- 1Department of Obstetrics and Gynecology, Shengjing Hospital of China Medical University, Shenyang, China
- 2Center for Molecular Science and Engineering, College of Science, Northeastern University, Shenyang, China
- 3NHC Key Laboratory of Reproductive Health and Medical Genetics (Liaoning Research Institute of Family Planning), China Medical University, Shenyang, China
- 4Department of Cell Biology, Key Laboratory of Cell Biology, Ministry of Public Health, and Key Laboratory of Medical Cell Biology, Ministry of Education, China Medical University, Shenyang, China
To more precisely control the degradation rate of poly(trimethylene carbonate) (PTMC), self-blending films were prepared via the ternary self-blending of pure PTMC with a molecular weight of 334, 152, and 57 kg/mol. The in vitro enzymolysis degradation of the ternary self-blending films was performed in lipase solutions. The results showed that ternary self-blending could control the degradation of PTMC by adjusting the mass ratio of high/medium/low molecular weight PTMC in the composition, and the PTMC334/PTMC152/PTMC57 films with a mass ratio of 1/4/16 showed mass loss of 85.96% after seven weeks of degradation, while that of PTMC334/PTMC152/PTMC57 films with a mass ratio of 1/1/1 was 96.39%. The former and latter’s degradation rate constant was 13.263 and 23.981%/w, respectively, and the former presented better morphology stability than the latter. The strategy of ternary self-blending could simultaneously bestow PTMC with a lower degradation rate and good morphology stability, indicating that ternary self-blending is an efficient way to control the degradation performance of PTMC more precisely.
Introduction
Biomaterials play a critical role in tissue engineering and drug delivery systems (Woodruff and Hutmacher, 2010; Danhier et al., 2012; Raquez et al., 2013). As one of the essential biomaterials, poly(trimethylene carbonate) (PTMC) has also fascinated the extensive attention of researchers given its excellent biocompatibility and well biodegradation behavior, showing great potential applications in biomedical fields (Li et al., 2020; Mohajeri et al., 2020). Owing to its advantage of no acidic degradation products, PTMC is particularly suitable as a carrier for biodegradable contraceptive implants (Yang et al., 2012; Yang et al., 2013). However, the incompatibility between the morphology stability and degradation rate of PTMC hinders its application in biodegradable long-term contraceptive implants. Specifically, PTMC of low molecular weight presents a slower degradation rate while it deforms easily at room temperature (Zhang et al., 2006), usually causing an explosive release, which is undesirable in the implant systems. The increase in molecular weight can strengthen the morphology stability of PTMC, which also could result in a faster degradation rate (Yang et al., 2015a), leading to a failure in the achievement of long-term applications. Hence, it is necessary to simultaneously endow PTMC with a lower degradation rate and good morphology stability, making it the most promising carrier for biodegradable long-term contraceptive implants.
Significant efforts have been devoted to this aim. Copolymerization is one of the most efficient strategies to improve polymer properties. It has been reported that poly(ε-caprolactone) (PCL) has a slow degradation rate and good mechanical properties in vivo due to its semicrystalline nature. Therefore, it will reduce the degradation rate and heighten the morphology stability of PTMC when introducing PCL segments into the structure of PTMC (Yang et al., 2014a). Similar observations also have been found for the copolymers of trimethylene carbonate (TMC) and 5,5-dimethyl-trimethylene carbonate (DTC) (Hou et al., 2019; Hou et al., 2020). In addition, cross-linking could form a three-dimensional network structure between cross-linking points, which has been demonstrated as an effective strategy to improve the morphology stability and decrease the degradation rate of PTMC (Yang et al., 2014b; Yang et al., 2016; Hou et al., 2017).
Although the strategies mentioned above could tailor the degradation rate and morphology stability, they also influence the comprehensive properties of PTMC. For instance, an undesired late inflammatory response might be evoked by the crystalline fragments formed during the degradation of PTMC copolymers (Bos et al., 1991). Hence, it is necessary to develop a more effective and appropriate method to tailor the degradation rate and morphology stability of PTMC. Blending is another common strategy to improve the performance of polymers (Han et al., 2010; Joy et al., 2020), and the more significant advantage of self-blending is that it does not introduce other components while improving polymer properties, totally remaining the performance advantages of polymer without adverse effects. In our previous work, binary self-blending of PTMC was performed with high molecular weight PTMC and low molecular weight PTMC. The results of in vitro enzymatic degradation showed that the degradation rate and morphology stability of the binary self-blending films could be regulated d by the mass ratio of high molecular weight PTMC to low molecular weight PTMC (Hou et al., 2021), indicating that binary self-blending is an efficient strategy to handle the degradation properties of PTMC.
Ternary self-blending was performed to the high/medium/low molecular weight PTMC in this study to obtain the ternary self-blending films. The in vitro enzymatic degradation of the obtained ternary self-blending films was performed in lipase solutions to investigate the role of ternary self-blending in the degradation rate and morphology stability of PTMC, with the aim of more precisely controlling the degradation behaviors of PTMC to meet the requirements of being a carrier for the biodegradable long-term contraceptive implants.
Experimental Section
Materials
TMC monomer was purchased from Daigang Biomaterial Co., Ltd. (Jinan, Shandong, China), recrystallized twice with ethyl acetate before use, and dried under vacuum at 37°C to remove the solvent. Sn(Oct)2 (95%) and lipase solutions (from Thermomyces lanuginosus, ≥100,000 U/g) were purchased from Sigma-Aldrich and used as received without any treatment. All other solvents and reagents were of analytical grade and used as provided except where noted.
Methods
Synthesis of PTMC
PTMC of different molecular weights were prepared as described previously (Yang et al., 2015a). The bulk ring-opening polymerization (ROP) of TMC was carried out in a heat-sealed glass tube under a high vacuum at 130 ± 2°C for 24 h using Sn(Oct)2 as a catalyst. After polymerization, all simple polymers were removed from the glass tube and dissolved in chloroform, precipitated and purified in cold methanol, and dried at 37°C under vacuum. The synthesized homopolymer and TMC monomer conversion were characterized from 1H NMR spectra obtained in CDCl3 on a Bruker ARX 300 (Bruker, Zurich, Switzerland) using tetramethylsilane (TMS) as an internal standard. Number-average (Mn) and polydispersity indices (PDI) of PTMC homopolymers were determined by GPC (Waters, Milford, MA, United States), and the sample was eluted at 35°C with THF at a rate of 1 ml/min. Values are calculated using polystyrene as a standard.
Preparation of PTMC Films and the Ternary Self-Blending Films
PTMC films and the ternary self-blending films were fabricated by solvent casting. In short, PTMC homopolymers of different molecular weights were dissolved separately in chloroform or self-blended according to the preset mass ratios in chloroform and then were transferred to Teflon® dishes. After vacuum drying to constant weight, the films were obtained using a puncher. The thermal properties of the homopolymer and self-blending films were evaluated using Netzsch DSC 200 F3 (Netzsch, Selb, Germany). The test temperature was −100–100°C, with a 10°C/min heating rate under a nitrogen flow.
In Vitro Enzymatic Degradation Study
The enzymolysis degradation of PTMC films and the ternary self-blending films (10 mm diameter; approximately 2 mm thickness) was performed in lipase (from Thermomyces lanuginosus, ≥100,000 U/g). The films were put into glass tubes containing 500 μL lipase solutions and conditioned at 37°C with gentle shaking for 8 h per day. The degradation medium was refreshed twice a week. Two parallel samples were removed at one-week intervals, washed with distilled water, and blotted dry. Subsequently, the related measurements were carried out after drying the films to constant weight under vacuum. The mass loss of the samples was obtained according to the following equations:
wi represents the initial weight of the sample before degradation, and wd represents the dry weight of the piece after degradation.
The microscopic morphologies of film surface were analyzed by SEM (HITACHI SU8010, Chiyoda-ku, Tokyo, Japan) after sputter coating the samples with gold using an ion sputtering apparatus. The pH of the degradation medium was measured using a Toledo-Mettler InLabMicro™ pH meter with a microelectrode (Toledo-Mettler, Zurich, Switzerland).
Results and Discussion
PTMC Synthesis
As shown in Table 1, PTMC homopolymers of different molecular weights and broad polydispersity indices were prepared by ROP of TMC in this study, and the high/medium/low molecular weight was 334, 152, and 57 kg/mol, respectively. 1H NMR test results indicated nearly complete monomer conversion during polymerization.
Preparation of PTMC Films and the Ternary Self-Blending Films
PTMC films and the ternary self-blending films were fabricated by solvent casting. After drying, the obtained PTMC films were transparent, which was led by the amorphous nature of PTMC, as shown by the DSC curve without melting peak. The glass transition temperature (Tg) increased from −17.8 to −14.4°C as the molecular weight raised from 57 to 334 kDa (Table 2). The ternary self-blending films were also amorphous and transparent, and the Tg values were in the range of −16.0∼−16.8°C and decreased slightly as the mass ratio of low molecular weight PTMC increased in the composition. The low Tg value makes the ternary self-blending films flexible at physiological temperature and more suitable for subcutaneous tissues.
In Vitro Enzymatic Degradation
Enzymes such as lipase are capable of degrading polycarbonate polymers. In this study, the degradation behaviors of the ternary self-blending films were investigated in lipase solutions (from Thermomyces lanuginosus, ≥100,000 U/g), with high/medium/low molecular weight PTMC films as a straightforward comparison. Figure 1 shows the changes in mass loss of the ternary self-blending films and PTMC films with degradation time.
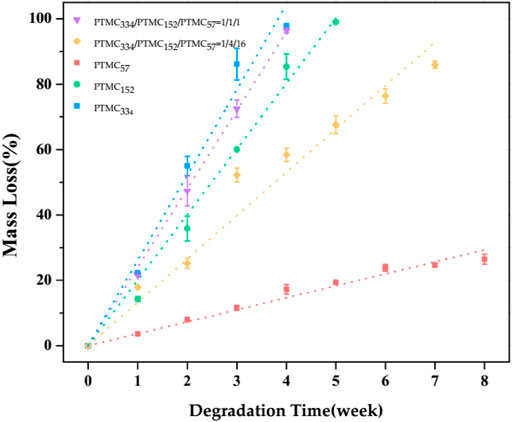
FIGURE 1. The mass loss of PTMC films and the ternary self-blending films as a function of degradation time.
As shown in Figure 1, the mass loss of the ternary self-blending films and PTMC films presented an excellent linear relationship with the degradation time. The high molecular weight PTMC334 films gave the fastest degradation with a mass loss of 97.95% after four weeks of degradation, and the medium molecular weight PTMC152 films showed relatively slower degradation than PTMC334 films and lost their initial mass of 85.36% after four weeks of degradation. The low molecular weight PTMC57 films degraded slowest, displaying a mass loss of 26.46% in eight weeks. Obviously, the higher the molecular weight, the more significant degradation of PTMC films. The result was in coincidence with that of our previous work (Yang et al., 2015a). As for the ternary self-blending films, the mass loss was related to the mass ratio of high/medium/low molecular weight PTMC in the composition. The PTMC334/PTMC152/PTMC57 films with a mass ratio of 1/1/1 showed a mass loss of 96.39% after four weeks of degradation, while those with a mass ratio of 1/4/16 displayed much slower degradation with a mass loss of 85.96% after seven weeks of degradation (Figure 1).
The degradation rate constant k was calculated from the slope of the linear fitting curve of mass loss (Yang et al., 2015b). The k values of PTMC films and the ternary self-blending films were listed in Table 3, as the mass fraction of high molecular weight PTMC334 raised in the composition from 4.76 and 33.3 to 100%, the k value increased obviously from 13.263 and 23.981 to 26.085%/w, respectively, for the PTMC334/PTMC152/PTMC57 films with a mass ratio of 1/4/16, 1/1/1, and 1/0/0, while the k value significantly decreased from 23.981 and 13.263 to 3.664%/w (Table 3) as the proportion of low molecular weight PTMC57 in the ternary self-blending films increased from 33.3 and 76.19 to 100% for the PTMC334/PTMC152/PTMC57 films with a mass ratio of 1/1/1, 1/4/16, and 0/0/1. The degradation rate constant k of the ternary self-blending films enlarged obviously with the fraction increase of high molecular weight PTMC334 and reduced with that of low molecular weight PTMC57 in the composition, confirming again that the molecular weight has an important impact on the degradation rate of PTMC. The results showed that the degradation rate of PTMC could be controlled by ternary self-blending via the regulation of the composition. In our previous work (Hou et al., 2021), the binary self-blending films were prepared using high molecular weight PTMC334 and low molecular weight PTMC57, and the PTMC334/PTMC57 self-blending films with a mass ratio of 1/1 showed a k value of 19.4645%/w, which was similar to that of PTMC152 films (k = 20.052%/w) in this study. Interestingly, the k value of PTMC334/PTMC152/PTMC57 films with a mass ratio of 1/1/1 was increased to 23.981%/w after introducing PTMC152 into the PTMC334/PTMC57 binary self-blending films. Furthermore, the k value of PTMC334/PTMC57 self-blending films with a mass ratio of 1/16 was 5.219%/w (Hou et al., 2021), which significantly increased to 13.263%/w for PTMC334/PTMC152/PTMC57 films with a mass ratio of 1/4/16. It was attributed to the decrease in the content of low molecular weight PTMC57 in the composition caused by the introduction of PTMC152 into the PTMC334/PTMC57 self-blending films because the molecular weight plays a vital role in the degradation of PTMC; the lower the molecular weight, the much slower the degradation of PTMC (Yang et al., 2015a). The results indicated that the degradation rate of PTMC films could be tailored more precisely via ternary self-blending as compared with binary self-blending.
In addition to the controllable degradation rate, good morphology stability is also a desired property for PTMC to be applied as the carrier of biodegradable implants. The macroscopic morphology of PTMC films and the ternary self-blending films with degradation time was recorded to observe the morphology stability during degradation, displayed in Figure 2. As shown in Figure 2, the film of low molecular weight PTMC57 had poor morphology stability and changed the initial shape to spherical at week 1, while the PTMC152 film could retain its initial appearance in two weeks and became irregular after four weeks of degradation. High molecular weight PTMC334 film presented better morphology stability, and it can still maintain the film shape after three weeks of degradation, although there were visible holes on the surface. The higher the molecular weight, the much better the morphology stability. As for the ternary self-blending films, the PTMC334/PTMC152/PTMC57 films with a mass ratio of 1/4/16 showed better morphology stability than those with a mass ratio of 1/1/1, which remained the initial shape with no deformation last for four weeks and could keep the film-like form after six weeks of degradation. The morphology stability and degradation rate results indicated that ternary self-blending of high/medium/low molecular weight PTMC could make PTMC have a slower degradation rate and better morphology stability simultaneously during the degradation periods. More specifically, the degradation cycle of the PTMC334/PTMC152/PTMC57 films with a mass ratio of 1/4/16 was long enough, and it could retain good morphology stability during the degradation process, making it a promising candidate for long-term implants in vivo.
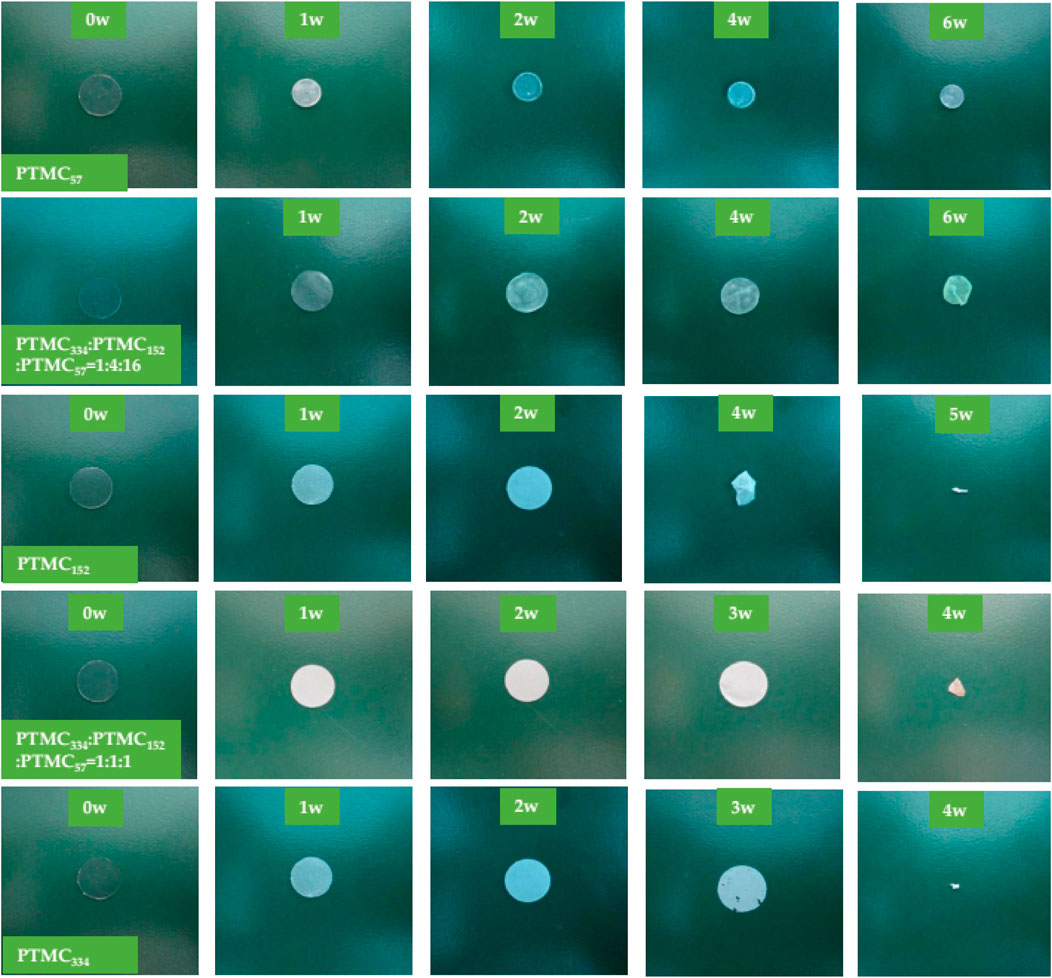
FIGURE 2. The macroscopic observation of the PTMC films PTMC films and ternary self-blending films at different times in 37°C lipase.
It has been reported that the enzymes can play a role as a surfactant to disperse the degradation products into the surrounding media (Yang et al., 2015a). The surface of PTMC samples would be changed accordingly with holes and pits during the degradation periods. Figure 3 shows the morphology changes of PTMC films and the ternary self-blending films before and after degradation in lipase solutions. As a representative, the surface morphology changes of PTMC334/PTMC152/PTMC57 films with a mass ratio of 1/4/16 were described in detail. The film surface was flat and smooth before the degradation, and it became rough with cracks as the degradation occurred after one week, and then small holes and pits emerged on the sample surface after three weeks of degradation, indicating the loss of degradation products. As the degradation prolonged to week 5, the erosion became prominent, and the sample surface became much rough, as noted in the number increase of holes. The results indicated that more degradation products were dispersed to the medium with the aid of lipase.
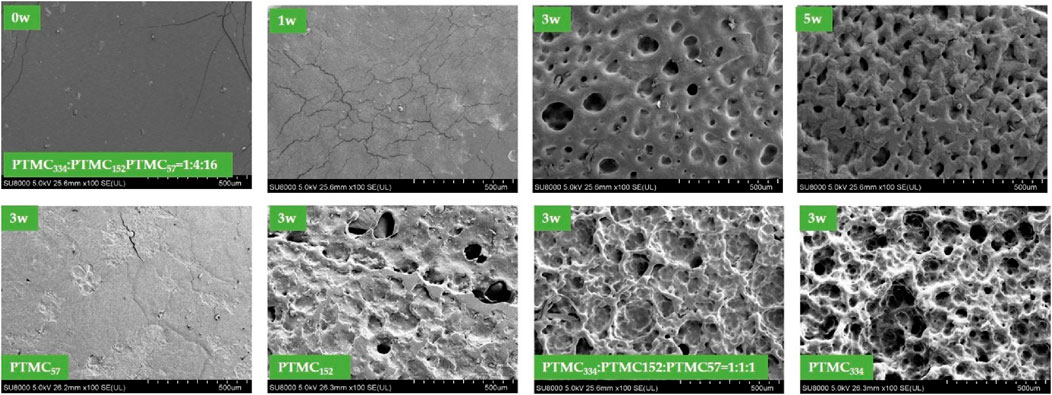
FIGURE 3. The surface morphology of PTMC films and the ternary self-blending films after degradation in lipase solutions.
Figure 3 also shows the surface morphology of the PTMC films and the ternary self-blending films with different compositions after three weeks of degradation. The surface morphology changed with the design of the resulting films. After three weeks of degradation, more prominent and bottomless pits were observed on the surface of PTMC334 and PTMC334/PTMC152/PTMC57 films with a mass ratio of 1/1/1, indicating that more severe degradation occurred. The size of pits on the surface of PTMC152 and PTMC334/PTMC152/PTMC57 films with a mass ratio of 1/4/16 became much smaller, resulting in minor roughness. The PTMC57 presented a relatively smooth surface with cracks rather than pits or holes. The SEM results were coincidental with that of mass loss.
It has been reported that PTMC does not produce acidic products during degradation (Yang et al., 2015a). For the ternary self-blending films, the degradation products also should be nonacidic due to their polycarbonate structure. In Figure 4, the pH of the lipase solution as a function of the degradation time was displayed. As expected, no acidification was observed when the degradation of ternary self-blending films occurred, powerfully demonstrating that the ternary self-blending films degraded without acidic products. It is beneficial to eliminate the inflammatory evoked by the acidic degradation products (Zhu et al., 1991; Karp et al., 2003; Sachlos et al., 2003). In addition, the accelerated degradation caused by the autocatalysis (Bergsma et al., 1995) will not occur during the degradation of the ternary self-blending films, promoting the more widespread application of the ternary self-blending films in the biomedical fields.
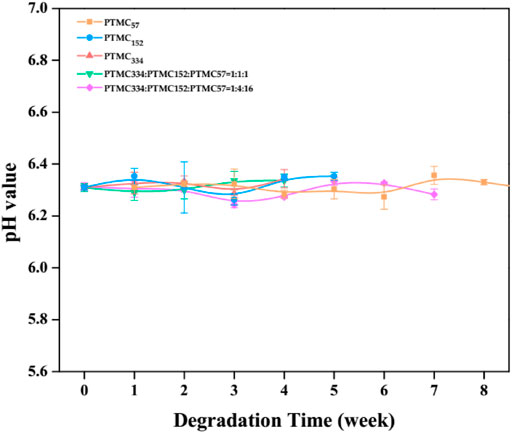
FIGURE 4. The pH of lipase solutions of PTMC films and the ternary self-blending films during enzymatic degradation.
Conclusion
In this study, PTMC with high/medium/low molecular weight of 334, 152, and 57 kg/mol, respectively, was prepared, and ternary self-blending of high/medium/low molecular weight PTMC was performed to obtain the self-blending PTMC films. The in vitro enzymatic degradation behavior of the ternary self-blending films was carried out in lipase solutions compared to that of the pure high/medium/low molecular weight PTMC films to investigate the effect of ternary self-blending on the degradation behaviors of PTMC. The results showed that the ternary self-blending films presented glass transition temperature lower than physiological temperature, making them more suitable for application in vivo. The results of mass loss and degradation rate constant indicated that ternary self-blending could lower the degradation rate of high molecular weight PTMC and enhance that of low molecular weight PTMC. The composition also influenced the degradation of the ternary self-blending films. The higher the content of the low molecular weight PTMC in the composition, the lower the degradation rate of the ternary self-blending films, as shown by the results of degradation rate constant and SEM. The PTMC334/PTMC152/PTMC57 film with a mass ratio of 1/4/16 showed a lower degradation rate constant of 13.263%/w, which also displayed better morphology stability to make the initial shape last for six weeks, with no acidic degradation products formed during degradation periods. The results indicated that ternary self-blending of high/medium/low molecular weight PTMC could more precisely control the in vitro enzymatic degradation of PTMC to meet the requirement as carriers for the biodegradable long-term contraceptive implants.
Data Availability Statement
The original contributions presented in the study are included in the article/Supplementary Material; further inquiries can be directed to the corresponding authors.
Author Contributions
GC and ZH performed the experiments, GC collected and analyzed the data and wrote the initial draft; LY and QY conceived and designed the experiments; WS and PL reviewed and edited the draft; JG performed the tests.
Funding
This work was supported by the National Key Research & Development Program of China (2016YFC1000902), the National Natural Science Foundation of China (No. 81872125), the Liaoning Revitalization Talents Program (XLYC1807142), the Department of Science & Technology of Liaoning Province (2018225079), the Educational Department of Liaoning Province (ZF2019040), the Shenyang Science and Technology Bureau (RC190426), and the Outstanding Scientific Fund of Shengjing Hospital (No. 201704).
Conflict of Interest
The authors declare that the research was conducted in the absence of any commercial or financial relationships that could be construed as a potential conflict of interest.
Publisher’s Note
All claims expressed in this article are solely those of the authors and do not necessarily represent those of their affiliated organizations or those of the publisher, the editors, and the reviewers. Any product that may be evaluated in this article or claim that may be made by its manufacturer is not guaranteed or endorsed by the publisher.
References
Bergsma, J., De Bruijn, W. C., Rozema, F. R., Bos, R. R., and Boering, G. (1995). Late Degradation Tissue Response to Poly(?-Lactide) Bone Plates and Screws. Biomaterials 16 (1), 25–31. doi:10.1016/0142-9612(95)91092-d
Bos, R. R. M., Rozema, F. B., Boering, G., Nijenhius, A. J., Pennings, A. J., Verwey, A. B., et al. (1991). Degradation of and Tissue Reaction to Biodegradable poly(L-Lactide) for Use as Internal Fixation of Fractures: A Study in Rats. Biomaterials 12 (1), 32–36. doi:10.1016/0142-9612(91)90128-w
Danhier, F., Ansorena, E., Silva, J. M., Coco, R., Le Breton, A., and Préat, V. (2012). PLGA-Based Nanoparticles: An Overview of Biomedical Applications. J. controlled release 161 (2), 505–522. doi:10.1016/j.jconrel.2012.01.043
Han, J., Branford-White, C. J., and Zhu, L.-M. (2010). Preparation of Poly(ε-Caprolactone)/poly(trimethylene Carbonate) Blend Nanofibers by Electrospinning. Carbohydr. Polym. 79 (1), 214–218. doi:10.1016/j.carbpol.2009.07.052
Hou, Z., Hu, J., Li, J., Zhang, W., Li, M., Guo, J., et al. (2017). The In Vitro Enzymatic Degradation of Cross-Linked Poly(trimethylene Carbonate) Networks. Polymers 9 (11), 605. doi:10.3390/polym9110605
Hou, Z., Zhang, W., Guo, J., Chen, Z., Hu, J., and Yang, L. (2019). The In Vitro Enzymatic Degradation of Poly(trimethylene Carbonate-Co-2, 2′-dimethyltrimethylene Carbonate). Eur. Polym. J. 112, 51–59. doi:10.1016/j.eurpolymj.2018.12.027
Hou, Z., Li, P., Guo, J., Wang, J., Hu, J., and Yang, L. (2020). The Effect of Molecular Weight on thermal Properties and Degradation Behavior of Copolymers Based on TMC and DTC. Polym. Degrad. Stab. 175, 109128. doi:10.1016/j.polymdegradstab.2020.109128
Hou, Z., Chen, S., Li, Z., Chen, Z., Hu, J., Guo, J., et al. (2021). Controllable Degradation of Poly (Trimethylene Carbonate) via Self-Blending with Different Molecular Weights. Polym. Degrad. Stab. 189, 109596. doi:10.1016/j.polymdegradstab.2021.109596
Joy, J., Aid-Launais, R., Pereira, J., Pavon-Djavid, G., Ray, A. R., Letourneur, D., et al. (2020). Gelatin-Polytrimethylene Carbonate Blend Based Electrospun Tubular Construct as a Potential Vascular Biomaterial. Mater. Sci. Eng. C 106, 110178. doi:10.1016/j.msec.2019.110178
Karp, J. M., Shoichet, M. S., and Davies, J. E. (2003). Bone Formation on Two-Dimensional poly(DL-Lactide-Co-Glycolide) (PLGA) Films and Three-Dimensional PLGA Tissue Engineering Scaffoldsin Vitro. J. Biomed. Mater. Res. 64A (2), 388–396. doi:10.1002/jbm.a.10420
Li, X., Chen, H., Xie, S., Wang, N., Wu, S., Duan, Y., et al. (2020). Fabrication of Photo-Crosslinkable Poly(Trimethylene Carbonate)/Polycaprolactone Nanofibrous Scaffolds for Tendon Regeneration. Int. J. Nanomedicine 15, 6373–6383. doi:10.2147/IJN.S246966
Mohajeri, S., Chen, F., de Prinse, M., Phung, T., Burke-Kleinman, J., Maurice, D. H., et al. (2020). Liquid Degradable Poly(trimethylene-Carbonate-Co-5-Hydroxy-Trimethylene Carbonate): An Injectable Drug Delivery Vehicle for Acid-Sensitive Drugs. Mol. Pharmaceutics 17 (4), 1363–1376. doi:10.1021/acs.molpharmaceut.0c00064
Raquez, J. M., Habibi, Y., Murariu, M., and Dubois, P. (2013). Polylactide (PLA)-Based Nanocomposites. Prog. Polym. Sci. 38 (10-11), 1504–1542. doi:10.1016/j.progpolymsci.2013.05.014
Sachlos, E., Czernuszka, J. T., and Czernuszka, J. (2003). Making Tissue Engineering Scaffolds Work. Review: The Application of Solid Freeform Fabrication Technology to the Production of Tissue Engineering Scaffolds. Eur. Cel Mater 5 (1), 29–40. doi:10.22203/ecm.v005a03
Woodruff, M. A., and Hutmacher, D. W. (2010). The Return of a Forgotten Polymer-Polycaprolactone in the 21st century. Prog. Polym. Sci. 35 (10), 1217–1256. doi:10.1016/j.progpolymsci.2010.04.002
Yang, L.-Q., Yang, D., Guan, Y.-M., Li, J.-X., and Li, M. (2012). Random Copolymers Based on Trimethylene Carbonate and ε-Caprolactone for Implant Applications: Synthesis and Properties. J. Appl. Polym. Sci. 124 (5), 3714–3720. doi:10.1002/app.35355
Yang, L.-Q., He, B., Meng, S., Zhang, J.-Z., Li, M., Guo, J., et al. (2013). Biodegradable Cross-Linked Poly(trimethylene Carbonate) Networks for Implant Applications: Synthesis and Properties. Polymer 54 (11), 2668–2675. doi:10.1016/j.polymer.2013.03.059
Yang, L., Li, J., Meng, S., Jin, Y., Zhang, J., Li, M., et al. (2014). The In Vitro and In Vivo Degradation Behavior of Poly (Trimethylene Carbonate-Co-ε-Caprolactone) Implants. Polymer 55 (20), 5111–5124. doi:10.1016/j.polymer.2014.08.027
Yang, L., Li, J., Jin, Y., Zhang, J., Li, M., and Gu, Z. (2014). Highly Efficient Cross-Linking of Poly(trimethylene Carbonate) via Bis(trimethylene Carbonate) or Bis(ε-Caprolactone). Polymer 55 (26), 6686–6695. doi:10.1016/j.polymer.2014.10.072
Yang, L., Li, J., Zhang, W., Jin, Y., Zhang, J., Liu, Y., et al. (2015). The Degradation of Poly(trimethylene Carbonate) Implants: The Role of Molecular Weight and Enzymes. Polym. Degrad. Stab. 122, 77–87. doi:10.1016/j.polymdegradstab.2015.10.016
Yang, L., Li, J., Jin, Y., Li, M., and Gu, Z. (2015). In Vitro enzymatic Degradation of the Cross-Linked Poly(ε-Caprolactone) Implants. Polym. Degrad. Stab. 112, 10–19. doi:10.1016/j.polymdegradstab.2014.12.008
Yang, L., Li, J., Li, M., and Gu, Z. (2016). The In Vitro and In Vivo Degradation of Cross-Linked Poly(trimethylene Carbonate)-Based Networks. Polymers 8 (4), 151. doi:10.3390/polym8040151
Zhang, Z., Kuijer, R., Bulstra, S. K., Grijpma, D. W., and Feijen, J. (2006). The In Vivo and In Vitro Degradation Behavior of Poly(trimethylene Carbonate). Biomaterials 27 (9), 1741–1748. doi:10.1016/j.biomaterials.2005.09.017
Keywords: poly(trimethylene carbonate), ternary self-blending, In vitro enzymatic degradation, lipase, degradation rate, form-stability
Citation: Cai G, Hou Z, Li P, Sun W, Guo J, Yang L and Yang Q (2021) More Precise Control of the In Vitro Enzymatic Degradation via Ternary Self-Blending of High/Medium/Low Molecular Weight Poly(trimethylene carbonate). Front. Mater. 8:733535. doi: 10.3389/fmats.2021.733535
Received: 30 June 2021; Accepted: 18 August 2021;
Published: 15 September 2021.
Edited by:
Yilong Cheng, Xi’an Jiaotong University, ChinaCopyright © 2021 Cai, Hou, Li, Sun, Guo, Yang and Yang. This is an open-access article distributed under the terms of the Creative Commons Attribution License (CC BY). The use, distribution or reproduction in other forums is permitted, provided the original author(s) and the copyright owner(s) are credited and that the original publication in this journal is cited, in accordance with accepted academic practice. No use, distribution or reproduction is permitted which does not comply with these terms.
*Correspondence: Liqun Yang, yanglq@lnszjk.com.cn; Qing Yang, yangqing_sj@126.com