- 1School of New Energy and Materials, Southwest Petroleum University, Chengdu, China
- 2Centre for Additive Manufacturing, Faculty of Engineering, University of Nottingham, Nottingham, United Kingdom
Polymer nanocomposites with excellent physical and chemical properties and multifunctional performance have been widely used in various fields. Biaxial stretching is not only an advanced film manufacturing process, but also a deformation mode in other processing methods such as blow film extrusion and thermoforming. In recent research, high-performance polymer nanocomposites have been fabricated via sequential and simultaneous biaxial stretching. This fabrication method enhances the mechanical properties, optical performance, and thermal properties of polymer nanocomposites by changing the structure or orientation of materials during the process of stretching. Therefore, it is particularly suitable for use in optimizing material performance and preparing thin films with excellent properties in the packaging industry. With the emergence of new materials and technologies, polymer nanocomposites prepared by biaxial stretching have demonstrated multifunctional properties and their range of applications has further expanded. In this mini-review, the effect of biaxial stretching on the structure and properties of nanocomposites based on various nanofillers is discussed and applications are summarized. In addition, the challenges and future prospects of this technology are analyzed. The presented work will be beneficial for improving preparation processes and improving future research for the production of high-performance polymer nanocomposites.
Introduction
Over the years, polymer composites reinforced with nanofillers have attracted significant attention. These materials are widely applied in medical equipment, electronics, and aerospace applications, and are used in wearable electronic devices, electromagnetic interference shielding, and for antistatic protection (Oladele et al., 2020; Guo et al., 2021). Nanofillers can provide polymers with new functionalities such as high electrical conductivity, dielectric properties, and a unique response to stimulation by light, thermal, pH, or magnetic fields. There may be slippage between surfaces of individual nanofillers in the absence of adhesive and polymers can be used as an adhesive to fix the nanofiller directional position and transfer the load between nanofillers efficiently (Christian et al., 2019). However, polymer nanocomposites produced by traditional synthesis methods such as solution processing, extrusion, or calendaring are always randomly oriented, which limits their properties. Improving the dispersion of nanofillers in polymer matrices and enhancing interface adhesion between the nanofiller and matrix are crucial strategies for the preparation of high-performance polymer nanocomposites (Lavagna et al., 2020; Min et al., 2020).
It is known that the improvement of polymeric mechanical properties via chain orientation is a simple and efficient strategy. Methods for achieving this include biaxial stretching, blow molding, extrusion, and melt spinning. Compared to conventional methods, the biaxial stretching process results in a high degree of orientation and regular alignment of chains by intermolecular friction (Li et al., 2020). The structure, orientation degree, and surface morphology of polymers can be changed by adjusting the stretching ratio (SR) of biaxial stretching to enhance strength and tensile modulus in the oriented direction (Soon et al., 2012; Mayoral et al., 2021). Molecular alignment of amorphous polymer chains in the oriented direction and strain-induced crystallization are also influenced by biaxial tension (Delpouve et al., 2012; Ouchiar et al., 2016; Van Berkel et al., 2018). Generally, the degree of molecular orientation, amount of strain-induced crystallization, and extent of amorphous chain confinement all affect the barrier performance and thermomechanical properties of polymer nanocomposites (Delpouve et al., 2012; Mayoral et al., 2021). Another benefit of biaxial stretching is the ability to disperse nanofillers in the polymer matrix without using compatibilizers (Xiang et al., 2015). Therefore, biaxial stretching is particularly suitable for use in optimizing polymer material performance and preparing thin films with excellent properties in the packaging industry.
Biaxial tension is not only an advanced composite manufacturing process, but also a deformation mode in other processing methods, such as blow film extrusion (Espinosa et al., 2016) and thermoforming (Mayoral et al., 2021). Moreover, the use of biaxial stretching in changing the structure, dispersion, and orientation of neat polymer materials to enhance performance has been widely studied for polymers including biaxially oriented polypropylene (Xing et al., 2019), biaxially oriented polyethylene (Chen et al., 2020), and biaxially oriented polyethylene terephthalate (Onyishi and Oluah, 2020). However, there is significantly less research on the performance and applications of biaxially oriented polymer nanocomposites.
This mini-review summarizes the effect of biaxial stretching on the structure and properties of polymer nanocomposites. The application of biaxially stretched polymer nanocomposites in the packaging industry is outlined and reported data of polymer nanocomposites in field applications are described and discussed. Moreover, the advances and challenges of producing polymer nanocomposites via biaxial stretching method are outlined.
Biaxial Stretching of Polymer Nanocomposites
With unique optical, electrical, thermal, and mechanical properties, nanomaterials have been reported as reinforcing materials for the fabrication of multifunctional nanocomposites combined with the process of biaxial stretching.
Polymer/Clay Nanocomposites
Polymer/clay nanocomposites, also known as polymer/layered silicate nanocomposites, have excellent electrical conductivity, magnetic properties, and thermal insulation properties. Moreover, the addition of clay can reduce gas permeability and flammability as well as improve mechanical properties (Singha and Hedenqvist, 2020; Kovačević et al., 2021). The Toyota Company produced a nylon 6/clay nanocomposite, finding a significant enhancement in modulus and strength with the addition of clay (Kojima et al., 1993). The most commonly used clays include saponite, montmorillonite (Liu et al., 2018), and hectorite. Figure 1A shows a schematic of biaxial stretching modes including simultaneous and sequential biaxial stretching. Taking clay/polymer nanocomposites as an example, Figures 1B–F illustrate the effect of biaxial stretching on the morphology and properties of clay/polymer nanocomposites.
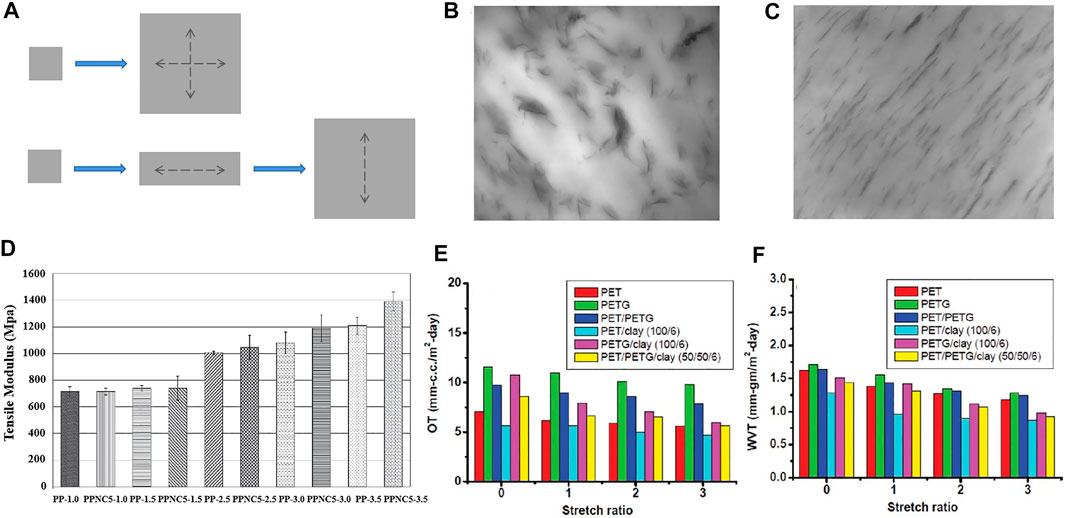
FIGURE 1. (A) Schematic of biaxial stretching modes (SR = 3). TEM images: (B) unstretched sheet, (C) stretched sheet (SR = 3), magnification = 28,500, scale bar = 500 nm. (D) Tensile modulus of PP/clay nanocomposites (Abu-Zurayk et al., 2009). Effect of SR on (E) oxygen transmission rate and (F) water vapor transmission rate of biaxially stretched PET/clay, PETG/clay, and PET/PETG/clay nanocomposites (Wu et al., 2015).
The properties of polymer/clay nanocomposites mainly depend on the degree of exfoliation, content, and orientation of the clay (Chowreddy et al., 2019; Maria et al., 2011). From Figures 1B,C, it can be seen that for the biaxial stretching process of clay-filled nanocomposites, the initial biaxial tension causes the delamination of clay tactoids. Subsequent stretching reduces stack thickness, indicating that a higher degree of exfoliation occurred as SR increasing, and uniformly dispersed clay nanoparticles have an oriented arrangement in the direction of stretching. A 44% improvement in yield stress for 5 wt% clay-filled PP compared with pure PP at the same SR has been demonstrated (Abu-Zurayk et al., 2009). Furthermore, orientation distribution also becomes narrower with increasing SR (Abu-Zurayk et al., 2010). The presence of clay agglomeration can decrease the tensile properties of nanocomposites, which are more affected by the orientation of clay tactoids than the dispersion degree (Wang et al., 2007). Figure 1D shows that the addition of clay and biaxial tension does not enhance the tensile modulus of the composites until SR = 2.5 due to the presence of large tactoids under low strain tension, which has no effect on the tensile properties of the PP/clay nanocomposites. With an increase in SR above 2.5, the clay nanoparticle degree of orientation and degree of exfoliation increase, improving the tensile properties of the composites.
Soon et al. (2012) studied the morphology, mechanical properties, and barrier properties of biaxially stretched polyethylene terephthalate (PET)/mica nanocomposites, finding that the tensile process promoted the orientation and exfoliation of mica. The mechanical properties of the nanocomposites increased with an increase in SR. Furthermore, barrier properties improved with the addition of mica and the effect of drawing. Wu et al. (2015) also compared the tensile properties, optical performances, gas barrier properties, water vapor barrier properties, and cold crystallization behavior of biaxially stretched PET/clay, Poly (ethylene glycol-co-1,3/1,4-cyclohexanedimethanol terephthalate) (PETG)/clay, and PET/PETG/clay nanocomposite films. A significant enhancement in tensile, optical, and gas barrier properties was achieved due to the improved dispersion and orientation degree of clay platelets during the biaxial stretching process. Biaxial stretching improved the oxygen transmission rate performance of the PET/clay, PETG/clay, and PET/PETG/clay nanocomposite films by approximately 18, 45, and 34%, respectively, shown in Figure 1E. The water vapor transmission rate performance of PET/clay, PETG/clay, and PET/PETG/clay was improved by 32, 35, and 35%, respectively, shown in Figure 1F. Moreover, the biaxial tension process triggered a strain-induced crystallization in the amorphous PETG matrix and PET/PETG composite.
Polymer/Carbon Nanocomposites
With extraordinary electrical, mechanical, and thermal properties, carbon nanotubes (CNTs) have been widely studied since their first discovery (Iijima, 1991). CNTs are an ideal functional and reinforcing material, with ultra-strong mechanical properties that significantly improve the strength and toughness of polymer nanocomposites. The unique photoelectric properties of CNTs can also endow polymer nanocomposites with new photoelectric functionalities. The development of carbon nanotube networks in CNT/PP nanocomposites demonstrated a unique process of destruction and rebuilding of a conductive network during simultaneous biaxial stretching (Shen et al., 2012a; Shen et al., 2012b). Xiang et al. (2018) prepared multiwalled carbon nanotube/high-density polyethylene (MWCNT/HDPE) nanocomposites, showing that the processability and deformation stability of HDPE was significantly enhanced with the addition of carbon nanofillers (4 wt%) upon biaxial stretching (Table 1). At an SR of 3, the resistivity of a biaxially stretched MWCNT/HDPE nanocomposite increased by ∼108 compared with an unstretched sample, indicating that biaxial deformation resulted in the damage of conductive pathways in the composite material while interlacing or entanglement of the 1D MWCNTs was beneficial for rebuilding conductive network.
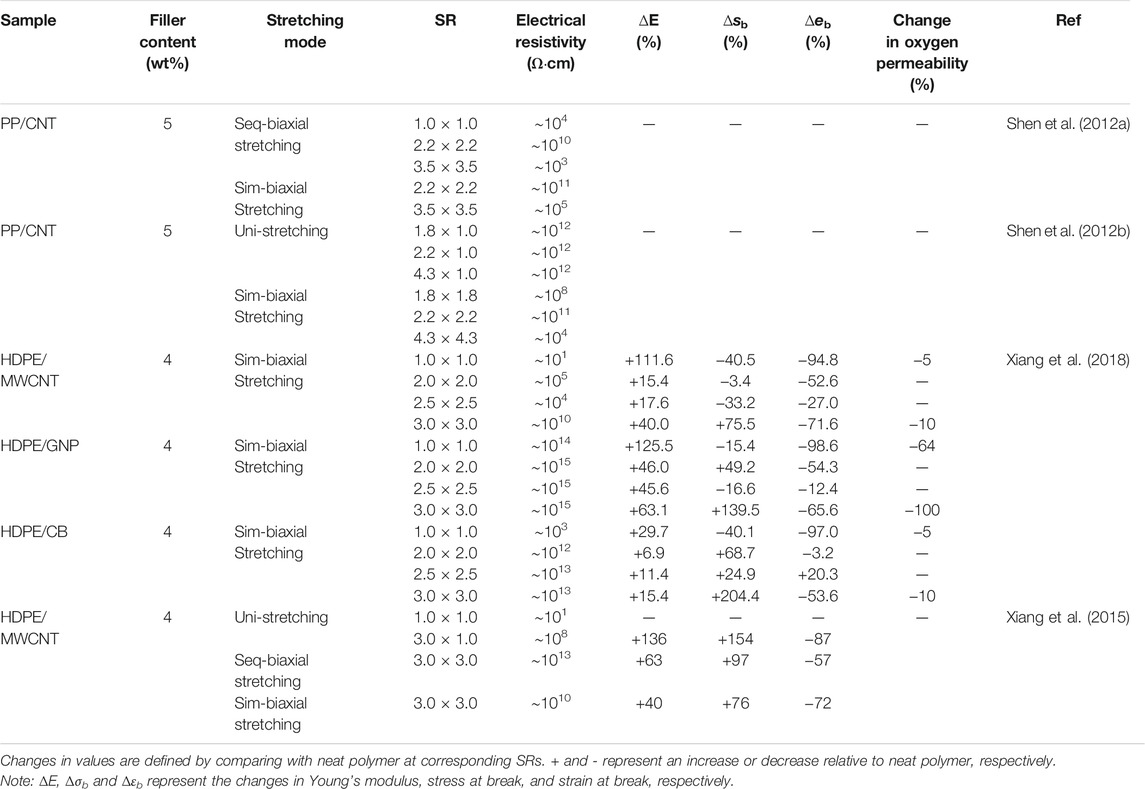
TABLE 1. Effect of carbon nanofillers on the mechanical, electrical, and barrier properties of uniaxially, seq-biaxially, and sim-biaxially stretched polymeric nanocomposites.
Furthermore, uniaxially, sequential (seq-) biaxially, and simultaneous (sim-) biaxially stretched neat HDPE and MWCNT/HDPE nanocomposites were conducted to study the processability of HDPE with the addition of 4 wt% MWCNTs and the effect of tension on the final structure and properties of nanocomposites, as shown in Table 1. It showed that sequential biaxial stretching improved the tensile properties of nanocomposites effectively, due to more agglomerate disentanglement during stretching. A similar phenomenon had been reported for PP/CNT composite by Shen et al. (2012a). While the tensile properties of simultaneous biaxially stretched nanocomposites can be improved via reducing the strain rate, due to more disentanglement of the MWCNTs at a lower strain rate, therefore the resistivity of simultaneous biaxially stretched composites stretched at a lower strain rate increased by 1011 Ω cm (Xiang et al., 2015).
Graphene nanoplatelets (GNPs) are another exceptional two-dimensional carbon nanofiller. GNPs consist of single-atom thickness sp2 hybrid carbon sheets and have excellent mechanical and electrical properties (Pei et al., 2021; Wang and Shi, 2015; Yang B. et al., 2020). A biaxially stretched GNP/HDPE nanocomposite exhibited a 63% enhancement to Young’s modulus, with the oxygen permeability coefficient reduced to only 4.1 × 10−16 cm3 cm/cm2 s Pa at SR = 3, as shown in Table 1. Liu et al. (2020) prepared a polydimethylsiloxane (PDMS)/GNP composite film near the threshold concentration, finding that biaxial stretching effectively enhanced the sensitivity of the PDMS/GNP composite film by an order of magnitude compared with traditional uniaxial stretching. Traditionally prepared GNP/polymer nanocomposites often have poor dispersion, while the shear force during biaxial stretching is beneficial to improve the dispersion of nanofillers in polymer matrices (Shen et al., 2011).
As an amorphous carbon nanoparticle, carbon black (CB) has the characteristics of disordered graphite-like structure, small particle size, good conductivity, and strong ultraviolet absorption. CB/polymer nanocomposites with good mechanical, thermal, electrical, and chemical properties (Kausar, 2017; Azizi et al., 2019), are widely used in solar cells (Yue et al., 2016), super-capacitors (Xing et al., 2015), sensors (Yang H. et al., 2020), and electromagnetic shielding (Zhang et al., 2021). The morphology and dispersion state of CB particles are important factors affecting the properties of polymer composites. During biaxial tension, clustered CB can disperse more evenly in a polymer matrix. Compared with biaxially stretched pure HDPE, the stress at break (σb) of a biaxially stretched CB/HDPE nanocomposite at the same SR increased by 204% due to the intensive breakup of CB clusters (Table 1).
In conclusion, material processability, electrical properties, and barrier properties are affected by different carbon nanofillers, and biaxial tension affects the structure and properties of polymer/carbon nanocomposites. Moreover, there is also some research investigating the synergetic effect of hybrid carbon nanofillers to further improve the mechanical, tensile, barrier, and electrical properties of polymer nanocomposites with lower cost (Xiang et al., 2017; Xiang et al., 2020).
Polymer/Oxide Nanocomposites
The introduction of oxide nanoparticles to a polymer matrix for the enhancement of physical and mechanical properties has been widely studied. Uniformly dispersed nanoparticles can improve Young’s modulus, tensile strength, conductivity, and thermal capacity as well as significantly decrease polymer combustibility (Benabid et al., 2019; Deepalekshmi et al., 2019). However, these nanofillers may also result in increasing rigidity, leading to fractures or cracks and causing the degradation of the composite material’s properties (Tang et al., 2020; Goncharova Daria et al., 2021).
A study investigating the effect of biaxial orientation on the mechanical performances of PP/ZnO nanocomposites found that the addition of ZnO nanoparticles increased the polymer’s yield point, but biaxial orientation at a higher degree of deformation allowed the plasticity of the polymer nanocomposites to be maintained if a high content of ZnO nanoparticles were incorporated into the polymer matrix (Kechek’yan et al., 2018). Lepot et al. (2011) investigated the effect of introducing 0, 2, 5, and 7.5 wt% ZnO nanoparticles on the processing and properties of biaxially oriented polypropylene (BOPP)-ZnO nanocomposites, finding that the addition of ZnO nanoparticles improved mechanical and oxygen barrier properties of BOPP-ZnO nanocomposites. Figures 2A,B showed that the BOPP-ZnO films with 5 wt% ZnO had a good dispersion.
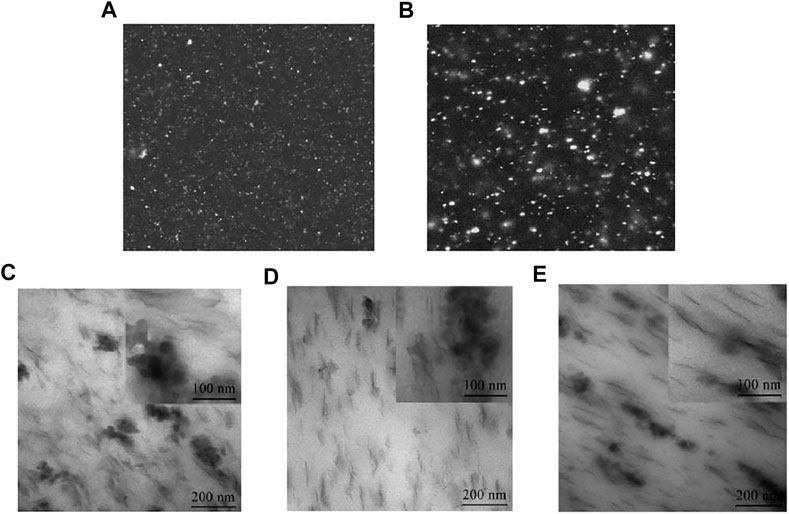
FIGURE 2. SEM images (A) Surface of ZnO-BOPP nanocomposite with spherical ZnO. (B) Cross-section of ZnO-BOPP nanocomposite (Lepot et al., 2011). TEM images of the (C) unstretched (1.0 × 1.0), (D) uniaxially stretched (3.0 × 1.0), and (E) biaxially stretched (3.0 × 2.0) PA6-66-MMT-NS films (Liu et al., 2019).
Uniaxially and biaxially stretched PA6-66-MMT-nanosilica composite films were prepared through a cast film process, finding that tension led to an oriented conformation of the MMT and nanosilica, as shown in Figures 2C,E.
From Table 2, for the uniaxially stretched films, the tensile strength in the stretching direction gradually increased with increasing SR, and the biaxially stretched nanocomposite films showed more balanced mechanical properties and thermal shrinkage along the machine direction and transverse direction compared with the uniaxially stretched composite (Liu et al., 2019).
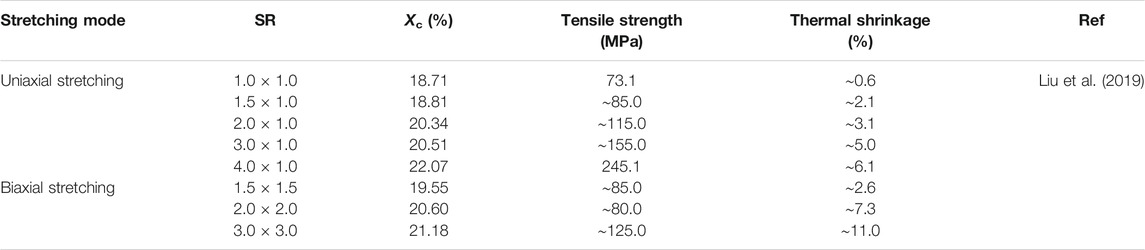
TABLE 2. The relative crystallinity (Xc), tensile strength, and thermal shrinkage of uniaxially and biaxially stretched PA6-66-MMT-nanosilica composites.
In conclusion, reinforced polymer/oxide materials combined with biaxial stretching can effectively enhance nanocomposite properties.
Applications
The application classification of biaxially stretched nanocomposites is shown in Figure 3. The development of new polymer films for packaging and agricultural applications has recently attracted significant attention (Liu et al., 2014; Lai et al., 2020; Zhou et al., 2020). Studies have shown that the barrier properties and mechanical properties of polymer nanocomposites are enhanced after being biaxially stretched into films. Due to this, biaxially stretched polymer nanocomposites are widely used in the packaging industry (Shen et al., 2011; Youm et al., 2020; Li et al., 2021). Biodegradable polymers such as poly (butylene adipate-co-terephthalate) (PBAT) have the advantage of low environmental impact, but poor oxygen permeation and water vapor barrier properties limit their practical application (Falcão et al., 2017; Roy and Rhim, 2020). Well-dispersed and oriented nanofillers, such as GNPs and organically modified montmorillonite (OMMT), can efficiently improve the barrier properties of nanocomposites via biaxial stretching, revealing a promising solution (Xiang et al., 2017; Li et al., 2018). By way of example, Li et al. (2018) prepared a series of 0–13 wt% PBAT/OMMT nanocomposite films via film blowing and biaxial stretching, finding that the addition of 13 wt% OMMT significantly reduced water vapor permeation values and two technologies enhanced in-plane polymer chain alignment, which aligns OMMT particles. Furthermore, OMMT layers were better oriented parallel to film surfaces with biaxial orientation, indicating that biaxial stretching had the ability to improve the water vapor barrier properties more effectively. Also, research into PBAT/GNP nanocomposite films prepared by biaxially stretched orientation and rod scraping demonstrate that these films possess good barrier properties, UV-blocking properties, and aging performance (Li et al., 2021). These studies demonstrate the potential for the use of biaxially oriented and biodegradable nanocomposites in sustainably developed packaging and the agricultural industry. Moreover, biaxially stretched nanocomposites reinforced by nanometer-scale materials have the advantage of high transparency compared with the use of other fillers. Compared with traditional packaging materials, food and beverage packaging products produced with PET-mica nanocomposites are lighter. In addition, mica loadings (1, 2, and 5 wt%) have been shown to improve the mechanical properties of PET at high temperatures, which can be applied for hot filling of containers (Soon et al., 2012).
Some research into the application of biaxially stretched polymer nanocomposites in sensors and electromagnetic interference (EMI) shielding has also been published. For instance, a sensor prepared near the threshold concentration (0.98 wt%) based on GNP/PDMS composite was reported for monitoring human motion. Biaxial stretching was shown to efficiently improve the sensitivity (gauge factor (GF) = 687.43) of the GNP/PDMS composite compared with uniaxially stretched sample (GF = 68.81). Furthermore, the biaxially stretched sensor showed excellent signal responses to various bending degrees of knee joint (Liu et al., 2020). The application of nanocomposite films assisted by biaxial stretching in EMI shielding has been studied recently. Wang et al. (2021) prepared nanocellular MWCNT/poly (ether-block-amide) (PEBA) nanocomposite films for high-performance EMI shielding applications with the assistance of biaxial stretching, finding that MWCNTs and biaxial stretching caused a greatly refined cellular structure. The cellular structure became more obviously refined with the increasing SR, leading to enhanced EMI shielding performance (shielding effectiveness up to 41 dB) and electrical conductivity. In addition, the conductive and flexible MWCNT/PEBA nanocomposite film with excellent absorption-dominated EMI shielding performance has potential in other applications such as lightning strike protection in aerospace due to the similar requirement on electrical conductivity.
Discussion
According to literature, the orientation and structural changes of polymeric nanocomposites caused by the biaxial stretching process are clearly beneficial for enhancing material properties. Biaxial stretching can also be used to enhance the performance of more materials and their composites. Although sequential biaxial stretching and simultaneous biaxial stretching have been used to fabricate various polymer nanocomposites, there is still potential for optimization. To design multifunctional polymer nanocomposites, biaxial stretching and structure design can be combined.
Overall, the field of biaxially oriented polymer matrix nanocomposites is still in its infancy. The future development direction of biaxially oriented polymer nanocomposites should focus on optimizing the preparation process, reducing production costs, and improving the compatibility between nanofillers and polymer matrices. Furthermore, the potential application of biaxially oriented polymer nanocomposites in many fields has not been widely studied. Polymer nanocomposites prepared by biaxial stretching have significant potential practical value and broad development prospects.
Conclusion
In this mini-review, the effect of biaxial stretching on the properties of polymer composites with different nanofillers has been summarized. A suitable stretching ratio and careful choice of nanofiller can provide polymer nanocomposite materials with a unique structure and surface morphology as well as a high orientation degree to enhance mechanical, electrical, and barrier properties. As multifunctional high-performance materials, biaxially stretched polymer nanocomposites have a significant potential for application in the fields of packaging, strain sensors, and capacitors.
Author Contributions
XC as the main writer of the mini-review, completed the collection and analysis of relevant literature and the writing of the first draft of the paper; YW, HL, CZ, and YL participate in the analysis and collation of literature; DX and ZZ are the designers and directors of the mini-review, guiding the mini-review writing. All authors read and agree to the final mini-review.
Funding
This work was also supported within the framework of the National Key Research and Development Program (2019YFE0120300), the Sichuan Science and Technology Program (2021YFH0031), the International Cooperation Project of Chengdu (2019-GH02-00054-HZ) and the Scientific Research Starting Project of SWPU (2019QHZ011).
Conflict of Interest
The authors declare that the research was conducted in the absence of any commercial or financial relationships that could be construed as a potential conflict of interest.
Publisher’s Note
All claims expressed in this article are solely those of the authors and do not necessarily represent those of their affiliated organizations, or those of the publisher, the editors and the reviewers. Any product that may be evaluated in this article, or claim that may be made by its manufacturer, is not guaranteed or endorsed by the publisher.
Acknowledgments
The authors thank University of Nottingham for the support of this work.
References
Abu-Zurayk, R., Harkin-Jones, E., Mcnally, T., Menary, G., Martin, P., and Armstrong, C. (2009). Biaxial Deformation Behavior and Mechanical Properties of a Polypropylene/clay Nanocomposite. Composites Sci. Tech. 69, 1644–1652. doi:10.1016/j.compscitech.2009.03.014
Abu-Zurayk, R., Harkin-Jones, E., Mcnally, T., Menary, G., Martin, P., Armstrong, C., et al. (2010). Structure-property Relationships in Biaxially Deformed Polypropylene Nanocomposites. Composites Sci. Tech. 70, 1353–1359. doi:10.1016/j.compscitech.2010.04.011
Azizi, S., David, E., Fréchette, M. F., Nguyen-Tri, P., and Ouellet-Plamondon, C. M. (2019). Electrical and thermal Phenomena in Low-Density Polyethylene/carbon Black Composites Near the Percolation Threshold. J. Appl. Polym. Sci. 136, 47043. doi:10.1002/app.47043
Benabid, F., Kharchi, N., Zouai, F., Mourad, A.-H. I., and Benachour, D. (2019). Impact of Co-mixing Technique and Surface Modification of ZnO Nanoparticles Using Stearic Acid on Their Dispersion into HDPE to Produce HDPE/ZnO Nanocomposites. Polym. Polym. Composites 27, 389–399. doi:10.1177/0967391119847353
Chen, Q., Wang, Z., Zhang, S., Cao, Y., and Chen, J. (2020). Structure Evolution and Deformation Behavior of Polyethylene Film during Biaxial Stretching. ACS omega 5, 655–666. doi:10.1021/acsomega.9b03250
Chowreddy, R. R., Nord-Varhaug, K., and Rapp, F. (2019). Recycled Poly(Ethylene Terephthalate)/Clay Nanocomposites: Rheology, Thermal and Mechanical Properties. J.Polym. Environ. 27, 37–49. doi:10.1007/s10924-018-1320-6
Delpouve, N., Stoclet, G., Saiter, A., Dargent, E., and Marais, S. (2012). Water Barrier Properties in Biaxially Drawn Poly(lactic Acid) Films. J. Phys. Chem. B. 116, 4615–4625. doi:10.1021/jp211670g
Espinosa, K. R., Castillo, L. A., and Barbosa, S. E. (2016). Blown Nanocomposite Films from Polypropylene and Talc. Influence of Talc Nanoparticles on Biaxial Properties. Mater. Des. 111, 25–35. doi:10.1016/j.matdes.2016.08.045
Falcão, G. A. M., Vitorino, M. B. C., Almeida, T. G., Bardi, M. A. G., Carvalho, L. H., and Canedo, E. L. (2017). PBAT/organoclay Composite Films: Preparation and Properties. Polym. Bull. 74, 4423–4436. doi:10.1007/s00289-017-1966-6
Goncharova, D. A., Bolbasov, E. N., Nemoykina, A. L., Aljulaih, A. A., Tverdokhlebova, T. S., Kulinich, S. A., et al. (2021). Structure and Properties of Biodegradable PLLA/ZnO Composite Membrane Produced via Electrospinning. Materials 14, 2–13. doi:10.3390/MA14010002
Guo, Z., Poot, A. A., and Grijpma, D. W. (2021). Advanced Polymer-Based Composites and Structures for Biomedical Applications. Eur. Polym. J. 149, 110388. doi:10.1016/J.EURPOLYMJ.2021.110388
Harito, C., Bavykin, D. V., Yuliarto, B., Dipojono, H. K., and Walsh, F. C. (2019). Polymer Nanocomposites Having a High Filler Content: Synthesis, Structures, Properties, and Applications. Nanoscale 11, 4653–4682. doi:10.1039/C9NR00117D
Iijima, S. (1991). Helical Microtubules of Graphitic Carbon. Nature 354, 56–58. doi:10.1038/354056a0
Kausar, A. (2017). Contemporary Applications of Carbon Black-Filled Polymer Composites: An Overview of Essential Aspects. J. Plast. Film Sheeting 34, 256–299. doi:10.1177/8756087917725773
Kechek’yan, P. A., Bazhenov, S. L., and Kechek’yan, A. S. (2018). The Influence of Biaxial Orientation on the Mechanical Properties of Polyethylene Filled with ZnO Nanoparticles. Polym. Sci. Ser. A. 60, 373–380. doi:10.1134/S0965545X18030057
Kojima, Y., Usuki, A., and Kawasumi, M. (1993). Synthesis and Mechanical Properties of Nylon6-Clay Hybrid. J. Mater. Life Soc. 11, 1179–1185. doi:10.1557/JMR.1993.1185
Kovačević, Z., Flinčec Grgac, S., and Bischof, S. (2021). Progress in Biodegradable Flame Retardant Nano-Biocomposites. Polymers 13, 741. doi:10.3390/POLYM13050741
Lai, L., Li, J., Liu, P., Wu, L., Severtson, S. J., and Wang, W.-J. (2020). Mechanically Reinforced Biodegradable Poly(butylene Adipate-Co-Terephthalate) with Interactive Nanoinclusions. Polymer 197, 122518. doi:10.1016/j.polymer.2020.122518
Lavagna, L., Marchisio, S., Merlo, A., Nisticò, R., and Pavese, M. (2020). Polyvinyl Butyral‐based Composites with Carbon Nanotubes: Efficient Dispersion as a Key to High Mechanical Properties. Polym. Composites 41, 3627–3637. doi:10.1002/pc.25661
Lee, M. G., Lee, S., Cho, J., Bae, S., and Jho, J. Y. (2020). Effect of the Fluorination of Graphene Nanoflake on the Dispersion and Mechanical Properties of Polypropylene Nanocomposites. Nanomaterials 10, 1171. doi:10.3390/nano10061171
Lepot, N., Van Bael, M. K., Van den Rul, H., D'Haen, J., Peeters, R., Franco, D., et al. (2011). Influence of Incorporation of ZnO Nanoparticles and Biaxial Orientation on Mechanical and Oxygen Barrier Properties of Polypropylene Films for Food Packaging Applications. J. Appl. Polym. Sci. 120, 1616–1623. doi:10.1002/app.33277
Li, J., Chen, Q., Zhang, Q., Fan, T., Gong, L., Ye, W., et al. (2020). Improving Mechanical Properties and Biocompatibilities by Highly Oriented Long Chain Branching Poly(lactic Acid) with Bionic Surface Structures. ACS Appl. Mater. Inter. 12, 14365–14375. doi:10.1021/acsami.9b20264
Li, J., Lai, L., Wu, L., Severtson, S. J., and Wang, W.-J. (2018). Enhancement of Water Vapor Barrier Properties of Biodegradable Poly(butylene Adipate-Co-Terephthalate) Films with Highly Oriented Organomontmorillonite. ACS Sust. Chem. Eng. 6, 6654–6662. doi:10.1021/acssuschemeng.8b00430
Li, J., Wang, S., Lai, L., Liu, P., Wu, H., Xu, J., et al. (2021). Synergistic Enhancement of Gas Barrier and Aging Resistance for Biodegradable Films with Aligned Graphene Nanosheets. Carbon 172, 31–40. doi:10.1016/J.CARBON.2020.09.071
Liu, A., Ni, Z., Chen, J., and Huang, Y. (2020). Highly Sensitive Graphene/Polydimethylsiloxane Composite Films Near the Threshold Concentration with Biaxial Stretching. Polymers 12, 71. doi:10.3390/polym12010071
Liu, E. K., He, W. Q., and Yan, C. R. (2014). ‘'White Revolution' to 'white Pollution'-Agricultural Plastic Film Mulch in China. Environ. Res. Lett. 9, 091001. doi:10.1088/1748-9326/9/9/091001
Liu, X., Liu, Y., Yang, J., Wang, W., Lai, D., Mao, L., et al. (2019). Effects of the Biaxial Orientation on the Mechanical and Optical Properties and Shrinkage of Polyamide 6-66-Montmorillonite-Nanosilica Nanocomposite Films. J. Appl. Polym. Sci. 136, 47504. doi:10.1002/app.47504
Liu, X., Liu, Y., Yang, J., Yi, Z., Mao, L., Zheng, W., et al. (2018). A Comparative Study of the Structure and Mechanical Properties of Uniaxially and Biaxially Stretched PA6-66/Montmorillonite Films. Nanosci Nanotechnol Lett. 10, 167–176. doi:10.1166/nnl.2018.2618
Mayoral, B., Menary, G., Martin, P., Garrett, G., Millar, B., Douglas, P., et al. (2021). Characterizing Biaxially Stretched Polypropylene/Graphene Nanoplatelet Composites. Front. Mater. 8. doi:10.3389/fmats.2021.687282
Oladele, I. O., Omotosho, T. F., Adediran, A. A., and Morales-Cepeda, A. (2020). Polymer-Based Composites: An Indispensable Material for Present and Future Applications. Int. J. Polym. Sci. 2020, 1–12. doi:10.1155/2020/8834518
Onyishi, H. O., and Oluah, C. K. (2020). Effect of Stretch Ratio on the Induced Crystallinity and Mechanical Properties of Biaxially Stretched PET. Phase Transitions 93, 924–934. doi:10.1080/01411594.2020.1813291
Ouchiar, S., Stoclet, G., Cabaret, C., Addad, A., and Gloaguen, V. (2016). Effect of Biaxial Stretching on Thermomechanical Properties of Polylactide Based Nanocomposites. Polymer 99, 358–367. doi:10.1016/j.polymer.2016.07.020
Pei, H., Yang, L., Xiong, Y., Chen, Y., Shi, S., and Jing, J. (2021). Fabrication, Characterisation and Properties of Polyvinyl Alcohol/graphene Nanocomposite for Fused Filament Fabrication Processing. Plastics, Rubber and Composites 50, 263–275. doi:10.1080/14658011.2020.1868668
Ponnamma, D., Cabibihan, J.-J., Rajan, M., Pethaiah, S. S., Deshmukh, K., Gogoi, J. P., et al. (2019). Synthesis, Optimization and Applications of ZnO/polymer Nanocomposites. Mater. Sci. Eng. C. 98, 1210–1240. doi:10.1016/j.msec.2019.01.081
Romero-Guzmán, M. E., Flores, O., Flores, A., Romo-Uribe, A., Campillo, B. B., Araceli, B., et al. (2011). Cold-drawn Induced Microstructure in PVC-Bentonite Nanocomposites. Polym. Adv. Technol. 22, 836–846. doi:10.1002/pat.1586
Roy, S., and Rhim, J.-W. (2020). Curcumin Incorporated Poly(Butylene Adipate-Co-Terephthalate) Film with Improved Water Vapor Barrier and Antioxidant Properties. Materials 13, 4369. doi:10.3390/ma13194369
Shen, J., Champagne, M. F., Gendron, R., and Guo, S. (2012b). The Development of Conductive Carbon Nanotube Network in Polypropylene-Based Composites during Simultaneous Biaxial Stretching. Eur. Polym. J. 48, 930–939. doi:10.1016/j.eurpolymj.2012.03.005
Shen, J., Champagne, M. F., Yang, Z., Yu, Q., Gendron, R., and Guo, S. (2012a). The Development of a Conductive Carbon Nanotube (CNT) Network in CNT/polypropylene Composite Films during Biaxial Stretching. Composites A: Appl. Sci. Manufacturing 43, 1448–1453. doi:10.1016/j.compositesa.2012.04.003
Shen, Y., Harkin-Jones, E., Hornsby, P., Mcnally, T., and Abu-Zurayk, R. (2011). The Effect of Temperature and Strain Rate on the Deformation Behaviour, Structure Development and Properties of Biaxially Stretched PET-clay Nanocomposites. Composites Sci. Tech. 71, 758–764. doi:10.1016/j.compscitech.2011.01.024
Singha, S., and Hedenqvist, M. S. (2020). A Review on Barrier Properties of Poly(Lactic Acid)/Clay Nanocomposites. Polymers 12, 1095. doi:10.3390/polym12051095
Soon, K., Harkin-Jones, E., Rajeev, R. S., Menary, G., Martin, P. J., and Armstrong, C. G. (2012). Morphology, Barrier, and Mechanical Properties of Biaxially Deformed Poly(ethylene Terephthalate)-Mica Nanocomposites. Polym. Eng. Sci. 52, 532–548. doi:10.1002/pen.22114
Tang, Z., Fan, F., Chu, Z., Fan, C., Qin, Y., Bikiaris, D., et al. (2020). Barrier Properties and Characterizations of Poly(lactic Acid)/ZnO Nanocomposites. Molecules 25, 1310. doi:10.3390/molecules25061310
Van Berkel, J. G., Guigo, N., SbirrazzuoliKolstad, N., and Nathanael, S. (2018). Biaxial Orientation of Poly(ethylene 2,5-furandicarboxylate): An Explorative Study. Macromol. Mater. Eng. 303, 1700507. doi:10.1002/mame.201700507
Wang, G., Zhao, J., Ge, C., Zhao, G., and Park, C. B. (2021). Nanocellular Poly(ether-Block-Amide)/MWCNT Nanocomposite Films Fabricated by Stretching-Assisted Microcellular Foaming for High-Performance EMI Shielding Applications. J. Mater. Chem. C. 9, 1245–1258. doi:10.1039/d0tc04099a
Wang, K., Liang, S., Zhao, P., Qu, C., Tan, H., Du, R., et al. (2007). Correlation of Rheology-Orientation-Tensile Property in Isotactic Polypropylene/organoclay Nanocomposites. Acta Materialia 55, 3143–3154. doi:10.1016/j.actamat.2007.01.020
Wang, X., and Shi, G. (2015). Flexible Graphene Devices Related to Energy Conversion and Storage. Energy Environ. Sci. 8, 790–823. doi:10.1039/c4ee03685a
Wu, J.-H., Yen, M.-S., Kuo, M. C., Tsai, Y., and Leu, M.-T. (2014). Poly(ethylene Terephthalate)/poly(ethylene Glycol-Co-1,3/1,4-Cyclohexanedimethanol Terephthalate)/clay Nanocomposites: Mechanical Properties, Optical Transparency, and Barrier Properties. J. Appl. Polym. Sci. 131, a–n. doi:10.1002/app.39869
Xiang, D., Harkin-Jones, E., and Linton, D. (2015). Characterization and Structure-Property Relationship of Melt-Mixed High Density Polyethylene/multi-Walled Carbon Nanotube Composites under Extensional Deformation. RSC Adv. 5, 47555–47568. doi:10.1039/c5ra06075c
Xiang, D., Wang, L., Tang, Y., Harkin-Jones, E., Zhao, C., and Li, Y. (2017). Processing-property Relationships of Biaxially Stretched Binary Carbon Nanofiller Reinforced High Density Polyethylene Nanocomposites. Mater. Lett. 209, 551–554. doi:10.1016/j.matlet.2017.08.104
Xiang, D., Wang, L., Zhang, Q., Chen, B., Li, Y., and Harkin-Jones, E. (2018). Comparative Study on the Deformation Behavior, Structural Evolution, and Properties of Biaxially Stretched High-Density Polyethylene/carbon Nanofiller (Carbon Nanotubes, Graphene Nanoplatelets, and Carbon Black) Composites. Polym. Compos. 39, E909–E923. doi:10.1002/pc.24328
Xiang, D., Zhang, X., Han, Z., Zhang, Z., Zhou, Z., Harkin-Jones, E., et al. (2020). 3D Printed High-Performance Flexible Strain Sensors Based on Carbon Nanotube and Graphene Nanoplatelet Filled Polymer Composites. J. Mater. Sci. 55, 15769–15786. doi:10.1007/s10853-020-05137-w
Xing, B., Cao, J., Wang, Y., Yi, G., Zhang, C., Chen, L., et al. (2015). Preparation of lignite-based Activated Carbon with High Specific Capacitance for Electrochemical Capacitors. Funct. Mater. Lett. 08, 1550031. doi:10.1142/S1793604715500319
Xing, Z., Lu, W., Cheng, L., Zhang, C., Xiao, Y., Shi, X., et al. (2019). Influences of Bi-axial Orientation on the Crystallization and DC Breakdown Properties of Polypropylene Films. Proc. 21st Int. Symp. High Voltage Eng. 599, 91–98. doi:10.1007/978-3-030-31680-8_10
Yang, B., Pan, Y., Yu, Y., Wu, J., Xia, R., Wang, S., et al. (2020a). Filler Network Structure in Graphene Nanoplatelet (GNP)-filled Polymethyl Methacrylate (PMMA) Composites: From Thermorheology to Electrically and Thermally Conductive Properties. Polym. Test. 89, 106575. doi:10.1016/j.polymertesting.2020.106575
Yang, H., Gong, L. H., Zheng, Z., and Yao, X. F. (2020b). Highly Stretchable and Sensitive Conductive Rubber Composites with Tunable Piezoresistivity for Motion Detection and Flexible Electrodes. Carbon 158, 893–903. doi:10.1016/j.carbon.2019.11.079
Youm, J. S., Park, J.-J., and Kim, J. C. (2020). Effect of Electron Beam Irradiation on Gas-Barrier Property of Biaxially Drawn Nylon/Montmorillonite Nanocomposite Films. Macromol. Res. 28, 925–931. doi:10.1007/s13233-020-8119-8
Yue, G., Li, F., Yang, G., and Zhang, W. (2016). Efficient Nickel Sulfide and Graphene Counter Electrodes Decorated with Silver Nanoparticles and Application in Dye-Sensitized Solar Cells. Nanoscale Res. Lett. 11, 239. doi:10.1186/s11671-016-1456-z
Zhang, W., Wei, L., Ma, Z., Fan, Q., and Ma, J. (2021). Advances in Waterborne Polymer/carbon Material Composites for Electromagnetic Interference Shielding. Carbon 177, 412–426. doi:10.1016/J.CARBON.2021.02.093
Keywords: biaxial stretching, polymer, nanocomposites, nanofillers, orientation
Citation: Chen X, Xiang D, Zhou Z, Wu Y, Li H, Zhao C and Li Y (2021) Biaxial Stretching of Polymer Nanocomposites: A Mini-Review. Front. Mater. 8:725422. doi: 10.3389/fmats.2021.725422
Received: 15 June 2021; Accepted: 01 September 2021;
Published: 13 September 2021.
Edited by:
Andreas J. Brunner, Retired, Zürich, SwitzerlandReviewed by:
Jianfeng Wang, Zhengzhou University, ChinaPei Huang, Chongqing University, China
Souvik Chakraborty, Clausthal University of Technology, Germany
Copyright © 2021 Chen, Xiang, Zhou, Wu, Li, Zhao and Li. This is an open-access article distributed under the terms of the Creative Commons Attribution License (CC BY). The use, distribution or reproduction in other forums is permitted, provided the original author(s) and the copyright owner(s) are credited and that the original publication in this journal is cited, in accordance with accepted academic practice. No use, distribution or reproduction is permitted which does not comply with these terms.
*Correspondence: Dong Xiang, ZHhpYW5nMDFAaG90bWFpbC5jb20=; Zuoxin Zhou, YWxmcmVkemhvdTYxQGhvdG1haWwuY29t