- 1Electronic Materials Research Laboratory, Key Laboratory of the Ministry of Education and International Center for Dielectric Research, School of Electronic Science and Engineering, Xi’an Jiaotong University, Xi’an, China
- 2Department of Intelligent Systems Design Engineering, Faculty of Engineering, Toyama Prefectural University, Imizu, Japan
- 3Guangdong JC Technological Innovation Electronics Co., Ltd, Zhaoqing, China
Environment-friendly lead-free piezoelectric ceramics with great properties and high thermal stability are desired in the industry. In this work, the Sm3+-modified lead-free 0.915(K0.45Na0.5Li0.05)NbO3–0.075BaZrO3–0.01(Bi0.5Na0.5)TiO3 (KNLN-BZ-BNT) ceramics are prepared. The piezoelectric properties are improved with the introduction of Sm3+, and the optimal properties (d33 = 325 pC/N and d33* = 384 pm/V) are achieved in the ceramic modified with 0.3 mol% Sm3+ ions. Meanwhile, this sample shows good thermal stability such that the values of d33* decreased less than 20% when the temperature raised from 30 to 180oC. These results show the Sm3+-modified KNLN-BZ-BNT ceramics are good for further applications even under high temperature.
Introduction
In recent years, environmental problems are a major concern in the whole world (Zhang et al., 2017; Zeng et al., 2020; Zheng et al., 2021). Lead is widely used in various industrial products such as glasses, gasoline, and batteries (Uchino, 1996; Shung, 2015; Wu et al., 2015). Ceramics based on lead zirconate titanate [Pb(Zr, Ti)O3 (PZT)], the most widely used piezoelectric material, also contain PbO more than 60% (Saito et al., 2004; Jiang et al., 2016). The Restriction of Hazardous Substances Directive (RoHS) has been implemented to minimize the use of toxic materials in the end products (Saito et al., 2004; Zhang et al., 2007). Consequently, researches on lead-free piezoelectric materials are highly needed now (Saito et al., 2004; Zhao et al., 2017; Zhao et al., 2019). Among several lead-free piezoelectric materials, such as BaTiO3-, (Bi, Na)TiO3-, and BiFeO3-based piezoelectric materials, the (K, Na)NbO3 (KNN)-based piezoelectric materials, with high Curie temperature and high piezoelectric properties, are considered a potential candidate for PZT-based materials in the industry (Shrout and Zhang, 2007; Zhang et al., 2007).
The morphotropic phase boundary (MPB) between the rhombohedral phase and the tetragonal phase contributes to the excellent performance of PZT-based materials (Shrout and Zhang, 2007; Zhang et al., 2007). Furthermore, as to the vertical MPB, the PZT and PZT-based ceramics show excellent thermal stability. For the lead-free piezoelectric KNN ceramics, a phase boundary between the orthorhombic phase and the tetragonal phase called the polymorphic phase transition appeared at about 200oC (Egerton and Dillon, 1959; Karaki et al., 2013; Wang et al., 2013). By decreasing the temperature to near room temperature, the electrical properties, especially the piezoelectric property, were dramatically enhanced. Unfortunately, the high piezoelectric coefficients only remain stable in a narrow temperature range (Karaki et al., 2013).
To solve this problem, Karaki et al. constructed an MPB of rhombohedral and tetragonal phases by the introduction of BaZrO3 (Karaki et al., 2013). The piezoelectric properties were expectedly improved. Meanwhile, by introducing Bi0.5Na0.5TiO3 in an appropriate amount, a vertical MPB has appeared in 0.915(K0.45Na0.5Li0.05)NbO3–0.075BaZrO3–0.01(Bi0.5Na0.5)TiO3 (KNLN-BZ-BNT) ceramics, and excellent thermal stability was manifested. In this work, to further improve the properties of KNLN-BZ-BNT ceramics, the doped ceramics with 0.1, 0.3, and 0.5 mol% Sm3+ ions are prepared. The crystalline phase, micro-morphologies, electrical properties, and thermal stability are investigated. For comparison, the KNLN-BZ-BNT ceramics in our previous work are discussed together (Quan et al., 2018; Quan et al., 2019; Quan et al., 2020).
Experimental Details
0.915(K0.45Na0.5Li0.05)NbO3–0.075BaZrO3–0.01(Bi0.5-xSmxNa0.5)TiO3, where x = 0.1, 0.3, 0.5, ceramics were synthesized by a solid oxide reaction process. Reagent-grade oxide/carbonate powders, K2CO3 (99%), Na2CO3 (99.8%), Li2CO3 (98%), Nb2O5 (99.5%), BaCO3 (99%), ZrO2 (99%), Bi2O3 (99%), TiO2 (98%), and Sm2O3 (99%), were selected as starting raw materials. The powders were weighed according to stoichiometry and mixed through ball milling, with partially stabilized ZrO2 balls as media, in alcohol for 15 h at 300 rpm. After drying at 80oC, the powder mixtures were calcined at 800oC for 2 h. The calcined powders were re-milled for 15 h and then pressed into disks of 8 mm diameter and 1 mm thickness at 200 MPa. The green disks were heated at 600oC for 2 h to remove the organics and then sintered at 1,200 oC for 4 h in a sealed alumina curable. To minimize the volatilization of volatile elements, the green compacts were embedded in the calcined powders during sintering. The final pellets were polished and coated with silver paste on both sides, to characterize the electrical properties.
The crystalline phase structure was evaluated using an X-ray diffractometer (D/MAX-2400, Rigaku, Cu Kα radiation, Japan). The temperature dependence of the dielectric constant and dielectric loss was measured using an LCR meter (4980A, Agilent Technologies, Inc.). A ferroelectric testing system (TF Analyzer 2000E, aixACCT) was used to characterize the piezoelectric strain and the P–E and S–E hysteresis loops. The piezoelectric coefficients were measured by a piezoelectric testing system (ZJ-1, CAS), after poling in a silicon oil bath at 30 kV/cm for 10 min.
Results and Discussion
The X-ray diffraction (XRD) patterns of un-doped and doped KNLN-BZ-BNT ceramics with different amounts of Sm3+ ions are shown in Figure 1A. It can be seen that all the samples show a pure perovskite structure. The introduction of Sm3+ ions (less than 0.5 mol%) hardly changes the crystalline phase of ceramics. The details of (200) and (002) peaks for all samples are shown in Figure 1B, showing an invisible difference, attributed to fewer Sm3+ ions. Besides, the splits of (002) and (200) become a little bit wider with the addition of Sm3+, suggesting a phase close to the tetragonal one after Sm3+ addition.
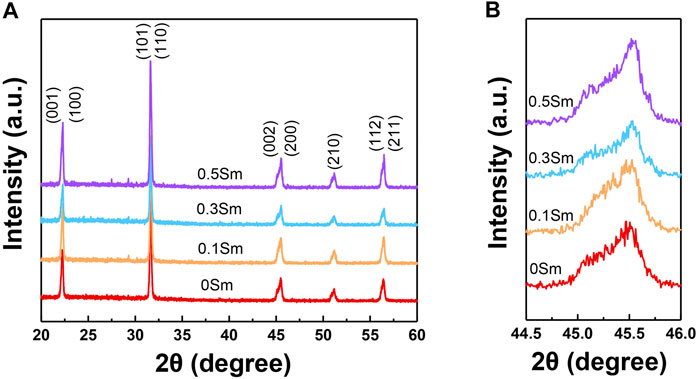
FIGURE 1. XRD patterns of un-doped (Quan et al., 2018) and Sm3+-modified KNLN-BZ-BNT ceramics. (A) 2θ is between 10 and 60°, and (B) 2θ is between 44.5 and 46°.
Figure 2A shows the room-temperature polarization–electrical field (P–E) hysteresis loops of the un-doped and doped samples with different amounts of Sm3+ ions. All the samples show a well-saturated P–E loop. The un-doped KNLN-BZ-BNT ceramic shows the lowest remanent polarization, Pr, of 9.70 μC/cm2. The 0.1 mol% Sm3+–doped ceramic shows the highest Pr of 12.3 μC/cm2. The decreased Pr values for 0.3 and 0.5 mol% Sm3+–doped KNLN-BZ-BNT ceramics could be attributed to the more tetragonal phase. The variation of maximum polarization (Pmax) with the amount of Sm3+ ions shows the same trend as the Pr. The lowest and highest Pmax appeared in the un-doped and 0.1 mol% Sm3+ ion–doped KNLN-BZ-BNT ceramics, respectively. The bipolar electric field–strain (S–E) loops are shown in Figure 2B. Those S–E loops show typical butterfly shapes with high strain, suggesting a typical ferroelectric property. It can be seen that the KNLN-BZ-BNT ceramics show improved piezoelectric strains after doping with Sm3+ ions. The highest strain appeared in the 0.3 mol% and 0.5 mol% Sm3+–doped ceramics, which is around 0.12%.
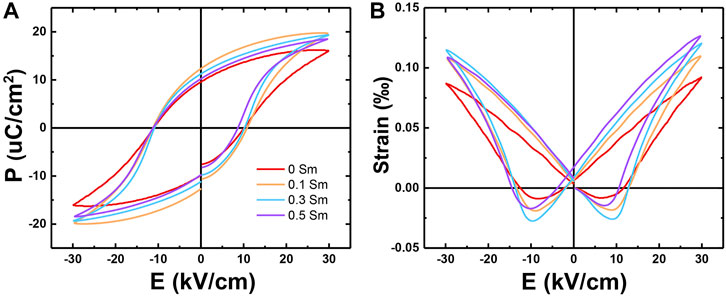
FIGURE 2. (A) Room-temperature polarization–electrical field hysteresis loops; (B) bipolar electric field–strain curves of un-doped (Quan et al., 2018) and Sm3+-modified KNLN-BZ-BNT ceramics.
The temperature dependence of dielectric constants and dielectric losses at the frequency of 1 kHz for all samples is shown in Figure 3. At room temperature, the dielectric constant of the un-doped KNLN-BZ-BNT ceramic is 1,441. Doping with the Sm3+ ions, all the ceramics show a high dielectric constant of 1800 and a low dielectric loss of ∼3%, suitable for further applications. It can be noticed that introducing Sm3+ ions did not affect the Curie temperatures (TC) of KNLN-BZ-BNT ceramics, and all the samples show a TC of 240oC. On further inspection, all the samples did not show dielectric anomaly before the temperature was up to TC, indicating a good ferroelectric-stable characteristic. The temperature of 240oC is enough for some high-temperature applications, such as actuators in car engines (Turner et al., 1994).
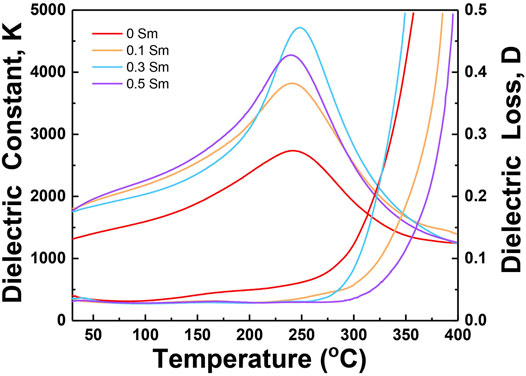
FIGURE 3. Temperature-dependent dielectric constant and dielectric loss of un-doped (Quan et al., 2018) and Sm3+-modified KNLN-BZ-BNT ceramics.
To measure the d33* of the samples, the unipolar strains are measured and shown in Figure 4A. The d33* was calculated by (Zhu et al., 2015; Li et al., 2018)
where S is the strain under E (electric field). The un-doped KNLN-BZ-BNT ceramic shows the lowest strain of 0.087%. The Sm3+-doped ceramics show the enhanced unipolar strains. The strain of the 0.1% Sm3+–doped ceramic is 0.105%. A similar strain of 0.114% was obtained in the doped ceramics with 0.3 mol% and 0.5 mol% Sm3+. Figure 4B plots the variation of d33 and d33* of all the ceramics with the amount of Sm3+. It can be seen that the lowest value appeared in the un-doped KNLN-BZ-BNT ceramic. With the increasing amount of Sm3+ ions, the d33 and d33* increased and the highest values were obtained in the ceramic doped with 0.3 mol% Sm3+ ions. The highest d33 and d33* are 325 pC/N and 384 pm/V, respectively. These results indicate that the 0.3 mol% Sm3+ addition is the most effective way to improve the piezoelectric response of KNLN-BZ-BNT ceramics.
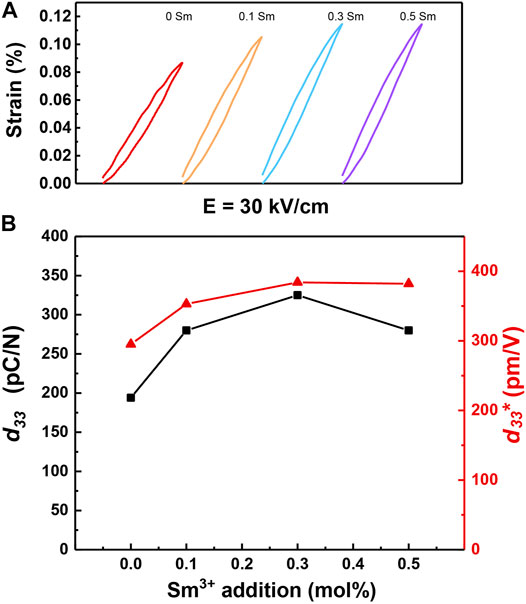
FIGURE 4. (A) Unipolar piezoelectric strains and (B) variation of d33 and d33* of un-doped (Quan et al., 2018) and Sm3+-modified KNLN-BZ-BNT ceramics.
The doping of Sm3+ improved the piezoelectric and ferroelectric properties of KNLN-BZ-BNT ceramics. And the optimal performances were achieved in the sample with 0.3 mol% Sm3+ addition. To investigate the thermal stability of the Sm3+-doped KNLN-BZ-BNT ceramics, the temperature dependence of unipolar strain and P–E loops is shown in Figures 5A,B, respectively. The strain under 30 kV/cm at room temperature is 0.114%; then, it decreased slightly with the increasing temperature. When the temperature went up to 180oC, the unipolar strain remained 0.094%, which shows good thermal stability. The samples show good ferroelectric properties even at the temperature of 180oC. The Pr decreased from 11.19 μC/cm2 at room temperature (30oC) to 7.91 μC/cm2 at 180oC, and the Pmax decreased from 19.29 μC/cm2 to 16.15 μC/cm2. To manifest the temperature dependence of ferroelectric and piezoelectric properties of KNLN-BZ-BNT with 0.3 mol% Sm3+ ions, the variation of Pr and normalized d33* with temperature is plotted in Figure 5C. It can be found that the normalized d33* of the sample decreased less than 20% when the temperature raised from 30 to 180oC, which is better than that in the PZT-5H ceramics (Fang et al., 2019).
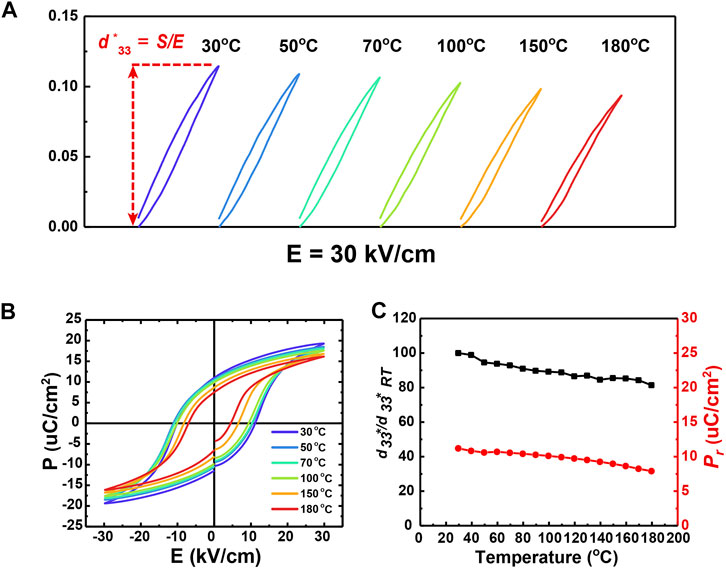
FIGURE 5. (A) Piezoelectric strain under a 3 kV/mm unipolar field and (B) ferroelectric P–E hysteresis loops measured at different temperatures from 30 to 180°C; (C) temperature dependence of the normalized piezoelectric strain d33* and Pr for the KNLN-BZ-BNT ceramic with 0.3% Sm3+ addition.
Conclusion
The un-doped and doped KNLN-BZ-BNT ceramics with 0.1, 0.3, and 0.5 mol% Sm3+ ions were prepared. The remanent polarization, Pr, and piezoelectric coefficients, d33 and d33*, were improved with the introduction of Sm3+ ions. The best performances appeared in the sample with 0.3 mol% Sm3+ ions, showing a d33 of 325 pC/N, a d33* of 384 pm/V, a Pr of 11.19 μC/cm2, and a high strain of 0.114% at 30 kV/cm. Furthermore, the 0.3 mol% Sm3+–doped KNLN-BZ-BNT ceramic shows good thermal stability. The d33* values decreased less than 20% when the temperature raised from 30 to 180oC. These excellent results show the Sm3+-modified KNLN-BZ-BNT ceramics are good for further applications even under high temperature.
Data Availability Statement
The original contributions presented in the study are included in the article/Supplementary Material, and further inquiries can be directed to the corresponding authors.
Author Contributions
YQ did the experiments and wrote this article. LW (second author), WR, JZ (fourth author), JZ (fifth author), and TK designed this work and helped in writing. KZ and ZW helped in performing the experiments. ZJ and LW (10th author) helped in performing the data test.
Funding
This work was supported by the Natural Science Foundation of China (Grant No. 51602243, 51902246, and 51911530125), the Key Research Project of Shaanxi Province of China (Grant No. 2018ZDXM-GY-150), the Xijiang Innovation Team Introduction Program of Zhaoqing, China Postdoctoral Science Foundation (Grant No. 2018M643633 and 2019M663697), the Natural Science Fundamental Research Project of Shaanxi Province of China (No. 2019JQ590), the “111 Project” of China (B14040), and the Fundamental Research Funds for the Central Universities.
Conflict of Interest
ZJ and LW were employed by Guangdong JC Technological Innovation Electronics Co., Ltd.
The remaining authors declare that the research was conducted in the absence of any commercial or financial relationships that could be construed as a potential conflict of interest.
References
Egerton, L., and Dillon, D. M. (1959). Piezoelectric and Dielectric Properties of Ceramics in the System Potassium-Sodium Niobate. J. Am. Ceram. Soc. 42 (9), 438–442. doi:10.1111/j.1151-2916.1959.tb12971.x
Fang, M., Rajput, S., Dai, Z., Ji, Y., Hao, Y., and Ren, X. (2019). Understanding the Mechanism of thermal-stable High-Performance Piezoelectricity. Acta Materialia 169, 155–161. doi:10.1016/j.actamat.2019.03.011
Jiang, L., Xing, J., Tan, Z., Wu, J., Chen, Q., Xiao, D., et al. (2016). High Piezoelectricity in (K,Na)(Nb,Sb)O3-(Bi,La,Na,Li)ZrO3 lead-free Ceramics. J. Mater. Sci. 51 (10), 4963–4972. doi:10.1007/s10853-016-9801-2
Karaki, T., Katayama, T., Yoshida, K., Maruyama, S., and Adachi, M. (2013). Morphotropic Phase Boundary Slope of (K, Na, Li)NbO3-BaZrO3 Binary System Adjusted Using Third Component (Bi, Na)TiO3 Additive. Jpn. J. Appl. Phys. 52 (9S1), 09KD11. doi:10.7567/jjap.52.09kd11
Li, P., Zhai, J., Shen, B., Zhang, S., Li, X., Zhu, F., et al. (2018). Ultrahigh Piezoelectric Properties in Textured (K,Na)NbO3 -Based Lead-Free Ceramics. Adv. Mater. 30 (8), 1705171. doi:10.1002/adma.201705171
Quan, Y., Fei, C., Ren, W., Wang, L., Niu, G., Zhao, J., et al. (2020). Lead-free KNN-Based Textured Ceramics for High Frequency Ultrasonic Transducer Application. IEEE Trans. Ultrason. Ferroelectrics, Frequency Control. 68 (5), 1979–1987. doi:10.1109/TUFFC.2020.3039120
Quan, Y., Ren, W., Niu, G., Wang, L., Zhao, J., Zhang, N., et al. (2018). Large Piezoelectric Strain with superior thermal Stability and Excellent Fatigue Resistance of lead-free Potassium Sodium Niobate-Based Grain Orientation-Controlled Ceramics. ACS Appl. Mater. Inter. 10 (12), 10220–10226. doi:10.1021/acsami.8b01554
Quan, Y., Wang, L., Ren, W., Niu, G., Zhao, J., Zhuang, J., et al. (2019). Effects of MnO2 Addition on the Electrical Properties of lead-free Textured Potassium Sodium Niobate-Based Ceramics. Ferroelectrics 553 (1), 51–59. doi:10.1080/00150193.2019.1683495
Saito, Y., Takao, H., Tani, T., Nonoyama, T., Takatori, K., Homma, T., et al. (2004). Lead-free Piezoceramics. Nature 432 (7013), 84–87. doi:10.1038/nature03028
Shrout, T. R., and Zhang, S. J. (2007). Lead-free Piezoelectric Ceramics: Alternatives for PZT?. J. Electroceram 19 (1), 113–126. doi:10.1007/s10832-007-9047-0
Shung, K. K. (2015). Diagnostic Ultrasound: Imaging and Blood Flow Measurements. Boca Raton: CRC Press.
Turner, R. C., Fuierer, P. A., Newnham, R. E., and Shrout, T. R. (1994). Materials for High Temperature Acoustic and Vibration Sensors: A Review. Appl. Acoust. 41 (4), 299–324. doi:10.1016/0003-682x(94)90091-4
Uchino, K. (1996). Piezoelectric Actuators and Ultrasonic Motors. Norwell: Springer Science & Business Media.
Wang, K., Yao, F.-Z., Jo, W., Gobeljic, D., Shvartsman, V. V., Lupascu, D. C., et al. (2013). Temperature-Insensitive (K,Na)NbO3-Based Lead-Free Piezoactuator Ceramics. Adv. Funct. Mater. 23 (33), 4079–4086. doi:10.1002/adfm.201203754
Wu, J., Xiao, D., and Zhu, J. (2015). Potassium-Sodium Niobate Lead-Free Piezoelectric Materials: Past, Present, and Future of Phase Boundaries. Chem. Rev. 115 (7), 2559–2595. doi:10.1021/cr5006809
Zeng, Y., Jiang, L., Sun, Y., Yang, Y., Quan, Y., Wei, S., et al. (2020). 3D-Printing Piezoelectric Composite with Honeycomb Structure for Ultrasonic Devices. Micromachines 11 (8), 713. doi:10.3390/mi11080713
Zhang, M.-H., Wang, K., Du, Y.-J., Dai, G., Sun, W., Li, G., et al. (2017). High and Temperature-Insensitive Piezoelectric Strain in Alkali Niobate lead-free Perovskite. J. Am. Chem. Soc. 139 (10), 3889–3895. doi:10.1021/jacs.7b00520
Zhang, S., Xia, R., and Shrout, T. R. (2007). Lead-free Piezoelectric Ceramics vs. PZT? J. Electroceram 19 (4), 251–257. doi:10.1007/s10832-007-9056-z
Zhao, J., Ren, W., Niu, G., Zhang, N., Dong, G., Wang, L., et al. (2017). Recoverable Self-Polarization in lead-free Bismuth Sodium Titanate Piezoelectric Thin Films. ACS Appl. Mater. Inter. 9 (34), 28716–28725. doi:10.1021/acsami.7b04033
Zhao, J., Zhang, N., Ren, W., Niu, G., Walker, D., Thomas, P. A., et al. (2019). Polar Domain Structural Evolution under Electric Field and Temperature in the (Bi0.5 Na0.5 )TiO3 -0.06BaTiO3 Piezoceramics. J. Am. Ceram. Soc. 102 (1), 437–447. doi:10.1111/jace.15883
Zheng, K., Quan, Y., Zhuang, J., Zhao, J., Ren, W., Wang, L., et al. (2021). Achieving High Piezoelectric Performances with Enhanced Domain-wall Contributions in -textured Sm-Modified PMN-29PT Ceramics. J. Eur. Ceram. Soc. 41 (4), 2458–2464. doi:10.1016/j.jeurceramsoc.2020.11.027
Keywords: lead-free piezoelectric, KNN-based ceramics, rare earth, piezoelectric properties, thermal stability
Citation: Quan Y, Wang L, Ren W, Zhao J, Zhuang J, Zheng K, Wang Z, Karaki T, Jiang Z and Wen L (2021) Enhanced Electrical Properties of Lead-Free Piezoelectric KNLN-BZ-BNT Ceramics With the Modification of Sm3+ Ions. Front. Mater. 8:695330. doi: 10.3389/fmats.2021.695330
Received: 14 April 2021; Accepted: 05 May 2021;
Published: 28 May 2021.
Edited by:
Chunlong Fei, Xidian University, ChinaReviewed by:
Wanlin Zhu, Pennsylvania State University (PSU), United StatesJiapu Li, Huazhong University of Science and Technology, China
Copyright © 2021 Quan, Wang, Ren, Zhao, Zhuang, Zheng, Wang, Karaki, Jiang and Wen. This is an open-access article distributed under the terms of the Creative Commons Attribution License (CC BY). The use, distribution or reproduction in other forums is permitted, provided the original author(s) and the copyright owner(s) are credited and that the original publication in this journal is cited, in accordance with accepted academic practice. No use, distribution or reproduction is permitted which does not comply with these terms.
*Correspondence: Lingyan Wang, l.y.wang@mail.xjtu.edu.cn; Wei Ren, wren@mail.xjtu.edu.cn