- 1Department of Materials Science and Engineering, Institute of Space Technology, Islamabad, Pakistan
- 2School of Materials Science and Engineering, Northwestern Polytechnical University, Xi’an, China
- 3Department of Metallurgical Engineering, Faculty of Chemical and Process Engineering, NED University of Engineering and Technology, Karachi, Pakistan
Bioceramic coatings on metallic implants provide a wear-resistant and biocompatible layer, that own ability to develop bone-like apatite in physiological environments to ensure bonding with hard tissues. These bioceramics primarily belong to Calcium Phosphates (CaPs), bioactive glasses, and glass-ceramics. Several techniques are used to deposit these coatings such as; electrophoretic deposition (EPD), plasma spray (PS), and Radio frequency magnetron sputtering (RFMS). Most of these techniques require a high-temperature operation or sintering treatment. This causes either thermal decomposition of bioceramic or results in delamination and cracking of the bioceramic coating due to differences in thermal expansion behavior of metals and bioceramics. RFMS is primarily carried out either at room temperature. However, annealing is performed or substrate is heated at various temperatures ∼400–1,200°C for 2 or 4 h under dry argon (very low temperature compared to other techniques) to ensure crystallization of bioceramics and improve coating adhesion. Chemical composition stability and excellent surface finish are the premium features of RFMS, due to less heat involvement. Moreover, RFMS has the unique ability to develop one-unit/ multilayered composite coatings and the flexibility of in-situ reactions to yield oxides and nitrides. Single or multiple targets can be employed with the insertion of Oxygen and Nitrogen to yield versatile coatings. Due to this attractive set of features RFMS has a strong potential in the field of bioceramic coatings. In recent years, several multifunctional bioceramic coatings have been deposited on metallic substrates using RFMS for biomedical applications. This review focuses on the recent efforts made in order to deposit multifunctional bioceramic RFMS coatings with surface characteristics necessary for biomedical applications and highlights future directions for the improved biological performance of RFMS bioceramic coatings.
Introduction
The demand for biomaterials is increasing day by day to address the needs of an aging population. These biomedical materials are beneficial for physically disordered people as a result of an injury or any natural disease (e.g., thyroidal disorder, bone cancer, etc.) (Pawlik et al., 2019; Pandey et al., 2020). In this connection, bone implants (either orthopedic or dentistry) are the most prevalent use of such materials in the human body. Other aspects that contribute to increased implant surgery include bone weakening (osteoporosis), trauma, and inflammation in bone joints (osteoarthritis) (Bellucci et al., 2012; Ur Rehman et al., 2017).
A fact is that about 2.8 million cases of bone repair are performed annually worldwide. According to reports, hip replacements and knee arthroplasties are expected to increase by 174 and 673%, respectively, from their current levels by 2030 (Bellucci et al., 2013). The anticipation of such a huge demand for biomaterials in the coming years has sparked the interest of scientists and researchers from all around the world (Liu et al., 2019; Ahmed et al., 2020; Ahmed and Rehman, 2020).
Bone-related implants once implanted, serve as a medium for contact and interaction with the surrounding cells and tissues. Therefore, selecting bone biomaterials is an important step in creating optimal bone implants (Zhang et al., 2014). In general, bone implants are selected based on their inherent biocompatibility, biodegradability, and mechanical characteristics, as well as cell behavior. In addition, hydrophobicity, molecular weight, and physicochemical properties are also crucial (Chopplet and Theirry, 2020; Nawaz and Ur Rehman, 2021).
Bioceramics, polymers, and biomedical metals are commonly studied materials for bone-related implants. Bioceramics are brittle with poor fracture toughness, inadequate mechanical strength, and a high elastic modulus compared to the cortical bone. In contrast to this, polymers’ mechanical strength and elastic modulus are much lower than that of the cortical bone, which limits their application in weight-bearing sites. Therefore, metals are preferred for load-bearing implant applications (Manivasagam et al., 2010; Nawaz et al., 2020). Biomaterials are utilized in various parts of the human body as implants, as shown in Figure 1.
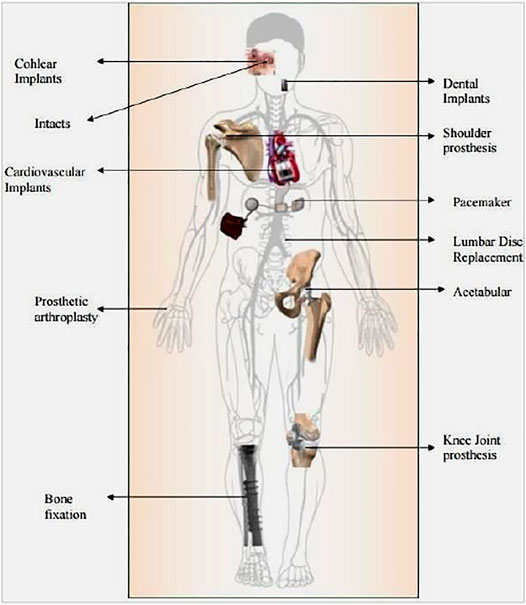
FIGURE 1. Various parts of the human body where biomaterials are used as implants Adapted from (Pandey et al., 2020).
Metals for biomedical applications offer better structural functions than ceramic and polymer-based biomaterials; this is because metals exhibit excellent mechanical biocompatibility (young modulus, toughness, etc.), high strength, and lower densities which are essential requirements for load-bearing implant applications. Metals can replace unhealthy natural parts or repair various organs of the human body for a long time. The use of metal-made implants was primarily established by the need to repair bone, and then their use for orthopedic purposes was expanded along with the use of short-term pins and screws, followed by the use of permanent implants for total joint replacement (Gao et al., 2017).
As far as biometals are concerned currently, load-bearing bone implants are manufactured using Stainless steels (SS), Co or Ti-based alloys. Apart from the advantage of strength, these alloys contain some toxic metallic ions (e.g., Ni, Cr, and V) which are responsible for biocompatibility complications. Moreover, elastic moduli of these metals are much higher as compared to the cortical bone which results in stress shielding effect. Further, the need for revision surgery is also a concern with metallic implants. These metals are non-bioresorbable, meaning they will remain in the body indefinitely. On the other hand, biodegradable metals, mainly including Mg, Zn, Fe, and their alloys, are considered potential load-bearing bone biomaterials (Barati Darband et al., 2017). Among these, Mg and its alloys have attracted the most attention because their Young’s moduli and densities are closer to those of cortical bone. They can effectively relieve the stress-shielding effect and curse of revisionary surgery due to their degradability. Figure 2 demonstrates that how biomedical materials are being revolutionized during the last 7 decades (Yang et al., 2020). The story of biomedical material started with the metallic implants where the focus was to meet the mechanical compatibility. The second-generation biomedical materials were bioactive and can form a robust bond with the natural tissue. Thus, the second generation introduces the concept of bioceramics. Although, the bioceramics presented poor mechanical properties. Yet, their favorable degradation kinetics revolutionize the biomedical industry. Finally, the third generation introduces the concept of bone tissue engineering and the possibility to repair the defective tissues. Furthermore, the concept of a targeted drug delivery system further improved the performance of biomedical materials (Nimbalkar et al., 2020).
This review paper summarizes the recent developments in the field of bioceramic coatings highlighting the importance of the coatings obtained via magnetron sputtering. Various types of bioceramic coatings including hydroxyapatite (HA), calcium phosphate (CaP), bioactive glasses (BGs), etc. are discussed. We highlighted the advantages and challenges associated with each type of coatings by collecting the data from the available literature. Finally, concluding remarks and future prospect is presented.
Need of Bioceramic Coatings
The physiological environment contains a relatively higher concentration of chloride ions at relatively higher humidity. Thus, the metallic implants corrode in the human body leading to the leaching of unwanted toxic metal ions. Therefore, the performance of current orthopedic implants and prosthesis components composed of metallic biomaterials is relatively limited (Ur Rehman et al., 2020). The strategies for functionalizing metallic implant surfaces through changes in the material’s surface composition, structure, and morphology while maintaining mechanical qualities are needed. As a result, dental and orthopedic implants will have considerably improved performance and service life. Owing to their superior osteoconductive capabilities and high chemical stability, bioceramic coatings appear to be the option for the functionalization of implants that are in direct contact with bone. In particular, the following are some necessary aspects that justify the fact that why bioceramic coatings are vital for a metallic implant (Prasad et al., 2017).
a) Biocompatibility
Human blood and bone tissue come into close contact with the implant material being employed. The human body may be affected by two types of unfavorable changes. The unfavorable alterations are as follows: the first is a change in the genome’s DNA (i.e., genotoxic), and the second is cell damage (i.e., cytotoxic). To avoid these undesirable effects the higher biocompatible bioceramic coatings (e.g., HA or calcium phosphates) are very useful for metallic implant surfaces (Wegst et al., 2015).
b) Osseointegration
Osseointegration refers to the capacity of an implant’s surface to integrate with surrounding bone and tissues. Bioceramics [such as HA and biphasic Calcium Phosphate (BCP)] have a high ability to form osseous tissues which assist bone formation (Rizwan et al., 2017).
c) Corrosion resistance
Implant corrosion is a serious and concerning problem. It emerges as a consequence of metal implants exposure to chloride ions and protein in human blood. The metallic implant conducts an oxidation reaction, which yields metallic ions. The formation of a thin and adhesive layer of bioceramic on the surface of the implant will preserve the metal substrate and ultimately enhance the corrosion resistance (Mahapatro and Arshanapalli, 2017).
d) Drug delivery
Porous bioceramic coatings may be employed to deliver drugs, growth factors and proteins at a controlled rate (Singh et al., 2015). Nano-featured coatings exhibit enhanced ability of controlled drug delivery due to larger surface area (Patel et al., 2012; Patel et al., 2014b). Antibiotics are particularly loaded in bioceramic coatings on metallic implants, to resist surgical site and hospital acquired infections. Patel et al., 2012 has reported the loading of ampicillin (a well-known antibiotic) on Chitosan–nanobioactive glass coating. In a similar work, (Patel et al., 2016) has studied the drug (dexamethasone phosphate) delivery potential of mesoporous silica-shelled hydroxyapatite nanoparticles and chitosan composite layer.
e) Microbial resistance
The usage of implants with antibacterial characteristics, as well as superior mechanical and physical-chemical properties and strong bond bonding, is the latest medical trend. Cu and Ag have been utilized to improve the antibacterial characteristics of materials over the years. Despite their capabilities, such coating materials have significant disadvantages like cytotoxicity and harmful long-term effects. In this scenario, bioceramic coatings (such as bioglass and zirconia-based ceramics) might be a good choice (Bellucci et al., 2012).
Bioceramic Coating Techniques
Ceramic-based films have been employed for therapeutic purposes in orthopedics (Choi et al., 2018). Most of the research for biomedical implants is influenced by this philosophy. Biopolymers like peptides, polysaccharides, as well as triglycerides are used to construct support as well as shielding for a calcified structure such as shell, bone, even teeth (Liu et al., 2004). Biopolymers are also reported to regulate growth rate, phase composition, crystal volume, as well as crystalline alignment of biomineralization. Nature’s technique of hard tissue synthesis is progressively being imitated by replicating organic resorption activities as well as practicing their tactics to enhance the properties and performance of bioceramic surfaces (Mali et al., 2016). Template-mediated self-organization is being used to build solution-based coating processes that imitate biological resorption paths. Regulated crystalline nucleation and regulated phase formation are required for the optimal implementation of such approaches (Qaid et al., 2019). Uniform coatings are being deposited to practically any material using such procedures (Fathi et al., 2003). Osteoinductive entities, like the non-collagen extracellular matrix (ECM), proteins, osteocalcin, osteonectin, osteopontin, or cytokines, including components of recombinant human bone morphogenetic protein (rhBMP), can be incorporated into biomimmetically deposited coatings to stimulate potent osteoinductivity. Biomimetic carbonated hydroxyapatite development (Hamdi et al., 2019).
Biomimetics is a new era material science topic that probes deep into the secrets about how Nature’s route functions and explores the elements of science engineering, to imitate therapeutic products (Wu et al., 2020). To deposit biomimetic carbonated hydroxyapatite, substrates are placed in simulated body fluid (SBF)/ Hank’s mixture for many weeks at 37°C. This procedure results in the creation of a bone-like bioactive layer upon this substrate. This process is used to make nebulous or amorphous-crystalline Ca-P coats of 1–5 mm thickness (Zafar et al., 2018). As the substrates are completely immersed in the solution, even those samples having complex geometries can still be coated with such a method (Heimann and Lehmann). For example, Ti implants are commonly employed as templates for biomimetic deposition, yet such implantation was annealed (to improve the adherence and crystallinity of biomimetic apatite layer) before final usage (Zhou et al., 2019). The most significant feature of this technique is its ability to deposit bone-like apatite at almost room temperature. Complex structures can be coated by such techniques. This technique has some limitations, such as it consumes time and necessitates replenishing continuously to ensure constant ionic concentration and pH. (Campbell, 2003).
Electrochemical Deposition Technique
Low-temperature production, as well as precise control of coating thickness through deposition parameters, are two advantages of the electrochemical deposition (ECD) (Heimann and Lehmann). A three-electrode assembly soaked in an electrolytic cell as well as coupled to an electrical device is generally used in this method (Chatterjee et al., 2019). A titanium/magnesium alloy cathode (implant material serves as a working electrode while an Ag/AgCl reference electrode acts as a reference electrode in the presence of a platinum reference electrode. For the deposition of CaPs a calcium ion (Ca2+) carrier, like calcium dihydrogen phosphate or calcium acetate, along with a phosphate ion (PO42−) carrier (including calcium or sodium dihydrogen phosphate, or sodium glycerophosphate) are widely used in electrolytic solutions (Pina et al., 2018). In ECD, the pH of the solution, current density, the electrolyte temperature, and the ionic strength derive the type as well as stoichiometric calculations of calcium phosphate deposited at the cathode (Furko et al., 2016a). Electrolytic deposition of calcium phosphate coating in the continuous current mode is restricted to current densities. Combination strategies, such as the utilization of pulsed currents and the insertion of hydrogen peroxide (H2O2) into electrolytic solution are being recommended to fix this issue (Oh et al., 2006).
Electrophoretic Deposition
The mechanism of electro-kinetic motion of colloidal particulates in the presence of an electric field is known as (EPD) (Riau et al., 2016). It is a robust minimum material-processing approach for fabricating various combinations of biopolymer and bioceramics, for example, hydroxyapatite/chitosan, hydroxyapatite/poly (-caprolactone) coatings, carbon nanotubes (CNTs)/chitosan, etc (Pezzotti, 2021). It’s a wet deposition process that uses colloidal ceramics. It has the potential of depositing homogeneous coatings on complex-shaped substrates while consuming minimal material. The deposition rate, crystallite size, as well as thicknesses of the deposited coatings, can all be adjusted by adjusting the suspensions as well as applied electric field values (Furko et al., 2016b). EPD is a cost-effective process that led to, homogeneous coatings with the controlled morphology and composition (Das and Shukla, 2019). The costings thickness obtained via EPD varies from few nanometers to few micrometers. The coating thickness can be manipulated by varying the deposition voltage and deposition time. Co-EPD of composite from single suspension is a challenging task. Furthermore, EPD is affected by the minor changes in deposition parameters, i.e., applied electric field, pH, temperature, humidity, etc. The major concern with the EPD is relatively low adhesion between the coatings and the substrate (Heimann, 2013).
Plasma Techniques
Plasma Electrolytic oxidation (PEO), also known as micro-arc oxidation (MAO) develops an adherent porous coating layer on valve metals through oxidation beyond the breakdown potential (Gul et al., 2019). In the process of MAO, ions from valve metals combine with anions in the electrolyte by traveling far from the metallic surface, whereas oxygen travels concurrently towards the substrate as a result of a strong electric field. This yields in the development of a porous oxide layer, and during the process it can incorporate ions/particles present in the electrolyte inside the ceramic layer (Love et al., 2013). Under the influence of a strong electric field, collisions between traveling atoms and ions cause local heating (Joule heating) as a result of the strong electrical resistance of the oxide layer. Several ceramics incorporated MAO layers have been developed in recent years through particle suspension in the electrolyte (Su et al., 2019). Hydroxyapatite and other CaPs have been incorporated to improve biological performance, while SiC, ZrO2, and other high hardness ceramics have been employed to improve the wear resistance of MAO layers. The autosintering nature of the MAO technique makes it an extremely attractive technique to deposit bioceramics. However, it has an inherent limitation of applying to only valve metals (Fathi et al., 2003). Due to benefits including procedure efficiency, high deposition kinetics, minimal substrate temperature, as well as sustainability, plasma spraying is the most commonly used technology for preparing coatings for load-bearing prostheses. Bioinert, as well as bioactive coatings, can be deposited in a cost-effective manner using this set of techniques (Kaur et al., 2019), Through this method, 30–300 mm, thick, non—homogeneous HAp coatings with significant deposition potential are produced. The coatings produced are wear and corrosion-resistant. In certain circumstances, an adhesive strength of more than 15 MPa may be achieved (Alagarsamy et al., 2020). Heat causes degradation of the bioceramics. Moreover, major limitations of plasma spray techniques include thickness inhomogeneity, inadequate crystallinity, line-of-sight nature, as well as minimal adhesion strength to metallic surfaces. Rapid depressurization causes fractures in the coating (Arcos et al., 2009). Plasma-sprayed coated prostheses demonstrate an obvious advantage of owing greater surface area, which leads to improved osseointegration in vivo (Bansal et al., 2020).
Radio Frequency Magnetron Sputtering
RF magnetron sputtering, unlike many other technologies, permits coating to be deposited upon the surface of metallic implants, ceramics, or even polymeric materials (Qadir et al., 2019; Bociaga et al., 2016). In the sputtering process, the material needs to be deposited (target) serves as a cathode, and receives a bombardment of high-energy ions (Bociaga et al., 2019). These ions are produced as a result of plasma generation from Ar Bombardment, causes the target to erode (sputter) and the eroded atoms deposit onto the substrate (Bolbasov et al., 2018; Das and Shukla, 2020b). This conventional sputtering exhibits slower deposition rates, higher substrate heating, and limited plasma ionization efficiency (Shukla et al., 2017; Prosolov et al., 2017). These limitations of conventional sputtering have been counterbalanced by magnetron sputtering, which carries a set of permanent magnets under the target resulting in enhanced plasma and increased deposition rate (Qadir et al., 2019; Bociaga et al., 2016).
RF-sputtered coatings exhibit bioceramic composition control, spatial homogeneity, as well as the capacity to coat implants with complex shapes owing to good adherence (Hamdi et al., 2019). Coatings created by RF magnetron sputtering (a plasma-chemical technique) are often amorphous and therefore require a moderate temperature annealing to impart crystallinity (Kozelskaya et al., 2018; Calderon Velasco et al., 2016). With its faster deposition potential, magnetron sputtering is far more favorable than other sputtered processes, (Safavi et al., 2020). It also has excellent substrate adherence and can coat complicated pieces with greater ease than other sputtering processes (Prosolov K. A. et al., 2019). Efficient, productivity high reproducibility, ability to coat samples with 3D symmetry, and low internal stresses are the major advantages of this technique (Calderon Velasco et al., 2016). Table 1 illustrates different bioceramic coating techniques showing their pros and cons. Figure 3 shows the different technologies available to deposit bioceramic coatings.
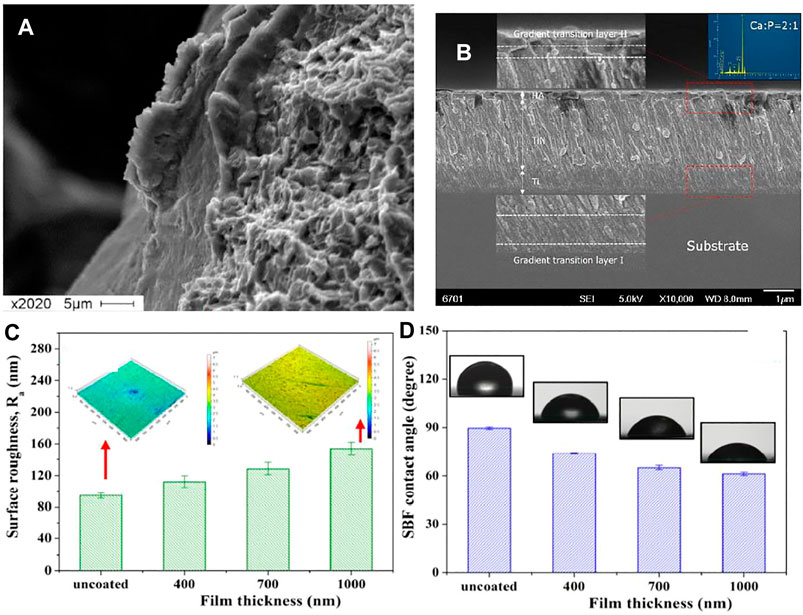
FIGURE 3. (A,B) SEM Surface morphology of Ti6Al4V 3D-lattice structures generated by additive manufacturing with HA coating. Adapted from (Chudinova et al., 2016). Reproduced with permission from IOP Publishing (United Kingdom) (C) Surface roughness (Ra) variation with the change in coating thickness and, (D) variation in contact angle (measure by using SBF drop) with the change in the thickness of the coatings. Adapted from (Behera et al., 2018). Reproduced with the permission from Journal of Elsevier.
The Current State of the Art in RF Magnetron Sputtered Bioceramic Coatings
Bioceramics are deposited on metals using different techniques. The earlier section discusses the merits and demerits of each coating technique. This section summarizes the recent developments related to the deposition of bioceramics using RF Magnetron sputtering. Ti and its alloys due to their excellent biocompatibility and good corrosion resistance are primarily materials of choice for bone and dental implants (Ibrahim et al., 2017). However, like other metals, these alloys also lack bioactivity. To impart bioactivity and enhance biological performance, bioceramic coatings are being developed on the surface of these alloys. Due to the proven performance of Ti and its alloys as implant materials, the highest share of RF magnetron sputtering bioceramic coatings has been received by them. Researchers have reported the ability of RF magnetron sputtering to deposit a thick layer (millimeter range) of adherent bioceramics on pure (Ivanova et al., 2018) developed HA coating on Ti with preferred orientation along all three axes and evaluated the mechanical behavior of the coatings concerning orientation. A novel approach of water added into the working chamber of RF magnetron sputtering was used in this study to compensate for OH− group loss during processing.
Among Ti alloys, Ti6Al4V (TC4) has received the highest research interest related to RF magnetron sputtering of bioceramics, as it is the most widely employed Ti alloy for the production of biomedical implants and surgical tools. RF magnetron sputtering has also been reported to successfully deposit HA-based coatings on porous scaffolds of TC4 alloy (Chernozem et al., 2017). Apart from pristine HA ion-substituted HA (which is known to further enhance biocompatibility) and Biphasic calcium phosphate (BCP) have also been deposited onto TC4 alloy using RF magnetron sputtering (Behera et al., 2018; Prosolov et al., 2018). Along with biocompatible coatings antimicrobial layers based on metallic ion containing bioceramics have also been deposited using RF magnetron sputtering. Zn-substituted HA, which exhibits antibacterial behavior along with improved osteointegration was deposited using RF magnetron sputtering with the heated substrate (Graziani et al., 2017). Substrate heating at 400°C resulted in completely crystallized Zn-substituted HA deposition with columnar morphology. Similar findings from different researchers suggest that controlled heating of the substrate can improve the crystallinity of the bioceramic coatings. (Lenis et al., 2019) has reported the deposition of homogenous crack-free BCP with prolonged (up to 8 h) RF magnetron sputtering. Relatively newer Ti alloy (Ti–35Nb–10Zr) is also a good candidate for potential biomedical applications. Si-HA layer was also shown to be successfully synthesized on this alloy using RF magnetron sputtering to favor enhanced osseointegration as an orthopedic implant. Figure 3A shows the SEM image depicting a fairly uniform morphology. Figure 3B shows the bilayer structure of TiN and HA layer deposited on Ti substrate. The coating was uniform throughout the substrate surface. The inset of Figure 3B confirms the presence of Ca and P in the top layer. Figure 3C shows that the average roughness (Ra) increases with the increase in the coating thickness. Thus, it is possible to tune the surface topography as a function of coating thickness to achieve roughness values suitable for bone regeneration applications (Ureña et al., 2018). Another important aspect of the biomedical coating is wettability. The surface should promote the protein attachment owe to the favorable surface chemistry, wettability, and topography (Lee et al., 2013; Rehman et al., 2019). Since magnetron sputtering has shown the potential to improve the surface wettability as the function of coating thickness and surface chemistry (Jeong et al., 2016). Figure 3D shows that the SBF contact angle decreases with the increase in the coating thickness. The increase in coating thickness means more HA deposition and subsequently leading to mildly hydrophilic character, which in turn is suitable for the initial protein attachment (Bumgardner et al., 2003; Menzies and Jones, 2010; Farris et al., 2011).
In conventional RF-magnetron sputtering, it’s not possible to incorporate controlled porosity (Dorozhkin, 2012). Chernozem et al. (Chernozem et al., 2017) introduced a unique strategy to control porosity in the HA layer by growing vertically aligned TiO2 nanotubes through anodization of Ti and then covering it with HA through RF-magnetron sputtering. RF-magnetron sputtering can accommodate simple reactions as well to prepare oxides and nitrides through the introduction of Oxygen or Nitrogen gases in the sputtering chamber (Alias et al., 2020). Qi et al. (2019) synthesized a TiN-HA composite (two targets, i.e., Ti and HA) layer on TC4 alloy through reactive magnetron sputtering. TiN interlayer was used to improve the adhesion of HA. Using a similar strategy. HA-based composite layer with TiO2 and TiN interlayers have also been reported by introducing O and N gases in the sputtering chamber (Quirama et al., 2017). Apart from CaP based bioceramics other bioactive ceramics have also been reported to be magnetron sputtered. Hopeite is hydrated zinc phosphate which is a bioactive ceramic and commonly used as a coating on dental implants (Herschke et al., 2006). (Das and Shukla, 2020b) reported a hopeite-based bioactive layer on TC4 alloy using RF-magnetron sputtering. Figure 4A shows the SEM image of hopeite coated sample subsequent to tensile pull-out test. Figure 4B demonstrated the adhesion strength of the coatings as a factor of distance as well as deposit temperature. It was suggested that the increase in the coating temperature led to the increase in the adhesion strength, as indicated by the increase in the critical load (Figure 4B). Figure 4C depicted the strain change of the (002) as well as (300) planes of the HA coatings placed in an Ar + 10% H2O atmosphere. Figure 4D shows the Raman spectra of HA monolayer and TiN/TiO2/HA trilayers. The tri-layer coating system shows the shifting of the peaks associated with the HA. Thus, indicating the possible chemical interaction between the different layer. The strong chemical linkage between the different layer will lead to the increase in the adhesion strength between the coating layer. Thus, the coatings obtained via RF magnetron sputtering can lead to the coatings with the better mechanical and surface properties for the orthopedic and dental applications.
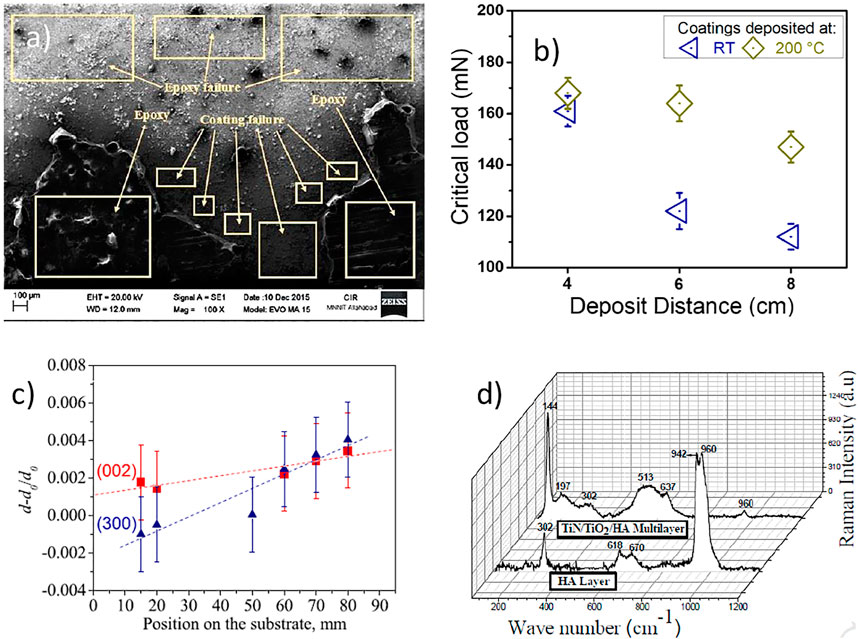
FIGURE 4. (A) Scanning electron microscopy images of hopeite coated sample after the tensile pull-out test. Adapted from (Das and Shukla, 2020b). Reproduced with permission from Transactions of the Institute of Metal Finishing (B) As a factor of distance as well as deposit temperature, the load capacity (critical load) of the coatings were evaluated. Adapted from (Lenis et al., 2019). Reproduced with the permission from Elsevier (C) Depicted the strain change of the (002) as well as (300) plans of the HA coatings placed in an Ar + 10% H2O atmosphere. Adapted from (Ivanova et al., 2018). Reproduced with the permission from Elsevier (D) Raman spectra of HA monolayer and TiN/TiO2/HA. Adapted from (Quirama et al., 2017). Reproduced with permission from Elsevier.
Magnesium-based alloys are under research focus in recent years due to their comparable young modulus with bones, lower density, and biodegradability (Zheng et al., 2014). The primary limitation of these alloys is their fast dissolution rate in physiological environments (Barati Darband et al., 2017). To improve dissolution behavior and improve biocompatibility bioceramic coatings have been developed on these alloys. Bita et. al. (2016) exploited RF magnetron sputtering to achieve the adherence of HA and BG on biodegradable prosthetic magnesium–calcium (Mg-Ca) alloy. According to the study, a post-deposition tempering approach at 500°C/1 h in the air is sufficient to trigger the crystallization of a 1 µm thick layer. Figures 5A,B presents a comparison of oxides content of bioactive glasses Cohesion/ adhesion pull-out strength higher than the minimum acceptable value defined in ISO 13779-2 (standard regulating the manufacturing of superior load-bearing implant type) were obtained using all varieties of sputtered HA as well as BG layers. Mg alloy AZ91 has also been evaluated for the in vitro biological performance after the deposition of RF magnetron sputtered HA (Surmeneva et al., 2019). A homogeneous and pore-free HA coating was developed using RF magnetron sputtering with a substrate bias of −25 and a substrate bias of −100 V, followed by post-deposition annealing. –100 V bias substrate yielded a better surface for bone marrow stromal cells (BMSCs) proliferation and decreased dissolution of Mg ions. The negative biasing of the metallic substrate is an attractive research direction to overcome the inherent inability of RF magnetron sputtering to deposit porous layers.
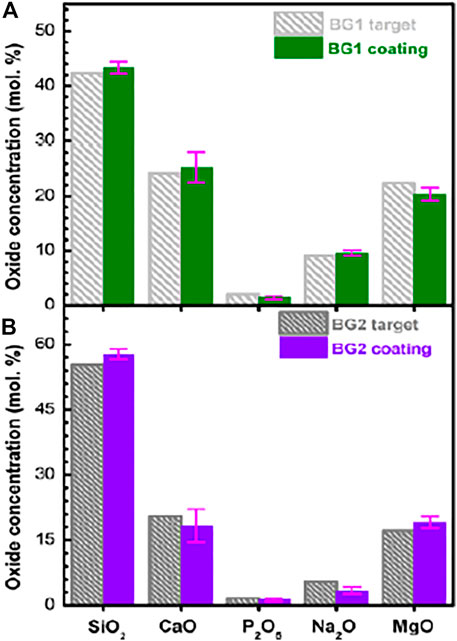
FIGURE 5. (A,B) Oxide concentration (mol%) of BG target materials with RF-MS deposited coatings are compared in histograms. Adapted from (Bita et al., 2016). Reproduced with the permission from Journal of Adhesion Science and Technology.
RF magnetron sputtering owns a unique ability to deposit bioceramic layers to yield composite coatings in a single step, as well as they can be employed as a subsequent step after any other coating technique. For single-step composite coatings, two strategies may be employed. One target with desired composite’s content may be prepared or multiple targets can be used (Alias et al., 2019) and (Alias et al., 2019). Behera et al deposited TiO2-BCP layer on TC4 alloy by synthesizing a single target with varying content of each bioceramic. Figure 6A shows the SEM image of anodized Ti surface and Figure 6B shows the SEM image from side one to show the aspect ratio TiO2 nanotubes. Figure 6C shows variation in the surface wettability with the change in the composition of two-layer, i.e., TiO2-BCP content in the coatings. It was shown that the increase in the TiO2 content from 0−50% induces hydrophilic character. Thus, the increase in TiO2 content is beneficial in achieving contact angle values suitable for bone regeneration applications (Bumgardner et al., 2003). The best compromise of adhesion strength and biological performance was achieved at equivalent amounts of BCP and TiO2 (in target). Furthermore, multilayered coatings with separate targets (Ag and HA) were also prepared for orthopedic and dental applications.
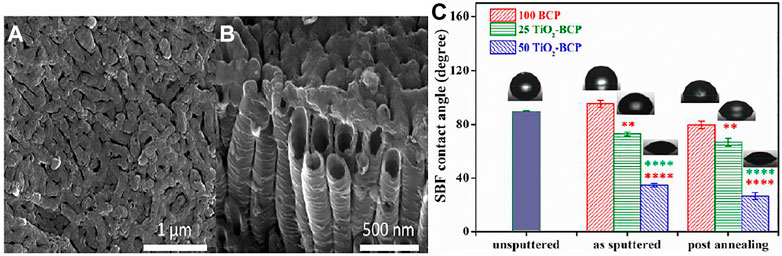
FIGURE 6. (A,B) Scanning electron microscopy of upper and side overview respectively of calcium phosphate coated with titania synthesized at 60 V for 30 min of electrochemical anodization in an electrolyte after annealing. Adapted from (Chernozem et al., 2017). Reproduced with the permission from Elsevier (C) Wettability results of 100 BCP, 25 TiO2-BCP, and 50 TiO2-BCP films with and without annealing. Adapted from (Behera et al., 2020). Reproduced with permission from Elsevier.
Table 2 provides an insight regarding the recent developments in the field of bioceramic coatings using RF magnetron sputtering (Lenis et al., 2019).
Improvement in the Surface Properties via Magnetron Sputtering
In a recent work (Pantaroto et al., 2021) used magnetron sputtering to develop various crystalline phases of TiO2 on titanium substrates (CpTi). Sputtered films had an excellent mechanical adherence to the substrates and were more resistant to abrasion. The TiO2 films produced measured 312–338 nm thick, thus, they had no effect on prosthesis adaption because a gap of 150 nm is within approved clinical limits. Figure 7 describes the mechanism of TiO2 deposition on CpTi, as this study is among the pioneering works on the deposition of TiO2 on titanium substrates using reactive RF magnetron sputtering.
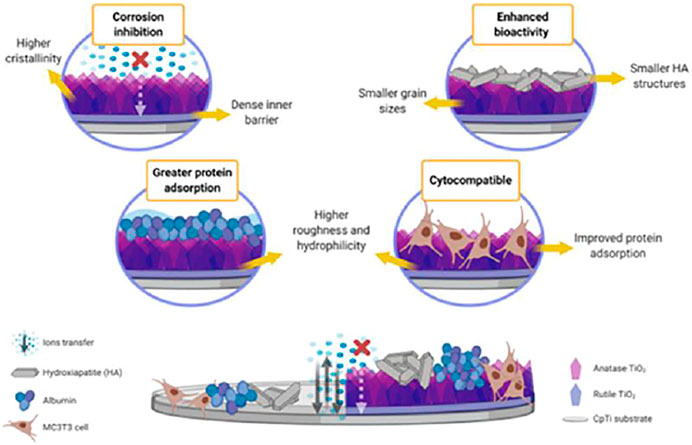
FIGURE 7. Proposed mechanism of sputtered bi-phasic rutile and anatase TiO2 film on the electrochemical and biological behavior of cpTi substrate. Adapted from (Pantaroto et al., 2021). Reproduced with the Permission from Elsevier.
Multilayer bioactive coatings have also been deposited using RF magnetron sputtering. (Hamdi et al., 2019) deposited triple-layered (HAp/Al2O3/TiO2) thin film coatings on the Ti alloy substrates. The presence of interim Al2O3/TiO2 layers aided in the improvement of HAp coating adherence to the substrate. As a corollary, HAp layers figured prominently in the surface’s biocompatibility, whereas interim Al2O3/TiO2 layers were used to improve the adherence of the HAp coating to the substrate along with the substrate’s corrosion tendency. The increased porosity is associated with enhanced osseointegration by allowing bone to grow into the coatings, resulting in mechanical interlock (as shown in Figure 8). By RF magnetron sputtering, (Das and Shukla, 2017) effectively produced columnar HA coatings on UNS S31254 substrate (MS). HA coatings produced on SS254 by magnetron sputtering yielded improved surface characteristics, adhesion, as well as biocompatibility of HA coatings. yield. The developed layer is well suited for possible orthopaedic implant applications due to the anhanced bioactivity, promoting better osseointegration (Figure 8).
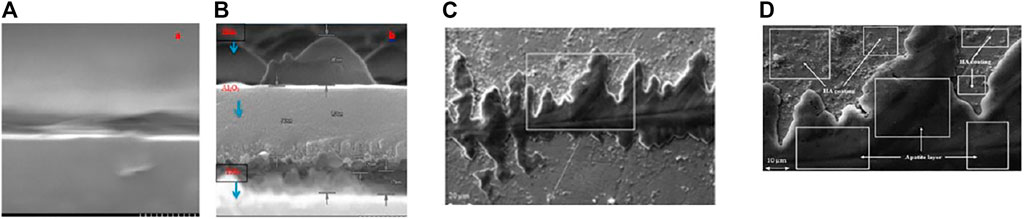
FIGURE 8. The cross-sectional SEM image of (A) Ti-6Al-4V original alloys, and (B) triplelayered (HAp/Al2O3/TiO2) thin film. Adapted from (Hamdi et al., 2019). Reproduced with the permission from Elsevier. (C,D) SEM micrographs of HA-coated sample after immersion test at (A) 1,000× and (B) 2,500×. Adapted from (Das and Shukla, 2017). Reproduced with the permission of Maney Publishing.
Along with metallic substrate RF magnetron sputtering has also been employed to coat polymeric substrates for biomedical applications (Tverdokhlebov et al., 2012; Goreninskii et al., 2017) examined the features of thin calcium-phosphate coatings generated on the surface of the thermoplastic copolymer of vinilidene fluoride and tetrafluoroethylene (VDF–TeFE) by radio-frequency magnetron sputtering of a solid target constructed of hydroxyapatite. The VDF–TeFE polymer coating’s surface is a homogenous film made up of polymer globules of elongated ellipsoids with 1.02 and 0.71 nm diameters. In orthopaedics and traumatology, the proposed surface modification technique can be employed to assist the adhesion and proliferation of osteogenic cells in order to manufacture implants (Figure 9). It was shown that hydroxyapatite produced from fish scale and sputtered onto AZ31Magnesium alloy using an RF magnetron sputtering technique enhanced the stability of biodegradable alloy. The degradation rate of untreated AZ31 was calculated to be 0.021 d-1 k, however it was reduced to 20% for the 9-h coated AZ31 (i.e., 0.016 d-1). It was also reported that the hydrophobic surfaces limit solid-liquid interfacial interactions, thus, prevent degradation.
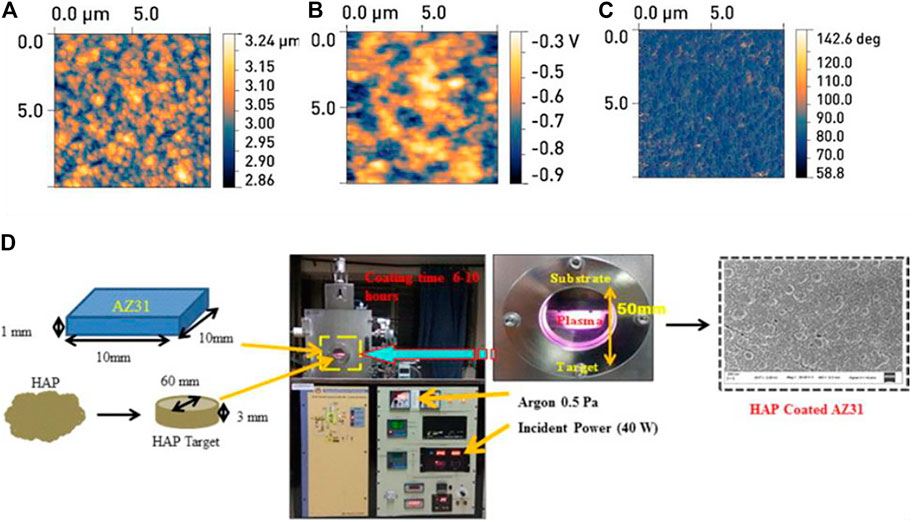
FIGURE 9. AFM images of the surface samples of the group II: (A) relief, (B) map of the distribution of the potential, (C) the image of the phase contrast. The scanning area is 10 m × 10 m. Adapted from (Tverdokhlebov et al., 2012). Reproduced with the permission from Elsevier. (D) Schematic illustration of HAp (target) deposition on AZ31 (substrates).
(Masoorianfar et al., 2020) modified the surface of metallic implants by the addition of fluorine into HA. This fluorine substituted HA is known to enhance the dissolution resistance of HA in biological environments (Rizwan et al., 2020). The higher affinity of fluorine ion, apatite layer is formed without change in size, topography and composition (Figure 10). Degradation decreased by the incorporation of fluorine within apatite layer so, FA is considered as a potential candidate for enhanced corrosion resistance in biological environment.
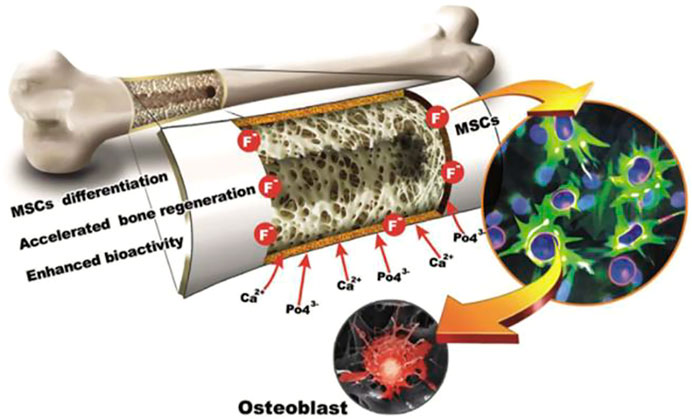
FIGURE 10. The fluorhydroxyapatite (FA) tissue and surface manufactured thin film of FA employed in this research are shown in this diagram. Adapted from (Mansoorianfar et al., 2020). Reproduced with the permission from Elsevier.
Concluding Remarks and Future Directions
The surface modification via coating process can tune the chemical, physical and biological properties of the implant material. Various coating techniques including conversion coatings, electrochemical plating, EPD, anodizing, RF magnetron sputtering are being used to develop biomedical coatings with favorable mechanical and biological characteristics (Kumar et al., 2019). Magnetron sputtering is the method of choice to tune the implant surface with relatively higher thermal, mechanical, and chemical stability. The coating deposited via magnetron sputtering can yield better wear and corrosion resistance compared to the other counterparts. For example, ion-substituted calcium phosphate coatings deposited via magnetron sputtering are being widely adopted for dental and orthopedic applications owe the favorable microstructure, adhesion strength, chemical and surface properties (Shi et al., 2008). Although, the magnetron sputtering requires higher capital investment. However, the running coat of the process is affordable. The coating properties can be controlled by varying the discharge power, bias voltage, deposition time, substrate temperature, and post-deposition annealing treatment.
The real challenge in the coating process is to develop the surface with good adhesion, strength, wettability, chemical structure, surface topography, wear resistance, electrochemical stability, antibacterial properties, and negligible cytotoxicity. It is quite challenging for any coating process to meet all the requirements. For example, the coatings deposited via magnetron sputtering lack porosity (surface topography). Thus, the coatings developed via the magnetron sputtering process can result in poor osseointegration. The osseointegration of the coatings can be improved by changing the microstructure, surface morphology, and thickness of the coatings (Samiee et al., 2021). The magnetron sputtering process requires relatively lower processing temperatures. Thus, it is possible to achieve the coatings with different percentages of crystallinity depending upon the feedstock. For example, amorphous HA coatings and ion-substituted HA were shown to improve the cell attachment compared to the crystalline HA (Vladescu et al., 2018). To improve the cellular interaction of the coatings magnetron sputtering is recently being coupled with EPD. The first layer deposited via EPD creates a particular pattern on the implant surface. Later, magnetron sputtering was used to deposit mechanically and chemically robust layers. However, the topography of the first was maintained. Thus, the favorable topography resulted in improved osseointegration and cellular attachment, and proliferation. Furthermore, bilayer and tri-layer structures have also been deposited via magnetron sputtering to achieve the ideal topography for biomedical applications (Kumar et al., 2019).
Another challenge associated with the magnetron sputtering is the longer deposition times, which restricts its commercialization. To commercialize the subject process, it is an important future direction to reduce the deposition time, i.e., increase the deposition rate. Future research is expected to improve the deposition kinetics of the magnetron sputtering; thus, the process is successfully translated to the clinic Table 3.
Although, bio-ceramics deposited via magnetron sputtering led to better bone cell attachment, proliferation, growth, osseointegration, and antibacterial properties. However, further research efforts are required to improve the biocompatibility of the deposits to realize the real benefit of excellent mechanical and chemical properties attributed to magnetron sputtering. A comprehensive understanding of the detailed chemical, morphological and structural characteristics of coatings are required. Future research directions include in vivo assessments of coatings.
All in all, the magnetron sputtering process led to bioactive coatings with excellent adhesion strength and corrosion resistance. Magnetron sputtering can fabricate nanostructured coatings on metallic and polymeric surfaces. Magnetron sputtering can also be a useful process to control the degradation kinetic of Mg-based alloys. Therefore, it is expected that the magnetron sputtering would broaden the spectrum of clinical applications in the coming years.
Author Contributions
MA writes most sections of the original draft. SU wrote the introduction section, draw the graphics, and review the manuscript. MR wrote the magnetron sputtering section and thoroughly review the whole draft and provide feedback. MU Review the paper and write concluding remarks.
Conflict of Interest
The authors declare that the research was conducted in the absence of any commercial or financial relationships that could be construed as a potential conflict of interest.
Publisher’s Note
All claims expressed in this article are solely those of the authors and do not necessarily represent those of their affiliated organizations, or those of the publisher, the editors and the reviewers. Any product that may be evaluated in this article, or claim that may be made by its manufacturer, is not guaranteed or endorsed by the publisher.
References
Ahmadi, R., and Afshar, A. (2021). In Vitro study: Bond Strength, Electrochemical and Biocompatibility Evaluations of TiO2/Al2O3 Reinforced Hydroxyapatite Sol-Gel Coatings on 316L SS. Surf. Coat. Tech. 405, 126594. doi:10.1016/j.surfcoat.2020.126594
Ahmed, Y., Nawaz, A., Singh Virk, R., Wadood, A., and Rehman, M. A. U. (2020). Fabrication and Characterization of Zein/bioactive Glass (BG) Deposited on Pre‐treated Magnesium via Electrophoretic Deposition. Int. J. Ceram. Eng. Sci. 2, 254–263. doi:10.1002/ces2.10066
Ahmed, Y., and Ur Rehman, M. A. (2020). Improvement in the Surface Properties of Stainless Steel via Zein/hydroxyapatite Composite Coatings for Biomedical Applications. Surf. Inter. 20, 100589. doi:10.1016/j.surfin.2020.100589
Alagarsamy, K., Vishwakarma, V., Kaliaraj, G. S., Vasantha, N. C., and Samuel, S. J. R. (2020). Biological Adhesion and Electrochemical Behavior of Ag-ZrO2 Bioceramic Coatings for Biomedical Applications. J. Adhes. Sci. Tech. 34, 349–368. doi:10.1080/01694243.2019.1666627
Alias, R., Mahmoodian, R., Genasan, K., Vellasamy, K. M., Hamdi Abd Shukor, M., and Kamarul, T. (2020). Mechanical, Antibacterial, and Biocompatibility Mechanism of PVD Grown Silver-Tantalum-Oxide-Based Nanostructured Thin Film on Stainless Steel 316L for Surgical Applications. Mater. Sci. Eng. C 107, 110304. doi:10.1016/j.msec.2019.110304
Alias, R., Mahmoodian, R., Rizwan, M., and Abd Shukor, M. H. (2019). Study the Effect of thermal Annealing on Adhesion Strength of Silver-Tantalum Oxide Thin Film Deposited by Reactive Magnetron Sputtering. J. Adhes. Sci. Tech. 33, 1626–1643. doi:10.1080/01694243.2018.1549770
Arcos, D., Izquierdo-Barba, I., and Vallet-Regí, M. (2009). Promising Trends of Bioceramics in the Biomaterials Field. J. Mater. Sci. Mater. Med. 20, 447–455. doi:10.1007/s10856-008-3616-x
Avcu, E., Yıldıran Avcu, Y., Baştan, F. E., Rehman, M. A. U., Üstel, F., Boccaccini, A. R., et al. (2018). Tailoring the Surface Characteristics of Electrophoretically Deposited Chitosan-Based Bioactive Glass Composite Coatings on Titanium Implants via Grit Blasting. Prog. Org. Coat. 123, 362–373. doi:10.1016/j.porgcoat.2018.07.021
Bansal, P., Singh, G., and Sidhu, H. S. (2020). Investigation of Corrosion Behavior and Surface Properties of Plasma Sprayed HA/Sr Reinforced Coatings on CoCr Alloys. Mater. Chem. Phys. 253, 123330. doi:10.1016/j.matchemphys.2020.123330
Barati Darband, G., Aliofkhazraei, M., Hamghalam, P., and Valizade, N. (2017). Plasma Electrolytic Oxidation of Magnesium and its Alloys: Mechanism, Properties and Applications. J. Magnesium Alloys 5, 74–132. doi:10.1016/j.jma.2017.02.004
Behera, R. R., Das, A., Hasan, A., Pamu, D., Pandey, L. M., and Sankar, M. R. (2020). Effect of TiO2 Addition on Adhesion and Biological Behavior of BCP-TiO2 Composite Films Deposited by Magnetron Sputtering. Mater. Sci. Eng. C 114, 111033. doi:10.1016/j.msec.2020.111033
Behera, R. R., Das, A., Pamu, D., Pandey, L. M., and Sankar, M. R. (2018). Mechano-tribological Properties and In Vitro Bioactivity of Biphasic Calcium Phosphate Coating on Ti-6Al-4V. J. Mech. Behav. Biomed. Mater. 86, 143–157. doi:10.1016/j.jmbbm.2018.06.020
Bellucci, D., Cannillo, V., and Sola, A. (2012). A New Highly Bioactive Composite for Bone Tissue Repair. Int. J. Appl. Ceram. Technol. 9, 455–467. doi:10.1111/j.1744-7402.2011.02641.x
Bellucci, D., Sola, A., Gazzarri, M., Chiellini, F., and Cannillo, V. (2013). A New Hydroxyapatite-Based Biocomposite for Bone Replacement. Mater. Sci. Eng. C 33, 1091–1101. doi:10.1016/j.msec.2012.11.038
Bita, A.-I., Stan, G. E., Niculescu, M., Ciuca, I., Vasile, E., and Antoniac, I. (2016). Adhesion Evaluation of Different Bioceramic Coatings on Mg-Ca Alloys for Biomedical Applications. J. Adhes. Sci. Tech. 30, 1968–1983. doi:10.1080/01694243.2016.1171569
Bociaga, D., Kaminska, M., Sobczyk-Guzenda, A., Jastrzebski, K., Swiatek, L., and Olejnik, A. (2016). Surface Properties and Biological Behaviour of Si-DLC Coatings Fabricated by a Multi-Target DC-RF Magnetron Sputtering Method for Medical Applications. Diamond Relat. Mater. 67, 41–50. doi:10.1016/j.diamond.2016.01.025
Bociaga, D., Sobczyk-Guzenda, A., Komorowski, P., Balcerzak, J., Jastrzebski, K., Przybyszewska, K., et al. (2019). Surface Characteristics and Biological Evaluation of Si-DLC Coatings Fabricated Using Magnetron Sputtering Method on Ti6Al7Nb Substrate. Nanomaterials 9, 812. doi:10.3390/nano9060812
Bolbasov, E. N., Antonova, L. V., Stankevich, K. S., AshrafovMatveeva, А., Matveeva, V. G., Velikanova, E. A., et al. (2017). The Use of Magnetron Sputtering for the Deposition of Thin Titanium Coatings on the Surface of Bioresorbable Electrospun Fibrous Scaffolds for Vascular Tissue Engineering: A Pilot Study. Appl. Surf. Sci. 398, 63–72. doi:10.1016/j.apsusc.2016.12.033
Bolbasov, E. N., Maryin, P. V., Stankevich, K. S., Kozelskaya, A. I., Shesterikov, E. V., Khodyrevskaya, Y. I., et al. (2018). Surface Modification of Electrospun Poly-(l-Lactic) Acid Scaffolds by Reactive Magnetron Sputtering. Colloids Surf. B: Biointerfaces 162, 43–51. doi:10.1016/j.colsurfb.2017.11.028
Bumgardner, J. D., Wiser, R., Elder, S. H., Jouett, R., Yang, Y., and Ong, J. L. (2003). Contact Angle, Protein Adsorption and Osteoblast Precursor Cell Attachment to Chitosan Coatings Bonded to Titanium. J. Biomater. Sci. Polym. Edition 14, 1401–1409. doi:10.1163/156856203322599734
Calderon Velasco, S., Cavaleiro, A., and Carvalho, S. (2016). Functional Properties of Ceramic-Ag Nanocomposite Coatings Produced by Magnetron Sputtering. Prog. Mater. Sci. 84, 158–191. doi:10.1016/j.pmatsci.2016.09.005
Campbell, A. A. (2003). Bioceramics for Implant Coatings. Mater. Today 6, 26–30. doi:10.1016/S1369-7021(03)01128-3
Chatterjee, S., Saxena, M., Padmanabhan, D., Jayachandra, M., and Pandya, H. J. (2019). Futuristic Medical Implants Using Bioresorbable Materials and Devices. Biosens. Bioelectron. 142, 111489. doi:10.1016/j.bios.2019.111489
Chernozem, R. V., Surmeneva, M. A., Krause, B., Baumbach, T., Ignatov, V. P., Tyurin, A. I., et al. (2017). Hybrid Biocomposites Based on Titania Nanotubes and a Hydroxyapatite Coating Deposited by RF-Magnetron Sputtering: Surface Topography, Structure, and Mechanical Properties. Appl. Surf. Sci. 426, 229–237. doi:10.1016/j.apsusc.2017.07.199
Chioibasu, D., Duta, L., Popescu-pelin, G., Popa, N., Milodin, N., Iosub, S., et al. (2019). Animal Origin Bioactive Hydroxyapatite Thin Films Synthesized by RF-Magnetron Sputtering on 3D Printed Cranial Implants. Metals 9, 1332. doi:10.3390/met9121332
Choi, A. H., Conway, R. C., Cazalbou, S., and Ben-Nissan, B. (2018). Maxillofacial Bioceramics in Tissue Engineering: Production Techniques, Properties, and Applications. Elsevier, 63–93. doi:10.1016/B978-0-08-102203-0.00003-2
Chopplet, D., and Theirry, J. P. (2020). Improvement in the Surface Properties of Stainless Steel via Zein/Hydroxyapatite Composite Coatings for Biomedical Applications. Surf. Interfaces 20, 100589. doi:10.1016/j.surfin.2020.100589
Chudinova, E., Surmeneva, M., Koptioug, A., Scoglund, P., and Surmenev, R. (2016). Additive Manufactured Ti6Al4V Scaffolds with the RF- Magnetron Sputter Deposited Hydroxyapatite Coating. J. Phys. Conf. Ser. 669, 012004. doi:10.1088/1742-6596/669/1/012004
Das, A., and Shukla, M. (2020a). Multifunctional Hopeite Nanocoating on Ti64 Substrates by Pulsed Laser Deposition and Radio Frequency Magnetron Sputtering for Orthopedic Implant Applications: A Comparative Study. J. Cent. South. Univ. 27, 2198–2209. doi:10.1007/s11771-020-4441-8
Das, A., and Shukla, M. (2020b). New Generation Hopeite Coating on Ti6Al4V (TC4) by Radio Frequency Magnetron Sputtering for Prosthetic-Orthopaedic Implant Applications: Synthesis and Characterisation. Trans. IMF 98, 88–96. doi:10.1080/00202967.2020.1724718
Das, A., and Shukla, M. (2017). Surface Morphology Andin Vitrobioactivity of Biocompatible Hydroxyapatite Coatings on Medical Grade S31254 Steel by RF Magnetron Sputtering Deposition. Trans. IMF 95, 276–281. doi:10.1080/00202967.2017.1323675
Das, A., and Shukla, M. (2019). Surface Morphology, Bioactivity, and Antibacterial Studies of Pulsed Laser Deposited Hydroxyapatite Coatings on Stainless Steel 254 for Orthopedic Implant Applications. Proc. Inst. Mech. Eng. L: J. Mater. Des. Appl. 233, 120–127. doi:10.1177/1464420716663029
Dorozhkin, S. V. (2012). Calcium Orthophosphate Coatings, Films and Layers. Prog. Biomater. 1, 1. doi:10.1186/2194-0517-1-1
Drevet, R., Ben Jaber, N., Fauré, J., Tara, A., Ben Cheikh Larbi, A., and Benhayoune, H. (2016). Electrophoretic Deposition (EPD) of Nano-Hydroxyapatite Coatings with Improved Mechanical Properties on Prosthetic Ti6Al4V Substrates. Surf. Coat. Tech. 301, 94–99. doi:10.1016/j.surfcoat.2015.12.058
Durdu, S., Aktug, S. L., Aktas, S., Yalcin, E., Cavusoglu, K., Altinkok, A., et al. (2017). Characterization and In Vitro Properties of Anti-bacterial Ag-Based Bioceramic Coatings Formed on Zirconium by Micro Arc Oxidation and thermal Evaporation. Surf. Coat. Tech. 331, 107–115. doi:10.1016/j.surfcoat.2017.10.023
Durdu, S., Aktug, S. L., Aktas, S., Yalcin, E., and Usta, M. (2018). Fabrication and In Vitro Properties of Zinc-Based Superhydrophilic Bioceramic Coatings on Zirconium. Surf. Coat. Tech. 344, 467–478. doi:10.1016/j.surfcoat.2018.03.062
Farris, S., Introzzi, L., Biagioni, P., Holz, T., Schiraldi, A., and Piergiovanni, L. (2011). Wetting of Biopolymer Coatings: Contact Angle Kinetics and Image Analysis Investigation. Langmuir 27, 7563–7574. doi:10.1021/la2017006
Fathi, M. H., Salehi, M., Saatchi, A., Mortazavi, V., and Moosavi, S. B. (2003). In Vitro corrosion Behavior of Bioceramic, Metallic, and Bioceramic-Metallic Coated Stainless Steel Dental Implants. Dental Mater. 19, 188–198. doi:10.1016/S0109-5641(02)00029-5
Fedotkin, A. Y., Bolbasov, E. N., Kozelskaya, A. I., Dubinenko, G., Shesterikov, E. V., Ashrafov, A., et al. (2019). Calcium Phosphate Coating Deposition by Radio Frequency Magnetron Sputtering in the Various Inert Gases: The Pilot Study. Mater. Chem. Phys. 235, 121735. doi:10.1016/j.matchemphys.2019.121735
Furko, M., Jiang, Y., Wilkins, T. A., and Balázsi, C. (2016a). Electrochemical and Morphological Investigation of Silver and Zinc Modified Calcium Phosphate Bioceramic Coatings on Metallic Implant Materials. Mater. Sci. Eng. C 62, 249–259. doi:10.1016/j.msec.2016.01.060
Furko, M., Jiang, Y., Wilkins, T., and Balázsi, C. (2016b). Development and Characterization of Silver and Zinc Doped Bioceramic Layer on Metallic Implant Materials for Orthopedic Application. Ceramics Int. 42, 4924–4931. doi:10.1016/j.ceramint.2015.12.006
Gao, C., Peng, S., Feng, P., and Shuai, C. (2017). Bone Biomaterials and Interactions with Stem Cells. Bone Res. 5, 1–33. doi:10.1038/boneres.2017.59
Goreninskii, S. I., Bogomolova, N. N., Malchikhina, A. I., Golovkin, A. S., Bolbasov, E. N., Safronova, T. V., et al. (2017). Biological Effect of the Surface Modification of the Fibrous Poly(L-Lactic Acid) Scaffolds by Radio Frequency Magnetron Sputtering of Different Calcium-Phosphate Targets. BioNanoSci. 7, 50–57. doi:10.1007/s12668-016-0383-x
Graziani, G., Bianchi, M., Sassoni, E., Russo, A., and Marcacci, M. (2017). Ion-substituted Calcium Phosphate Coatings Deposited by Plasma-Assisted Techniques: A Review. Mater. Sci. Eng. C 74, 219–229. doi:10.1016/j.msec.2016.12.018
Gul, H., Khan, M., and Khan, A. S. (2020). Bioceramics: Types and Clinical Applications. Types and Clinical Applications. Elsevier, 53–83. doi:10.1016/B978-0-08-102834-6.00003-3
Hamdi, D. A., Jiang, Z.-T., No, K., Rahman, M. M., Lee, P.-C., Truc, L. N. T., et al. (2019). Biocompatibility Study of Multi-Layered Hydroxyapatite Coatings Synthesized on Ti-6Al-4V Alloys by RF Magnetron Sputtering for Prosthetic-Orthopaedic Implant Applications. Appl. Surf. Sci. 463, 292–299. doi:10.1016/j.apsusc.2018.08.157
Heimann, R. B. (2017). 15. Hydroxylapatite Coatings: Applied Mineralogy Research in the Bioceramics Field. Highlights Appl. Mineral., 301–316. doi:10.1515/9783110497342-015
Heimann, R. B., and Lehmann, H. D. Bio-Ceramics with Clinical Bio-Glasses – an Introduction Biomimetic Approaches for Biomaterials Development Bio-Nanomaterials Ceramics Science and.
Heimann, R. B. (2013). Structure, Properties, and Biomedical Performance of Osteoconductive Bioceramic Coatings. Surf. Coat. Tech. 233, 27–38. doi:10.1016/j.surfcoat.2012.11.013
Herschke, L., Rottstegge, J., Lieberwirth, I., and Wegner, G. (2006). Zinc Phosphate as Versatile Material for Potential Biomedical Applications Part 1. J. Mater. Sci. Mater. Med. 17, 81–94. doi:10.1007/s10856-006-6332-4
Ibrahim, M. Z., Sarhan, A. A. D., Yusuf, F., and Hamdi, M. (2017). Biomedical Materials and Techniques to Improve the Tribological, Mechanical and Biomedical Properties of Orthopedic Implants - A Review Article. J. Alloys Compd. 714, 636–667. doi:10.1016/j.jallcom.2017.04.231
Ivanova, A. A., Surmeneva, M. A., Tyurin, A. I., and Surmenev, R. A. (2018). Correlation between Structural and Mechanical Properties of RF Magnetron Sputter Deposited Hydroxyapatite Coating. Mater. Characterization 142, 261–269. doi:10.1016/j.matchar.2018.05.042
Jeong, Y.-H., Choe, H.-C., and Brantley, W. A. (2016). Hydroxyapatite-silicon Film Deposited on Ti-Nb-10Zr by Electrochemical and Magnetron Sputtering Method. Thin Solid Films 620, 114–118. doi:10.1016/j.tsf.2016.07.086
Kaur, G., Kumar, V., Baino, F., Mauro, J. C., Pickrell, G., Evans, I., et al. (2019). Mechanical Properties of Bioactive Glasses, Ceramics, Glass-Ceramics and Composites: State-Of-The-Art Review and Future Challenges. Mater. Sci. Eng. C 104, 109895. doi:10.1016/j.msec.2019.109895
Kozelskaya, A. I., Bolbasov, E. N., Golovkin, A. S., Mishanin, A. I., Viknianshchuk, A. N., Shesterikov, E. V., et al. (2018). Modification of the Ceramic Implant Surfaces from Zirconia by the Magnetron Sputtering of Different Calcium Phosphate Targets: A Comparative Study. Materials 11, 1949. doi:10.3390/ma11101949
Kumar, D. D., Kaliaraj, G. S., Kirubaharan, A. M. K., Alagarsamy, K., Vishwakarma, V., and Baskaran, R. (2019). Biocorrosion and Biological Properties of Sputtered Ceramic Carbide Coatings for Biomedical Applications. Surf. Coat. Tech. 374, 569–578. doi:10.1016/j.surfcoat.2019.06.022
Lee, J. H., Jang, H. L., Lee, K. M., Baek, H.-R., Jin, K., Hong, K. S., et al. (2013). In Vitro and In Vivo Evaluation of the Bioactivity of Hydroxyapatite-Coated Polyetheretherketone Biocomposites Created by Cold spray Technology. Acta Biomater. 9, 6177–6187. doi:10.1016/j.actbio.2012.11.030
Lee, T. C., Abdullah, H. Z., Koshy, P., and Idris, M. I. (2018). Ultraviolet-assisted Biomimetic Coating of Bone-like Apatite on Anodised Titanium for Biomedical Applications. Thin Solid Films 660, 191–198. doi:10.1016/j.tsf.2018.06.021
Lenis, J. A., Gómez, M. A., and Bolívar, F. J. (2019). Effect of Deposition Temperature and Target-Substrate Distance on the Structure, Phases, Mechanical and Tribological Properties of Multi-Layer HA-Ag Coatings Obtained by RF Magnetron Sputtering. Surf. Coat. Tech. 378, 124936. doi:10.1016/j.surfcoat.2019.124936
Li, S., Yu, W., Zhang, W., Zhang, G., Yu, L., and Lu, E. (2018). Evaluation of Highly Carbonated Hydroxyapatite Bioceramic Implant Coatings with Hierarchical Micro-/nanorod Topography Optimized for Osseointegration. Int. J. Nanomedicine 13, 3643–3659. doi:10.2147/IJN.S159989
Liu, Y., Groot, K. D., and Hunziker, E. B. (2004). Osteoinductive Implants: The Mise-En-Scène for Drug-Bearing Biomimetic Coatings. Ann. Biomed. Eng. 32, 398–406. doi:10.1023/B:ABME.0000017536.10767.0f
Liu, Y., Zheng, Y., Chen, X. H., Yang, J. A., Pan, H., Chen, D., et al. (2019). Fundamental Theory of Biodegradable Metals-Definition, Criteria, and Design. Adv. Funct. Mater. 29, 1805402–1805421. doi:10.1002/adfm.201805402
Love, C. A., Cook, R. B., Harvey, T. J., Dearnley, P. A., and Wood, R. J. K. (2013). Diamond like Carbon Coatings for Potential Application in Biological Implants-A Review. Tribology Int. 63, 141–150. doi:10.1016/j.triboint.2012.09.006
Mahapatro, A., and Arshanapalli, S. A. (2017). Bioceramic Coatings on Magnesium Alloys. J. Bio Tribo Corros 3, 1–9. doi:10.1007/s40735-017-0099-7
Mali, S. A., Nune, K. C., and Misra, R. D. K. (2016). Biomimetic Nanostructured Hydroxyapatite Coatings on Metallic Implant Materials. Mater. Tech. 31, 782–790. doi:10.1080/10667857.2016.1224609
Manivasagam, G., Dhinasekaran, D., and Rajamanickam, A. (2010). Biomedical Implants: Corrosion and its Prevention - A Review∼!2009-12-22∼!2010-01-20∼!2010-05-25∼!. Recent Patents Corrosion Sci. 2, 40–54. doi:10.2174/1877610801002010040
Mansoorianfar, M., Mansourianfar, M., Fathi, M., Bonakdar, S., Ebrahimi, M., Zahrani, E. M., et al. (2020). Surface Modification of Orthopedic Implants by Optimized Fluorine-Substituted Hydroxyapatite Coating: Enhancing Corrosion Behavior and Cell Function. Ceramics Int. 46, 2139–2146. doi:10.1016/j.ceramint.2019.09.197
Menzies, K. L., and Jones, L. (2010). The Impact of Contact Angle on the Biocompatibility of Biomaterials. Optom. Vis. Sci. 87, 387–399. doi:10.1097/OPX.0b013e3181da863e
Mohammed, M. T., and Hussein, S. M. (2019). Synthesis and Characterization of Nanocoatings Derived by Sol-Gel onto a New Surgical Titanium Surface. Mater. Res. Express 6, 076426. doi:10.1088/2053-1591/ab1722
Nawaz, A., Bano, S., Yasir, M., Wadood, A., Ur Rehman, M. A., and Rehman, M. A. U. (2020). Ag and Mn-Doped Mesoporous Bioactive Glass Nanoparticles Incorporated into the Chitosan/gelatin Coatings Deposited on PEEK/bioactive Glass Layers for Favorable Osteogenic Differentiation and Antibacterial Activity. Mater. Adv. 1, 1273–1284. doi:10.1039/D0MA00325E
Nawaz, A., and Ur Rehman, M. A. (2021). Chitosan/gelatin‐based Bioactive and Antibacterial Coatings Deposited via Electrophoretic Deposition. J. Appl. Polym. Sci. 138, 50220. doi:10.1002/app.50220
Nimbalkar, S., Dhatrak, P., Gherde, C., and Joshi, S. (2021). A Review Article on Factors Affecting Bone Loss in Dental Implants. Mater. Today Proc. 43, 970–976. doi:10.1016/j.matpr.2020.07.428
Oh, S., Oh, N., Appleford, M., and Ong, J. L. (2006). Bioceramics for Tissue Engineering Applications €" A Review. Am. J. Biochem. Biotechnol. 2, 49–56. doi:10.3844/ajbbsp.2006.49.56
Pandey, A., Awasthi, A., and Saxena, K. K. (2020). Metallic Implants with Properties and Latest Production Techniques: a Review. Adv. Mater. Process. Tech. 6, 405–440. doi:10.1080/2374068X.2020.1731236
Pantaroto, H. N., Cordeiro, J. M., Pereira, L. T., de Almeida, A. B., Nociti Junior, F. H., Rangel, E. C., et al. (2021). Sputtered Crystalline TiO2 Film Drives Improved Surface Properties of Titanium-Based Biomedical Implants. Mater. Sci. Eng. C 119, 111638. doi:10.1016/j.msec.2020.111638
Patel, K. D., El-Fiqi, A., Lee, H.-Y., Singh, R. K., Kim, D.-A., Lee, H.-H., et al. (2012). Chitosan-nanobioactive Glass Electrophoretic Coatings with Bone Regenerative and Drug Delivering Potential. J. Mater. Chem. 22, 24945. doi:10.1039/c2jm33830k
Patel, K. D., Kim, T.-H., Lee, E.-J., Han, C.-M., Lee, J.-Y., Singh, R. K., et al. (2014a). Nanostructured Biointerfacing of Metals with Carbon Nanotube/chitosan Hybrids by Electrodeposition for Cell Stimulation and Therapeutics Delivery. ACS Appl. Mater. Inter. 6, 20214–20224. doi:10.1021/am505759p
Patel, K. D., Singh, R. K., Lee, E.-J., Han, C.-M., Won, J.-E., Knowles, J. C., et al. (2014b). Tailoring Solubility and Drug Release from Electrophoretic Deposited Chitosan-Gelatin Films on Titanium. Surf. Coat. Tech. 242, 232–236. doi:10.1016/j.surfcoat.2013.11.049
Patel, K. D., Singh, R. K., Lee, J.-H., and Kim, H.-W. (2019). Electrophoretic Coatings of Hydroxyapatite with Various Nanocrystal Shapes. Mater. Lett. 234, 148–154. doi:10.1016/j.matlet.2018.09.066
Patel, K. D., Singh, R. K., Mahapatra, C., Lee, E.-J., and Kim, H.-W. (2016). Nanohybrid Electro-Coatings toward Therapeutic Implants with Controlled Drug Delivery Potential for Bone Regeneration. J. Biomed. Nanotechnol. 12, 1876–1889. doi:10.1166/jbn.2016.2301
Pawlik, A., Rehman, M. A. U., Nawaz, Q., Bastan, F. E., Sulka, G. D., Boccaccini, A. R., et al. (2019). Fabrication and Characterization of Electrophoretically Deposited Chitosan-Hydroxyapatite Composite Coatings on Anodic Titanium Dioxide Layers. Electrochimica Acta 307, 465–473. doi:10.1016/j.electacta.2019.03.195
Pezzotti, G. (2021). Surface Chemistry of Bioceramics: The Missing Key. Elsevier, 297–324. doi:10.1016/b978-0-08-102999-2.00013-2
Pina, S., Reis, R. L., and Oliveira, J. M. (2018). Ceramic Biomaterials for Tissue Engineering. Elsevier, 95–116. doi:10.1016/B978-0-08-102203-0.00004-4
Prasad, K., Bazaka, O., Chua, M., Rochford, M., Fedrick, L., Spoor, J., et al. (2017). Metallic Biomaterials: Current Challenges and Opportunities. Materials 10, 884. doi:10.3390/ma10080884
Prosolov, K. A., Belyavskaya, O. A., Bolat-Ool, A. A., Khlusov, I. A., Nikolaeva, O. A., Prosolov, A. B., et al. (2019a). Antibacterial Potential of Zn- and Cu-Substituted Hydroxyapatite-Based Coatings Deposited by RF-Magnetron Sputtering. J. Phys. Conf. Ser. 1393, 012118. doi:10.1088/1742-6596/1393/1/012118
Prosolov, K. A., Belyavskaya, O. A., Rau, J. V., Prymak, O., Epple, M., and Sharkeev, Y. P. (2018). Deposition of Polycrystalline Zinc Substituted Hydroxyapatite Coatings with a Columnar Structure by RF Magnetron Sputtering: Role of Iin-Ssitu Substrate Heating. J. Phys. Conf. Ser. 1115, 032077. doi:10.1088/1742-6596/1115/3/032077
Prosolov, K. A., Popova, K. S., Belyavskaya, O. A., Rau, J. V., Gross, K. A., Ubelis, A., et al. (2017). RF Magnetron-Sputtered Coatings Deposited from Biphasic Calcium Phosphate Targets for Biomedical Implant Applications. Bioactive Mater. 2, 170–176. doi:10.1016/j.bioactmat.2017.07.003
Prosolov, K., Belyavskaya, O., Linders, J., Loza, K., Prymak, O., Mayer, C., et al. (2019b). Glancing Angle Deposition of Zn-Doped Calcium Phosphate Coatings by RF Magnetron Sputtering. Coatings 9 (4), 220. doi:10.3390/coatings9040220
Qadir, M., Li, Y., and Wen, C. (2019). Ion-substituted Calcium Phosphate Coatings by Physical Vapor Deposition Magnetron Sputtering for Biomedical Applications: A Review. Acta Biomater. 89, 14–32. doi:10.1016/j.actbio.2019.03.006
Qaid, T. H., Ramesh, S., Yusof, F., Basirun, W. J., Ching, Y. C., Chandran, H., et al. (2019). Micro-arc Oxidation of Bioceramic Coatings Containing Eggshell-Derived Hydroxyapatite on Titanium Substrate. Ceramics Int. 45, 18371–18381. doi:10.1016/j.ceramint.2019.06.052
Qi, J., Yang, Y., Zhou, M., Chen, Z., and Chen, K. (2019). Effect of Transition Layer on the Performance of Hydroxyapatite/titanium Nitride Coating Developed on Ti-6Al-4V alloy by Magnetron Sputtering. Ceramics Int. 45, 4863–4869. doi:10.1016/j.ceramint.2018.11.183
Quirama, A., Echavarría, A. M., Meza, J. M., Osorio, J., and Bejarano G, G. (2017). Improvement of the Mechanical Behavior of the Calcium Phosphate Coatings Deposited onto Ti6Al4V alloy Using an Intermediate TiN/TiO2 Bilayer. Vacuum 146, 22–30. doi:10.1016/j.vacuum.2017.09.024
Razavi, M., Fathi, M., Savabi, O., Tayebi, L., and Vashaee, D. (2020). Biodegradable Magnesium Bone Implants Coated with a Novel Bioceramic Nanocomposite. Materials 13, 1315–15. doi:10.3390/ma13061315
Rehman, M. A. U., Munawar, M. A., Schubert, D. W., Boccaccini, A. R., Atiq, M., Rehman, U., et al. (2019). Electrophoretic Deposition of Chitosan/gelatin/bioactive Glass Composite Coatings on 316L Stainless Steel: A Design of experiment Study. Surf. Coat. Tech. 358, 976–986. doi:10.1016/j.surfcoat.2018.12.013
Riau, A. K., Mondal, D., Setiawan, M., Palaniappan, A., Yam, G. H. F., Liedberg, B., et al. (2016). Functionalization of the Polymeric Surface with Bioceramic Nanoparticles via a Novel, Nonthermal Dip Coating Method. ACS Appl. Mater. Inter. 8, 35565–35577. doi:10.1021/acsami.6b12371
Rizwan, M., Genasan, K., Murali, M. R., Balaji Raghavendran, H. R., Alias, R., Cheok, Y. Y., et al. (2020). In Vitro evaluation of Novel Low-Pressure Spark Plasma Sintered HA-BG Composite Scaffolds for Bone Tissue Engineering. RSC Adv. 10, 23813–23828. doi:10.1039/d0ra04227g
Rizwan, M., Hamdi, M., and Basirun, W. J. (2017). Bioglass 45S5-Based Composites for Bone Tissue Engineering and Functional Applications. J. Biomed. Mater. Res. 105, 3197–3223. doi:10.1002/jbm.a.36156
Safavi, M. S., Surmeneva, M. A., Surmenev, R. A., and Khalil-Allafi, J. (2021). RF-magnetron Sputter Deposited Hydroxyapatite-Based Composite & Multilayer Coatings: A Systematic Review from Mechanical, Corrosion, and Biological Points of View. Ceramics Int. 47, 3031–3053. doi:10.1016/j.ceramint.2020.09.274
Samiee, M., Hanachi, M., Seyedraoufi, Z. S., Eshraghi, M. J., and Shajari, Y. (2021). Biodegradable Magnesium alloy Coated with TiO2/MgO Two-Layer Composite via Magnetic Sputtering for Orthopedic Applications: A Study on the Surface Characterization, Corrosion, and Biocompatibility. Ceramics Int. 47, 6179–6186. doi:10.1016/j.ceramint.2020.10.196
Sebastin, A. X. S., and Uthirapathy, V. (2020). In Vitro Electrochemical Behavior of Sol‐Gel Derived Hydroxyapatite/Graphene Oxide Composite Coatings on 316L SS for Biomedical Applications. ChemistrySelect 5, 12140–12147. doi:10.1002/slct.202003368
Shi, J. Z., Chen, C. Z., Yu, H. J., and Zhang, S. J. (2008). Application of Magnetron Sputtering for Producing Bioactive Ceramic Coatings on Implant Materials. Bull. Mater. Sci. 31, 877–884. doi:10.1007/s12034-008-0140-z
Shukla, K., Rane, R., Alphonsa, J., Maity, P., and Mukherjee, S. (2017). Structural, Mechanical and Corrosion Resistance Properties of Ti/TiN Bilayers Deposited by Magnetron Sputtering on AISI 316L. Surf. Coat. Tech. 324, 167–174. doi:10.1016/j.surfcoat.2017.05.075
Singh, J., Chatha, S. S., and Singh, H. (2021). Synthesis and Characterization of Plasma Sprayed Functional Gradient Bioceramic Coating for Medical Implant Applications. Ceramics Int. 47, 9143–9155. doi:10.1016/j.ceramint.2020.12.039
Singh, R. K., Jin, G.-Z., Mahapatra, C., Patel, K. D., Chrzanowski, W., and Kim, H.-W. (2015). Mesoporous Silica-Layered Biopolymer Hybrid Nanofibrous Scaffold: A Novel Nanobiomatrix Platform for Therapeutics Delivery and Bone Regeneration. ACS Appl. Mater. Inter. 7, 8088–8098. doi:10.1021/acsami.5b00692
Singh, S., Prakash, C., and Singh, H. (2020). Deposition of HA-TiO2 by Plasma spray on β-phase Ti-35Nb-7Ta-5Zr alloy for Hip Stem: Characterization, Mechanical Properties, Corrosion, and Iin-Vvitro Bioactivity. Surf. Coat. Tech. 398, 126072. doi:10.1016/j.surfcoat.2020.126072
Su, Y., Cockerill, I., Zheng, Y., Tang, L., Qin, Y.-X., and Zhu, D. (2019). Biofunctionalization of Metallic Implants by Calcium Phosphate Coatings. Bioactive Mater. 4, 196–206. doi:10.1016/j.bioactmat.2019.05.001
Surmeneva, M. A., Ivanova, A. A., Tian, Q., Pittman, R., Jiang, W., Lin, J., et al. (2019). Bone Marrow Derived Mesenchymal Stem Cell Response to the RF Magnetron Sputter Deposited Hydroxyapatite Coating on AZ91 Magnesium alloy. Mater. Chem. Phys. 221, 89–98. doi:10.1016/j.matchemphys.2018.09.030
Tverdokhlebov, S. I., Bolbasov, E. N., Shesterikov, E. V., Malchikhina, A. I., Novikov, V. A., and Anissimov, Y. G. (2012). Research of the Surface Properties of the Thermoplastic Copolymer of Vinilidene Fluoride and Tetrafluoroethylene Modified with Radio-Frequency Magnetron Sputtering for Medical Application. Appl. Surf. Sci. 263, 187–194. doi:10.1016/j.apsusc.2012.09.025
Ur Rehman, M. A., Bastan, F. E., Nawaz, A., Nawaz, Q., and Wadood, A. (2020). Electrophoretic Deposition of PEEK/bioactive Glass Composite Coatings on Stainless Steel for Orthopedic Applications: an Optimization for In Vitro Bioactivity and Adhesion Strength. Int. J. Adv. Manuf. Technol. 108, 1849–1862. doi:10.1007/s00170-020-05456-x
Ur Rehman, M. A., Ferraris, S., Goldmann, W. H., Perero, S., Bastan, F. E., Nawaz, Q., et al. (2017). Antibacterial and Bioactive Coatings Based on Radio Frequency Co-sputtering of Silver Nanocluster-Silica Coatings on PEEK/Bioactive Glass Layers Obtained by Electrophoretic Deposition. ACS Appl. Mater. Inter. 9, 32489–32497. doi:10.1021/acsami.7b08646
Ureña, J., Tsipas, S., Jiménez-Morales, A., Gordo, E., Detsch, R., and Boccaccini, A. R. (2018). Cellular Behaviour of Bone Marrow Stromal Cells on Modified Ti-Nb Surfaces. Mater. Des. 140, 452–459. doi:10.1016/j.matdes.2017.12.006
Vladescu, A., Mihai Cotrut, C., Ak Azem, F., Bramowicz, M., Pana, I., Braic, V., et al. (2018). Sputtered Si and Mg Doped Hydroxyapatite for Biomedical Applications. Biomed. Mater. 13, 025011. doi:10.1088/1748-605X/aa9718
Wegst, U. G. K., Bai, H., Saiz, E., Tomsia, A. P., and Ritchie, R. O. (2015). Bioinspired Structural Materials. Nat. Mater 14, 23–36. doi:10.1038/nmat4089
Wu, L., Zhou, C., Zhang, B., Lei, H., Wang, W., Pu, X., et al. (2020). Construction of Biomimetic Natural Wood Hierarchical Porous-Structure Bioceramic with Micro/Nanowhisker Coating to Modulate Cellular Behavior and Osteoinductive Activity. ACS Appl. Mater. Inter. 12, 48395–48407. doi:10.1021/acsami.0c15205
Xiong, Y., Hu, Q., Hu, X., and Song, R. (2017). Microstructure and Corrosion Resistance of Ti 3 O 5 -HA Bio-Ceramic Coating Fabricated on AZ80 Magnesium alloy. Surf. Coat. Tech. 325, 239–247. doi:10.1016/j.surfcoat.2017.06.055
Xiong, Y., Yang, Z., Hu, X., and Song, R. (2019). Bioceramic Coating Produced on AZ80 Magnesium Alloy by One-step Microarc Oxidation Process. J. Materi Eng. Perform. 28, 1719–1727. doi:10.1007/s11665-019-03925-3
Yang, Y., He, C., Dianyu E, E., Yang, W., Qi, F., Xie, D., et al. (2020). Mg Bone Implant: Features, Developments and Perspectives. Mater. Des. 185, 108259. doi:10.1016/j.matdes.2019.108259
Zafar, M. S., Farooq, I., Awais, M., Najeeb, S., Khurshid, Z., and Zohaib, S. (2019). Bioactive Surface Coatings for Enhancing Osseointegration of Dental Implants. Elsevier, 313–329. doi:10.1016/B978-0-08-102196-5.00011-2
Zeng, Y., Pei, X., Yang, S., Qin, H., Cai, H., Hu, S., et al. (2016). Graphene Oxide/hydroxyapatite Composite Coatings Fabricated by Electrochemical Deposition. Surf. Coat. Tech. 286, 72–79. doi:10.1016/j.surfcoat.2015.12.013
Zhang, B., Myers, D., Wallace, G., Brandt, M., and Choong, P. (2014). Bioactive Coatings for Orthopaedic Implants-Recent Trends in Development of Implant Coatings. Int. J. Mol. Sci. 15, 11878–11921. doi:10.3390/ijms150711878
Zheng, Y. F., Gu, X. N., and Witte, F. (2014). Biodegradable Metals. Mater. Sci. Eng. R: Rep. 77, 1–34. doi:10.1016/j.mser.2014.01.001
Keywords: bioceramics (BC), calcium phosphates (CaPs), magnetron sputtering, composite coatings thickness, biocompatibile
Citation: Akhtar M, Uzair SA, Rizwan M and Ur Rehman MA (2022) The Improvement in Surface Properties of Metallic Implant via Magnetron Sputtering: Recent Progress and Remaining Challenges. Front. Mater. 8:747169. doi: 10.3389/fmats.2021.747169
Received: 25 July 2021; Accepted: 13 December 2021;
Published: 14 January 2022.
Edited by:
Asma Tufail Shah, COMSATS University Islamabad, Lahore Campus, PakistanReviewed by:
Rajendra Kumar Singh, Institute of Tissue Regeneration Engineering (ITREN), South KoreaMuhammad Awais, Taibah University, Saudi Arabia
Copyright © 2022 Akhtar, Uzair, Rizwan and Ur Rehman. This is an open-access article distributed under the terms of the Creative Commons Attribution License (CC BY). The use, distribution or reproduction in other forums is permitted, provided the original author(s) and the copyright owner(s) are credited and that the original publication in this journal is cited, in accordance with accepted academic practice. No use, distribution or reproduction is permitted which does not comply with these terms.
*Correspondence: Muhammad Rizwan, bWF0ZXJpYWxpc3Qucml6QGdtYWlsLmNvbQ==; Muhammad Atiq Ur Rehman, YXRpcXVlMS4xQGhvdG1haWwuY29t