- Department of Physics, Isfahan University of Technology, Isfahan, Iran
M-type strontium hexaferrite (SrM) thin films show excellent magnetic properties and uniaxial magnetic anisotropy. We systematically investigated the magnetism of SrM films prepared by pulsed-laser deposition on different substrates [Al2O3 (
Introduction
M-type hexagonal ferrites are promising materials for various applications, including microwave devices, magnetic field sensors, and data storage (Díaz-Castañón et al., 2001; Chen and Harris, 2012). They have favorable electrical properties (Tang et al., 2016), high chemical stability, low production cost (Zi et al., 2008), and unique magnetic properties such as high magnetization, high values of coercivity, and strong uniaxial magnetic anisotropy (Harris, 2012; Zhang et al., 2014). In hexaferrite thin films, the easy axis of magnetization is usually along the c-axis, and thin films with c-axis orientation find utility in specialized applications (Zhang et al., 2019). Thin films that can be used for microwave filters (Sun et al., 2016), phase shifters (Wu, 2012), and delay lines must be fabricated with in-plane orientation (IPCA). However, thin films used for circulators and isolators must possess an out-of-plane c-axis (OCA) orientation (Özgür et al., 2009; Meng et al., 2012). Strontium M-type hexagonal ferrite (SrM) belongs to the magnetoplumbite phase of ferrites (Pullar, 2012; Jotania, 2014). Researchers have classified hexagonal ferrites according to the location of their constituent subunit blocks. SrM has a hexagonal structure with a space group of P63/mmc and consists of four blocks (RSR*S*), where
Nowadays, investigators strive to determine deposition conditions that lead to improved performance. For example, deposition parameters that have been the foci of optimization studies include the choice of substrate (Hylton et al., 1993), substrate temperature (Xu et al., 2013a; Wei et al., 2020), working gas type and pressure (Masoudpanah et al., 2012), postdeposition annealing (Borisov et al., 2013), laser process conditions (Yu et al., 2020), and film thickness (Sun et al., 2016). Several common deposition techniques are available to obtain hexaferrite films of various crystallographic quality, including sol-gel (Masoudpanah and Seyyed Ebrahimi, 2012), molecular beam epitaxy (MBE) (Liu et al., 2010), liquid phase epitaxy (LPE) (Kranov et al., 2006; Wu et al., 2020), screen printing (Chen et al., 2006), radio frequency (RF) magnetron sputtering (Zhang et al., 2010; Xu et al., 2013a; Patel et al., 2018; Abuzir et al., 2020), direct current (DC) magnetron sputtering (Zhang et al., 2014; Zhang et al., 2019), spin-coating (Meng et al., 2014a; Meng et al., 2014b), and pulsed laser deposition (PLD) (Eason, 2007). The last method has been found to be a more effective technique than other reported methods for the deposition of oxide, nitride, and carbide thin films (Eason, 2007; Wei et al., 2016).
This work systematically investigated the effects of annealing on the structural and magnetic properties of SrM thin films deposited by PLD on various substrates. We discuss our results in terms of the effect of different magnetic anisotropy mechanisms on the structural and magnetic properties of SrM thin films.
Experimental
The films were deposited by PLD onto various single-crystal substrates from a sintered SrFe12O19 target prepared by the solid-state method. A KrF excimer laser producing monochromatic light at a wavelength of 248 nm at 25 ns pulses was used to produce a laser fluence of about 1.5 J/cm2 at the surface of the ceramic target. A pulse repetition rate of 10 Hz was employed. The base pressure in the PLD chamber was 2 × 10−4 Pa, and the substrate temperature was 800°C. After deposition, the films were annealed at 1,000°C in air for 2 hours to further complete the film’s crystallinity.
It is expected that the crystal structure and orientation of the substrate play an important role in determining the texture and properties of the films. Therefore, here we deposited SrM thin films on different substrates: Al2O3 (
Results and Discussions
Figure 2 shows the XRD patterns of films deposited on different substrates before and after heat treatment. This allows for the evaluation of the impact of the annealing treatment on the structural properties of SrM thin films, which indicates annealing can be used to improve crystallinity that may result in improved superior magnetic properties (Zheng et al., 2016). Also, the effect of different substrates is observed. The X-ray patterns and the known JCPDS card (01-080-1197) were compared, and the presence and identification of all diffraction peaks confirmed the hexagonal structure (Chen et al., 2010). The X-ray diffraction pattern of the sample
The XRD patterns of
In-plane lattice parameter a and out-of-plane lattice parameter c of the SrM film were calculated using the formula:
where d is the interplanar distance and h, k, and l are Miller indices. The bulk (target) lattice parameters are a = 5.914 and c = 23.283. We calculated the stain ratio for each film. The results are shown in Table 1. The in-plane and out-of-plane lattice parameters of the films on ALO, STO, and LNO substrates are less than the bulk value, indicating compressive strain. On the other hand, the films on the ZNO substrate are under tensile strain (Malek et al., 2015). As can be seen from Table 1, the strain increased after annealing.
In our work, we used Raman spectra to investigate the effect of strain on thin films after annealing (Wang et al., 2004) (Figure 3). In Raman spectroscopy, the incident phonons either gain quanta or lose quanta by interacting with the vibrational modes of the material. If it gains energy, it gets blue-shifted, and if it loses, it is red-shifted. The amount of the shift determines the energy of the phonon in the material. Raman spectroscopy is a powerful tool for ascertaining lattice strain (Lisfi and Williams, 2003). If the material lattice experiences compressive strain, the Raman shift increases; if it is under tensile strain, the Raman shift decreases. These shifts are called blue shift and red shift, respectively. Since the lattice constants of SrFe12O19 are larger than the AlO substrate, the strain created in the film should be compressive. The Raman spectra of the thin films (shown in Figure 3A) deposited on Al2O3 show a substantial peak at 672
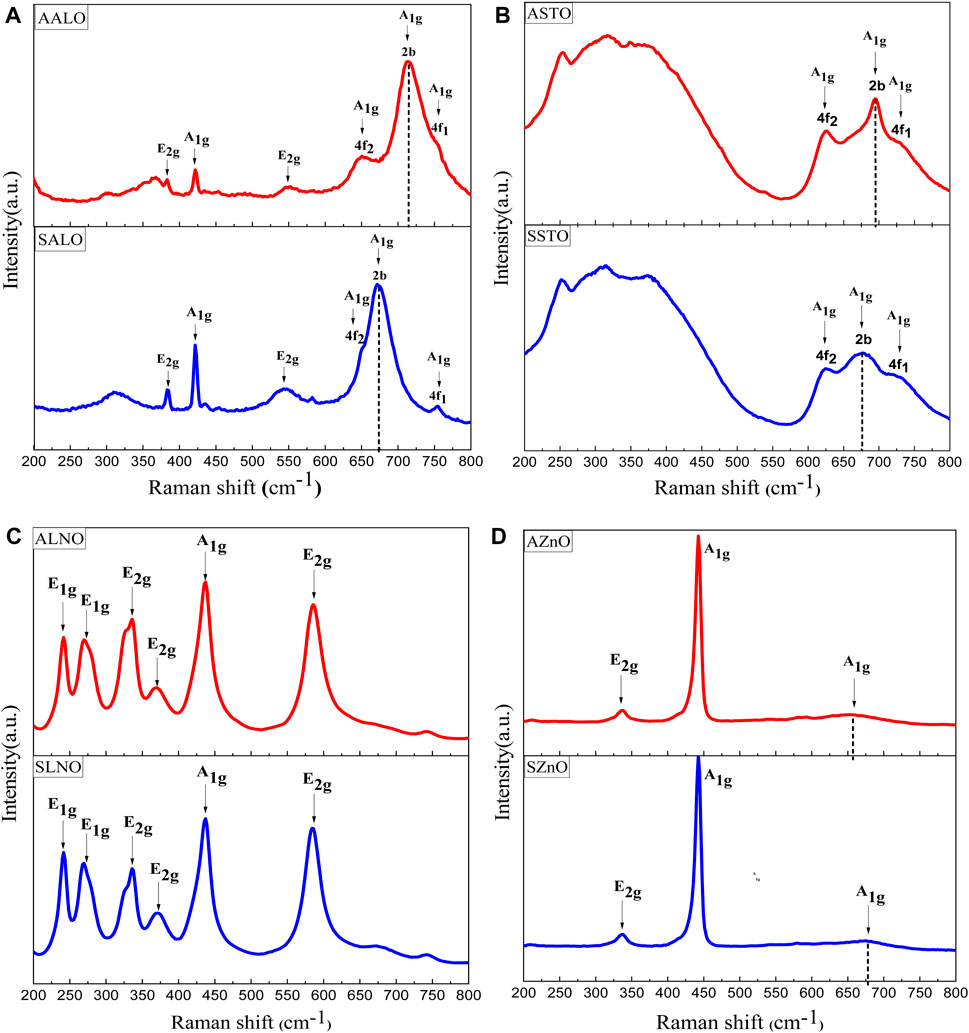
FIGURE 3. Raman spectra of SrFe12O19 thin films before (blue line) and after (red line) annealing on different substrates show (A) Al2O3 (
Figure 4 shows SEM micrographs of the surface morphology of as-deposited thin films and the films annealed at 1,000°C. It is known that ferrite microstructure depends on various parameters such as annealing temperature and time, substrate type, and deposition temperature. The SEM images illustrate an increase in the average grain sizes upon annealing. It has been shown by others that strontium ferrite grains often appear acicular-like or platelet-like (Xu et al., 2013b). According to the literature, we can determine the orientation of the c-axis from the shape and alignment of grains in SEM images. Platelet-like grains tend to have an out-of-plane orientation of the c-axis, while acicular-like grains have either an in-plane or a random orientation of the c-axis (Meng et al., 2014b). Therefore, nearly all the samples studied here have an out-of-plane orientation except for two samples
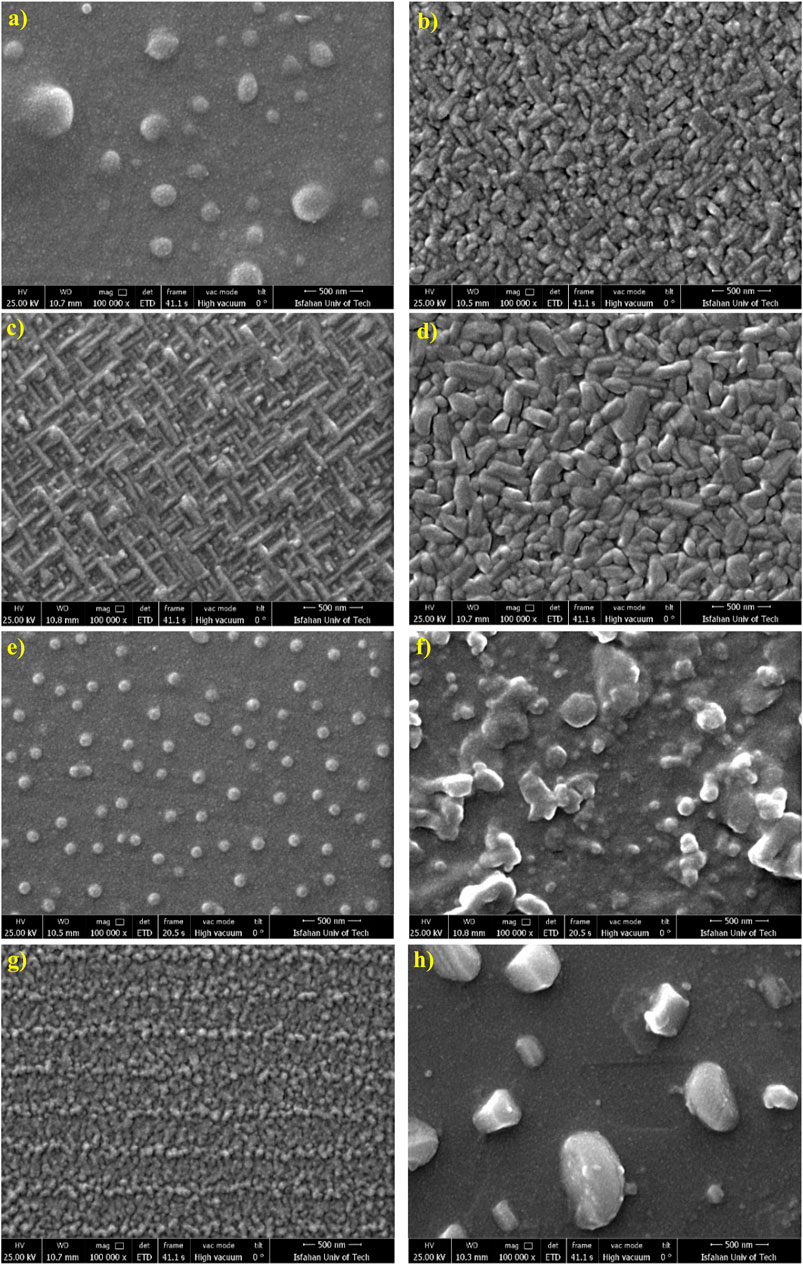
FIGURE 4. SEM images of films. (A) Surface of film SALO, (B) surface of film AALO, (C) surface of film SSTO, (D) surface of film ASTO, (E) surface of film SZnO, (F) surface of film AZnO, (G) surface of film SLNO, and (H) surface of film ALNO.
The magnetic properties of the strontium ferrite thin films were measured by a superconducting quantum interference device (SQUID) magnetometer. Magnetic hysteresis loops were measured with magnetic fields applied along the perpendicular and the in-plane directions to the films at room temperature ( Figure 5H). The normalized hysteresis curves are shown in Figure 5. The magnetic parameters such as saturation magnetization (Ms), remanent magnetization (Mr), and coercivity (Hc) were determined from the M-H loops and are tabulated in Table 2. It was found that
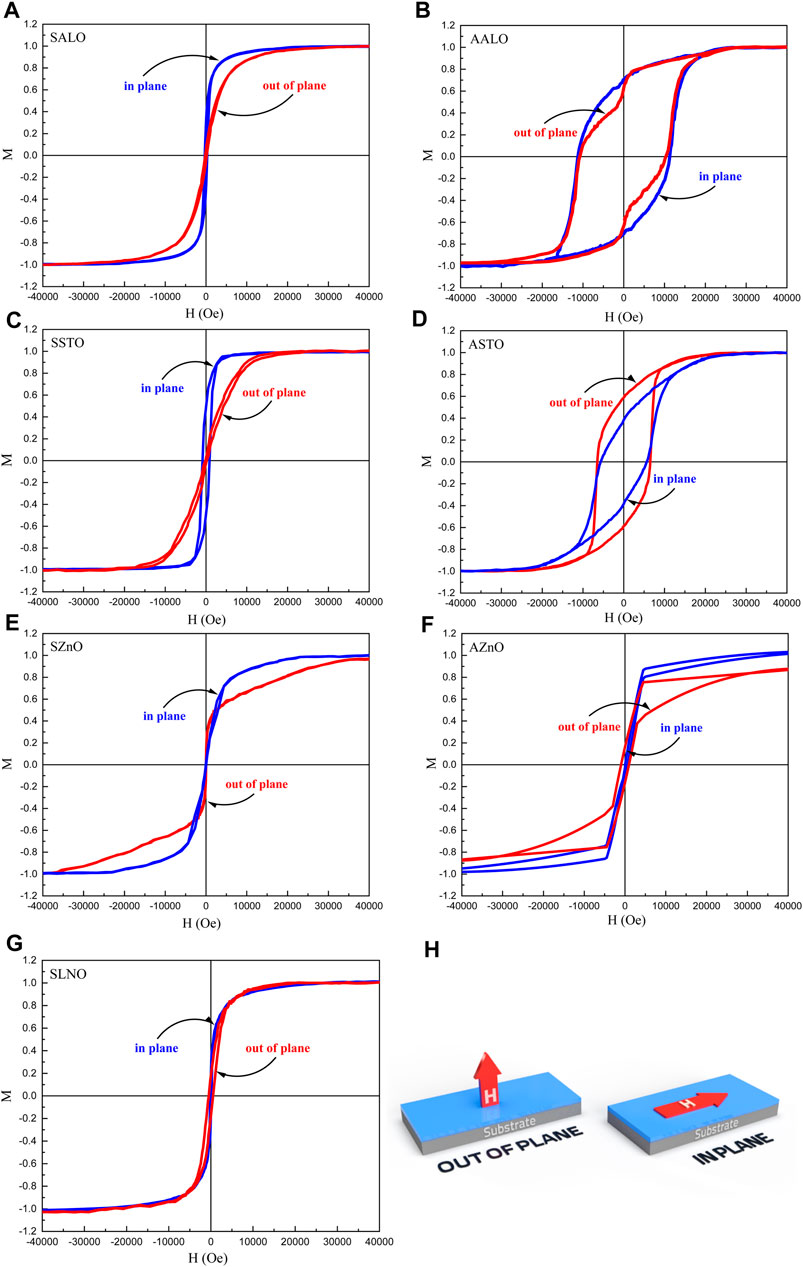
FIGURE 5. Normalized magnetic hysteresis loops of films with various substrates, before annealing: (A) SALO, (C) SSTO, (E) SZnO, and (G) SLNO, and after annealing: (B) AALO, (D) ASTO, and (F) AZnO. (H) Schematic of the applied magnetic field direction during the M–H measurements of the SrM film.
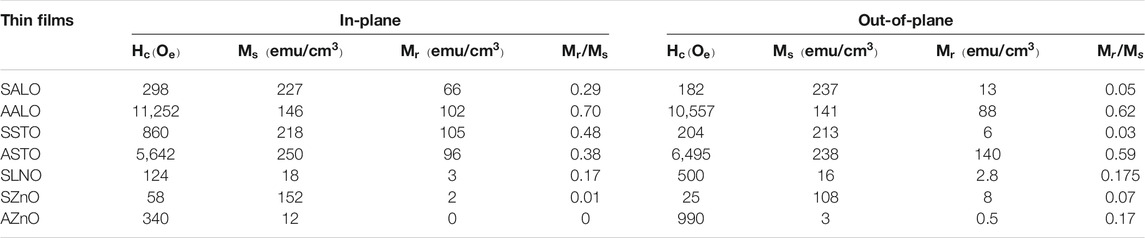
TABLE 2. Magnetic characteristics of the SrM films grown on different substrates in an applied magnetic field, parallel to (in-plane) or perpendicular to (out-of-plane) the c-axis of SrM.
The
The
Conclusion
M-type strontium hexaferrite (SrM) thin films prepared by pulsed laser deposition on Al2O3 (
Data Availability Statement
The original contributions presented in the study are included in the article/Supplementary Material. Further inquiries can be directed to the corresponding author.
Author Contributions
MK fabricated the samples and carried out the experiment. MK wrote the draft of the manuscript. PK conceived the original idea, supervised the project, and revised the manuscript.
Conflict of Interest
The authors declare that the research was conducted in the absence of any commercial or financial relationships that could be construed as a potential conflict of interest.
Publisher’s Note
All claims expressed in this article are solely those of the authors and do not necessarily represent those of their affiliated organizations, or those of the publisher, the editors and the reviewers. Any product that may be evaluated in this article, or claim that may be made by its manufacturer, is not guaranteed or endorsed by the publisher.
References
Abuzir, A. R., Salman, S. A., and Mazher, J. (2020). Magnetron Sputtered Perpendicular Barium Hexaferrite Thin Films Produced by the Multilayered Method. J. Supercond. Nov. Magn. 33 (12), 3819–3825. doi:10.1007/s10948-020-05647-3
Borisov, P., Alaria, J., Yang, T., McMitchell, S. R. C., and Rosseinsky, M. J. (2013). Growth of M-type Hexaferrite Thin Films with Conical Magnetic Structure. Appl. Phys. Lett. 102, 032902. doi:10.1063/1.4776223
Chen, Z., and Harris, V. G. (2012). Ferrite Film Growth on Semiconductor Substrates towards Microwave and Millimeter Wave Integrated Circuits. J. Appl. Phys. 112 (8), 081101. doi:10.1063/1.4739219
Chen, Y., Geiler, A. L., Sakai, T., Yoon, S. D., Vittoria, C., and Harris, V. G. (2006). Microwave and Magnetic Properties of Self-Biased Barium Hexaferrite Screen Printed Thick Films. J. Appl. Phys. 99 (8), 08M904–4. doi:10.1063/1.2163288
Chen, Z., Yang, A., Mahalingam, K., Averett, K. L., Gao, J., Brown, G. J., et al. (2010). Structure, Magnetic, and Microwave Properties of Thick Ba-Hexaferrite Films Epitaxially Grown on GaN/Al2O3 Substrates. Appl. Phys. Lett. 96 (24), 242502–242504. doi:10.1063/1.3446867
Chen, D.-M., Li, Y.-X., Han, L.-K., Long, C., and Zhang, H.-W. (2016). Perpendicularly Oriented Barium Ferrite Thin Films with Low Microwave Loss, Prepared by Pulsed Laser Deposition. Chin. Phys. B 25, 068403–068406. doi:10.1088/1674-1056/25/6/068403
Chen, D., Chen, Z., Wang, G., Chen, Y., Li, Y., and Liu, Y. (2017). Effect of Al on the Microstructure, Magnetic and Millimeter-Wave Properties of High Oriented Barium Hexaferrite Thin Films. J. Magnetism Magn. Mater. 444, 7–11. doi:10.1016/j.jmmm.2017.07.090
Díaz-Castañón, S., Leccabue, F., Watts, B. E., Yapp, R., Asenjo, A., and Vázquez, M. (2001). Oriented PbFe12O19 Thin Films Prepared by Pulsed Laser Deposition on Sapphire Substrate. Mater. Lett. 47 (6), 356–361. doi:10.1016/S0167-577X(00)00266-4
Eason, R. (2007). Pulsed Laser Deposition of Thin Films: Applications-Led Growth of Functional Materials. Southampton, United Kingdom: John Wiley & Sons.
Gao, Q., Hong, G., Ni, J., Wang, W., Tang, J., and He, J. (2009). Uniaxial Anisotropy and Novel Magnetic Behaviors of CoFe2O4 Nanoparticles Prepared in a Magnetic Field. J. Appl. Phys. 105 (7), 07A516. doi:10.1063/1.3072019
Harris, V. G. (2012). Modern Microwave Ferrites. IEEE Trans. Magn. 48 (3), 1075–1104. doi:10.1109/TMAG.2011.2180732
Hylton, T. L., Parker, M. A., Coffey, K. R., and Howard, J. K. (1993). Properties of Epitaxial Ba‐hexaferrite Thin Films on A‐, R‐, and C‐plane Oriented Sapphire Substrates. J. Appl. Phys. 73, 6257–6259. doi:10.1063/1.354065
Izadkhah, H., Zare, S., Somu, S., Lombardi, F., and Vittoria, C. (2017). Utilizing Alternate Target Deposition to Increase the Magnetoelectric Effect at Room Temperature in a Single Phase M-type Hexaferrite. MRS Commun. 7 (2), 97–101. doi:10.1557/mrc.2017.36
Jotania, R. (2012). Crystal Structure, Magnetic Properties and Advances in Hexaferrites: A Brief Review. AIP Conf. Proc. 1621, 596–599. doi:10.1063/1.4898528
Kaur, B., Bhat, M., Licci, F., Kumar, R., Kulkarni, S. D., Joy, P. A., et al. (2006). Modifications in Magnetic Anisotropy of M-type Strontium Hexaferrite Crystals by swift Heavy Ion Irradiation. J. Magn. Magn. Mater. 305 (2), 392–402. doi:10.1016/j.jmmm.2006.01.110
Kimura, T. (2012). Magnetoelectric Hexaferrites. Annu. Rev. Condens. Matter Phys. 3 (1), 93–110. doi:10.1146/annurev-conmatphys-020911-125101
Kranov, Y. A., Abuzir, A., Prakash, T., McIlroy, D. N., and Yeh, W. J. (2006). Barium Hexaferrite Thick Films Made by Liquid Phase Epitaxy Reflow Method. IEEE Trans. Magn. 42 (10), 3338–3340. doi:10.1109/TMAG.2006.879629
Kreisel, J., Pignard, S., Vincent, H., Sénateur, J. P., and Lucazeau, G. (1998). Raman Study of BaFe12O19 Thin Films. Appl. Phys. Lett. 73, 1194–1196. doi:10.1063/1.122124
Lavorato, G., and Winkler, E. (2016). Thickness Dependence of Exchange Coupling in Epitaxial. Phys. Rev. B. 94, 054405. doi:10.1103/PhysRevB.94.054405
Lisfi, A., and Williams, C. M. (2003). Magnetic Anisotropy and Domain Structure in Epitaxial CoFe2O4 Thin Films. J. Appl. Phys. 93 (10), 8143–8145. doi:10.1063/1.1541651
Liu, H., Avrutin, V., Xiao, B., Rowe, E., Liu, H. R., Özgür, Ü., et al. (2010). Epitaxial Relationship of MBE Grown Barium Hexaferrite (0001) Films on Sapphire (0001). J. Cryst. Growth 312 (5), 671–675. doi:10.1016/j.jcrysgro.2009.12.013
Malek, M. F., Mamat, M. H., Musa, M. Z., Soga, T., Rahman, S. A., Alrokayan, S. A. H., et al. (2015). Metamorphosis of Strain/stress on Optical Band gap Energy of ZAO Thin Films via Manipulation of thermal Annealing Process. J. Lumin. 160, 165–175. doi:10.1016/j.jlumin.2014.12.003
Masoudpanah, S. M., and Seyyed Ebrahimi, S. A. (2012). Synthesis and Characterization of Nanostructured Strontium Hexaferrite Thin Films by the Sol-Gel Method. J. Magn. Magn. Mater. 324 (14), 2239–2244. doi:10.1016/j.jmmm.2012.02.109
Masoudpanah, S. M., Seyyed Ebrahimi, S. A., and Ong, C. K. (2012). Effect of Oxygen Pressure on Microstructure and Magnetic Properties of Strontium Hexaferrite (SrFe12O19) Film Prepared by Pulsed Laser Deposition. J. Magn. Magn. Mater. 324 (7), 1440–1443. doi:10.1016/j.jmmm.2011.12.004
Meng, S., Yue, Z., and Li, L. (2012). In-plane C-axis Oriented Barium Hexaferrite Films Prepared by Magnetron Sputtering. Mater. Lett. 86, 92–95. doi:10.1016/j.matlet.2012.07.023
Meng, S., Yue, Z., Zhang, X., and Li, L. (2014). Quasi-epitaxial Barium Hexaferrite Thin Films Prepared by a Topotactic Reactive Diffusion Process. Appl. Surf. Sci. 290, 340–345. doi:10.1016/j.apsusc.2013.11.079
Meng, S., Yue, Z., and Li, L. (2014). Effect of Ethylene Glycol on the Orientation and Magnetic Properties of Barium Ferrite Thin Films Derived by Chemical Solution Deposition. J. Magn. Magn. Mater. 354, 290–294. doi:10.1016/j.jmmm.2013.11.016
Özgür, Ü., Alivov, Y., and Morkoç, H. (2009). Microwave Ferrites, Part 1: Fundamental Properties. J. Mater. Sci.: Mater. Electroni. 20 (9), 789–834. doi:10.1007/s10854-009-9923-2
Patel, R., Ikeda, Y., Onoda, H., Tainosho, T., Hisamatsu, Y., Sharmin, S., et al. (2018). Magnetic Properties of Epitaxial Barium Hexaferrite (0001) Thin Films Deposited by Radio Frequency Magnetron Sputtering. IEEE Trans. Magn. 54 (2), 1–4. doi:10.1109/TMAG.2017.2756687
Pullar, R. C. (2012). Hexagonal Ferrites: A Review of the Synthesis, Properties and Applications of Hexaferrite Ceramics. Prog. Mater. Sci. 57 (7), 1191–1334. Sep. 2012. doi:10.1016/J.PMATSCI.2012.04.001
Sun, K., Li, Q., Guo, H., Yang, Y., Yu, Z., Xu, Z., et al. (2016). Magnetic Property and Stress Study of Barium Hexaferrite Thin Films with Different Structures. J. Alloys Compd. 663, 645–650. doi:10.1016/j.jallcom.2015.12.193
Tang, R., Zhou, H., Zhao, R., Jian, J., Wang, H., Huang, J., et al. (2016). Dielectric Relaxation and Polaronic Conduction in Epitaxial BaFe12O19hexaferrite Thin Film. J. Phys. D: Appl. Phys. 49, 115305–115311. doi:10.1088/0022-3727/49/11/115305
Wang, Y. C., Ding, J., Yi, J. B., Liu, B. H., Yu, T., and Shen, Z. X. (2004). High-Coercivity Co-ferrite Thin Films on (100)-SiO2 Substrate. Appl. Phys. Lett. 84 (14), 2596–2598. Apr. 2004. doi:10.1063/1.1695438
Wei, G., Wei, L., Chen, Y., Yan, S., Mei, L., and Jiao, J. (2016). Self-assembled Epitaxial BaFe12O19 Nano-Island Film Grown on Al2O3 Substrate by Pulsed Laser Deposition. Mater. Lett. 181, 212–215. doi:10.1016/j.matlet.2016.06.006
Wei, X., Zheng, H., Chen, W., Wu, Q., Zheng, P., Zheng, L., et al. (2020). Crystal Structure, Morphology and Magnetic Properties of Hexagonal M-type Barium Ferrite Film Based on the Substrate Temperature. Chem. Phys. Lett. 752, 137541. doi:10.1016/j.cplett.2020.137541
Wu, Y., Yang, Q., Zhang, D., Zhang, Y., Rao, Y., Wen, Q., et al. (2020). The Submicron Garnet Film with Perpendicular Magnetic Anisotropy Prepared by Liquid Phase Epitaxy Method. J. Magn. Magn. Mater. 506, 166689. doi:10.1016/j.jmmm.2020.166689
Xu, Z., Lan, Z., Sun, K., Yu, Z., Guo, R., Zhu, G., et al. (2013). Deposition of Perpendicular C -axis Oriented BaM Thin Films on (001) Al 2 O 3 Substrates by Introducing an Interfacial BaM Buffer Layer. J. Magn. Magn. Mater. 345, 72–76. doi:10.1016/j.jmmm.2013.06.018
Xu, Z. Y., Lan, Z. W., Sun, K., Yu, Z., Guo, R. D., Jiang, X. N., et al. (2013). Properties of Ba-Hexaferrite Thin Films with Different Layer Structures. Amr 774-776, 935–939. doi:10.4028/www.scientific.net/AMR.774-776.935
Yu, C., Sokolov, A. S., Kulik, P., and Harris, V. G. (2020). Stoichiometry, Phase, and Texture Evolution in PLD-Grown Hexagonal Barium Ferrite Films as a Function of Laser Process Parameters. J. Alloys Compd. 814, 152301. doi:10.1016/j.jallcom.2019.152301
Zhang, L., Su, X. D., Chen, Y., Li, Q. F., and Harris, V. G. (2010). Radio-frequency Magnetron Sputter-Deposited Barium Hexaferrite Films on Pt-Coated Si Substrates Suitable for Microwave Applications. Scr. Mater. 63 (5), 492–495. doi:10.1016/j.scriptamat.2010.05.013
Zhang, X., Yue, Z., Meng, S., and Yuan, L. (2014). Magnetic Properties of In-Plane Oriented Barium Hexaferrite Thin Films Prepared by Direct Current Magnetron Sputtering. J. Appl. Phys. 116, 243909–243924. doi:10.1063/1.4905028
Zhang, X., Meng, S., Song, D., Zhang, Y., Yue, Z., and Harris, V. G. (2017). Epitaxially Grown BaM Hexaferrite Films Having Uniaxial axis in the Film Plane for Self-Biased Devices. Sci. Rep. 7, 44193. doi:10.1038/srep44193
Zhang, X., Zhang, Y., Cao, S., Yue, Z., and Zhang, J. (2019). BaFe12O19 Films Prepared on Al2O3 (0 0 0 1) by Direct Current Magnetron Sputtering. Mater. Lett. 248, 24–27. doi:10.1016/j.matlet.2019.03.139
Zheng, H., Han, M., Zheng, L., Deng, J., Zheng, P., Wu, Q., et al. (2016). Magnetic Properties of Hexagonal Barium Ferrite Films on Pt/MgO(111) Substrates Annealed at Different Temperatures. J. Magn. Magn. Mater. 413 (111), 25–29. doi:10.1016/j.jmmm.2016.04.010
Keywords: hexaferrite, thin film, annealing, coercivity, magnetization
Citation: Khojaste khoo M and Kameli P (2021) Structure and Magnetization of Strontium Hexaferrite (SrFe12O19) Films Prepared by Pulsed Laser Deposition. Front. Mater. 8:717251. doi: 10.3389/fmats.2021.717251
Received: 30 May 2021; Accepted: 17 August 2021;
Published: 06 October 2021.
Edited by:
Ahmad Gholizadeh, Damghan University, IranReviewed by:
Vincent Harris, Northeastern University, United StatesManish Kumar, Pohang University of Science and Technology, South Korea
Copyright © 2021 Khojaste khoo and Kameli. This is an open-access article distributed under the terms of the Creative Commons Attribution License (CC BY). The use, distribution or reproduction in other forums is permitted, provided the original author(s) and the copyright owner(s) are credited and that the original publication in this journal is cited, in accordance with accepted academic practice. No use, distribution or reproduction is permitted which does not comply with these terms.
*Correspondence: P. Kameli, a2FtZWxpQGNjLml1dC5hYy5pcg==