- 1Department of Chemistry, College of Science, University of Hafr Al Batin, Hafr Al Batin, Saudi Arabia
- 2Department of Physics, College of Science, University of Hafr Al Batin, Hafr Al Batin, Saudi Arabia
- 3Department of Chemistry, King Fahd University of Petroleum and Minerals, Dhahran, Saudi Arabia
Titanium(IV) oxide (TiO2, titania) is well-known for its excellent photocatalytic properties, wide bandgap, chemical resistance, and photostability. Nanostructured TiO2 is extensively utilized in various electronic and energy-related applications such as resistive switching memory devices, flat panel displays, photodiodes, solar water-splitting, photocatalysis, and solar cells. This article presents recent advances in the design and nanostructuring of TiO2-containing antireflective self-cleaning coatings for solar cells. In particular, the energy harvesting efficiency of a solar cell is greatly diminished by the surface reflections and deposition of environmental contaminants over time. Nanostructured TiO2 coatings not only minimize reflection through the graded transition of the refractive index but simultaneously improve the device’s ability to self-clean and photocatalytically degrade the pollutants. Thus, novel approaches to achieve higher solar cell efficiency and stability with pristine TiO2 and TiO2-containing nanocomposite coatings are highlighted herein. The results are compared and discussed to emphasize the key research and development shortfalls and a commercialization perspective is considered to guide future research.
Introduction
The environmental challenges such as global warming, air pollution, and climate change resulting from the burning of fossil fuels and growing awareness about these issues prompted the use of alternative and renewable energy sources. In the last two decades, solar energy has emerged as one such alternative with worldwide solar energy generation reaching 724 TWh in 2019 (Ritchie and Roser, 2020). Albeit it is a small share of the total worldwide energy generation from renewable sources, i.e., hydropower, solar, wind, geothermal, biomass, and biofuels (Figure 1A), solar energy share has grown from 0.04 in 2000 to 10.3% in 2019 (Figure 1B).
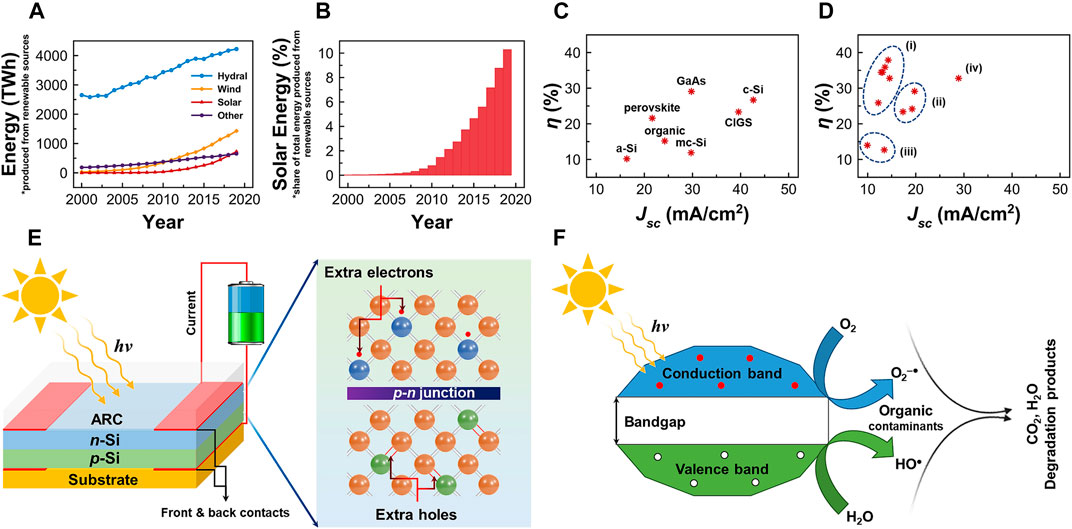
FIGURE 1. (A) Total worldwide energy production from renewable sources, i.e., hydropower, wind, solar, and others including biofuels and biomass, etc., in the last two decades; data obtained from Ritchie and Roser (2020). (B) The share (%) of solar energy among total energy produced from renewable sources over the last two decades; data obtained from Ritchie and Roser (2020). (C) Confirmed incident photon-to-current conversion efficiency (IPCE, η (%)) and short-circuit current density (Jsc (mA/cm2)) of single-junction terrestrial solar cell based on different materials, data obtained from Green et al. (2021). (D) Confirmed IPCE (η (%)) and Jsc (mA/cm2) of multi-junction terrestrial solar cell based on different materials, i) GaInP-based multi-junction cells; ii) c-Si multijunction solar cells with perovskite and GaAsP; iii) a-Si/nc-Si based multi-junction cells; and iv) mechanically-stacked GaAs/c-Si, data obtained from Green et al. (2021). Abbreviations: a-Si,amorphous silicon; c-Si,crystalline silicon; CIGS, copper indium gallium selenide, Cu(In,Ga)Se; GaAs, gallium arsenide thin film; mc-Si, multicrystalline silicon; nc-Si, nanocrystalline silicon. (E) A typical design of single p-n junction silicon solar cell showing the antireflective coating on top. (F) A schematic of the self-cleaning process: the mechanism of photooxidative degradation of organic contaminants under sunlight irradiation. The charge carriers, i.e. pairs of electrons (e−) and holes (h+), are formed by the absorption of photons, which initiates the oxidative and reductive pathways at the conduction and valence bands, respectively via further reactions with active oxygen species or hydrolysis and leads to the photocatalytic degradation of contaminants to mineralized products.
The rise in solar energy harvesting is backed by years of research and innovation starting from the development of silicon p-n junction solar cells with a significantly improved solar energy conversion efficiency in 1954 (Chapin et al., 1954; Loferski, 1993). Shortly after, silicon solar cells became commercially available (Stabler, 1960), and led the space solar cell industry for decades (Bailey and Raffaelle, 2012). However, over the past two decades, several other materials and dual or multiple p-n junction cell designs with improved quantum efficiency have been commercialized for terrestrial applications (Green et al., 2021). Nowadays, the performance of these cells has greatly improved, see Figures 1C,D.
Yet, a major limiting factor for the quantum efficiency of solar cells is the optical loss due to reflection. For instance, in the air atmosphere, a polished silicon surface reflects >30% of the incident solar radiations (Thomas et al., 1989). Therefore, to reduce optical loss due to high reflectivity and improve the energy harvesting capability of solar cells, different solutions have been proposed such as the utilization of the antireflective coatings (ARCs), surface texturization, or a combination of both to increase the optical path length of the light within solar cells (Singh and Verma, 2019; Abu-Shamleh et al., 2021; Sagar and Rao, 2021).
Furthermore, in terrestrial sunlight-to-electricity generation applications, solar modules and panels are exposed to dust, organic contaminants, industrial pollutants, and harsh environmental conditions, e.g., sand storms (Said et al., 2015; Quan and Zhang, 2017), which also detriment their efficiency. The development of self-cleaning coatings is desired to address these issues (Soklič et al., 2015; Huang et al., 2018; Zhi and Zhang, 2018). Ideally, a coating material for terrestrial solar cell applications should be robust, transparent, antireflective, and capable of self-cleaning itself.
Titania (TiO2) is an ideal material due to its excellent physicochemical properties and has been widely investigated in such applications, e.g., high-efficiency solar cells (O’Regan and Grätzel, 1991; Kim et al., 2008), ARCs (Wan et al., 2010; Manea et al., 2013; Salvaggio et al., 2016), and photocatalysis (Fujishima et al., 2000; Fujishima et al., 2008) especially photooxidative self-cleaning (Paz et al., 1995; Muraca et al., 2019). TiO2 has a bandgap of 3.2 eV and absorbs light in a wide range of frequencies. Also, in photocatalysis, TiO2 nanoparticles (P25) are considered as the photocatalytic standard.
Other oxides such as Ta2O5 (Thomas et al., 1989; Sagar and Rao, 2021) could offer better antireflective properties but are not good enough for photocatalytic degradation of environmental contaminants. Therefore, these oxides are often used in combination with TiO2 to form self-cleaning ARCs. Herein, the role of self-cleaning ARCs based on TiO2 nanostructures and nanocomposites in reducing the reflectivity and improving the overall energy harvesting capability is discussed. For high-efficiency solar cell applications, the referenced literature is critically analyzed with a particular emphasis on the scalability of coating methods, industrial viability, cost-effectiveness, and commercialization viewpoints.
Antireflective Properties of TiO2
TiO2 Single-Layer Antireflective Coatings
Figure 1E presents the design of a typical single-junction silicon solar cell showing ARC as an integral part of the device. ARCs based on TiO2 have been known to significantly reduce the solar averaged reflectance of the silicon solar cells (San Vicente et al., 2001; San Vicente et al., 2002). For instance, Cai et al. (2012) produced quasi-omnidirectional ARCs by direct hydrothermal fabrication of rutile-TiO2 nanorod arrays on Ti-foils. These mesocrystalline TiO2 nanorods suppressed the reflection to <2.0% in the near-infrared (NIR) and <0.5% in the visible regions. The excellent antireflection properties of TiO2 nanorod arrays were attributed to the hierarchical tips-on-rod nanostructures that provided an optimal graded refractive index.
Consequently, TiO2 ARCs with reduced reflectivity can enhance the quantum efficiency, or so-called incident photon-to-current conversion efficiency (IPCE). The researchers have shown that a compact TiO2 layer (60–100 nm) on dye-sensitized solar cells can improve the IPCE by 50% (Abdullah and Rusop, 2013; Abdullah and Rusop, 2014). The enhancement in IPCE is attributed to the lower optical loss (67% decreased reflectivity), the red-shift of transmittance peak, i.e., greater energy absorption in the visible region, and reduced electron-hole recombination at the interface of TiO2 (Abdullah and Rusop, 2013; Abdullah and Rusop, 2014).
To facilitate mass production and reduce the cost-to-efficiency ratio, atmospheric-pressure chemical vapor deposition (APCVD) is proposed (Hocine et al., 2013; Hocine et al., 2015). Thus-formed thin TiO2 ARCs exhibit a 75% decrease in reflectivity and a 27% increase in efficiency of multicrystalline silicon solar cells, which is attributed to the enhanced light transmission by TiO2 ARC and increased short-circuit current (Hocine et al., 2013). Leem et al. (2014) produced ARCs composed of TiO2 subwavelength nanostructures through electron beam evaporation and dry etching and observed 33% enhancement in IPCE of GaAs solar cells.
TiO2-Containing Multi-Layer Antireflective Coatings
Double-layer AR (DLAR) or triple-layer AR (TLAR) coatings further reduce the reflectivity and have long been adopted to boost solar cell efficiency (Jiao and Anderson, 1987; Aiken, 2000; Zhang et al., 2006). Compared to commercial single-layer TiO2 coatings, DLAR from single-material TiO2 reduces the solar weighted average reflectance and improves the efficiency of silicon solar cells (Richards, 2003). A notable achievement in this regard was reported by Lien et al. (2006), who deposited TiO2 SLAR, SiO2/TiO2 DLAR, and SiO2/SiO2–TiO2/TiO2 TLAR coatings on silicon solar cells via a sol-gel process. They observed a 39.5% increase in solar cell efficiency with SiO2/SiO2–TiO2/TiO2 TLAR coatings.
Liu et al. (2014) also obtained ∼32% enhancement in short-circuit current and IPCE of GaAs multi-junction solar cells with SiO2/graded-index TiO2 DLAR top coatings. There are several other reports in favor of reduced broadband reflectance exhibited by DLAR or TLAR coatings based on TiO2 and SiO2 (Ali et al., 2014; Li et al., 2014; Lin et al., 2018; Liao et al., 2020; Yuan et al., 2020). Recently, Zhang et al. (2021) tested TiO2/Al2O3 DLAR and TiO2/Al2O3/MgF2 TLAR coatings for GaInP/InGaAs/Ge triple-junction solar cells. They reported a respective decrease of 18.9 and 22.2% in reflectivity, while an increase of 21.29 and 29.44% in IPCE.
Nonetheless, irrespective of the ARC material, composition, fabrication procedure, cell type, design, etc., a minimum of 8.5% (Li et al., 2014) to 54.5% (Ho et al., 2015) enhancements in IPCE have been reported. Therefore, DLAR or TLAR coatings can be utilized to improve the overall performance of different types of solar cells provided they do not require multiple fabrication steps and do not add to their complexity.
Photooxidative Self-Cleaning Performance of TiO2
The self-cleaning ARCs are desirable for terrestrial solar cell application to withstand harsh environments, air pollution, and organic contaminations (San Vicente et al., 2012). Due to its excellent photocatalytic properties, TiO2 is widely employed in self-cleaning ARCs for solar cell cover glass and solar panels, self-cleaning windows, indoor air purification systems, sterilization of water, degradation of organic contaminants, and antibacterial applications (Faustini et al., 2010; Banerjee et al., 2015). Figure 1F illustrates the mechanism of photocatalytic decomposition of organic contaminants on TiO2 surface under sunlight irradiation. Photooxidative properties of commercially available TiO2 particles are generally demonstrated by a conventional dye-degradation experiment under UV illumination (Nakata et al., 2011).
Efforts to move from UV to visible light and efficiently utilize solar energy with TiO2 are still underway. To achieve this goal, TiO2 is often doped or mixed with different materials, e.g., non-metals, metals, metallic oxides, etc., for better photocatalytic performance under direct sunlight (Medina-Valtierra et al., 2009; Li and He, 2013; Suárez et al., 2017). For example, TiO2 films doped with 5% Ni show an increased light transmission in the visible and NIR regions and lower reflectance (Haider et al., 2016). V-doped TiO2:SiO2 sol-gel ARCs with 15% V-TiO2 content also demonstrate low refractive index and excellent photooxidative degradation of methylene blue (k = 0.033 min−1) (Adak et al., 2017).
TiO2 doping with metal atoms such as V alters its electronic structure and influences its photoactivity. V atoms may act as traps for photogenerated electrons and holes and reduce their recombination (Adak et al., 2017). The electrons from V4+ centers are photoexcited to the TiO2 conduction band, while the resulting V5+ centers bind with surface oxygen atoms. Consequently, ●OH free radicals are formed in the presence of holes (Klosek and Raftery, 2002). Additionally, superoxide-free radicals (O2−●) are produced by the reduction of O2 on TiO2 surface, which are strong oxidants for organic contaminants.
Therefore, V-doped TiO2 improves photooxidative decomposition of methylene blue under visible-light illumination (Adak et al., 2017). A similar phenomenon is observed by Naufal et al. (2017) with 2% Sm3+-doped TiO2, which exhibits a highly defective surface due to oxygen deficiency and significantly improves photocatalytic oxidation of methylene blue (k = 0.103 min−1) under sunlight illumination.
Furthermore, graphene or reduced-graphene oxide (rGO) have rarely been incorporated in TiO2 ARCs despite their excellent electrical properties. A recent study reveals that rGO-TiO2 surfaces are an outstanding alternative for photooxidative self-cleaning applications (Prabhu et al., 2017). This is supported by a sharp increase in photocurrent response of 0.5 wt% rGO-TiO2 upon illumination, which suggests the greater separation of photogenerated electrons and holes. Being a zero-bandgap conductor, rGO promotes the transfer of photogenerated electrons and reduces the recombination rate (Wang et al., 2013; Qiu et al., 2015). Therefore, rGO-TiO2 could act as a potential self-cleaning ARC in energy harvesting devices.
Physicochemical Strategies for Improved TiO2 Antireflection and Self-Cleaning Properties
In general, two strategies are used to design ARCs (Keshavarz Hedayati and Elbahri, 2016), the conventional stacking layers (single or multiple layers) and ultrathin plasmonic metasurfaces. However, the works reviewed herein only involve conventional single- or multi-layer ARC for solar cells and modules. The formation of DLAR or TLAR coatings enhances the efficiency of solar cells by suppressing reflectivity and improving the short-circuit current. However, several other physicochemical strategies have been adopted for enhanced performance of TiO2 ARCs. These include the inclusion of a porogen (pore-forming agent) and chemical acid-etching with 5% HF that increase the porosity and surface roughness, respectively, and reduce reflectivity (Rad et al., 2020).
It is believed that the formation of 3D nanoporous structures may achieve zero-reflectance conditions (Bravo et al., 2007; Guldin et al., 2013). For instance, Nakata et al. (2011) concluded that the self-cleaning effect and high transmittance of a thick layer of TiO2 particles are derived from its porous structure. In this regard, an innovative way to produce highly porous ARC involves the sol-gel synthesis of TiO2 nanoparticles combined with block-copolymer self-assembly that yields excellent antireflective and photocatalytic properties (Guldin et al., 2013).
The functionalization of TiO2 surface with silane coupling agents, i.e., tetraethoxysilane and methyltriethoxysilane, is shown to decrease the reflectance of bare-TiO2 films from 26 to 17.3% and 15.5%, respectively (Purcar et al., 2019). The surface modification of TiO2 photocatalyst and SiO2/TiO2/SiO2-TiO2 TLAR with fluoroalkylsilane and hexamethyldisilazane, respectively, promotes hydrophobicity and self-cleaning (Nakajima et al., 2000; Ye et al., 2013). Similarly, metal-doped TiO2 ARCs are shown to alter the bandgap, increase solar energy absorption and conversion, and improve self-cleaning properties (Haider et al., 2016; Adak et al., 2017).
Another strategy involves metal plasma ion implantation of various metal ions (e.g., Fe, Cr, V) in high-quality TiO2 films, which activates the TiO2 surface and improves the sunlight absorption rate (Weng and Huang, 2013). The implantation of Fe3+ is particularly observed to decrease the recombination of photogenerated holes and electrons and increase oxygen vacancies (Yu et al., 2006; Weng and Huang, 2013). This also leads to dissociative adsorption of water molecules, which in turn increases surface hydroxyl content and self-cleaning ability of TiO2.
A photocatalytic ARC using a heterojunction structure between low bandgap WO3 (2.8 eV) and higher bandgap TiO2 (3.2 eV) nanoparticles is developed by Noh and Myong (2014). WO3 nanoparticles not only generate a self-cleaning effect but act as visible-light sensitizers and transfer the excited electrons to higher bandgap TiO2. Thus, WO3-TiO2 ARC shows 4.3% higher energy output for silicon-based photovoltaic modules (Noh and Myong, 2014).
Recently, vertically aligned Pt-decorated p-MoS2 nanostructures are fabricated on n-TiO2 nanotube arrays to benefit from Pt/MoS2 Schottky heterojunction and MoS2/TiO2p-n heterojunction for visible-light degradation of methylene blue (Dong et al., 2020). These innovations are particularly helpful in understanding the underlying mechanisms to improve the efficiency and lifetime of solar energy harvesting devices. However, there is a need to overcome several practical challenges for large-scale production and application of these devices, as discussed below.
Notable Achievements and Practical Challenges
Table 1 presents notable achievements and selected examples to provide an overview of the impact of TiO2 ARCs on enhancing antireflectivity and efficiency. TiO2 is an n-type semiconductor due to inherent oxygen vacancies (Nowotny et al., 2006). Therefore, TiO2 layers help electron injection and ease electron transport by providing an electrical path for the photogenerated electrons (Chong et al., 2005; Dong et al., 2010). In solar cell applications, n-TiO2 ARC deposited on p-Si (Figure 1E) combines to form a heterojunction, thereby allowing one type of charge carriers (electrons) and blocking holes to improve the efficiency of the solar cells (Avasthi et al., 2013; Singh et al., 2017). TiO2 ARCs can also get rid of the environmental contaminants through photooxidative degradation in the presence of sunlight (Naufal et al., 2017).
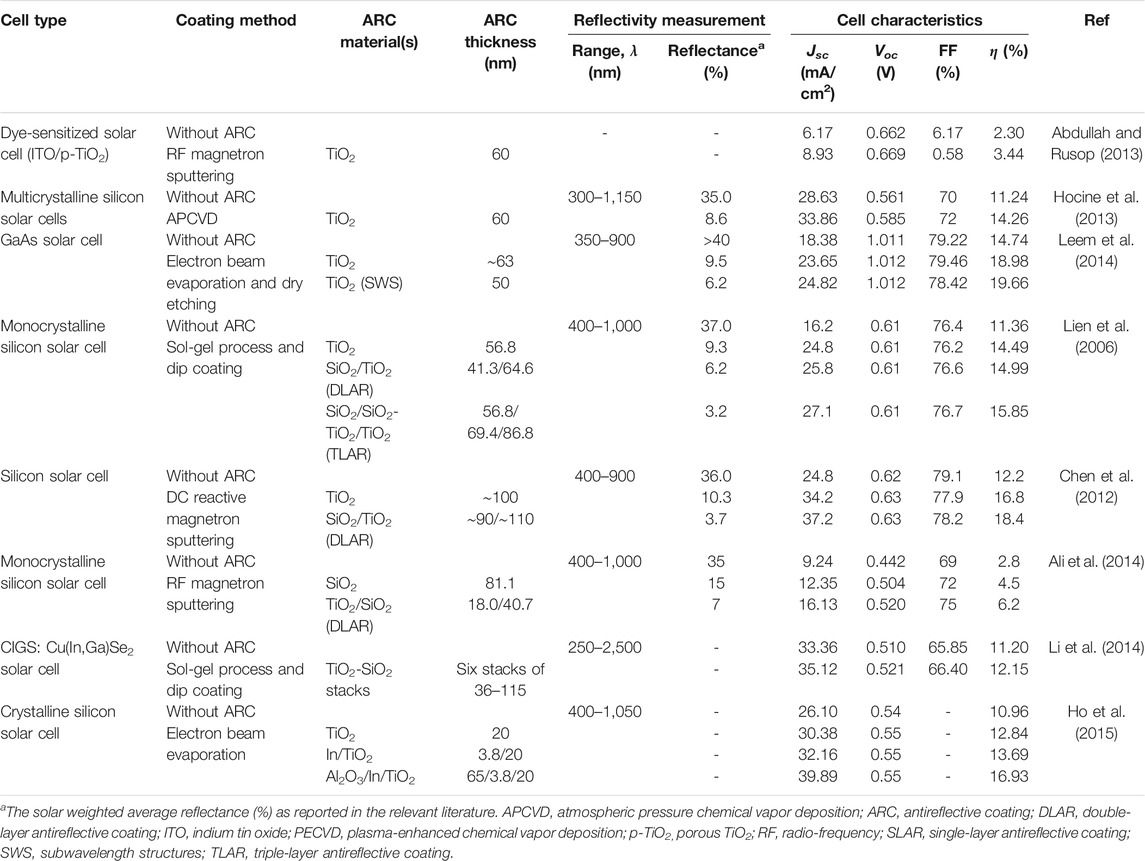
TABLE 1. Notable achievements in the development of TiO2-based antireflective coatings (ARCs) and figures of merit showing the resulting enhancement in solar cells’ performance.
In addition to its self-cleaning properties, the hardness of TiO2 ARC makes it a scratch-resistant shielding cover for solar cells and prevents mechanical damage (Wang et al., 2015; Mazur et al., 2016). An ultrathin (∼23 nm) layer of nanoporous TiO2 deposited on SiO2-TiO2 ARC not only ensures self-cleaning but acts as a protective barrier against abrasion and mechanical damage, thus providing high chemical and mechanical durability (Miao et al., 2013).
A temperature and humidity stress test reveals that DLAR with a denser TiO2/ZrO2 barrier layer is more durable showing only 1% reduction in transmittance after 96 h (Li et al., 2013). Albeit the persistence of optical activity over a long period is not usually tested, a few cases reported in the literature reveal that TiO2 ARCs resist aging and maintain low reflectivity (≤1%) after 1,200 h of testing (Xu et al., 2013).
However, there are several practical challenges associated with the fabrication of ARCs. Considering the commercialization perspective and industrial-scale fabrication of ARCs, it is important to maintain the cost-efficiency balance and ease in the fabrication of uniform TiO2 ARCs. For solar cells and modules, self-cleaning ARCs are generally deposited by vacuum methods such as physical/chemical vapor deposition, sputtering, and thermal evaporation. These methods are reproducible and yield extremely stable ARCs with the controlled thickness (Shahiduzzaman et al., 2017; Pae et al., 2018). However, these are expensive and are less versatile.
The sol-gel process is an alternative wet-chemical route that has been largely studied for the fabrication of self-cleaning ARCs (San Vicente et al., 2001; San Vicente et al., 2002). Compared to vacuum processes, the sol-gel method is low-cost and offers greater flexibility in the choice of raw materials, solvents, molar ratios, and experimental conditions, e.g., pH, temperature, etc. (Wang and Shen, 2010). However, the sol-gel process can have reproducibility issues along with a possibility of poor adhesion and mechanical strength due to excessive solvent evaporation. To improve mechanical properties and avoid abrasion damage, post-deposition heat treatment is necessary (Langlet et al., 2001), which may increase cost and fabrication time/steps. Also, the coating procedure for sol-gel materials (dip- or spin-coating) is tedious and irreplicable.
Similarly, extremely high gains in efficiency of solar cells have been reported with innovative ARC-fabrication techniques such as electron beam evaporation and dry-etching (Leem et al., 2014). The fabrication of plasma ion-implanted or vertically-aligned composite nanostructures is also fascinating and delivers excellent results (Weng and Huang, 2013; Dong et al., 2020). However, these methods are less suitable for mass production at the industrial scale due to inherent reproducibility issues and an increased number of steps involved and cost. Therefore, an important aspect of future research is to find an optimal cost-to-efficiency balance through innovative yet simple, scalable, reproducible, and cost-effective methods for the fabrication of TiO2 ARCs.
Concluding Remarks
The quantum efficiency of terrestrial solar cells is fundamentally restricted to approximately 30% due to high reflectivity, i.e., nearly 35% (Diedenhofen et al., 2012; Sheng et al., 2013), albeit higher efficiencies are theoretically and practically achievable with multi-junction solar cells (Pakhanov et al., 2018; Geisz et al., 2020). The optical loss due to reflectivity and environmental conditions can be countered through self-cleaning ARCs to achieve the true potential. TiO2 is a choice of material that can simultaneously perform both functions: antireflectivity and self-cleaning. Overall, the properties of TiO2 ARCs make them ideal candidates for high-efficiency, long-life solar cell production, and commercialization. However, there is a need to design scalable and cost-effective approaches for their fabrication.
Data Availability Statement
All relevant data presented in this study are included in the article, further inquiries can be directed to the corresponding author.
Author Contributions
AA conceived the idea, secured funding, administered the project, performed a literature survey and analyses, written the first draft, reviewed and edited the manuscript. AH, IU, and MS are co-investigators and helped in literature survey, review, and editing. AR reviewed and edited the work. The authors have read this work and approved it for publication.
Funding
The authors extend their appreciation to the Deputyship for Research and Innovation, Ministry of Education in Saudi Arabia for funding this research work through the project number IFP-A-202-1-1.
Conflict of Interest
The authors declare that the research was conducted in the absence of any commercial or financial relationships that could be construed as a potential conflict of interest.
Acknowledgments
AA gratefully acknowledges the financial support provided by the Agency for Research and Innovation, Ministry of Education in Saudi Arabia through the project number IFP-A-202-1-1. AA also appreciates the assistance received from the Deanship of Scientific Research, University of Hafr Al Batin, Saudi Arabia to carry out this work.
References
Abdullah, M. H., and Rusop, M. (2013). Novel ITO/arc-TiO2 Antireflective Conductive Substrate for Transmittance Enhanced Properties in Dye-Sensitized Solar Cells. Microelectronic Eng. 108, 99–105. doi:10.1016/j.mee.2013.02.081
Abdullah, M. H., and Rusop, M. (2014). RF Sputtered Tri-functional Antireflective TiO2 (Arc-TiO2) Compact Layer for Performance Enhancement in Dye-Sensitised Solar Cell. Ceramics Int. 40, 967–974. doi:10.1016/j.ceramint.2013.06.093
Abu-Shamleh, A., Alzubi, H., and Alajlouni, A. (2021). Optimization of Antireflective Coatings with Nanostructured TiO2 for GaAs Solar Cells. Photon. Nanostructures - Fundamentals Appl. 43, 100862. doi:10.1016/j.photonics.2020.100862
Adak, D., Ghosh, S., Chakrabarty, P., Mondal, A., Saha, H., Mukherjee, R., et al. (2017). Self-cleaning V-TiO2:SiO2 Thin-Film Coatings with Enhanced Transmission for Solar Glass Cover and Related Applications. Solar Energy 155, 410–418. doi:10.1016/j.solener.2017.06.014
Aiken, D. J. (2000). High Performance Anti-reflection Coatings for Broadband Multi-junction Solar Cells. Solar Energ. Mater. Solar Cell 64, 393–404. doi:10.1016/S0927-0248(00)00253-1
Ali, K., Khan, S. A., and Jafri, M. Z. M. (2014). Effect of Double Layer (SiO2/TiO2) Anti-reflective Coating on Silicon Solar Cells. Int. J. Electrochem. Sci. 9, 10. doi:10.1186/1556-276x-9-175
Avasthi, S., McClain, W. E., Man, G., Kahn, A., Schwartz, J., and Sturm, J. C. (2013). Hole-blocking Titanium-Oxide/silicon Heterojunction and its Application to Photovoltaics. Appl. Phys. Lett. 102, 203901. doi:10.1063/1.4803446
Bailey, S., and Raffaelle, R. (2012). “Operation of Solar Cells in a Space Environment,” in Practical Handbook Of Photovoltaics. Editors A. McEvoy, T. Markvart, and L. Castañer. Second Edition (Boston: Academic Press), 863–880. doi:10.1016/B978-0-12-385934-1.00027-1
Banerjee, S., Dionysiou, D. D., and Pillai, S. C. (2015). Self-cleaning Applications of TiO2 by Photo-Induced Hydrophilicity and Photocatalysis. Appl. Catal. B: Environ. 176–177, 396–428. doi:10.1016/j.apcatb.2015.03.058
Bravo, J., Zhai, L., Wu, Z., Cohen, R. E., and Rubner, M. F. (2007). Transparent Superhydrophobic Films Based on Silica Nanoparticles. Langmuir 23, 7293–7298. doi:10.1021/la070159q
Cai, J., Ye, J., Chen, S., Zhao, X., Zhang, D., Chen, S., et al. (2012). Self-cleaning, Broadband and Quasi-Omnidirectional Antireflective Structures Based on Mesocrystalline Rutile TiO2 Nanorod Arrays. Energy Environ. Sci. 5, 7575–7581. doi:10.1039/C2EE03573A
Chapin, D. M., Fuller, C. S., and Pearson, G. L. (1954). A New Silicon P‐n Junction Photocell for Converting Solar Radiation into Electrical Power. J. Appl. Phys. 25, 676–677. doi:10.1063/1.1721711
Chen, Y., Zhou, J. C., Zhao, B. X., and Sun, J. J. (2012). SPUTTERED TiO2 FILMS FOR PHOTOVOLTAIC CELL'S ANTIREFLECTION COATING. Int. J. Nanosci. 11, 1250008. doi:10.1142/S0219581X12500081
Chong, L. H., Mallik, K., Groot, C. H. d., and Kersting, R. (2005). The Structural and Electrical Properties of Thermally Grown TiO2thin Films. J. Phys. Condens. Matter 18, 645–657. doi:10.1088/0953-8984/18/2/020
Diedenhofen, S. L., Grzela, G., Haverkamp, E., Bauhuis, G., Schermer, J., and Rivas, J. G. (2012). Broadband and Omnidirectional Anti-reflection Layer for III/V Multi-junction Solar Cells. Solar Energ. Mater. Solar Cell 101, 308–314. doi:10.1016/j.solmat.2012.02.022
Dong, J., Huang, J., Wang, A., Biesold-McGee, G. V., Zhang, X., Gao, S., et al. (2020). Vertically-aligned Pt-Decorated MoS2 Nanosheets Coated on TiO2 Nanotube Arrays Enable High-Efficiency Solar-Light Energy Utilization for Photocatalysis and Self-Cleaning SERS Devices. Nano Energy 71, 104579. doi:10.1016/j.nanoen.2020.104579
Dong, X., Tao, J., Li, Y., and Zhu, H. (2010). Oriented Single Crystalline TiO2 Nano-Pillar Arrays Directly Grown on Titanium Substrate in Tetramethylammonium Hydroxide Solution. Appl. Surf. Sci. 256, 2532–2538. doi:10.1016/j.apsusc.2009.10.100
Faustini, M., Nicole, L., Boissière, C., Innocenzi, P., Sanchez, C., and Grosso, D. (2010). Hydrophobic, Antireflective, Self-Cleaning, and Antifogging Sol−Gel Coatings: An Example of Multifunctional Nanostructured Materials for Photovoltaic Cells. Chem. Mater. 22, 4406–4413. doi:10.1021/cm100937e
Fujishima, A., Rao, T. N., and Tryk, D. A. (2000). Titanium Dioxide Photocatalysis. J. Photochem. Photobiol. C: Photochem. Rev. 1, 1–21. doi:10.1016/S1389-5567(00)00002-2
Fujishima, A., Zhang, X., and Tryk, D. (2008). TiO2 Photocatalysis and Related Surface Phenomena. Surf. Sci. Rep. 63, 515–582. doi:10.1016/j.surfrep.2008.10.001
Geisz, J. F., France, R. M., Schulte, K. L., Steiner, M. A., Norman, A. G., Guthrey, H. L., et al. (2020). Six-junction III–V Solar Cells with 47.1% Conversion Efficiency under 143 Suns Concentration. Nat. Energ. 5, 326–335. doi:10.1038/s41560-020-0598-5
Green, M., Dunlop, E., Hohl‐Ebinger, J., Yoshita, M., Kopidakis, N., and Hao, X. (2021). Solar Cell Efficiency Tables (Version 57). Prog. Photovolt Res. Appl. 29, 3–15. doi:10.1002/pip.3371
Guldin, S., Kohn, P., Stefik, M., Song, J., Divitini, G., Ecarla, F., et al. (2013). Self-Cleaning Antireflective Optical Coatings. Nano Lett. 13, 5329–5335. doi:10.1021/nl402832u
Haider, A. J., Najim, A. A., and Muhi, M. A. H. (2016). TiO2/Ni Composite as Antireflection Coating for Solar Cell Application. Opt. Commun. 370, 263–266. doi:10.1016/j.optcom.2016.03.034
Ho, W.-J., Lee, Y.-Y., Lin, C.-H., and Yeh, C.-W. (2015). Performance Enhancement of Plasmonics Silicon Solar Cells Using Al2O3/In NPs/TiO2 Antireflective Surface Coating. Appl. Surf. Sci. 354, 100–105. doi:10.1016/j.apsusc.2015.03.009
Hocine, D., Belkaid, M. S., Pasquinelli, M., Escoubas, L., Simon, J. J., Rivière, G. A., et al. (2013). Improved Efficiency of Multicrystalline Silicon Solar Cells by TiO2 Antireflection Coatings Derived by APCVD Process. Mater. Sci. Semiconductor Process. 16, 113–117. doi:10.1016/j.mssp.2012.06.004
Hocine, D., Belkaid, M. S., Pasquinelli, M., Escoubas, L., Torchio, P., and Moreau, A. (2015). Characterization of TiO2 Antireflection Coatings Elaborated by APCVD for Monocrystalline Silicon Solar Cells. Phys. Status Solidi C 12, 323–326. doi:10.1002/pssc.201400085
Huang, Z., Cai, C., Kuai, L., Li, T., Huttula, M., and Cao, W. (2018). Leaf-structure Patterning for Antireflective and Self-Cleaning Surfaces on Si-Based Solar Cells. Solar Energy 159, 733–741. doi:10.1016/j.solener.2017.11.020
Jiao, K. L., and Anderson, W. A. (1987). SiO2/TiO2 Double-Layer Antireflective Coating Deposited at Room Temperature for Metal/insulator/n-Si/p-Si Solar Cells. Solar Cells 22, 229–236. doi:10.1016/0379-6787(87)90046-9
Keshavarz Hedayati, M., and Elbahri, M. (2016). Antireflective Coatings: Conventional Stacking Layers and Ultrathin Plasmonic Metasurfaces, A Mini-Review. Materials 9, 497. doi:10.3390/ma9060497
Kim, Y. J., Lee, Y. H., Lee, M. H., Kim, H. J., Pan, J. H., Lim, G. I., et al. (2008). Formation of Efficient Dye-Sensitized Solar Cells by Introducing an Interfacial Layer of Long-Range Ordered Mesoporous TiO2 Thin Film. Langmuir 24, 13225–13230. doi:10.1021/la802340g
Klosek, S., and Raftery, D. (2001). Visible Light Driven V-Doped TiO2Photocatalyst and its Photooxidation of Ethanol. J. Phys. Chem. B 105, 2815–2819. doi:10.1021/jp004295e
Langlet, M., Burgos, M., Coutier, C., Jimenez, C., Morant, C., and Manso, M. (2001). Low Temperature Preparation of High Refractive Index and Mechanically Resistant Sol-Gel TiO2 Films for Multilayer Antireflective Coating Applications. J. Sol-Gel Sci. Techn. 22, 139–150. doi:10.1023/a:1011232807842
Leem, J. W., Su Yu, J., Jun, D.-H., Heo, J., and Park, W.-K. (2014). Efficiency Improvement of III-V GaAs Solar Cells Using Biomimetic TiO2 Subwavelength Structures with Wide-Angle and Broadband Antireflection Properties. Solar Energ. Mater. Solar Cell 127, 43–49. doi:10.1016/j.solmat.2014.03.041
Li, D., Wan, D., Zhu, X., Wang, Y., Han, Z., Han, S., et al. (2014). Broadband Antireflection TiO 2 -SiO 2 Stack Coatings with refractive-index-grade Structure and Their Applications to Cu(In,Ga)Se 2 Solar Cells. Solar Energ. Mater. Solar Cell 130, 505–512. doi:10.1016/j.solmat.2014.07.042
Li, J., Lu, Y., Lan, P., Zhang, X., Xu, W., Tan, R., et al. (2013). Design, Preparation, and Durability of TiO2/SiO2 and ZrO2/SiO2 Double-Layer Antireflective Coatings in Crystalline Silicon Solar Modules. Solar Energy 89, 134–142. doi:10.1016/j.solener.2012.12.011
Li, X., and He, J. (2013). Synthesis of Raspberry-like SiO2-TiO2 Nanoparticles toward Antireflective and Self-Cleaning Coatings. ACS Appl. Mater. Inter. 5, 5282–5290. doi:10.1021/am401124j
Liao, K., Chen, J., Xia, L., Zhong, S., and Luo, X. (2020). Study about the Effect of Antireflection Coating Stacks (TiO2-SiO2/SiO2/SiNx) on the Performances of Multicrystalline Silicon Solar Cells under the Encapsulation Condition. Opt. Mater. 109, 110318. doi:10.1016/j.optmat.2020.110318
Lien, S., Wuu, D., Yeh, W., and Liu, J. (2006). Tri-layer Antireflection Coatings (SiO2/SiO2-TiO2/TiO2) for Silicon Solar Cells Using a Sol-Gel Technique. Solar Energ. Mater. Solar Cell 90, 2710–2719. doi:10.1016/j.solmat.2006.04.001
Lin, W., Zheng, J., Yan, L., and Zhang, X. (2018). Sol-gel Preparation of Self-Cleaning SiO2-TiO2/SiO2-TiO2 Double-Layer Antireflective Coating for Solar Glass. Results Phys. 8, 532–536. doi:10.1016/j.rinp.2017.12.058
Liu, J.-J., Ho, W.-J., Lee, Y.-Y., and Chang, C.-M. (2014). Simulation and Fabrication of SiO2/graded-index TiO2 Antireflection Coating for Triple-junction GaAs Solar Cells by Using the Hybrid Deposition Process. Thin Solid Films 6. 585–590. doi:10.1016/j.tsf.2014.05.024
Loferski, J. J. (1993). The First Forty Years: A Brief History of the Modern Photovoltaic Age. Prog. Photovolt: Res. Appl. 1, 67–78. doi:10.1002/pip.4670010109
Manea, E., Pârvulescu, C. C., Purica, M., Budianu, E., and Comanescu, F. (2013). Antireflective Coatings with Nanostructured TiO2 Thin Films for Silicon Solar Cells. J. Nano Res. 21, 89–94. doi:10.4028/www.scientific.net/JNanoR.21.89
Mazur, M., Wojcieszak, D., Kaczmarek, D., Domaradzki, J., Song, S., Gibson, D., et al. (2016). Functional Photocatalytically Active and Scratch Resistant Antireflective Coating Based on TiO 2 and SiO 2. Appl. Surf. Sci. 380, 165–171. doi:10.1016/j.apsusc.2016.01.226
Medina-Valtierra, J., Frausto-Reyes, C., Ramírez-Ortiz, J., and Camarillo-Martínez, G. (2009). Self-Cleaning Test of Doped TiO2-Coated Glass Plates under Solar Exposure. Ind. Eng. Chem. Res. 48, 598–606. doi:10.1021/ie8008555
Miao, L., Su, L. F., Tanemura, S., Fisher, C. A. J., Zhao, L. L., Liang, Q., et al. (2013). Cost-effective Nanoporous SiO2-TiO2 Coatings on Glass Substrates with Antireflective and Self-Cleaning Properties. Appl. Energ. 112, 1198–1205. doi:10.1016/j.apenergy.2013.03.043
Muraca, A. R., Kershis, M. D., Camillone, N., and White, M. G. (2019). Ultrafast Dynamics of Acetone Photooxidation on TiO2(110). J. Chem. Phys. 151, 161103. doi:10.1063/1.5122269
Nakajima, A., Hashimoto, K., Watanabe, T., Takai, K., Yamauchi, G., and Fujishima, A. (2000). Transparent Superhydrophobic Thin Films with Self-Cleaning Properties. Langmuir 16, 7044–7047. doi:10.1021/la000155k
Nakata, K., Sakai, M., Ochiai, T., Murakami, T., Takagi, K., and Fujishima, A. (2011). Antireflection and Self-Cleaning Properties of a Moth-Eye-like Surface Coated with TiO2Particles. Langmuir 27, 3275–3278. doi:10.1021/la200438p
Naufal, B., Ullattil, S. G., and Periyat, P. (2017). A Dual Function Nanocrystalline TiO2 Platform for Solar Photocatalysis and Self Cleaning Application. Solar Energy 155, 1380–1388. doi:10.1016/j.solener.2017.08.005
Noh, H. N., and Myong, S. Y. (2014). Antireflective Coating Using a WO3-TiO2 Nanoparticle Photocatalytic Composition for High Efficiency Thin-Film Si Photovoltaic Modules. Solar Energ. Mater. Solar Cell 121, 108–113. doi:10.1016/j.solmat.2013.10.038
Nowotny, M. K., Bak, T., and Nowotny, J. (2006). Electrical Properties and Defect Chemistry of TiO2Single Crystal. I. Electrical Conductivity. J. Phys. Chem. B 110, 16270–16282. doi:10.1021/jp0606210
O’Regan, B., and Grätzel, M. (1991). A Low-Cost, High-Efficiency Solar Cell Based on Dye-Sensitized Colloidal TiO 2 Films. Nature 353, 737–740. doi:10.1038/353737a0
Pae, S. R., Byun, S., Kim, J., Kim, M., Gereige, I., and Shin, B. (2018). Improving Uniformity and Reproducibility of Hybrid Perovskite Solar Cells via a Low-Temperature Vacuum Deposition Process for NiOx Hole Transport Layers. ACS Appl. Mater. Inter. 10, 534–540. doi:10.1021/acsami.7b14499
Pakhanov, N. A., Andreev, V. M., Shvarts, M. Z., and Pchelyakov, O. P. (2018). State-of-the-art Architectures and Technologies of High-Efficiency Solar Cells Based on III-V Heterostructures for Space and Terrestrial Applications. Optoelectron.Instrument.Proc. 54, 187–202. doi:10.3103/s8756699018020115
Paz, Y., Luo, Z., Rabenberg, L., and Heller, A. (1995). Photooxidative Self-Cleaning Transparent Titanium Dioxide Films on Glass. J. Mater. Res. 10, 2842–2848. doi:10.1557/JMR.1995.2842
Prabhu, S., Cindrella, L., Joong Kwon, O., and Mohanraju, K. (2017). Superhydrophilic and Self-Cleaning rGO-TiO 2 Composite Coatings for Indoor and Outdoor Photovoltaic Applications. Solar Energ. Mater. Solar Cell 169, 304–312. doi:10.1016/j.solmat.2017.05.023
Purcar, V., Rădiţoiu, V., Dumitru, A., Nicolae, C.-A., Frone, A. N., Anastasescu, M., et al. (2019). Antireflective Coating Based on TiO2 Nanoparticles Modified with Coupling Agents via Acid-Catalyzed Sol-Gel Method. Appl. Surf. Sci. 487, 819–824. doi:10.1016/j.apsusc.2019.02.256
Qiu, B., Zhou, Y., Ma, Y., Yang, X., Sheng, W., Xing, M., et al. (2015). Facile Synthesis of the Ti3+ Self-Doped TiO2-Graphene Nanosheet Composites with Enhanced Photocatalysis. Sci. Rep. 5, 8591. doi:10.1038/srep08591
Quan, Y.-Y., and Zhang, L.-Z. (2017). Experimental Investigation of the Anti-dust Effect of Transparent Hydrophobic Coatings Applied for Solar Cell Covering Glass. Solar Energ. Mater. Solar Cell 160, 382–389. doi:10.1016/j.solmat.2016.10.043
Rad, A. S., Afshar, A., and Azadeh, M. (2020). Anti-reflection and Self-Cleaning Meso-Porous TiO2 Coatings as Solar Systems Protective Layer: Investigation of Effect of Porosity and Roughness. Opt. Mater. 107, 110027. doi:10.1016/j.optmat.2020.110027
Richards, B. S. (2003). Single-material TiO2 Double-Layer Antireflection Coatings. Solar Energ. Mater. Solar Cell 79, 369–390. doi:10.1016/S0927-0248(02)00473-7
Ritchie, H., and Roser, M. (2020). Renewable Energy Our World In Data. Available at: https://ourworldindata.org/renewable-energy (Accessed March 20, 2021).
Sagar, R., and Rao, A. (2021). Nanoscale TiO2 and Ta2O5 as Efficient Antireflection Coatings on Commercial Monocrystalline Silicon Solar Cell. J. Alloys Comp. 862, 158464. doi:10.1016/j.jallcom.2020.158464
Said, S. A. M., Al-Aqeeli, N., and Walwil, H. M. (2015). The Potential of Using Textured and Anti-reflective Coated Glasses in Minimizing Dust Fouling. Solar Energy 113, 295–302. doi:10.1016/j.solener.2015.01.007
Salvaggio, M. G., Passalacqua, R., Abate, S., Perathoner, S., Centi, G., Lanza, M., et al. (2016). Functional Nano-Textured Titania-Coatings with Self-Cleaning and Antireflective Properties for Photovoltaic Surfaces. Solar Energy 125, 227–242. doi:10.1016/j.solener.2015.12.012
San Vicente, G., Morales, A., Germán, N., Suarez, S., and Sánchez, B. (2012). SiO2/TiO2 Antireflective Coatings With Photocatalytic Properties Prepared by Sol-Gel for Solar Glass Covers. J. Solar Energ. Eng. 134, 041011. doi:10.1115/1.4007298
San Vicente, G., Morales, A., and Gutierrez, M. T. (2001). Preparation and Characterization of Sol-Gel TiO2 Antireflective Coatings for Silicon. Thin Solid Films 391, 133–137. doi:10.1016/S0040-6090(01)00963-4
San Vicente, G., Morales, A., and Gutiérrez, M. T. (2002). Sol-gel TiO2 Antireflective Films for Textured Monocrystalline Silicon Solar Cells. Thin Solid Films 403–404, 335–338. doi:10.1016/S0040-6090(01)01572-3
Shahiduzzaman, M., Yonezawa, K., Yamamoto, K., Ripolles, T. S., Karakawa, M., Kuwabara, T., et al. (2017). Improved Reproducibility and Intercalation Control of Efficient Planar Inorganic Perovskite Solar Cells by Simple Alternate Vacuum Deposition of PbI2 and CsI. ACS Omega 2, 4464–4469. doi:10.1021/acsomega.7b00814
Sheng, X., Shen, L., Kim, T., Li, L., Wang, X., Dowdy, R., et al. (2013). Doubling the Power Output of Bifacial Thin-Film GaAs Solar Cells by Embedding Them in Luminescent Waveguides. Adv. Energ. Mater. 3, 991–996. doi:10.1002/aenm.201201064
Singh, G., and Verma, S. S. (2019). Design and Analysis of Thin Film GaAs Solar Cells Using Silver Nanoparticle Plasmons. Photon. Nanostructures - Fundamentals Appl. 37, 100731. doi:10.1016/j.photonics.2019.100731
Singh, R., Kumar, M., Saini, M., Singh, A., Satpati, B., and Som, T. (2017). Growth of TiO2 Thin Films on Chemically Textured Si for Solar Cell Applications as a Hole-Blocking and Antireflection Layer. Appl. Surf. Sci. 418, 225–231. doi:10.1016/j.apsusc.2017.01.307
Soklič, A., Tasbihi, M., Kete, M., and Štangar, U. L. (2015). Deposition and Possible Influence of a Self-Cleaning Thin TiO2/SiO2 Film on a Photovoltaic Module Efficiency. Catal. Today 252, 54–60. doi:10.1016/j.cattod.2014.10.021
Suárez, L., Pulgarin, C., Giannakis, S., Bensimon, M., and Kiwi, J. (2017). New Evidence for Disinfection, Self-Cleaning and Pollutant Degradation Mediated by GF-TiO 2 -Cu Mats under Solar/visible Light in Mild Oxidative Conditions. J. Photochem. Photobiol. A: Chem. 346, 351–363. doi:10.1016/j.jphotochem.2017.06.025
Thomas, R. E., Varma, S., Waechter, D., Dodd, C. X., and Das, S. R. (1989). Screen Printed Ta2Os and TiO2 Antireflection Coatings for Crystalline and Polycrystalline Silicon Solar Cells. Can. J. Phys. 67, 430–434. doi:10.1139/p89-076
Wan, D., Chen, H.-L., Tseng, T.-C., Fang, C.-Y., Lai, Y.-S., and Yeh, F.-Y. (2010). Antireflective Nanoparticle Arrays Enhance the Efficiency of Silicon Solar Cells. Adv. Funct. Mater. 20, 3064–3075. doi:10.1002/adfm.201000678
Wang, P., Wang, J., Wang, X., Yu, H., Yu, J., Lei, M., et al. (2013). One-step Synthesis of Easy-Recycling TiO2-rGO Nanocomposite Photocatalysts with Enhanced Photocatalytic Activity. Appl. Catal. B: Environ. 132–133, 452–459. doi:10.1016/j.apcatb.2012.12.009
Wang, X., and Shen, J. (2010). Sol-gel Derived Durable Antireflective Coating for Solar Glass. J. Sol-gel Sci. Technol. 53, 322–327. doi:10.1007/s10971-009-2095-y
Wang, Y., Wu, J., Wang, H., and Chen, R. (2015). Effective Balance of Antireflection and Self-Cleaning Properties via Hollow Silica Nanospheres-Based Surface Coated with Scattered Titania Nanoparticles. Solar Energy 122, 763–772. doi:10.1016/j.solener.2015.10.003
Weng, K.-W., and Huang, Y.-P. (2013). Preparation of TiO 2 Thin Films on Glass Surfaces with Self-Cleaning Characteristics for Solar Concentrators. Surf. Coat. Techn. 231, 201–204. doi:10.1016/j.surfcoat.2012.06.058
Xu, Y. J., Liao, J. X., Cai, Q. W., and Yang, X. X. (2013). Preparation of a Highly-Reflective TiO2/SiO2/Ag Thin Film with Self-Cleaning Properties by Magnetron Sputtering for Solar Front Reflectors. Solar Energ. Mater. Solar Cell 113, 7–12. doi:10.1016/j.solmat.2013.01.034
Ye, L., Zhang, Y., Zhang, X., Hu, T., Ji, R., Ding, B., et al. (2013). Sol-gel Preparation of SiO2/TiO2/SiO2-TiO2 Broadband Antireflective Coating for Solar Cell Cover Glass. Solar Energ. Mater. Solar Cell 111, 160–164. doi:10.1016/j.solmat.2012.12.037
Yu, J., Zhou, M., Yu, H., Zhang, Q., and Yu, Y. (2006). Enhanced Photoinduced Super-hydrophilicity of the Sol-Gel-Derived TiO2 Thin Films by Fe-Doping. Mater. Chem. Phys. 95, 193–196. doi:10.1016/j.matchemphys.2005.09.021
Yuan, J., Yan, S., Xiao, Z., and Hu, J. (2020). Multifunctional Closed-Pore Silica/titania Composite Antireflective Coatings Based on Self-Assembly Process. Mater. Chem. Phys. 250, 123023. doi:10.1016/j.matchemphys.2020.123023
Zhang, W., Hu, K., Tu, J., Aierken, A., Xu, D., Song, G., et al. (2021). Broadband Graded Refractive index TiO2/Al2O3/MgF2 Multilayer Antireflection Coating for High Efficiency Multi-junction Solar Cell. Solar Energy 217, 271–279. doi:10.1016/j.solener.2021.01.012
Zhang, X., Fujishima, A., Jin, M., Emeline, A. V., and Murakami, T. (2006). Double-Layered TiO2−SiO2Nanostructured Films with Self-Cleaning and Antireflective Properties. J. Phys. Chem. B 110, 25142–25148. doi:10.1021/jp064442u
Keywords: antireflection, coatings, renewable energy, self-cleaning, solar cells, titania
Citation: Afzal A, Habib A, Ulhasan I, Shahid M and Rehman A (2021) Antireflective Self-Cleaning TiO2 Coatings for Solar Energy Harvesting Applications. Front. Mater. 8:687059. doi: 10.3389/fmats.2021.687059
Received: 28 March 2021; Accepted: 25 May 2021;
Published: 07 June 2021.
Edited by:
Yew Hoong Wong, University of Malaya, MalaysiaReviewed by:
Sharel Pei San E, Teesside University, United KingdomKuan Yew Cheong, Universiti Sains Malaysia Engineering Campus, Malaysia
Copyright © 2021 Afzal, Habib, Ulhasan, Shahid and Rehman. This is an open-access article distributed under the terms of the Creative Commons Attribution License (CC BY). The use, distribution or reproduction in other forums is permitted, provided the original author(s) and the copyright owner(s) are credited and that the original publication in this journal is cited, in accordance with accepted academic practice. No use, distribution or reproduction is permitted which does not comply with these terms.
*Correspondence: Adeel Afzal, YWFAYWFmemFsLmNvbQ==