- 1Department of Chemistry, University of Okara, Okara, Pakistan
- 2Department of Chemistry, Khwaja Fareed University of Engineering and Information Technology, Rahim Yar Khan, Pakistan
- 3Institute of Chemical Sciences, Bahauddin Zakariya University, Multan, Pakistan
- 4Department of Chemistry, University of Agriculture, Faisalabad, Pakistan
- 5Division of Inorganic Chemistry, Institute of Chemistry, Baghdad-ul-Jadeed Campus, The Islamia University of Bahawalpur, Bahawalpur, Pakistan
- 6Department of Chemistry, Faculty of Science, King Khalid University, Abha, Saudi Arabia
- 7Department of Chemistry, University of Sargodha, Sargodha, Pakistan
- 8Department of Urology, Jiading Branch of Shanghai General Hospital, Shanghai Jiao Tong University School of Medicine, Shanghai, China
- 9Department of Chemistry, Chemical Engineering and Biotechnology, Donghua University, Shanghai, China
High-tech electronic, optics, and storage devices require organic compounds with nonlinear optical (NLO) properties. This study designed D-π-A based dyes with donor triphenylamine (TPA) and acceptor dicyanovinylene (DCV) species by structurally modifying π-conjugated linkers. Our density functional theory (DFT) computations analyzed the impact of structural variations on the nonlinear optical (NLO) response of newly designed dyes. The B3LYP/6-31G(d,p) level determined the quantic chemical insights: frontier molecular orbital (FMOs), natural bond orbitals (NBOs), and nonlinear optical (NLO) properties of the designed dyes (DPTM-1 to DPTM-12). UV-Vis analysis based on the TD-DFT/CAM-B3LYP/6-311+G(d,p) level explored the optoelectronic properties. DPTM-1 and DPTM-5 showed the highest red-shifted absorption band at 519 and 506 nm. NBO analysis shows that DPTM-1 to DPTM-12 dyes have positive values for all donors (D) and π-spacers but negative values for acceptors (A). The π-spacers act as a conveyer between donor and acceptor moieties; thus, electrons were transferred smoothly from D to A units, which resulted in a charge separation state. Our calculations show the extent of NLO response in terms of electronic transitions, polarizability <α>, and first hyperpolarizability (β) values. The highest value of βtotal was 110,509.23 a.u. manifested in DPTM-6 due to 2,5-dimethyloxazole as a second π-linker, twice that of R (66,275.95 a.u.). Also, DPTM-6 and DPTM-8 exhibit the lowest energy band gap of 2.06 and 2.04 eV, respectively. In short, all DPTM-1 to DPTM-12 dyes manifested maximum absorption, lowest energy band gap, greater charge transfer from donor to the acceptor, and better first hyperpolarizability values as compared to the R and showed good NLO response. The present work represents new compounds with remarkable NLO properties and their applications in modern high-tech devices.
Introduction
With the rapid rise of high-tech electronic, optics, and storage devices, organic compounds with NLO properties gained unparalleled popularity and dominance in various disciplines, such as medicine, material science, atomic, molecular, and solid-state physics, surface interface sciences, and chemical dynamics (Eaton, 1991; Peng and Yu, 1994; Tsutsumi et al., 1998; Breitung et al., 2000; Christodoulides et al., 2010). Organic NLO materials, especially with larger π-extended frames, are significantly interesting as dynamic intermolecular charge transfer (ICT) materials with excellent NLO response, facile synthesis, optical switching, frequency shifting, and better signal reception. The presence of delocalized electrons in the organic compounds leads to further enhancement in the NLO response (Anthony et al., 2008; Yamashita, 2011; Ivanov et al., 2013; Khoo, 2014). A significant amount of NLO responses has been observed in organic compounds due to π-electrons with strong electric polarization, low dielectric coefficient, quick response, and high laser damage threshold and π-bond system involves charge distribution from donor to acceptor (Sung and Hsu, 1998; Hochberg et al., 2006). Intermolecular charge transfer occurs from electron-donating to the electron-withdrawing units by the π-conjugated linkers directly linked with the first hyperpolarizability (β) (Zyss, 1987; Prasad and Williams, 1991; Drozd and Marchewka, 2005; Janjua, 2017).
HOMO and LUMO allow charge transfer, but the π-conjugated system leads to charge transfer in an electric field where the π-spacer is a basic molecule (Datta and Pal, 2005; Siddiqui et al., 2012; Garza et al., 2014). By changing the conjugation system the properties of dyes can vary and the NLO response can improve by choosing the finest length of conjugation. Appropriate arrangement of donor, acceptors, and π-bridge plays a crucial role in designing a competent organic compound (Dalton, 2001; Dalton, 2002). This appropriate arrangement enhances the electronic distribution, induces charge separation, increases absorption range towards longer wavelength, reduces charge recombination, and decreases the HOMO-LUMO energy gap, resulting in an improved NLO response. There are large dipole moments between the ground and excited state of the compounds because of ICT, including electron density transfer from D to A units through π-bridge (Haid et al., 2012; Srinivasan et al., 2017). Literature has shown that NLO response can be improved when ICT is enhanced by ring twisting and optimizing the D-A pair (Dulcic et al., 1981; Berkovic et al., 1987). The organic dyes with π-bond system involved delocalization of charge. The major effect of π-bridge on NLO activity is also important in the prediction of optimized NLO properties. Although D units have a major impact on NLO properties, one cannot ignore the role of the acceptor unit. Different types of π-spacers are helpful in a good connection between HOMO and LUMO units to have a good NLO response (Khan et al., 2018a).
Literature is overflowing with various designs comprising A−π−D−π−A, D−A, D−π−A, D−π−A−π−D, D−A−π−A, D−π−π−A, and D−D−π−A (Khalid et al., 2020). It has been determined by both experimental and theoretical inspection that D−π−A types of designed dyes show excellent NLO responses (Khan et al., 2018b). Furthermore, the optimal length of π-conjugation, composited in between donor and acceptor, also contributes to an increased NLO response (Saeed et al., 2020). That is why, in this study, we have tailored the π-conjugated system and designed the D-π-A type of compounds (DPTM-1 to DPTM-12). To the best of our knowledge, these compounds have never been designed before for the efficient theoretical study for NLO properties.
Computational Procedure
In the present study, NBO analysis, electronic structures, absorption spectra, and NLO properties of D−π−A dyes were calculated by performing density functional theory and time-dependent density functional theory. All computational calculations were performed using Gaussian 09 package program (Olson and Boldyrev, 2012). Ground state geometry optimization of the dyes is determined in the gas phase to confirm that no imaginary frequencies are involved in dyes through the B3LYP (Civalleri et al., 2008) level of theory combined with a 6-31G(d,p) (Afzal et al., 2020; Bilal Ahmed Siddique et al., 2020; Hussain et al., 2020; Mehboob et al., 2020) basis set. The HOMO-LUMO energies and their differences were calculated using the same function. FMO investigation proved highly beneficial in understanding electronic transitions from donor to the acceptor orbital. The charge transfer interactions in the entitled compounds were observed in the NBO analysis. TD-DFT calculations with the CAM-B3LYP/6-311+G(d,p) level were used to comprehend the UV-Visible absorption spectra of the entitled molecules (Yanai et al., 2004). All input files were made by Gauss View 5.0 (Vural et al., 2017), whereas output effects were construed and visualized using Gauss View, Avogadro (Hanwell et al., 2012), and Chemcraft (Dena et al., 2019) programs. The Gaussian output presented six linear polarizability tensors (axx, ayy, azz, axy, axz, ayz) and ten first hyperpolarizability tensors (βxxx, βxyy, βxzz, βyyy, βxxy, βyzz, βzzz, βxxz, βyyz, βxyz) along the x, y, and z directions, respectively. Polarizabilities and first hyperpolarizability values of the design compounds (DPTM-1 to DPTM-12) have been calculated at the same level of theory. Equations 1 and 2 can be utilized for calculating the polarizability <α> and first hyperpolarizability (βtotal) of the entitled compounds (Karakas et al., 2007).
Results and Discussion
Khan et al. modified a D-π-A-based 2-((5-(2-(4-(diphenylamino)phenyl)benzo[d]thiazol-5-yl)thiophen-2yl)methylene)malononitrile dye (R) by changing π-conjugated linkers between the fixed donor (triphenylamine) and acceptor (dicyanovinylene) species to enhance its NLO properties (Khan et al., 2018a). In this present work, eight π-linkers that were not previously reported are used to design organic dye with potential NLO responses. The molecular and optimized structures of the entitled dyes, as shown in Figures 1, 2, have three fragments, D, π-spacer, and A. In the designed compounds (DPTM-1 to DPTM-12), triphenylamine (TPA) acts as a donor and dicyanovinylene (DCV) as an acceptor unit with various π-conjugated linkers. Various combinations were made to design twelve new TPA-DCV based D-π-A compounds involving six π-spacers used as first π-linkers and two π-spacers as second π-linkers. To judge the impact of structural changes on NLO properties, density functional theory (DFT) and time-dependent density functional theory (TD-DFT) calculations were performed.
Electronic Structure
The FMO analysis is considered a standard approach to predict the chemical stability in molecules (Gunasekaran et al., 2008). The frontier molecular orbitals, i.e., HOMO (highest occupied molecular orbital) and LUMO (lowest unoccupied molecular orbital), play an exceptional role in the absorption spectra and mechanical illustration of the compounds. Usually, HOMO is referred to as an electron donator, while LUMO is usually regarded as an acceptor (Amiri et al., 2016). Band gap (ELUMO-EHOMO) is considered an exceptional factor in predicting hardness, strength, softness, dynamic stability, and chemical reactivity of the deliberated dyes (Khalid et al., 2017), where a sizeable band gap shows minimum reactivity and maximum stability and hardness of a molecule. In contrast, highly reactive and soft molecules, predicted with a small ELUMO-EHOMO band gap, are far more polarized and serve as a finer competitor in offering the best NLO response (Parr et al., 1999; Lesar and Milošev, 2009; Chattaraj et al., 2011). EHOMO, ELUMO, and bandgap (ELUMO—EHOMO) for DPTM-1 to DPTM-12 have been determined by the DFT computational analysis. The calculated values are given in Table 1.
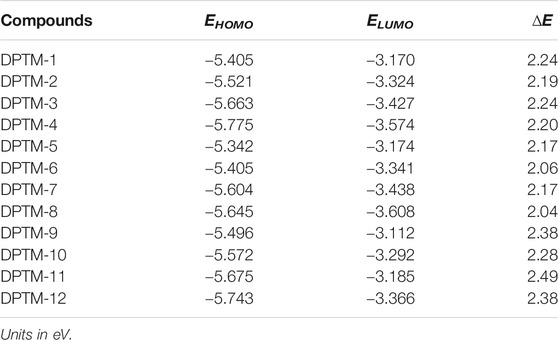
TABLE 1. The EHOMO, ELUMO, and energy gap (ELUMO-EHOMO) of investigated compounds (DPTM-1 to DPTM-12).
It is observed that all the designed dyes tend to show relatively low band gaps than the reference (R), i.e., 2.4 eV (Khan et al., 2018a), which manifests a remarkable outcome of the used π-conjugates in HOMO-LUMO transition states. The increasing energy gap order of R and DPTM-1 to DPTM-12 is observed as DPTM-8<DPTM-6<DPTM-7_DPTM-5<DPTM-2<DPTM-4<DPTM-1_DPTM-3<DPTM-10<DPTM-12_DPTM-9<R < DPTM-11 (Table 1).
The HOMO-LUMO band gap in DPTM-8 is the least among all dyes, i.e., 2.04 eV due to first π-linker 2,2′-dimethyl-5,5′-bioxazole and second π-linker 2,5-dimethyloxazole. These two π-conjugated linkers have provided a better bridge for transferring electrons from the TPA to DCV. ∆E of DPTM-11 was found greater than 2.49 eV. The first and second π-linkers 2,5-dimethylbenzo[d]oxazole and 2,5-dimethylfuran, used in DPTM-11, have interrupted the transfer of electrons from the TPA to DCV (Figures 1, 2). That is why DPTM-11 has shown the highest band gap among all the designed dyes. These smaller band gaps have shown favorable NLO properties. The HOMO-LUMO (+1, −1, +2, −2) value and band gaps are mentioned in the supplementary information (Supplementary Table 13 and Supplementary Figure 1). The structural display of HOMO-LUMO orbitals with electron density distribution pattern is shown in Figure 3. In HOMO, the orbital electron density is mainly exhibited on the donor TPA and partially present along with first π-linkers. In the case of LUMO, the electron density predominantly occurred on the acceptor side DCV and is slightly present along with second π-linkers. This revealed the charge transfer from donor to acceptor moieties and illustrated the enhanced properties of NLO materials. Structural modifications by changing the π-conjugated linkers exhibited remarkable results for NLO resources.
Global Reactivity Parameters
The bandgap (ELUMO-EHOMO) is crucial in describing the global reactivity parameters such as global hardness (η), global softness (
The outcomes from the equations are represented in Table 2.
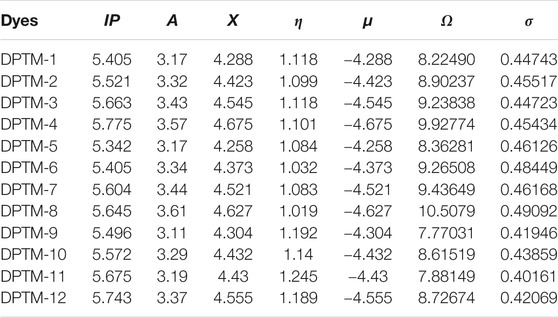
TABLE 2. Ionization potential (IP), electron affinity (A), electronegativity (X), global hardness (η), chemical potential (μ), global electrophilicity (ω), and global softness (σ) of entitled compounds (DPTM-1 to DPTM-12).
The electron affinity represents electron-withdrawing ability, while electron-donating tendency can be determined by the ionization potential. Among all the entitled dyes, maximum electron affinity is observed in DPTM-8, i.e., 3.61 a.u. while the maximum ionization potential, i.e., 5.775 a.u., is shown by DPTM-4 (Table 2). Electronegativity is a tendency to attract the electron towards itself, while chemical potential (µ) value describes the stability of molecules. A more negative value of chemical potential represents the stability of the molecules. The highest negative value of chemical potential is determined in DPTM-4 as -4.675. The bandgap and hardness of the molecules have a direct relation. A sizeable bandgap describes a less reactive molecule with greater hardness and high kinetic stability. Furthermore, if a molecule has less energy bandgap, it describes the more reactive molecule, with lesser hardness and lower kinetic strength.
Among all the designed compounds, the lowest hardness value is calculated in DPTM-6, i.e., 1.032 a.u. In DPTM-7, first and second π-conjugated linkers such as 5,5′-dimethyl-2,2′-bifuran and 2,5-dimethyloxazole provided a better path for transfer of electrons from HOMO-LUMO orbital in a minimum amount of energy. The least chemical stability and the maximum hardness are observed in DPTM-11 as 1.245 a.u. The decreasing order of hardness of the investigated molecules is DPTM-11>DPTM-9>DPTM-12>DPTM-10>DPTM-3>DPTM-1>DPTM-4>DPTM-2>DPTM-5>DPTM-7>DPTM-6> DPTM-8.
Softness is another factor that reveals molecule’s reactivity as it is directly related to chemical reactivity. In the designed moieties, DPTM-8 has higher value of softness, 0.49092 a.u. The DPTM-8 has first π-conjugated linkers 2,2′-dimethyl-5,5′-bioxazole and second π-linker 2,5-dimethyloxazole that play a crucial role in transfer of the electron density from D to A moieties without any barrier. The decreasing order of softness of investigated molecules is DPTM-8>DPTM-6>DPTM-7>DPTM-5>DPTM-2>DPTM-4>DPTM-1>DPTM-3>DPTM-10>DPTM-12>DPTM-9>DPTM-11. Therefore, DPTM-8 has shown the lowest band gap among all the dyes, showing maximum softness and significant NLO properties.
Natural Bond Orbital (NBO) Analysis
NBO is a technique that thoroughly explains the charge transfer and conjugative interactions between the donor and acceptor moieties (James et al., 2006; Szafran et al., 2007). It also provides a steady explanation of intermolecular interactions between two or more counterparts. It is based on the lone pair and bond pair interactions. It is a beneficial technique to study the transmission of electrons from the D orbital to the A orbital in the D-π-A system. The charge transfer of studied compounds findings is expressed in Table 3.
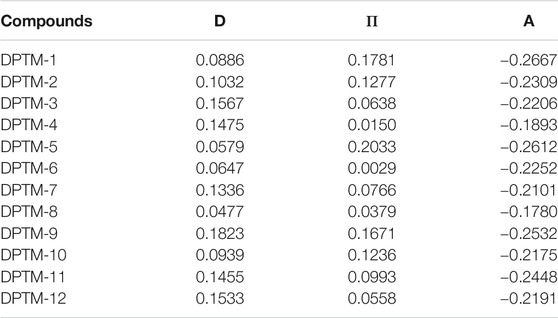
TABLE 3. NBO charges for donor, π-linkers, and acceptor of the investigated compounds (DPTM-1 to DPTM-12).
It has been observed from Table 3 that donor units contain a positive charge, due to which it has excellent electron-donating power. However, acceptor moiety holds a negative charge describing that acceptors will accept electrons effectively. The π-spacers have positive charges, demonstrating that they will not stop the electrons transfer and act as a bridge to smoothly transfer charge densities from donor to acceptor units. Transfer of charge from D (filled) orbital to A (vacant) orbital is because of the hyperconjugative and intra- and intermolecular interactions. It has been observed that the highest NBO charge values were observed for DPTM-1 and the least NBO charge values were observed for R. The most suitable push-pull architecture is seen in DPTM-1, which is conveying the charge towards π-bridge to act as a conveyer and allowed to pass the charge towards the acceptor unit. The electronic transition types of NBO (DPTM-1 to DPTM-12) are demonstrated in the supplementary information (Supplementary Tables 14–25). Thus, the results prove the successful transfer of charges from filled to vacant orbital through π-spacer. The NBO charge values of other dyes also showed a close relation to each other.
Nonlinear Optical (NLO) Properties
NLO dyes and materials are widely used in communication technology, signal processing optical switches, and memory devices. A thorough understanding of NLO properties is required for the design of these materials. Electronic properties are measured to be accountable for the power of photosensitive response, which in turn depends on linear effect (polarizability, α) and nonlinear effect (hyperpolarizabilities, β and γ, etc.).
Therefore, unfolding of NLO response of (DPTM-1 to DPTM-12), the nonlinear and linear factors are estimated using (B3LYP) level with 6-3IG (d, p) basis set. The <α> values in Table 4 describe the effect of π-linkers on NLO and linear properties of entitled compounds. Polarizability is defined by a second rank tensor <α>. The <α> of the R observed is 645.71 a.u. (Khan et al., 2018a). Odd number of design compounds having 2,5-dimethylfuran as a second π-linker, the highest value of <α> is observed for DPTM-5 (677.51 a.u.) with 5,5′-dimethyl-2,2′-bifuran as a conjugated first π-linker. This value lessens to 663.48 a.u. in DPTM-1 containing 5-dimethylfuro[3,2-b]furan as a first π-linker; this value more decreases to 640.19 a.u. inDPTM-3 having 2,5-dimethyloxazolo[5,4-d]oxazole as a first π-linker. In case of DPTM-7, DPTM-9, and DPTM-11 the value of <α> further decreases to 625.88, 615.55, and 595.48 a.u. with 2,2′-dimethyl-5,5′-bioxazole, 2,5-dmiethylbenzofuran, and 2,5-dimethylbenzo[d]oxazole first π-linker, respectively. In the same way, DPTM-2, DPTM-4, DPTM-6, DPTM-8, DPTM-10, and DPTM-12 have 2,5-dimethyloxazole as a second π-linker. Among these compounds, DPTM-6 comprises the highest value of <α> (668.19 a.u.) with 5,5′-dimethyl-2,2′-bifuran as a first π-linker. Moreover, this value decreases to 649.34, 629.01, 619.24, and 605.58 a.u. for DPTM-2, DPTM-4, DPTM-8, and DPTM-10 comprising 2,5dimethylfuro[3,2-b]furan, 2,5-dimethyloxazolo[5,4-d]oxazole, 2,2′-dimethyl-5,5′-bioxazole, and DPTM-2,5-dmiethylbenzofuran as first π-linker, respectively. The least value of <α> is observed in case of DPTM-12 (584.13 a.u.) with 2,5-dimethylbenzo[d]oxazole as a first π-linker. DPTM-5 showed an amplified value of <α > 677.51 (a.u.), indicating a strong NLO effect, while DPTM-12 molecule reveals retracted value of <α > 584.13 a.u. The overall decreasing order of polarizability is DPTM-5>DPTM-6>DPTM-1>R>DPTM-3>DPTM-4>DPTM-7>DPTM-8>DPTM-9>DPTM-10>DPTM-11>DPTM-12 (Table 4).
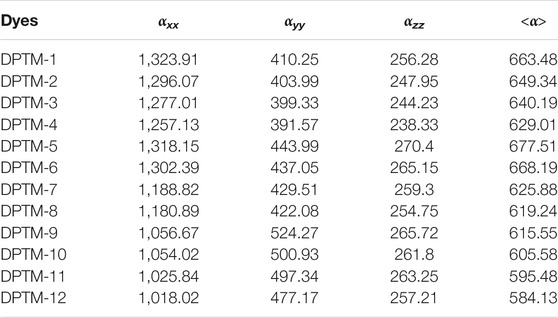
TABLE 4. Dipole polarizabilities and major contributing tensors (a.u.) of investigated compounds (DPTM-1 to DPTM-12).
According to the literature, polarizability of a molecule is influenced by the energy bandgap between LUMO and HOMO. Small amounts of energy gap are required to demonstrate a high level of linear polarizability. Generally speaking, compounds with a small ∆E and large <α> value suggest high β values (Qin and Clark, 2007). Electronic transitions along the x and y axes are commonly used to calculate polarizability. To illustrate dipole polarizability, Eq. 10 is utilized:
The transition moment is indicated as
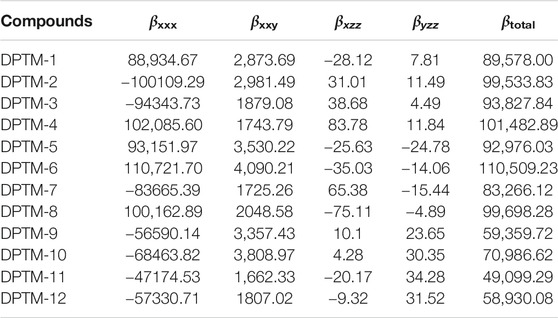
TABLE 5. The computed (βtotal) and major contributing tensor (a.u.) of the investigated compounds (DPTM-1 to DPTM-12).
A third rank tensor represents the first hyperpolarizability. The βtotal of the R observed is 66,275.95 a.u. (Khan et al., 2018a). Among all the compounds having 2,5-dimethylfuran as a second π-linker DPTM-3 has the highest value of βtotal (93,827.84 a.u.) with 2,5-dimethyloxazolo[5,4-d]oxazole as first π-linker. This value then decreases to 92,976.03 a.u. in DPTM-5 that further diminishes to 85,798 a.u. in DPTM-1. A decrease in the βtotal occurs from DPTM-7 to DPTM-9 and DPTM-11, with 83,266.12, 59,359.72, and 49,099.29 a.u. Similarly in the compounds having 2,5-dimethyloxazole as a second π-linker, DPTM-6 is observed with the highest value of βtotal (110,509.23 a.u.) and the least value is observed in DPTM-12 (58,930.08 a.u.). The overall increasing order for βtotal of all entitled dyes is DPTM-11<DPTM-12<DPTM-9<R<DPTM-10<DPTM-7<DPTM-1<DPTM-5<DPTM-3<DPTM-2<DPTM-8<DPTM-4<DPTM-6 (Table 5).
UV-Visible Spectral Analysis
UV-Vis spectroscopy provides valuable information about electronic transitions, charge transfer, and contributing configurations of the molecule. TD-DFT computations were carried out in the gas phase to calculate the electronic excitation spectra of the investigated designed compounds DPTM-1 to DPTM-12 at CAM-B3LYP/6-311G+(d,p) level. The transition between HOMO and LUMO is the main focus of the UV spectra. Electronic transitions were pointed out through wavelength, frequency, and computed transition energy. During TD-DFT computations, the six lowest singlet transitions were studied (Supplementary Tables 27–38). The wavelength related to λmax, oscillator strength (fos), transition nature, transition moment (
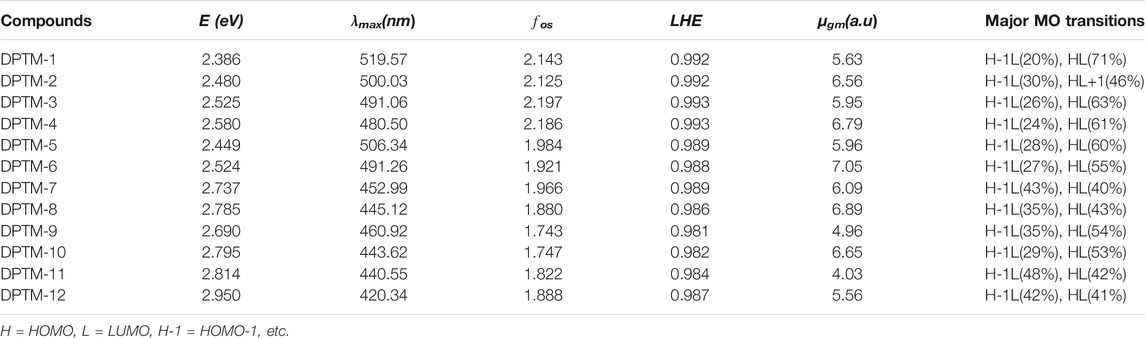
TABLE 6. Transition energy (eV), maximum absorption wavelengths (λmax), oscillator strengths (fos), light-harvesting efficiency (LHE), transition moment (
The maximum wavelength absorbed by all the studied compounds (DPTM-1 to DPTM-12) is in the visible region. The highest λmax has been observed in DPTM-1, which is 519.57 nm. The highest λmax value indicated that greater electronic transition occurred in DPTM-1 dye from HOMO to LUMO orbital. All the investigated dyes have shown greater λmax values from the R (430.50 nm) (Khan et al., 2018a) except the DPTM-12. From Table 6, it is apparent that the electron transitions of all dyes (except DPTM-7, DPTM-11, and DPTM-12) principally originate from TPA (H) to the DCV (L) along the x direction. In DPTM-7, DPTM-11, and DPTM-12, the charge transfer mainly occurs from H-1 to L. The decreasing order of λmax is DPTM-1>DPTM-5>DPTM-2>DPTM-6>DPTM-3>DPTM-4>DPTM-9>DPTM-7>DPTM-8>DPTM10> DPTM-11>R>DPTM-12 (Table 6).
The optical efficiency is directly related to a factor named light-harvesting efficiency (LHE). A larger LHE value is linked to the higher photocurrent response of the compound and vice versa. Eq. 11 used to represent LHE is (Nalwa, 2000)
In Eq. 11, f stands for strength of oscillations. LHE of DPTM-3 and DPTM-4 is the highest among all the investigated compounds as given in Table 6. These calculations indicate a better connection between donor and acceptor units leading to a potential NLO response. Oudar and Chemla (Oudar and Chemla, 1977) presented the two-level models which explain the relationship between charge transfer transitions, and the first hyperpolarizability depends upon sum-over-states (SOS) relation showed in
In this expression, βCT is the first hyperpolarizability, ∆μgm represents dipole moment difference, fgm represents the strength of oscillation from the lower to higher state, and
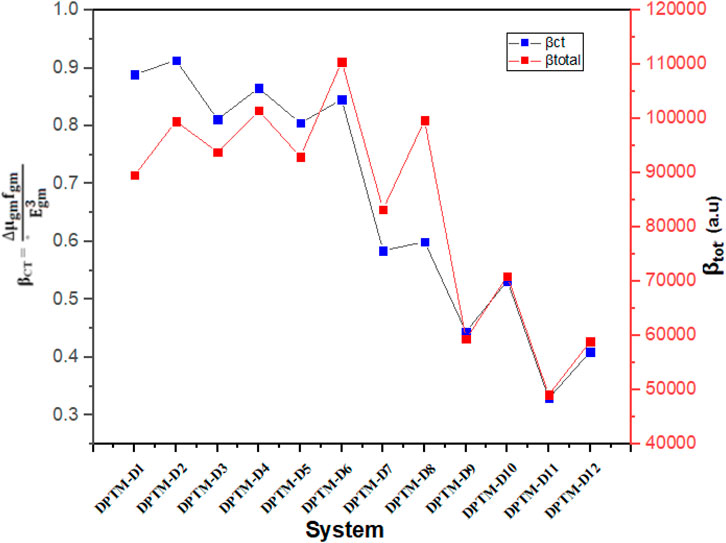
FIGURE 5. Relation between the βtotal values and the consistent βCT values of the investigated compounds (DPTM-1 to DPTM-12).
Conclusion
In summary, the objective of the present study was to investigate NLO properties of metal-free, theoretically designed organic dyes (DPTM-1 to DPTM-12) comprising of various π-linkers mixed with D and A. To elucidate the impact of various π-bridges on NLO properties, TD-DFT and DFT methods have been employed on designed dyes (DPTM-1 to DPTM-12). Electronic structures, absorption spectra, energy difference, and first hyperpolarizability values are explained by the quantum chemical methods. All dyes have shown maximum absorbance in the visible region, low transition energy, LHE, and oscillating strength values as compared to R. The highest red-shifted absorption spectra have been observed at 519.57 nm in DPTM-1. The NBO analysis explained the charge transfer mechanism from donor to acceptor part through the π-conjugated linkers. The highest NBO charge transfer was observed for DPTM-1. The FMO analysis illustrated electron density of all dyes, in HOMO electron density is primarily located on the DPA and first π linkers and in LUMO electron density is located at the DCV. Electron density transfer from the HOMO orbital to LUMO orbital plays an important role to enhance the NLO properties. The lowest HOMO to LUMO energy difference was observed for DPTM-4, DPTM-6, and DPTM-8 with the lowest Egap of 2.20, 2.06, and 2.04 eV, respectively. The greater amount of NLO properties was 101,482.89, 110,509.23, and 99,698.28 a.u. in DPTM-4, DPTM-6, and DPTM-8, respectively, with urea as a standard. The DPTM-6βtot value is twenty-six hundred times greater than urea. Among all designed compounds these compounds exhibit lowest energy band gap and highest NLO response. This present work also depicted the importance of structural tailoring of π-linkers in D−π−A dyes. It is a valuable theoretical approach for the researchers to design new excellent NLO materials. This work also provided new ways to experimentally synthesize new appealing NLO materials for their use in optics and electronics devices.
Data Availability Statement
The original contributions presented in the study are included in the article/Supplementary Material; further inquiries can be directed to the corresponding authors.
Author Contributions
All authors listed have made a substantial, direct, and intellectual contribution to the work and approved it for publication.
Funding
Funding in the Lu lab was provided by the Fundamental Research Funds for the Central Universities (2232021G-04) and Shanghai Science and Technology Committee (19ZR1471100). MI expresses his appreciation to the Deanship of Scientific Research at King Khalid University, Saudi Arabia, for funding through research groups program under grant number R.G.P. 1/318/42.
Conflict of Interest
The authors declare that the research was conducted in the absence of any commercial or financial relationships that could be construed as a potential conflict of interest.
Publisher’s Note
All claims expressed in this article are solely those of the authors and do not necessarily represent those of their affiliated organizations, or those of the publisher, the editors and the reviewers. Any product that may be evaluated in this article, or claim that may be made by its manufacturer, is not guaranteed or endorsed by the publisher.
Supplementary Material
The Supplementary Material for this article can be found online at: https://www.frontiersin.org/articles/10.3389/fmats.2021.719971/full#supplementary-material
References
Afzal, Z., Hussain, R., Khan, M. U., Khalid, M., Iqbal, J., Alvi, M. U., et al. (2020). Designing Indenothiophene-Based Acceptor Materials with Efficient Photovoltaic Parameters for Fullerene-free Organic Solar Cells. J. Mol. Model. 26, 1–17. doi:10.1007/s00894-020-04386-5
Amiri, S. S., Makarem, S., Ahmar, H., and Ashenagar, S. (2016). Theoretical Studies and Spectroscopic Characterization of Novel 4-Methyl-5-((5-Phenyl-1, 3, 4-Oxadiazol-2-Yl) Thio) Benzene-1, 2-diol. J. Mol. Struct. 1119, 18–24. doi:10.1016/j.molstruc.2016.04.053
Anthony, J. K., Kim, H. C., Lee, H. W., Mahapatra, S. K., Lee, H. M., Kim, C. K., et al. (2008). Particle Size-dependent Giant Nonlinear Absorption in Nanostructured Ni-Ti Alloys. Opt. Express 16, 11193–11202. doi:10.1364/oe.16.011193
Berkovic, G., Shen, Y. R., and Shadt, M. (1987). The Effect of Conjugation Length and Electron Donor Groups on the Second Order Nonlinear Polarizability of Cyano Substituted Aromatic Molecules. Mol. Crystals Liquid Crystals Incorporating Nonlinear Opt. 150, 607–616. doi:10.1080/00268948708074818
Bilal Ahmed Siddique, M., Hussain, R., Ali Siddique, S., Yasir Mehboob, M., Irshad, Z., Iqbal, J., et al. (2020). Designing Triphenylamine‐Configured Donor Materials with Promising Photovoltaic Properties for Highly Efficient Organic Solar Cells. ChemistrySelect 5, 7358–7369. doi:10.1002/slct.202001989
Breitung, E. M., Shu, C.-F., and Mcmahon, R. J. (2000). Thiazole and Thiophene Analogues of Donor−Acceptor Stilbenes: Molecular Hyperpolarizabilities and Structure−Property Relationships. J. Am. Chem. Soc. 122, 1154–1160. doi:10.1021/ja9930364
Chattaraj, P. K., Giri, S., and Duley, S. (2011). Update 2 of: Electrophilicity Index. Chem. Rev. 111, PR43–PR75. doi:10.1021/cr100149p
Christodoulides, D. N., Khoo, I. C., Salamo, G. J., Stegeman, G. I., and Van Stryland, E. W. (2010). Nonlinear Refraction and Absorption: Mechanisms and Magnitudes. Adv. Opt. Photon. 2, 60–200. doi:10.1364/aop.2.000060
Civalleri, B., Zicovich-Wilson, C. M., Valenzano, L., and Ugliengo, P. (2008). B3LYP Augmented with an Empirical Dispersion Term (B3LYP-D*) as Applied to Molecular Crystals. Cryst. Eng. Comm. 10, 405–410. doi:10.1039/b715018k
Dalton, L. (2002). “Nonlinear Optical Polymeric Materials: From Chromophore Design to Commercial Applications,” in Polymers for Photonics Applications I (Springer), 1–86. doi:10.1007/3-540-44608-7_1
Dalton, L. (2001). “The Role of Nonlinear Optical Devices in the Optical Communication Age,” in Nonlinear Optics for the Information Society (Springer), 1. doi:10.1007/978-94-015-1267-1_1
Datta, A., and Pal, S. (2005). Effects of Conjugation Length and Donor-Acceptor Functionalization on the Non-linear Optical Properties of Organic Push-Pull Molecules Using Density Functional Theory. J. Mol. Struct. THEOCHEM 715, 59–64. doi:10.1016/j.theochem.2004.10.054
Dena, A. S. A., Muhammad, Z. A., and Hassan, W. M. J. C. P. (2019). Spectroscopic, DFT Studies and Electronic Properties of Novel Functionalized Bis-1, 3, 4-thiadiazoles. Chem. Pap. 73, 2803–2812. doi:10.1007/s11696-019-00833-7
Drozd, M., and Marchewka, M. K. (2005). The Structure, Vibrational Spectra and Nonlinear Optical Properties of Neutral Melamine and Singly, Doubly and Triply Protonated Melaminium Cations-Theoretical Studies. J. Mol. Struct. THEOCHEM 716, 175–192. doi:10.1016/j.theochem.2004.11.020
Dulcic, A., Flytzanis, C., Tang, C. L., Pépin, D., Fétizon, M., and Hoppilliard, Y. (1981). Length Dependence of the Second‐order Optical Nonlinearity in Conjugated Hydrocarbons. J. Chem. Phys. 74, 1559–1563. doi:10.1063/1.441296
Eaton, D. F. (1991). Nonlinear Optical Materials: The Great and Near Great. Washington, DC: ACS Publications.
Fukui, K. (1982). Role of Frontier Orbitals in Chemical Reactions. science 218, 747–754. doi:10.1126/science.218.4574.747
Garza, A. J., Osman, O. I., Wazzan, N. A., Khan, S. B., Asiri, A. M., and Scuseria, G. E. (2014). A Computational Study of the Nonlinear Optical Properties of Carbazole Derivatives: Theory Refines experiment. Theor. Chem. Acc. 133, 1458. doi:10.1007/s00214-014-1458-9
Gunasekaran, S., Balaji, R. A., Kumeresan, S., Anand, G., and Srinivasan, S. (2008). Experimental and Theoretical Investigations of Spectroscopic Properties of N-Acetyl-5-Methoxytryptamine. Can. J. Anal. Sci. Spectrosc. 53, 149–162.
Haid, S., Marszalek, M., Mishra, A., Wielopolski, M., Teuscher, J., Moser, J.-E., et al. (2012). Significant Improvement of Dye-Sensitized Solar Cell Performance by Small Structural Modification in π-Conjugated Donor-Acceptor Dyes. Adv. Funct. Mater. 22, 1291–1302. doi:10.1002/adfm.201102519
Hanwell, M. D., Curtis, D. E., Lonie, D. C., Vandermeersch, T., Zurek, E., and Hutchison, G. R. J. J. O. C. (2012). Avogadro: an Advanced Semantic Chemical Editor, Visualization, and Analysis Platform. J. Cheminformatics 4, 1–17. doi:10.1186/1758-2946-4-17
Hochberg, M., Baehr-Jones, T., Wang, G., Shearn, M., Harvard, K., Luo, J., et al. (2006). Terahertz All-Optical Modulation in a Silicon-Polymer Hybrid System. Nat. Mater 5, 703–709. doi:10.1038/nmat1719
Hussain, R., Khan, M. U., Mehboob, M. Y., Khalid, M., Iqbal, J., Ayub, K., et al. (2020). Enhancement in Photovoltaic Properties of N , N ‐diethylaniline Based Donor Materials by Bridging Core Modifications for Efficient Solar Cells. ChemistrySelect 5, 5022–5034. doi:10.1002/slct.202000096
Ivanov, I. P., Li, X., Dolan, P. R., and Gu, M. (2013). Nonlinear Absorption Properties of the Charge States of Nitrogen-Vacancy Centers in Nanodiamonds. Opt. Lett. 38, 1358–1360. doi:10.1364/ol.38.001358
James, C., Raj, A. A., Reghunathan, R., Jayakumar, V. S., and Joe, I. H. (2006). Structural Conformation and Vibrational Spectroscopic Studies of 2,6-Bis(p-N,n-Dimethyl Benzylidene)cyclohexanone Using Density Functional Theory. J. Raman Spectrosc. 37, 1381–1392. doi:10.1002/jrs.1554
Janjua, M. R. S. A. (2017). Nonlinear Optical Response of a Series of Small Molecules: Quantum Modification of π-spacer and Acceptor. J. Iran Chem. Soc. 14, 2041–2054. doi:10.1007/s13738-017-1141-x
Karakas, A., Elmali, A., and Unver, H. (2007). Linear Optical Transmission Measurements and Computational Study of Linear Polarizabilities, First Hyperpolarizabilities of a Dinuclear Iron(III) Complex. Spectrochimica Acta A: Mol. Biomol. Spectrosc. 68, 567–572. doi:10.1016/j.saa.2006.12.029
Khalid, M., Ali, A., Jawaria, R., Asghar, M. A., Asim, S., Khan, M. U., et al. (2020). First Principles Study of Electronic and Nonlinear Optical Properties of A-D-π-A and D-A-D-π-A Configured Compounds Containing Novel Quinoline-Carbazole Derivatives. RSC Adv. 10, 22273–22283. doi:10.1039/d0ra02857f
Khalid, M., Ali, M., Aslam, M., Sumrra, S. H., Khan, M. U., Raza, N., et al. (2017). Frontier Molecular, Natural Bond Orbital, UV-Vis Spectral Stduy, Solvent Influence on Geometric Parameters, Vibrational Frequencies and Solvation Energies of 8-Hydroxyquinoline. Int. J. Pharm. Sci. Res. 8, 457. doi:10.13040/IJPSR.0975-8232.8(2).457-69
Khan, M. U., Khalid, M., Ibrahim, M., Braga, A. A. C., Safdar, M., Al-Saadi, A. A., et al. (2018a). First Theoretical Framework of Triphenylamine-Dicyanovinylene-Based Nonlinear Optical Dyes: Structural Modification of π-Linkers. J. Phys. Chem. C 122, 4009–4018. doi:10.1021/acs.jpcc.7b12293
Khan, M. U., Khalid, M., Ibrahim, M., Braga, A. A. C., Safdar, M., Al-Saadi, A. A., et al. (2018b). First Theoretical Framework of Triphenylamine-Dicyanovinylene-Based Nonlinear Optical Dyes: Structural Modification of π-Linkers. J. Phys. Chem. C 122, 4009–4018. doi:10.1021/acs.jpcc.7b12293
Khoo, I. C. (2014). Nonlinear Optics, Active Plasmonics and Metamaterials with Liquid Crystals. Prog. Quan. Elect. 38, 77–117. doi:10.1016/j.pquantelec.2014.03.001
Koopmans, T. (1934). Über die Zuordnung von Wellenfunktionen und Eigenwerten zu den Einzelnen Elektronen Eines Atoms. physica 1, 104–113. doi:10.1016/s0031-8914(34)90011-2
Lesar, A., and Milošev, I. (2009). Density Functional Study of the Corrosion Inhibition Properties of 1,2,4-triazole and its Amino Derivatives. Chem. Phys. Lett. 483, 198–203. doi:10.1016/j.cplett.2009.10.082
Mehboob, M. Y., Hussain, R., Khan, M. U., Adnan, M., Umar, A., Alvi, M. U., et al. (2020). Designing N-Phenylaniline-Triazol Configured Donor Materials with Promising Optoelectronic Properties for High-Efficiency Solar Cells. Comput. Theor. Chem. 1186, 112908. doi:10.1016/j.comptc.2020.112908
Nalwa, H. S. (2000). Handbook of Advanced Electronic and Photonic Materials and Devices, Ten-Volume Set. Academic Press.
Olson, J. K., and Boldyrev, A. I. (2012). Electronic Transmutation: Boron Acquiring an Extra Electron Becomes 'carbon'. Chem. Phys. Lett. 523, 83–86. doi:10.1016/j.cplett.2011.11.079
Oudar, J. L., and Chemla, D. S. (1977). Hyperpolarizabilities of the Nitroanilines and Their Relations to the Excited State Dipole Moment. J. Chem. Phys. 66, 2664–2668. doi:10.1063/1.434213
Parr, R. G., Donnelly, R. A., Levy, M., and Palke, W. E. (1978). Electronegativity: the Density Functional Viewpoint. J. Chem. Phys. 68, 3801–3807. doi:10.1063/1.436185
Parr, R. G., Szentpály, L. v., and Liu, S. (1999). Electrophilicity index. J. Am. Chem. Soc. 121, 1922–1924. doi:10.1021/ja983494x
Peng, Z., and Yu, L. (1994). Second-order Nonlinear Optical Polyimide with High-Temperature Stability. Macromolecules 27, 2638–2640. doi:10.1021/ma00087a039
Prasad, P. N., and Williams, D. J. (1991). Introduction to Nonlinear Optical Effects in Molecules and Polymers. New York: Wiley.
Qin, C., and Clark, A. E. (2007). DFT Characterization of the Optical and Redox Properties of Natural Pigments Relevant to Dye-Sensitized Solar Cells. Chem. Phys. Lett. 438, 26–30. doi:10.1016/j.cplett.2007.02.063
Saeed, A., Muhammad, S., Rehman, S.-U., Bibi, S., Al-Sehemi, A. G., and Khalid, M. (2020). Exploring the Impact of central Core Modifications Among Several Push-Pull Configurations to Enhance Nonlinear Optical Response. J. Mol. Graphics Model. 100, 107665. doi:10.1016/j.jmgm.2020.107665
Siddiqui, S. A., Rasheed, T., Faisal, M., Pandey, A. K., and Khan, S. B. (2012). Electronic Structure, Nonlinear Optical Properties, and Vibrational Analysis of Gemifloxacin by Density Functional Theory. Spectrosc. Int. J. 27. doi:10.1155/2012/614710
Srinivasan, V., Panneerselvam, M., Pavithra, N., Anandan, S., Sundaravel, K., Jaccob, M., et al. (2017). A Combined Experimental and Computational Characterization of D-π-A Dyes Containing Heterocyclic Electron Donors. J. Photochem. Photobiol. A: Chem. 332, 453–464. doi:10.1016/j.jphotochem.2016.09.026
Sung, P.-H., and Hsu, T.-F. (1998). Thermal Stability of NLO Sol-Gel Networks with Reactive Chromophores. Polymer 39, 1453–1459. doi:10.1016/s0032-3861(97)85885-5
Szafran, M., Komasa, A., and Bartoszak-Adamska, E. (2007). Crystal and Molecular Structure of 4-carboxypiperidinium Chloride (4-piperidinecarboxylic Acid Hydrochloride). J. Mol. Struct. 827, 101–107. doi:10.1016/j.molstruc.2006.05.012
Tsutsumi, N., Morishima, M., and Sakai, W. (1998). Nonlinear Optical (NLO) Polymers. 3. NLO Polyimide with Dipole Moments Aligned Transverse to the Imide Linkage. Macromolecules 31, 7764–7769. doi:10.1021/ma9803436
Vural, H., Orbay, M., and Ozdogan, T. J. B. Ö. K. (2017). A Combined Theoretical and Experimental Study of Cu (II) Complex with 2, 6-Pyridinedicarbonyl Dichloride, 132.
Yamashita, S. (2011). A Tutorial on Nonlinear Photonic Applications of Carbon Nanotube and Graphene. J. lightwave Technol. 30, 427–447. doi:10.1109/JLT.2011.2172574
Yanai, T., Tew, D. P., and Handy, N. C. (2004). A New Hybrid Exchange-Correlation Functional Using the Coulomb-Attenuating Method (CAM-B3lyp). Chem. Phys. Lett. 393, 51–57. doi:10.1016/j.cplett.2004.06.011
Keywords: nonlinear optical properties, π-conjugated linkers, density functional theory, metal-free organic dyes, triphenylamine-dicyanovinylene
Citation: Khan MU, Khalid M, Asim S, Momina , Hussain R, Mahmood K, Iqbal J, Akhtar MN, Hussain A, Imran M, Irfan A, Ali A, Rehman MFu, Jiang Y and Lu C (2021) Exploration of Nonlinear Optical Properties of Triphenylamine-Dicyanovinylene Coexisting Donor-π-Acceptor Architecture by the Modification of π-Conjugated Linker. Front. Mater. 8:719971. doi: 10.3389/fmats.2021.719971
Received: 03 June 2021; Accepted: 08 July 2021;
Published: 12 August 2021.
Edited by:
Ashok Kumar, National Physical Laboratory (CSIR), IndiaReviewed by:
Mohammad Chahkandi, Hakim Sabzevari University, IranHossein Asghar Rahnamaye Aliabad, Hakim Sabzevari University, Iran
Copyright © 2021 Khan, Khalid, Asim, Momina, Hussain, Mahmood, Iqbal, Akhtar, Hussain, Imran, Irfan, Ali, Rehman, Jiang and Lu. This is an open-access article distributed under the terms of the Creative Commons Attribution License (CC BY). The use, distribution or reproduction in other forums is permitted, provided the original author(s) and the copyright owner(s) are credited and that the original publication in this journal is cited, in accordance with accepted academic practice. No use, distribution or reproduction is permitted which does not comply with these terms.
*Correspondence: Muhammad Khalid, bXVoYW1tYWQua2hhbGlkQGtmdWVpdC5lZHUucGs=, S2hhbGlkQGlxLnVzcC5icg==; Yang Jiang, eWFuZ2ppYW5nMXN0QHllYWgubmV0; Changrui Lu, Y3JsdUBkaHUuZWR1LmNu