viability with increased levels of osteogenic markers such as ALP, OCN, OPN, BSP, RUNX-2 etc influenced by the presence of Mg in the implant surface coating (Gorrieri et al., 2006; Jiang et al., 2014; Mihailescu et al., 2016; Onder et al., 2018; Pardun et al., 2015; Park et al., 2013; Won et al., 2017; Yu et al., 2017; Xie et al., 2009; Zhao et al., 2013). There was also increased deposition of Type-I collagen which is an essential component of the extracellular matrix and increased Ca deposition that can contribute to bone mineralization (Onder et al., 2018; Park et al., 2013; Won et al., 2017).
The success of dental implants is not only dependent on osseointegration but on the disruption of the microbial biofilm that can affect the health of the peri-implant tissues. Various organisms like Prevotella intermedia, Porphromonas gingivalis, Actinobacillus actinomycetemcomitans have been identified as etiological factors that can result in peri-implantitis where there is a pathological loss of peri-implant supporting tissues (Leonhardt et al., 1999; Schmidlin et al., 2013). This has warranted research towards newer and effective strategies on antibacterial surface coatings to prevent microbial adhesion and colonization on dental implants (Holban et al., 2014). Two studies in this systematic review observed the anti-bacterial effect of Mg-based coatings and there was significantly higher inhibitory effect with these coatings on microbes like P. gingivalis, F. nucleatum, S. mutans, Micrococcus sp, Enterococcus sp, and Candida albicans (Mihailescu et al., 2016; Yu et al., 2017). But, the results of the study conducted by Yu et al., suggested that the Zn portion of Zn/Mg co-implanted surface could have contributed to the inhibitory effect because of Zn ions being established in the suppression of microbial adhesion and production of reactive oxygen species that are detrimental to the oral anaerobes (Yu et al., 2017). The study conducted by Mihailescu et al., suggested that BHA:MgO or BHA:MgF2 have anti-biofilm properties based on the hypothesis that these coatings exhibited bactericidal properties by killing cells during or after the contact with the coated surfaces (Mihailescu et al., 2016).
The potential limitation in this systematic review could be that the studies belong to the lower level of evidence (animal-model and in vitro design) and the quality assessment of the included studies revealed that there could be many potential sources of bias. The included studies had considerable heterogeneity and in most of the animal studies, the steps of randomization and blinding were not performed and some studies did not clearly explain the experimental protocol which is a potential confounding factor. The potential bias existing in these studies could have contributed to the positive outcomes obtained in it. There is a heterogeneity among the included studies in methodology, but the corresponding authors of the studies have been contacted for clarification or for providing any missing information relevant to performing to this review. Some disadvantages noted with Mg alloys include less corrosion resistance, lesser elastic modulus, but none has been identified yet with Mg coatings (Chakraborty Banerjee et al., 2019). But, from this systematic review, it is clear that there is a positive effect with Mg-based coatings on osteogenic activity and osseointegration in dental implants and these results could be ascertained as preliminary as the data was extracted mainly from in vitro and experimental animal models. However, future research should be targeted with more well-designed and non-biased clinical studies that focus on confirming these lab-oriented results.
Conclusion
From this systematic review of literature, the results from the in vitro studies show that Mg-based coatings improve the cellular behavior in terms of morphology and proliferation with increased expression of osteogenic markers and considerable antimicrobial activity. From the animal studies, it can be deduced that there was higher bone fill and BIC values with significant new bone formation. Even though the results are promising, there is considerable heterogeneity among the included studies, so clinical trials are warranted to provide compelling observations for outcomes that determine the long-term clinical success of dental implants.
Data Availability Statement
The raw data supporting the conclusions of this article will be made available by the authors, without undue reservation.
Author Contributions
Designing the search, analyzing the studies, analyzing results, writing manuscript and approval.
Conflict of Interest
The author declares that the research was conducted in the absence of any commercial or financial relationships that could be construed as a potential conflict of interest.
Publisher’s Note
All claims expressed in this article are solely those of the authors and do not necessarily represent those of their affiliated organizations, or those of the publisher, the editors and the reviewers. Any product that may be evaluated in this article, or claim that may be made by its manufacturer, is not guaranteed or endorsed by the publisher.
References
Albrektsson, T. (1998). Hard Tissue Response. Handbook of Biomaterial Properties. Boston, MA: Springer, 500–512. doi:10.1007/978-1-4615-5801-9_29
Albrektsson, T., and Wennerberg, A. (2004). Oral Implant Surfaces: Part 1--review Focusing on Topographic and Chemical Properties of Different Surfaces and In Vivo Responses to Them. Int. J. Prosthodont. 17 (5), 536–543.
Best, S. M., Porter, A. E., Thian, E. S., and Huang, J. (2008). Bioceramics: Past, Present and for the Future. J. Eur. Ceram. Soc. 28 (7), 1319–1327. doi:10.1016/j.jeurceramsoc.2007.12.001
Boanini, E., Gazzano, M., and Bigi, A. (2010). Ionic Substitutions in Calcium Phosphates Synthesized at Low Temperature. Acta Biomater. 6 (6), 1882–1894. doi:10.1016/j.actbio.2009.12.041
Brohede, U., Zhao, S., Lindberg, F., Mihranyan, A., Forsgren, J., Strømme, M., et al. (2009). A Novel Graded Bioactive High Adhesion Implant Coating. Appl. Surf. Sci. 255 (17), 7723–7728. doi:10.1016/j.apsusc.2009.04.149
Castellani, C., Lindtner, R. A., Hausbrandt, P., Tschegg, E., Stanzl-Tschegg, S. E., Zanoni, G., et al. (2011). Bone-Implant Interface Strength and Osseointegration: Biodegradable Magnesium Alloy Versus Standard Titanium Control. Acta Biomater. 7 (1), 432–440. doi:10.1016/j.actbio.2010.08.020
Cattani Lorente, M., Scherrer, S. S., Richard, J., Demellayer, R., Amez-Droz, M., and Wiskott, H. W. A. (2010). Surface Roughness and EDS Characterization of a Y-TZP Dental Ceramic Treated With the CoJet Sand. Dental Mater. 26 (11), 1035–1042. doi:10.1016/j.dental.2010.06.005
Chakraborty Banerjee, P., Al-Saadi, S., Choudhary, L., Harandi, S. E., and Singh, R. (2019). Magnesium Implants: Prospects and Challenges. Materials. 12 (1), 136. doi:10.3390/ma12010136
Cho, L-R., Kim, D-G., Kim, J-H., Byon, E-S., Jeong, Y-S., and Park, C-J. (2010). Bone Response of Mg Ion-Implanted Clinical Implants with the Plasma Source Ion Implantation Method. Clin. Oral Implants Res. 21, 848. doi:10.1111/j.1600-0501.2009.01862.x
Darimont, G. L., Cloots, R., Heinen, E., Seidel, L., and Legrand, R. (2002). In Vivo Behaviour of Hydroxyapatite Coatings on Titanium Implants: a Quantitative Study in the Rabbit. Biomaterials. 23 (12), 2569–2575. doi:10.1016/s0142-9612(01)00392-1
Gorrieri, O., Fini, M., Kyriakidou, K., Zizzi, A., Mattioli-Belmonte, M., Castaldo, P., et al. (2006). In Vitro Evaluation of Bio-Functional Performances of Ghimas Titanium Implants. Int. J. Artif. Organs. 29 (10), 1012–1020. doi:10.1177/039139880602901012
Hass, J. L., Garrison, E. M., Wicher, S. A., Knapp, B., Bridges, N., Mcllroy, D., et al. (2012). Synthetic Osteogenic Extracellular Matrix Formed by Coated Silicon Dioxide Nanosprings. J. Nanobiotechnology. 10 (1), 6. doi:10.1186/1477-3155-10-6
Hench, L. L. (2002). Third-Generation Biomedical Materials. Science. 295 (5557), 1014–1017. doi:10.1126/science.1067404
Holban, A.-M., Bleotu, C., Chifiriuc, M. C., Bezirtzoglou, E., and Lazar, V. (2014). Role ofPseudomonas Aeruginosaquorum Sensing (QS) Molecules on the Viability and Cytokine Profile of Human Mesenchymal Stem Cells. Virulence. 5 (2), 303–310. doi:10.4161/viru.27571
Hooijmans, C. R., Rovers, M. M., de Vries, R. B., Leenaars, M., Ritskes-Hoitinga, M., and Langendam, M. W. (2014). SYRCLE’s Risk of Bias Tool for Animal Studies. BMC Med. Res. Methodol. 14 (1), 14–43. doi:10.1186/1471-2288-14-43
Hoppe, A., Güldal, N. S., and Boccaccini, A. R. (2011). A Review of the Biological Response to Ionic Dissolution Products From Bioactive Glasses and Glass-Ceramics. Biomaterials. 32 (11), 2757–2774. doi:10.1016/j.biomaterials.2011.01.004
Jemat, A., Ghazali, M. J., Razali, M., and Otsuka, Y. (2015). Surface Modifications and Their Effects on Titanium Dental Implants. Biomed. Res. Int. 2015, 1–11. doi:10.1155/2015/791725
Jiang, X., Wang, G., Li, J., Zhang, W., Xu, L., Pan, H., et al. (2014). Magnesium Ion Implantation on a Micro/Nanostructured Titanium Surface Promotes its Bioactivity and Osteogenic Differentiation Function. Int. J. Nanomedicine. 8, 2387. doi:10.2147/ijn.s58357
Jiang, X., Zhang, w., Li, z., Ling Xu, L., Yuqin Jin, Y., et al. (2013). Effects of a Hybrid Micro/nanorod Topography-Modified Titanium Implant on Adhesion and Osteogenic Differentiation in Rat Bone Marrow Mesenchymal Stem Cells. Int J Nanomedicine. 8, 257. doi:10.2147/ijn.s39357
Leonhardt, Å., Renvert, S., and Dahlén, G. (1999). Microbial Findings at Failing Implants. Clin. Oral Implants Res. 10 (5), 339–345. doi:10.1034/j.1600-0501.1999.100501.x
Li, X., Li, Y., Liao, Y., Li, J., Zhang, L., and Hu, J. (2014). The Effect of Magnesium-Incorporated Hydroxyapatite Coating on Titanium Implant Fixation in Ovariectomized Rats. Int. J. Oral Maxillofac. Implants. 29 (1), 196–202. doi:10.11607/jomi.2893
Liang, H., Wan, Y. Z., He, F., Huang, Y., Xu, J. D., Li, J. M., et al. (2007). Bioactivity of Mg-Ion-Implanted Zirconia and Titanium. Appl. Surf. Sci. 253 (6), 3326–3333. doi:10.1016/j.apsusc.2006.07.038
Lung, C. Y. K., and Matinlinna, J. P. (2012). Aspects of Silane Coupling Agents and Surface Conditioning in Dentistry: An Overview. Dental Mater. 28 (5), 467–477. doi:10.1016/j.dental.2012.02.009
Mihailescu, N., Stan, G. E., Duta, L., Chifiriuc, M. C., Bleotu, C., Sopronyi, M., et al. (2016). Structural, Compositional, Mechanical Characterization and Biological Assessment of Bovine-Derived Hydroxyapatite Coatings Reinforced With MgF 2 or MgO for Implants Functionalization. Mater. Sci. Eng. C. 59, 863–874. doi:10.1016/j.msec.2015.10.078
Niinomi, M. (2008). Mechanical Biocompatibilities of Titanium Alloys for Biomedical Applications. J. Mech. Behav. Biomed. Mater. 1 (1), 30–42. doi:10.1016/j.jmbbm.2007.07.001
Onder, S., Calikoglu-Koyuncu, A. C., Kazmanli, K., Urgen, M., Kok, F. N., and Torun-Kose, G. (2018). Magnesium Doping on TiN Coatings Affects Mesenchymal Stem Cell Differentiation and Proliferation Positively in a Dose-Dependent Manner. Biomed Mater Eng. 29 (4), 427–438. doi:10.3233/bme-181000
Pang, K.-M., Lee, J.-W., Lee, J.-Y., Lee, J.-B., Kim, S.-M., Kim, M.-J., et al. (2014). Clinical Outcomes of Magnesium-Incorporated Oxidised Implants: a Randomised Double-Blind Clinical Trial. Clin. Oral Impl. Res. 25 (5), 616–621. doi:10.1111/clr.12091
Pardun, K., Treccani, L., Volkmann, E., Streckbein, P., Heiss, C., Gerlach, J. W., et al. (2015). Magnesium-containing Mixed Coatings on Zirconia for Dental Implants: Mechanical Characterization and In Vitro Behavior. J. Biomater. Appl. 30 (1), 104–118. doi:10.1177/0885328215572428
Park, J.-W., Kim, H.-K., Kim, Y.-J., Jang, J.-H., Song, H., and Hanawa, T. (2010). Osteoblast Response and Osseointegration of a Ti-6Al-4V alloy Implant Incorporating Strontium. Acta Biomater. 6 (7), 2843–2851. doi:10.1016/j.actbio.2010.01.017
Park, K.-D., Lee, B.-A., Piao, X.-H., Lee, K.-K., Park, S.-W., Oh, H.-K., et al. (2013). Effect of Magnesium and Calcium Phosphate Coatings on Osteoblastic Responses to the Titanium Surface. J. Adv. Prosthodont. 5 (4), 402. doi:10.4047/jap.2013.5.4.402
Revell, P., Damien, E., Zhang, X., Evans, P., and Howlett, C. (2004). The Effect of Magnesium Ions on Bone Bonding to Hydroxyapatite Coating on Titanium alloy Implants. Key Eng. Mat. 254, 447–450. 10.4028/www.scientific.net/KEM.254-256.447.
Richards, D. (2009). GRADING - Levels of Evidence. Evid. Based Dent. 10 (1), 24–25. doi:10.1038/sj.ebd.6400636
Rude, R. K., Gruber, H. E., Norton, H. J., Wei, L. Y., Frausto, A., and Kilburn, J. (2006). Reduction of Dietary Magnesium by Only 50% in the Rat Disrupts Bone and Mineral Metabolism. Osteoporos. Int. 17 (7), 1022–1032. doi:10.1007/s00198-006-0104-3
Sader, M. S., LeGeros, R. Z., and Soares, G. A. (2009). Human Osteoblasts Adhesion and Proliferation on Magnesium-Substituted Tricalcium Phosphate Dense Tablets. J. Mater. Sci. Mater. Med. 20 (2), 521–527. doi:10.1007/s10856-008-3610-3
Sawada, R., Kono, K., Isama, K., Haishima, Y., and Matsuoka, A. (2013). Calcium‐Incorporated Titanium Surfaces Influence the Osteogenic Differentiation of Human Mesenchymal Stem Cells. J. Biomed. Mater. Res. 101A (9), 2573–2585. doi:10.1002/jbm.a.34566
Schmidlin, P. R., Müller, P., Attin, T., Wieland, M., Hofer, D., and Guggenheim, B. (2013). Polyspecies Biofilm Formation on Implant Surfaces With Different Surface Characteristics. J. Appl. Oral Sci. 21 (1), 48–55. doi:10.1590/1678-7757201302312
Shi, X., Nakagawa, M., Kawachi, G., Xu, L., and Ishikawa, K. (2012). Surface Modification of Titanium by Hydrothermal Treatment in Mg-Containing Solution and Early Osteoblast Responses. J. Mater. Sci. Mater. Med. 23 (5), 1281–1290. doi:10.1007/s10856-012-4596-4
Staiger, M. P., Pietak, A. M., Huadmai, J., and Dias, G. (2006). Magnesium and its Alloys as Orthopedic Biomaterials: A Review. Biomaterials. 27 (9), 1728–1734. doi:10.1016/j.biomaterials.2005.10.003
Stanford, C. (2008). Surface Modifications of Dental Implants. Aust. Dental J. 53 (s1), S26–S33. doi:10.1111/j.1834-7819.2008.00038.x
Sul, Y.-T., Jeong, Y., Johansson, C., and Albrektsson, T. (2006). Oxidized, Bioactive Implants Are Rapidly and Strongly Integrated in Bone. Part 1 - Experimental Implants. Clin. Oral Implants Res. 17 (5), 521–526. doi:10.1111/j.1600-0501.2005.01230.x
Tao, Z.-S., Zhou, W.-S., He, X.-W., Liu, W., Bai, B.-L., Zhou, Q., et al. (2016). A Comparative Study of Zinc, Magnesium, Strontium-Incorporated Hydroxyapatite-Coated Titanium Implants for Osseointegration of Osteopenic Rats. Mater. Sci. Eng. C. 62, 226–232. doi:10.1016/j.msec.2016.01.034
Tschernitschek, H., Borchers, L., and Geurtsen, W. (2005). Nonalloyed Titanium as a Bioinert Metal-Aa Review. Quintessence Int. 36 (7–8), 523–530.
Won, S., Huh, Y.-H., Cho, L.-R., Lee, H.-S., Byon, E.-S., and Park, C. J. (2017). Cellular Response of Human Bone Marrow Derived Mesenchymal Stem Cells to Titanium Surfaces Implanted With Calcium and Magnesium Ions. Tissue Eng. Regen. Med. 14 (2), 123–131. doi:10.1007/s13770-017-0028-3
Xie, Y., Zhai, W., Chen, L., Chang, J., Zheng, X., and Ding, C. (2009). Preparation and In Vitro Evaluation of Plasma-Sprayed Mg2SiO4 Coating on Titanium alloy. Acta Biomater. 5 (6), 2331–2337. doi:10.1016/j.actbio.2009.03.003
Yamasaki, Y., Yoshida, Y., Okazaki, M., Shimazu, A., Uchida, T., Kubo, T., et al. (2002). Synthesis of Functionally Graded MgCO3 Apatite Accelerating Osteoblast Adhesion. J. Biomed. Mater. Res. 62 (1), 99–105. doi:10.1002/jbm.10220
Ying Kei Lung, C. (2017). Surface Coatings of Titanium and Zirconia. Adv. Mater. Sci. [Internet] 2 (2), 1. doi:10.15761/ams.1000124
Yu, J., Li, K., Zheng, X., He, D., Ye, X., and Wang, M. (2013). In Vitro and In Vivo Evaluation of Zinc-Modified Ca-Si-Based Ceramic Coating for Bone Implants. PLoS ONE. 8 (3), e57564. doi:10.1371/journal.pone.0057564
Yu, Y., Ding, T., Xue, Y., and Sun, J. (2016). Osteoinduction and Long-Term Osseointegration Promoted by Combined Effects of Nitrogen and Manganese Elements in High Nitrogen Nickel-Free Stainless Steel. J. Mater. Chem. B. 4 (4), 801–812. doi:10.1039/c5tb02190a
Yu, Y., Jin, G., Xue, Y., Wang, D., Liu, X., and Sun, J. (2017). Multifunctions of Dual Zn/Mg Ion Co-Implanted Titanium on Osteogenesis, Angiogenesis and Bacteria Inhibition for Dental Implants. Acta Biomater. 49, 590–603. doi:10.1016/j.actbio.2016.11.067
Zhao, S.-f., Jiang, Q.-h., Peel, S., Wang, X.-x., and He, F. m. (2013). Effects of Magnesium-Substituted Nanohydroxyapatite Coating on Implant Osseointegration. Clin. Oral Impl. Res. 24, 34–41. doi:10.1111/j.1600-0501.2011.02362.x
Zhou, Y., Jiang, T., Qian, M., Zhang, X., Wang, J., Shi, B., et al. (2008). Roles of Bone Scintigraphy and Resonance Frequency Analysis in Evaluating Osseointegration of Endosseous Implant. Biomaterials. 29 (4), 461–474. doi:10.1016/j.biomaterials.2007.10.021
Keywords: magnesium, dental implant surface coating, osseointegration, osteogenic markers, hydroxyapatite
Citation: Almehmadi AH (2021) Effect of Magnesium-Based Coatings on Titanium or Zirconia Substrates on Bone Regeneration and Implant Osseointegration- A Systematic Review. Front. Mater. 8:754697. doi: 10.3389/fmats.2021.754697
Received: 06 August 2021; Accepted: 19 October 2021;
Published: 01 November 2021.
Edited by:
Kumar Chandan Srivastava, Al Jouf University, Saudi ArabiaReviewed by:
Shivasakthy Manivasakan, Sri Balaji Vidyapeeth University, IndiaRitika Bhambhani, Guru Nanak Institute of Dental Sciences and Research, India
Copyright © 2021 Almehmadi. This is an open-access article distributed under the terms of the Creative Commons Attribution License (CC BY). The use, distribution or reproduction in other forums is permitted, provided the original author(s) and the copyright owner(s) are credited and that the original publication in this journal is cited, in accordance with accepted academic practice. No use, distribution or reproduction is permitted which does not comply with these terms.
*Correspondence: Ahmad H Almehmadi, YWhhbG1laG1hZGlAa2F1LmVkdS5zYQ==