- 1Microelectronics Research Unit, Faculty of Information Technology and Electrical Engineering, University of Oulu, Oulu, Finland
- 2Department of Hybrid Microsystems, Mikrosysteme, LTCC und HTCC, Fraunhofer IKTS, Dresden, Germany
- 3National Institute for Interdisciplinary Science and Technology (CSIR), Thiruvananthapuram, India
Editorial on the Research Topic
Dielectric Ceramics for Electronic Applications
Introduction
Electroceramics span paraelectrics and polar dielectrics such as ferroelectrics and piezoelectrics (Damjanovic, 1998; Sebastian et al., 2015). Due to their versatile properties, dielectric ceramics are widely used in telecommunication (e.g., antenna), medical (e.g., sensors and transducers), aerospace (e.g., structural dielectrics) industries, and in almost all electronics (e.g., active and passive components) (Nomura et al., 1994; Abrar et al., 2004; Reaney and Iddles, 2006; Kandi et al., 2015). The compositions and processing methods fundamentally affect the performances of dielectric ceramics. For instance, the ceramic compound determines the dielectric, thermal and mechanical properties (Sebastian et al., 2015). The processing method used to fabricate the ceramics significantly influence the microstructure and thus ferroelectric and piezoelectric properties (Wang et al., 2018). The shaping procedure and sintering temperature also define the applicability, integration capabilities with other components, and cost of the ceramics. This research topic aims to update the research community in terms of the recent advancement of compositional and processing investigations, as well as their influence on relevant properties. In addition, it provides perspectives beyond conventional applications.
Compositional Optimization
Wave-transparent ceramics are found significant for the development of space vehicles. Silicon nitride-based ceramics yield favored mechanical and dielectric properties for such an application (Kandi et al., 2015). In this research topic, Chen et al. studied the influence of Si3N4w volume fraction on the microstructure and properties of Si3N4w/Si3N4 ceramic composites. In this work, the researchers prepared the composites via the gel-casting process in combination with the chemical vapor infiltration (CVI). The results show that the Si3N4w/Si3N4 ceramic composites obtained good dielectric properties (dielectric constant 2-4, dielectric loss < 0.005). The composites with 25 vol% Si3N4w exhibited better flexural strength of up to 41.37 MPa compared to counterparts.
Barium titanate has excellent energy storage properties suitable for capacitors, so do its doped compositions (Li et al., 2017). In this research topic, Kavil et al. reported the dielectric properties of a family member of barium titanate, BaZr0.2[Ti(1-x)Mgx/3Ta2x/3]0.8O3 (BZTMT). The ceramics made via the solid-state route showed a considerably large tunability of relative permittivity and dielectric loss (at 1 MHz), ranging from 5,205 to 24 and from 1.8 × 10–2 to 2.0 × 10–5, respectively, with the x value changing from 0 to 1. This was attributed to the shift of the Curie temperature and such a performance may find appropriate applications in filters and resonators.
Ultra-Low Temperature Fabrication
In recent years, room-temperature densification and cold sintering have been developed to enable the fabrication of electroceramics at ultra-low temperatures (Kähäri et al., 2015; Guo et al., 2019). The advantages of ultra-low temperature (<350°C) fabrications of dielectric ceramics over the conventional high-temperature (usually >1,000°C) sintering include: 1) Significant cost and emission reduction, 2) precise shape control during densification, and 3) improvement of compatibility with other components (e.g., those made from semiconductors, metals and polymers) for co-fabrication of multi-component structures (Guo et al., 2019). In the above ultra-low temperature fabrication methods, a small amount of certain liquid as a transport phase is mixed with the ceramic particles as a body phase to form a solid-liquid core-shell microstructure (Guo et al., 2019; Nelo et al., 2019). The mixture is subject to hundreds of MPa, including ultra-high pressure (>GPa) at contacting points between the particles, and drives grain growth and densification thanks to the lowered activation energy at the transport interfaces.
The room-temperature densification method has been successfully adopted to several compositions to date. Figure 1 schematically explains the microstructure of a room-temperature densified ceramic in which the ceramic body (e.g., BST (Ba0.55Sr0.45)TiO3) form grains and the transport phase (e.g., LMO (Li2MoO4)) form grain boundary areas after stimulating mass transfer between grains/particles at room temperature (Nelo et al., 2019). Such a ceramic-ceramic composite structure is named “upside-down composite”.
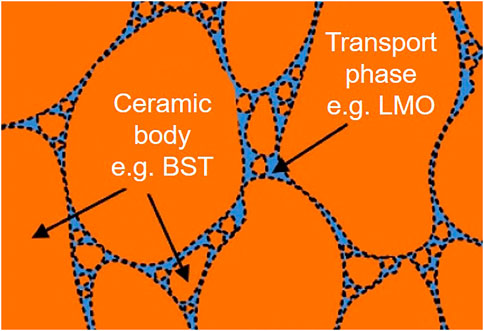
FIGURE 1. Schematic microstructure of a BST-LMO upside-down composite densified at room temperature. Reproduced with permission (Nelo et al., 2019). Open access under the CC BY-NC-ND license.
In this research topic, Kuzmic et al. applied this method to the SrTiO3 (ST)-LMO upside-down composites. As a result of the sufficient binding provided by the LMO, relative densities of 76–84% were achieved for the ST-LMO ceramics. Factors affecting the microstructure, such as particle size distribution, pressure, pressing time, ultrasonic treatment, drying time and other processing conditions, were studied and optimized in order to improve the functional properties. Relative permittivity of 65–78 and dielectric loss of 0.002–0.05 that can be useful in electroceramics industry were obtained.
Cold sintering is an alternative to the method of room-temperature densification for dielectric ceramics (Guo et al., 2019). By slightly increasing the fabrication temperature, the densification level may be further improved. In this research topic, Madhuri et al. (2021) used the cold sintering method to fabricate the (1-x)NaCa2Mg2V3O12-xNaCl ceramics. Relative densities of 80–94% were achieved under the densification conditions of 200°C and 450 MPa. The relative permittivity of ∼7 and a reasonably high microwave quality factor of 5,000–13,830 GHz were obtained with the x values of 0.4–0.7.
Photoresponsive Piezoelectrics
Dielectric ceramics showing ferroelectric and piezoelectric properties broaden the applications toward sensors, actuators, transducers and energy harvesters that offer the functions of electromechanical and electro-thermal coupling. This is a cornerstone of modern electronics. Most conventional piezoelectric materials do not respond to visible light due to their large optical band gaps. Reducing the band gap while maintaining the excellent piezoelectric properties has become a future direction in the research community. The newly developed photoresponsive piezoelectrics exhibiting electro-optical coupling functions in addition to the electromechanical and electro-thermal ones can be potentially used in opto-electrical dual-source actuators, single-material multi-sensors and multi-source energy harvesters. In this research topic, Bai summarized the recent development of narrow band gap piezoelectrics and provided the perspectives of this emerging field in a mini-review.
In summary, with the articles published in this research topic, the readers will understand some new research trends in dielectric and piezoelectric ceramics that may result in industrial advancement and renewal of electroceramics and electronics.
Author Contributions
All authors listed have made a substantial, direct, and intellectual contribution to the work and approved it for publication.
Conflict of Interest
The authors declare that the research was conducted in the absence of any commercial or financial relationships that could be construed as a potential conflict of interest.
References
Abrar, A., Zhang, D., Su, B., Button, T. W., Kirk, K. J., and Cochran, S. (2004). 1–3 Connectivity Piezoelectric Ceramic–Polymer Composite Transducers Made with Viscous Polymer Processing for High Frequency Ultrasound. Ultrasonics 42, 479–484. doi:10.1016/j.ultras.2004.02.008 |
Damjanovic, D. (1998). Ferroelectric, Dielectric and Piezoelectric Properties of Ferroelectric Thin Films and Ceramics. Rep. Prog. Phys. 61, 1267–1324. doi:10.1088/0034-4885/61/9/002
Guo, J., Floyd, R., Lowum, S., Maria, J.-P., Herisson de Beauvoir, T., Seo, J.-H., et al. (2019). Cold Sintering: Progress, Challenges, and Future Opportunities. Annu. Rev. Mater. Res. 49, 275–295. doi:10.1146/annurev-matsci-070218-010041
Kähäri, H., Teirikangas, M., Juuti, J., and Jantunen, H. (2015). Improvements and Modifications to Room-Temperature Fabrication Method for Dielectric Li2MoO4 Ceramics. J. Am. Ceram. Soc. 98, 687–689. doi:10.1111/jace.13471
Kandi, K. K., Thallapalli, N., and Chilakalapalli, S. P. R. (2015). Development of Silicon Nitride-Based Ceramic Radomes — A Review. Int. J. Appl. Ceram. Technol. 12, 909–920. doi:10.1111/ijac.12305
Li, W.-B., Zhou, D., and Pang, L.-X. (2017). Enhanced Energy Storage Density by Inducing Defect Dipoles in lead Free Relaxor Ferroelectric BaTiO3-Based Ceramics. Appl. Phys. Lett. 110, 132902. doi:10.1063/1.4979467
Nelo, M., Peräntie, J., Siponkoski, T., Juuti, J., and Jantunen, H. (2019). Upside-down Composites: Electroceramics without Sintering. Appl. Mater. Today 15, 83–86. doi:10.1016/j.apmt.2018.12.021
Nomura, T., Nakano, Y., Satoh, A., and Arashi, T. (1994). Multilayer Ceramic Chip Capacitor. Patent, US-5335139-A
Reaney, I. M., and Iddles, D. (2006). Microwave Dielectric Ceramics for Resonators and Filters in Mobile Phone Networks. J. Am. Ceram. Soc. 89, 2063–2072. doi:10.1111/j.1551-2916.2006.01025.x
Sebastian, M. T., Ubic, R., and Jantunen, H. (2015). Low-loss Dielectric Ceramic Materials and Their Properties. Int. Mater. Rev. 60, 392–412. doi:10.1179/1743280415Y.0000000007
Keywords: dielectric, piezoelectric, cold sintering, upside-down composite, room-temperature densification, photo-piezoelectric
Citation: Bai Y, Varghese J and Sebastian MT (2021) Editorial: Dielectric Ceramics for Electronic Applications. Front. Mater. 8:714522. doi: 10.3389/fmats.2021.714522
Received: 25 May 2021; Accepted: 28 May 2021;
Published: 09 June 2021.
Edited and reviewed by:
Valeria Cannillo, University of Modena and Reggio Emilia, ItalyCopyright © 2021 Bai, Varghese and Sebastian. This is an open-access article distributed under the terms of the Creative Commons Attribution License (CC BY). The use, distribution or reproduction in other forums is permitted, provided the original author(s) and the copyright owner(s) are credited and that the original publication in this journal is cited, in accordance with accepted academic practice. No use, distribution or reproduction is permitted which does not comply with these terms.
*Correspondence: Yang Bai, yang.bai@oulu.fi