- State Key Laboratory of Precision Electronics Manufacturing Technology and Equipment, Guangdong University of Technology, Guangzhou, China
The piezoelectric materials, such as ceramics, crystals, and films, have wide applications in the mechanical industry, medical imaging, electronic information, and ultrasonic devices, etc. Generally, adding oxide dopants, or introducing new solid solutions to form the morphotropic phase boundary of the piezoelectric materials were common strategies to enhance the electric properties. In recent decades, rare-earth elements doped piezoelectric materials have attracted much attention due to their multifunctional performances combining piezoelectric and photoluminescence properties, which has potential applications in ultrasonics, electronics, automatic control, machinery and optoelectronic fields. An overview of the recent investigations and perspectives on rare-earth doped piezoelectric ceramics, single crystals, and films were presented.
Introduction
Rare-earth (RE) elements contain rich structures and energy levels and exhibit excellent optical, electrical, magnetic, and nuclear properties, which were exploited in functional materials to enhance their properties and broaden applications (Singh et al., 2015; Steudel et al., 2015).
As one of the important functional materials, piezoelectric materials play a significant role in the fields of medicine, acoustics, machinery, and electronics due to the ability of mutual conversion between mechanical energy and electric energy. The electric performances of piezoelectric materials are affected by compositions, microstructure, and lattice defects like oxygen vacancies. Thus, extensive investigations have been carried out to enhance the properties of the piezoelectric materials by introducing new solid solutions or adding oxide dopants. The rare-earth ion doping may substitute the original ions and cause the formation of vacancies so that the distortion of the crystal lattices may affect the electric properties due to their unique ionic radius and different chemical valence (Qiu and Hng, 2002; Hagemann and Hennings, 2010; Singh et al., 2011). There are 17 members in the family of rare-earth elements, including 15 lanthanides (La, Ce, Pr, Nd, Pm, Sm, Eu, Gd, Tb, Dy, Ho, Er, Tm, Yb, Lu), Sc, and Y. Figure 1 shows the rare-earth elements doped piezoelectric materials and relative properties based on the reported works. Some piezoelectric materials with certain rare-earth doping can exhibit obvious photoluminescence properties because of the ladder-shaped 4f energy level and relatively long metastable state of some rare-earth ions (Tsonev, 2008; Sun et al., 2011; Pieter, 2013; Pieter, 2017). Figure 2A shows the main energy flow paths during the sensitization of lanthanide (Ln) luminescence. The energy levels of Pr3+, Nd3+, Sm3+, Eu3+, Tb3+, Dy3+, Ho3+, Er3+, Tm3+, and Yb3+ ions are also given schematically in Figure 2B. The electrons in the singlet (S0) ground state absorb energy and then jump to the singlet (S1) excited state. Besides, the excitation energy of (S1) state is transferred to the triplet state (T) based on intersystem crossing firstly and to the 4f states subsequently. Finally, the corresponding emission of lanthanide ions can be acquired (Sun et al., 2006; Dang et al., 2008).
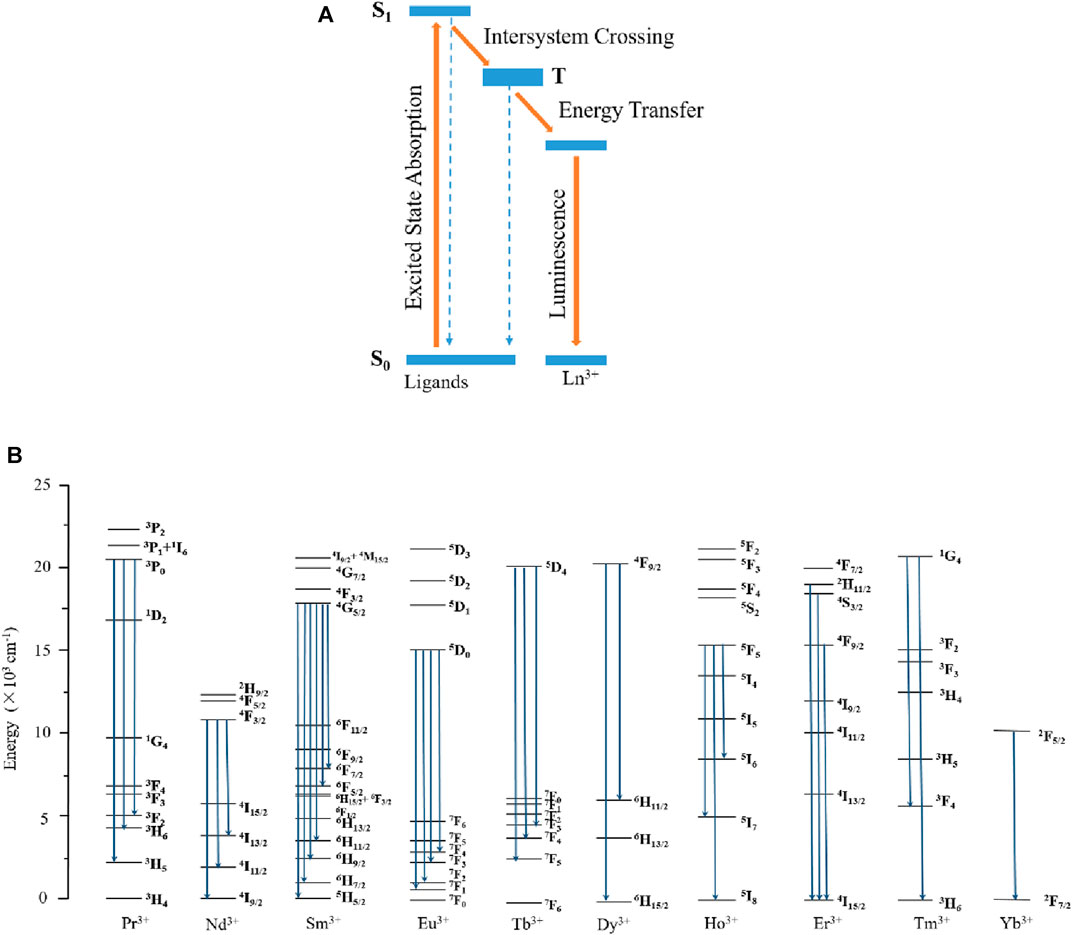
FIGURE 2. (A) The diagram of the main energy flow paths during the sensitization of lanthanide luminescence via the ligands. (B) The diagram of the energy levels of Pr3+, Nd3+, Sm3+, Eu3+,Tb3+, Dy3+,Ho3+, Er3+, Tm3+, and Yb3+ ions.
Although the rare-earth ion doped piezoelectric materials have attracted much attention owing to their outstanding electric and luminescence performance, there are few reviews reported in this research field. Most reviews are focused on a certain type of piezoelectric material or a fabricating method. Zheng et al. (2018) addressed the lead-free perovskite piezoelectric bulk materials. The relationships among the phase boundaries, domain configurations, and electrical properties in lead-free materials were discussed. Liu (2015) reviewed the progress on lead-free textured piezoelectric ceramics with an enhancement of the piezoelectric property. Chen et al. (2020a) paid attention to the progress of piezoelectric materials fabricated by additive manufacturing. In this paper, the effect of rare-earth doping on the electrical and optical properties of piezoelectric ceramics, crystals, and films are reviewed and discussed.
Rare-Earth Doped Piezoelectric Ceramics
Rare-Earth Doped Lead-Based Piezoelectric Ceramics
Piezoelectric ceramics are a kind of piezoelectric materials with a wide range of applications due to the characteristics of good electric properties, fine optical properties, low production cost, and simple preparation. In the past few decades, lead-based piezoelectric ceramics have dominated the market due to their good properties. In the following section, the rare-earth doped lead-based ceramics were summarized.
Rare-Earth Doped PZT-Based Ceramics
Pb(ZrxTi1−x)O3 (PZT) ceramics are the most studied and applied piezoelectric material. The piezoelectric constant d33 and Curie temperature Tc of PZT-5A ceramics can reach 375 pC/N and 365°C, respectively (Shrout and Zhang, 2007; Chen et al., 2018).
Shannigrahi et al. (2004) synthesized the PZT (60/40) ceramics doped with rare-earth (La, Nd, Sm, Eu, Gd, Dy, and Er) by sol-gel growth. Among them, the ceramic doped with La has the largest dielectric constant εr of 3,413, and the piezoelectric constant d33 and the remnant polarization Pr can reach 569 pC/N and 21.9 μC/cm2, respectively. Nevertheless, the Curie temperature Tc was lowest (∼156°C). The Dy doped ceramic has the highest Curie temperature Tc of 368°C. Khazanchi et al. (2005) reported Eu doped PZT (55/45) ceramics. The dielectric constant εr and the remnant polarization Pr of 2 mol% Eu doped samples increased to 1,132 and 26.05 μC/cm2, respectively. Pandey et al. (2009) developed Sm doped PZT (65/35) ceramics showing good electric properties, and the dielectric constant εr, the piezoelectric constant d33 and the planar electromechanical coupling coefficient kp of the 6 mol% Sm doped ceramic increased to 610, 172 pC/N, and 0.42, respectively. Perumal et al. (2019) reported that the Nd doped PZT (52/48) has good electrical properties (149 pC/N) and low conductivity (∼10−3 S/m). Guo et al. (2020) achieved Yb doped PZT based ceramics with good electric properties. The piezoelectric constant d33, planar electromechanical coupling coefficient kp, and the dielectric constant εr of the ceramics can reach 308 pC/N, 0.57, and 1,210, respectively. Kour et al. (2015) and Kour et al. (2016) reported that the piezoelectric constant d33 of the PZT ceramics with 10 mol% La doping was 381 pC/N. In conclusion, the rare-earth doped PZT ceramics exhibit good piezoelectric properties which are suitable for general applications, including sensors, actuators, and ultrasound transducers.
Except for the enhancement of electric performance after rare-earth doping, the optical properties also varied significantly. La-doped PZT (PLZT) transparent ceramics have also attracted great attention in fundamental research as well as in applications due to a wide variety of optical properties. Zhang et al. (2006) observed three emission peaks at 915, 1,066, and 1,347 nm in the Nd doped PLZT ceramics under the excitation of a continuous wave diode laser. Zeng et al. (2014) prepared the Dy doped PLZT ceramics by hot-pressing sintering. It was shown that the Dy substituting decreased the coercive field Ec, but improved the optical transmittance and electro-optic effect. Especially, the transparency can reach as high as 67% at 632.8 nm. Zhao et al. (2014) reported that the intensity of the emission peak (1800 nm) in Tm doped PLZT ceramics was obvious and strong, while the emission intensity reduced when doped with Ho. Strikingly, a quite strong emission at 1900–2200 nm emerged, whose full width at half maxima (FWHM) was up to 200 nm, which indicated Ho/Tm co-doped PLZT ceramics had potential application prospect in tunable lasers around 2000 nm. Moreover, the structural and spectroscopic properties of Nd, Er, Yb doped and co-doped PLZT ceramics were also studied as potential laser materials in the near infrared region (Camargo et al., 2004; Camargo et al., 2005). These studies indicated that the transparent PLZT ceramics with rare-earth doping were suitable for photonic devices, optic sensors, and multifunctional optic devices, etc.
Furthermore, the Pb(Zn1/3Nb2/3)O3-Pb(Zr51Ti49)O3 (PZN-PZT) system has recently attracted much attention owing to their better piezoelectric properties (d33 = 550 pC/N, kp = 0.69) compared with traditional PZT ceramics (Vittayakorn et al., 2006; Zheng et al., 2014; Li et al., 2015a). Deng et al. (2010) reported that the piezoelectric coefficient d33, dielectric constant εr and electromechanical coupling factor kp of the La doped 0.3 Pb(Zn1/3Nb2/3)O3-0.7 Pb(Zr0.51Ti0.49)O3 can reach 845 pC/N, 4,088, and 0.70, respectively. Wang et al. (2012) reported that La doped 0.25 Pb(Zn1/3Nb2/3)O3-0.75 Pb(Zr0.53Ti0.47)O3 ceramics near the morphotropic phase boundary had good piezoelectric properties (d33 = 570 pC/N, kp = 0.60). Fan et al. (2014) found that introducing the proper amount of Li2CO3 and Sm2O3 into 0.3PZN-0.7PZT ceramics could not only effectively improve the sinter ability and reduce the sintering temperature (∼900°C), but also enhance the electric properties (d33 = 483 pC/N, εr = 2,524, tan δ = 0.0178).
Rare-Earth Doped PMN-PT Ceramics
Pb(Mg1/3Nb2/3)O3-PbTiO3 (PMN-PT) ceramic is another kind of typical lead-based ceramic, which has been widely applied in multilayer capacitors, actuators, electro-optical modulators, ultrasonic transducers, and infrared detectors due to the good electric properties (d33 = 663 pC/N, εr = 5,260) (Choi et al., 1989; Kamzina et al., 2010; Zhang et al., 2014a).
Li et al. (2018) found an ultrahigh piezoelectricity in the Sm doped 0.71 PMN-0.29 PT ceramics. The piezoelectric constant d33 can reach as high as 1,500 pC/N, and the dielectric constant εr can arrive at about 13,000. But the Curie temperature decreased to 89°C. Guo et al. (2019a) reported the enhancement of electric properties in the 0.72 PMN-0.28 PT ceramics doped with 2.5 mol% Eu. The piezoelectric constant d33, dielectric constant εr, and electromechanical coupling coefficient k33 of the ceramics can reach 1,420 pC/N, 12,200, and 0.78, respectively. Guo et al. (2019b) also developed Sm doped 0.4 Pb(Mg1/3Nb2/3)O3-0.248PbZrO3-0.352PbTiO3 ternary system ceramics. These ceramics possess a high piezoelectric constant (d33 = 910 pC/N), good dielectric constant (εr = 4,090), and relatively high Curie temperature (Tc = 184°C). The previous studies also proved that the optical properties of PMN-PT ceramics can be also significantly improved by rare-earth doping. Wei et al. (2010) achieved a much larger Kerr effect in the La doped 0.75 PMN-0.25 PT transparent ceramic, The Kerr effect (second-order electro-optic effect) refers to the phenomenon of inductive birefringence that is proportional to the square of the electric field, providing useful devices such as light valves, deflectors and displays (Uchino, 1995). The quadratic electro-optic coefficient of the ceramics can reach as high as 66 × 10−6 m2/V2. Wei et al. (2012a) and Wei et al. (2012b) reported that the transparency of the Er doped 0.75 PMN-0.25 PT ceramics can reach 65% from visible light to infrared band. Besides, three broad peaks at 950–1,060, 1,220–1,290, and 1420–1560 nm can be observed under the excitation of a 521 nm laser. Liu et al. (2016) acquired a strong yellow-green up-conversion photoluminescence in the Ho/Yb co-doped 0.67 PMN-0.33 PT ceramics under the excited of a 980 nm laser. Ma et al. (2018) reported La doped 0.88 PMN-0.12 PT transparent ceramics. The transparency in the near infrared region increased to 70% with 1.0 mol% La doping. Fang et al. (2021) reported that the transparency and quadratic electro-optic coefficient of the Sm doped 0.88 PMN-0.21 PT transparent ceramics can reach 69.6% and 35 × 10−6 m2/V2, respectively. Lv et al. (2019) obtained strong visible up-conversion emissions at 491, 529, 539, 623, 649, 685, 710, and 737 nm and near-infrared down-conversion emissions around 1,061 nm and 1006 nm in the Pr/Yb co-doped 0.75 PMN-0.25 PT ceramics under the excitation of a 980 nm laser. These studies indicate that the rare-earth doped PMN-PT ceramics are promising multifunctional materials due to the improved electric properties, electro-optic effects, and various photoluminescence properties.
Rare-Earth Doped BS-PT Ceramics
The PMN-PT ceramics have good electric properties. However, the low Curie temperature (159°C) limits their applications. By contrast, the BiScO3-PbTiO3 (BS-PT) ceramics exhibit a high Curie temperature (Tc ∼ 450°C) and good piezoelectric property (d33 ∼ 460 pC/N) near the morphotropic phase boundary (Inaguma et al., 2004; Zhang et al., 2005). Yao et al. (2010) found the enhancement of the electric properties (d33 = 361 pC/N, kp = 0.40) in the La doped BS-PT ceramics. The highest Curie temperature can reach 467°C. Politova et al. (2011) reported a large piezoelectric coefficient (d33 = 350 pC/N) and the piezoelectric coupling coefficient (kt = 0.68) in the Nd doped BS-PT ceramics. Li et al. (2009) reported a high Curie temperature (Tc = 490°C) and good piezoelectric properties (d33 = 147 pC/N, kp = 0.28) in the Y doped BS-PT ceramics. The combination of high Curie temperature and good piezoelectric properties suggested that these rare-earth modified ceramics can be a promising candidate for high-temperature actuators and transducers. Table 1 shows the properties of some lead-based piezoelectric ceramics with rare-earth doping.
Rare-Earth Doped Lead-free Ceramics
Due to the increasing concern of lead pollution to the environment, it is significant and urgent to develop lead-free piezoelectric ceramics with good properties. There are three typical lead-free piezoelectric ceramics that have gained a lot of attention, including (Na0.5Bi0.5)TiO3-BaTiO3 (NBT-BT) ceramic (K0.5Na0.5)NbO3 (KNN) based ceramic, and BaTiO3 (BT) ceramic, which are considered as possible substitutes for lead-based ceramics. The following section summaries the lead-free piezoelectric ceramics with rare-earth doping.
Rare-Earth Doped BNT-BT Ceramic
BNT-BT ceramic is one of the most widely used lead-free piezoelectric ceramics owing to the good piezoelectric constant and high Curie temperature (d33 > 100 pC/N, Tc >280°C) (Panda, 2009; Jo et al., 2011). Li et al. (2004) reported the enhancement electric properties of the BNT-6BT ceramics doped with one at% La, showing the piezoelectric constant d33 increased from 117 to 125 pC/N, but the thick electromechanical coupling coefficient kt decreased slightly from 0.43 to 0.38. Liu et al. (2008) reported that the BNT-6BT ceramics doped with 0.4 wt% CeO2 had better performances compared to pure BNT-6BT ceramics. The piezoelectric constant d33 and thick electromechanical coupling coefficient kt of the doped ceramics were 120 pC/N and 0.52, respectively. Peng et al. (2010a), Peng et al. (2010b) and Peng et al. (2010c) reported the enhancement of electric properties in the Sm doped BNT-6BT ceramics. The piezoelectric constant d33 and coupling coefficient kp of the ceramics increased to 202 pC/N and 0.3, respectively. In the same year, this group found that Nd doping can also improve the electric properties of BNT-6BT ceramics. The piezoelectric constant d33 and planar electromechanical coupling coefficient kp of the ceramics can reach 175 pC/N and 0.31, respectively. Besides, this group also fabricated La2O3 doped BNT-6BT ceramics. After 0.6 wt% La2O3 doping, the ceramics with showed good piezoelectric properties (d33 = 167 pC/N, kp = 0.30).
Rare-earth doped BNT-BT ceramics also possess great photoluminescence properties. Jiang et al. (2018) reported the piezoelectric constant d33 and planar electromechanical coupling coefficient kp of the 2.5 mol% Dy doped BNT-6BT ceramic can reach 190 pC/N and 0.372, respectively. Moreover, the ceramics excited at 426 nm also exhibited strong emissions at 478 and 575 nm. This study showed the Dy doped BNT-BT ceramics had simultaneously good piezoelectricity and photoluminescence, suggesting a promising application in the electro-optic devices. Chi et al. (2015) observed visible up-conversion luminescence at 532, 540, and 600 nm, as well as near infrared (1440–1660 nm) and mid-infrared (2620–2840 nm) broadband down-conversion luminescence in the Er doped BNT-7BT ceramics under the excitation of 980 nm. Li et al. (2019a) achieved a strong orange luminescence in the Sm doped BNT-12BT ceramics when excited by blue light, and the emission intensity depends largely on the doping concentration.
Rare-Earth Doped KNN-Based Ceramics
KNN ceramics with high Curie temperature (Tc ∼ 400°C) and good electric properties (d33 ∼ 80 pC/N, kp ∼ 0.36) have been deemed as potential candidates for lead-based piezoelectric ceramics (Saito et al., 2004; Birol et al., 2006; Jaeger. 2010).
Du et al. (2017) studied the effect of the KNN ceramics doped with different rare-earth elements (Dy, Er, Eu, and Pr). The piezoelectric constant d33 increased slightly after doping, (KNN-Dy: 95 pC/N, KNN-Er: 91 pC/N, KNN-Eu: 84 pC/N and KNN-Pr: 94 pC/N). Wu et al. (C2017) fabricated Er doped KNN based ceramics with the piezoelectric constant of 70–90 pC/N, and the dielectric constant and loss is about 1,400 and 0.03, respectively. Zhai et al. (2019) reported good piezoelectric and ferroelectric properties in the KNN ceramics doped with Tb and Tm. The d33(Tb) and d33(Tm) can reach 140 pm/V and 127 pm/V, respectively.
In addition to studying the electric properties, the optical performances of rare-earth doped KNN ceramics were also investigated extensively. Yang et al. (2017) reported that the transparency of 1.5 mol% La2O3 doped KNN ceramic can reach as high as 74%). Geng et al. (2017) reported that Eu doped K0.47Na0.47Li0.06Nb0.94Bi0.06O3 ceramics presented high transmittance both in the near-infrared and middle infrared regions (close to 100%). Besides, the ceramics were efficiently excited by near-ultraviolet and blue light to realize strong reddish luminescence. Sun et al. (2012), and Sun et al. (2014) observed strong green (528 nm) and red (617 and 650 nm) emissions in the Pr doped KNN ceramics under the excitation of 450 nm. Two years later, this group found strong green (510–590 nm) and a relatively weak red (645–695 nm) emissions in the Er doped KNN ceramics under the 980 nm excitation, and it is possible to modulate the ratio of red to green emission by adjusting the Er concentration. Similarly, Wu et al. (2013), Wu et al. (2015a), and Wu et al. (2015b) found that the Er doped KNN ceramics fabricated by sol-gel method exhibited luminescent bands at 527, 548, 660, and 487 nm under the excitation of 980 nm. Besides, Er doping is beneficial to the formation of fine and uniform grains, which helps to enhance the up-conversion efficiency and luminous efficiency. Furthermore, seven down conversion emission bands (530, 548, 605, 618, 650, 672, and 740 nm) could be observed in the Er and Pr co-doped KNN ceramics under the excitation of 485 nm. Besides, three up-conversion emission bands (510–537 nm, 537–585 nm, and 640–690 nm), and two down-conversion emission bands (1,400–1700 nm and 2,600–2,850 nm) were found in the Er and Yb co-doped KNN ceramics. Zhang et al. (2014b) also achieved a strong orange emission in the Sm doped KNN ceramics under the excitation from 400 to 500 nm. Li et al. (2020a) also observed a bright photoluminescence with a strong orange emission in the Sm-doped 0.96(K0.48Na0.52) (Nb0.95Sb0.05)-0.04Bi0.5(Na0.82K0.18)0.5ZrO3 ceramics under visible light irradiation.
Rare-Earth Doped BT Ceramic
BT ceramic has received much attention due to the high dielectric constant, high resistivity and outstanding insulation performance, which has been extensively applied in positive temperature coefficient of resistivity (PTCR) thermistors, multilayer ceramic capacitors (MLCC), piezoelectric devices, optoelectronic elements, and semiconductors (Zhou et al., 2001). Among them, the application of MLCC is extremely wide, for improving the dielectric constant to meet the requirements of high capacitance. It is effective in significantly increasing the dielectric constant of BT ceramics with rare-earth doping (Caballero et al., 2000; Morrison et al., 2001).
Sun et al. (2010) reported the enhancement of dielectric properties in the Sm doped BT ceramics. Sm doping can inhibit the growth of the crystal grains and make the BT ceramics possess semiconductor characteristics. Li et al. (2012) achieved a large dielectric constant (70,000
Thus, rare-earth doped piezoelectric ceramics can effectively modify the electric properties and introduce excellent photoluminescence properties, which expanded the applications of piezoelectric ceramics greatly, such as actuating device, ultrasonic transducers, electro-optical deflector, potential phosphor, and multifunctional optoelectronics. Table 2 summarizes the properties of some lead-free based piezoelectric ceramics with rare-earth doping.
Rare-Earth Doped Piezoelectric Single Crystals
Rare-Earth Doped Lead-Based Single Crystals
In order to further improve the properties of the piezoelectric materials, the growth of piezoelectric single crystals has drawn wide concern. The discovery of relaxor-based ferroelectric single crystals represented by Pb(Mg1/3Nb2/3)O3-PbTiO3 (PMN-PT) and Pb(Zn1/3Nb2/3)O3-PbTiO3 (PZN-PT) are considered as a revolutionary breakthrough of piezoelectric materials, which tremendously improved the electric properties (d33 = 1780 pC/N) of piezoelectric materials compared to commercial piezoelectric PZT-5H ceramics (590 pC/N) (Kim et al., 2010).
Li et al. (2019b) reported a large piezoelectric constant d33 in the Sm doped PMN-PT single crystals, and the values (exceeding 3,400 pC/N) increased more than double compared with the Sm doped PMN-PT ceramics (1,510 pC/N). The Sm doped single crystals also showed improved compositional uniformity along the crystal boule. Furthermore, the electric field-induced strain can reach 30%, which is almost 90% higher than that of undoped PMN-PT single crystals. This study provided commercial opportunities for high-performance piezoelectric applications. Besides, PZN-9PT piezoelectric single crystal showed excellent electric properties (d33 > 1500pC/N, k33 > 90%) near the morphotropic phase boundary (Kuwata et al., 1982). However, the low coercive field (Ec ∼ 3.5 kV/cm) and low Curie temperature (Tc ∼ 170°C) limit the application of PZN-PT single crystal in high-power and high-temperature environments (Rajan et al., 2007). Xi et al. (2016a) and Xi et al. (2016b) reported a significantly enhanced coercive field Ec (11.6 kV/cm) in the Er doped PZN-9PT crystal. The Curie temperature Tc was around 178°C. Furthermore, the green and red up-conversion emission bands can be obtained in the ceramic under the 980 nm excitation. The group also reported the enhancement of the coercive field (Ec = 5.9 kV/cm), remnant polarization (Pr = 38.40 μC/cm2) and Curie temperature (Tc = 175°C) in the Ho doped PZN-9PT crystal. Li et al. (2019c) also prepared Tm/Yb co-doped PZN-9PT crystal with large coercive field Ec of 12.1 kV/cm, and the value is nearly four times higher than that of PZN-9PT crystal. The Curie temperature Tc was increased to 182.5°C. Under a 980 nm laser excitation, the modified crystals produce up-conversion with three colors [blue (480 nm), strong near-infrared (804 nm), and weak red (652 nm)]. Chen et al. (2020b) and Chen et al. (2020c) reported the coercive field Ec of Eu doped PZN-9PT crystal significantly increased to 11.0597 kV/cm, and it is triple higher than that of pure PZN-9PT crystal. Besides, under the excitation of 464 nm, four broadband peaks appear at around 593 nm, 613 nm, 657 nm, and 716 nm, respectively. Furthermore, the group also achieved a high Curie temperature (Tc = 250°C) and large coercive field (Ec = 11.0597 kV/cm) in the 0.15 Pb(Er1/2Nb1/2)O3-0.63 Pb(Zn1/3Nb2/3)O3-0.22PbTiO3 single crystal, and the crystal also exhibited a strong green light excited by a 980 nm laser. As mentioned above, the addition of rare-earth not only improves the temperature and electric field stability of PZN-PT single crystals but also induces photoluminescence properties, which gives the rare-earth doped PZN-PT single crystals possibilities for multifunctional optoelectronic device applications.
Rare-Earth Doped Lead-free Single Crystals
Na0.5Bi0.5TiO3 (BNT)-based lead-free crystals were reported to have superior electric properties, large band gap and high near-visible light absorption coefficient (Hiruma et al., 2010; Li et al., 2015b). In recent years, the electric and optical properties of the rare-earth doped BNT single crystals have been studied. Zhang et al. (2014c) prepared an Eu doped BNT single crystal by top-seeded solution growth, and observed that the crystal presented orange and red emissions under the excitation of 611 nm. The piezoelectric constant d33 and remnant polarization Pr of the crystal increased to 86 pC/N and 7.38 μC/cm2, respectively. Jiang et al. (2018) achieved an extremely strong green emission in the Er doped BNT single crystal under the 980 nm near-infrared excitation. But Er doping led to a slight decreasing of dielectric constant, dielectric loss and transmittance. He et al. (2013) reported high transparency Nd doped NBT single crystal by a top seeded solution growth method. The transparency reached more than 50% above 450 nm, and a strong emission band around 1,066 nm was found under laser excitation at 808 nm. These research results may help to extend various biomedical applications including intravascular imaging, photoacoustic imaging, and microbeam applications.
Rare-Earth Doped Piezoelectric Films
Compared to piezoelectric crystal and ceramics, piezoelectric films can greatly improve the operating frequency due to unlimited size. Currently, piezoelectric films such as PZT and Bi4Ti3O12 (BIT) thin films are widely applied in ferroelectric dynamic random-access memory, room temperature infrared detectors, piezoelectric motors, ultrasonic detectors, film capacitors and integrated optical waveguide devices (Xie et al., 2006).
Rare-Earth Doped PZT Films
Majumder et al. (2001) investigated rare-earth (Nd, Gd, and Ce) doped PZT (53/47) thin films by sol-gel technique, realizing an improvement of ferroelectric and dielectric properties of the PZT thin films with Ce and Gd. Nd doping was effective to increase the retained switchable polarization of PZT from 63 to 84%. Yu et al. (2003) fabricated the Eu doped PZT (52/42) thin films with an improved polarization and low leakage current by a sol-gel method. Nakaki et al. (2004) studied the effect of the PZT (40/60) thin films doped with various rare-earth elements (Y, Dy, Er, and Yb). The remnant polarization Pr of the films increased to 26 (PZT-Y), 25 (PZT-Dy) and 26 μC/cm2 (PZT-Er), respectively, while Yb doping degraded the remnant polarization Pr down to 16 μC/cm2. Pandey et al. (2007) reported the remnant polarization and dielectric constant of the Sm substituted PZT (65/35) thin films can reach about 52 μC/cm2 and 1,220, respectively. Moreover, the transparency of the film was close to 80% at 1200 nm. Rath et al. (2019) reported the enhancement of piezoelectric constant d33 (more than 130 pm/V) in La doped PZT film. Zhang et al. (2021) achieved a large remnant polarization (22.73 μC/cm2) in the 2 mol% Dy doped PZT (60/40) thin films. Moreover, the films also exhibited a significantly reduced leakage current density. All these studies indicated that rare-earth doping is one promising technique for improving the ferroelectric property of PZT film to meet a wide range of application requirements.
Rare-Earth Doped Lead-free Films
Due to the fatigue-free characteristics, the BIT films have been widely used in special applications, typically like nonvolatile ferroelectric random access memory. Kim et al. (2002) reported the enhancement of ferroelectric properties in the Eu doped BIT films. The remnant polarization Pr of the films was about 30 μC/cm2. Zhang et al. (2003) reported that the remnant polarization of Nd doped BIT films increased double (8.8 μC/cm2) compared to the pure BIT films. Ruan et al. (2008) reported a high optical transparency (>80%) in the Eu doped BIT films, and the emission spectra of the films presented two peaks at 594 and 617 nm under the excitation of 350 nm. Moreover, the films exhibited high fatigue resistance after 1010 switching cycles. Gao et al. (2009) observed two strong green emission bands centered at 524 and 545 nm and a weak red emission band centered at 667 nm in the Er/Yb co-doped BIT films under the excitation of 980 nm. Ding et al. (2011) observed two emission bands centered at 546 and 656 nm in the Ho/Yb co-doped BIT thin films under the excitation of 980 nm.
In addition to the research of rare-earth doped piezoelectric thin films, Xu et al. (2020) have also made remarkable achievements in the thick films. They obtained high d33 (343 pC/N), large Pr (12.45 μC/cm2) and low Ec (0.63 kV/mm) in the Er doped Ba0.85Ca0.15Ti0.9Zr0.1O3 textured thick films. Besides, two strong green emission bands centered at 525 and 550 nm and one weak red emission band of 660 nm were acquired under the excitation of a 980 nm laser. These results showed that rare-earth doped films significantly enhanced the electrical properties as well as the photoluminescence performances, which has high potential for multifunctional film devices applications.
Discussions
Electrical Properties
The rare-earth doping may influence the electrical and optical performances of the piezoelectric materials significantly. Two tables (Tables 1, 2) and Figure 3 summary the piezoelectric properties of the rare-earth doped piezoelectric ceramics with perovskite (ABO3) structure. It can be found that Sm doped PMN-PT ceramics exhibited the highest piezoelectric constants (1,510 pC/N) among the rare-earth doped ceramics listed, which may be due to the addition of aliovalent Sm3+ dopant on A-site of the perovskite structure with similar ion radius. It is noting that Sm3+ is the smallest ion among all the lanthanide rare Earth ions, which may completely occupy the A-site of PMN-PT solid solution and then replace the Pb ion (Li et al., 2020b). The significant effect on electric properties of Sm doping PMN-PT is mainly due to the local structure disorder. Besides, Eu3+ doped PMN-PT ceramics also exhibit excellent piezoelectric properties (d33∼1420pC/N). As we know, the Eu3+ have similar ionic radius and valence states to Sm3+ ions, which also proved that the rare Earth ions with a similar ionic radius to the A-site ions of the perovskite ferroelectric materials can be used to improve piezoelectric performance. Nevertheless, Sm doping reduced the Curie temperature of PMN-PT (89°C) that would limit their high temperature applications. The RE doped lead-based (PMN-PZT and BS-PT) and lead-free based (BNT and KNN) materials with relatively high Curie temperature were studied for enhancing piezoelectric constant. Therefore, it is difficult to obtain the samples with both excellent piezoelectric constant and high Curie temperature and more research works will be needed in the future to improve performances by regulating the local structure disorder of piezoelectric materials with a stale Curie temperature.
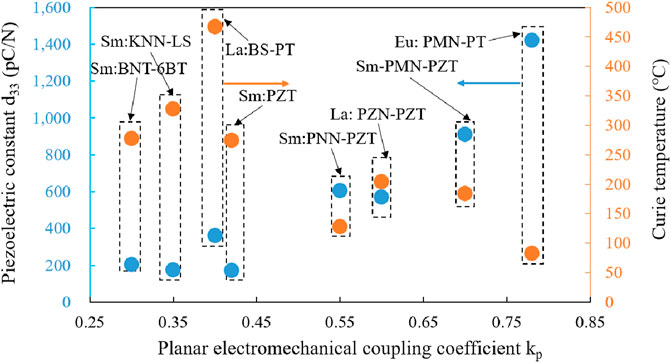
FIGURE 3. The properties of some piezoelectric ceramics with rare-earth doping (Pandey et al., 2009; Peng et al., 2010a; Yao et al., 2010; Wang et al., 2012; Hao et al., 2015; Peng et al., 2016; Guo et al., 2019a; Guo et al., 2019b).
Optical Properties
Except for the electric properties, the optical performances were discussed. On the one hand, the rare-earth doping can obtain transparent samples with high transmittance. Figure 4 gives the transmittance of some piezoelectric materials with rare-earth doping. It can be found that the transparency of the listed piezoelectric materials exceeds 60% in a specific wavelength range. For the ceramics, the grain boundary scattering loss is the main factor affecting the transmittance. Without exceeding the solubility limit, rare-earth can dissolve into the perovskite lattice homogeneously, meanwhile enhance the ceramic density, transparency, and other properties. However, excessive rare-earth ions may accumulate at the grain boundaries with increasing rare-earth content, which will lead to Rayleigh scattering loss due to the different refractive indices between grain boundaries and matrix. Besides, ion vacancies and crystal defects as well as the defect-induced poor crystallinity may decrease the transparency. Therefore, optimizing these factors to further improve the transparency of rare-earth doped piezoelectric materials may promote the development of multifunctional optical devices greatly.
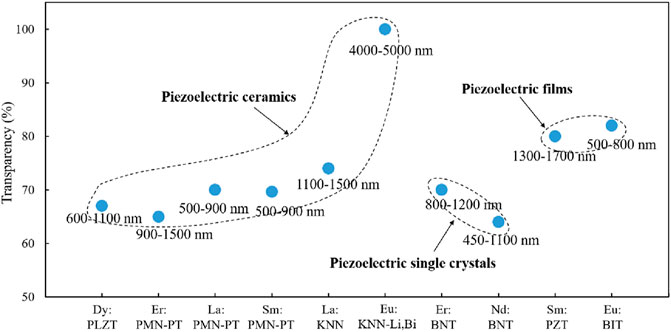
FIGURE 4. The transmittance of some rare Earth doped piezoelectric materials in a certain wavelength range [with the thickness of 0.35 mm (Dy:PLZT); 0.6 mm (La:PMN-PT); 0.85 mm (Sm:PMN-PT); 0.5 mm (La:KNN); 0.5 mm (Eu: KNN-Li,Bi); 1 mm (Nd:BNT); 300 nm (Eu:BIT)] (Pandey et al., 2007; Ruan et al., 2008; Wei et al., 2012b; He et al., 2013; Zeng et al., 2014; Geng et al., 2017; Yang et al., 2017; Jiang et al., 2018; Ma et al., 2018; Fang et al., 2021).
One the other hand, the rare-earth doped piezoelectric materials can get characteristic emission spectrum after being excited due to the ladder-shaped 4f energy level of rare-earth ions (Figure 2). Under the excited of 300–500 nm, some sharp down-conversion emissions can be achieved in the rare-earth doped materials. Typically, Eu3+ doping can acquire red (617 nm, 5D0 → 7F2) emission, and Sm3+ doping can get red (645 nm, 4G5/2 → 6H9/2) and orange (563 nm, 4G5/2 → 6H5/2) emissions. Pr3+ doping can obtain green (545 nm, 3P0 → 3H5) and red (617 nm, 3P0 → 3H6) emissions. Besides, some sharp up-conversion emissions can be also achieved in the rare-earth doped materials under the excitation of 980 nm. Green (500 nm, 2G11/2, 4S3/2 → 4I15/2) and red (670 nm, 4F9/2 → 4I15/2) emissions can be found for Er3+ doped samples, and blue (480 nm, 1G4 → 3H6) and red (652 nm, 1G4 → 3F4) emissions can be observed in Tm3+/Yb3+ co-doped samples. Green (529 nm 3P0 → 3H5), orange (623 nm 3P0 → 3H6) and red (649 nm 3P0 → 3F2) emissions were found in Pr3+/Yb3+ co-doped materials. The introduced photoluminescence performance can significantly expand the application range of piezoelectric materials. Especially, the materials can achieve up-conversion emissions with converting long-wavelength light (invisible to the naked eye) into visible light, which will have important application in the information science and technology fields such as laser, display, anti-counterfeiting, and will also open up new research fields in biomedicine or photoacoustic multi-mode images. However, some conditions including doping concentration, sintering temperature, chemical composition and preparation process have a great influence on the electrical performance and luminescence performance of the rare-earth doped piezoelectric materials. Adjusting these conditions to obtain both good electrical and optical properties is of great significance for the development of multifunctional materials and devices.
Conclusion and Perspective
The electric and photoluminescence properties of piezoelectric materials can be significantly modified by rare-earth doping. Consequently, rare-earth doping is an effective method to fabricate multifunctional materials. The research and development trend of rare-earth doped piezoelectric materials are prospected as follows. 1) With the needs of environmental protection and sustainable development of human society, it is urgent to develop environmental friendly lead-free piezoelectric materials with excellent performance to replace traditional lead-based ceramics. The future development trend is to find an appropriate concentration of rare-earth oxide and proper lead-free piezoelectric materials to achieve excellent electric properties. 2) For the large strain and high energy conversion efficiency applications, rare-earth doped piezoelectric single crystals are superior to traditional piezoelectric ceramics due to their great electric properties. The future development trend is to optimize the growth conditions and properties of single crystals to meet increasingly demanding applications. 3) The rare-earth doped piezoelectric materials are a type of optical-mechanical-electric integrated multifunctional material. Due to the unique properties of rare-earth ions and piezoelectric materials, this type of new material has great advantages in sensing, detection, and information transmission.
Author Contributions
YC and DZ wrote and edited the manuscript. ZP, MY, and XJ conceived the project and edited the manuscript.
Funding
This work was financially supported by National Science Foundation Grants (NSFC Grant No. 11804059, 51975131, 51805097), Guangdong Innovative and Entrepreneurial Research Team Program (Grant No. 2016ZT06G375) and Ministry of Science and Technology of China (Grant No. 2018YFF01010500).
Conflict of Interest
The authors declare that the research was conducted in the absence of any commercial or financial relationships that could be construed as a potential conflict of interest.
References
Battisha, I. K., Badr, Y., Shash, N. M., El-Shaarawy, M. G., and Darwish, A. G. A. (2010). Detection of Up-Conversion in Nano-Structure BaTiO3 Co-doped with Er3+ and Yb3+ Ions. J. Sol-gel Sci. Technol. 53, 543–550. doi:10.1007/s10971-009-2129-5
Birol, H., Damjanovic, D., and Setter, N. (2006). Preparation and Characterization of (K0.5Na0.5)NbO3 Ceramics. J. Eur. Ceram. Soc. 26, 861–866. doi:10.1016/j.jeurceramsoc.2004.11.022
Caballero, A. C., Fernández, J. F., Villegas, M., Moure, C., Durán, P., Florian, P., et al. (2000). Intermediate Phase Development in Phosphorus-Doped Barium Titanate. J. Am. Ceram. Soc. 83, 1499–1505. doi:10.1111/j.1151-2916.2000.tb01417.x
Cernea, M., Secu, C. E., Vasile, B. S., and Secu, M. (2013). Structural and Optical Characterization of Sol-Gel Derived Tm-Doped BaTiO3 Nanopowders and Ceramic. Curr. Appl. Phys. 13, 137–141. doi:10.1016/j.cap.2012.06.024
Chen, C., Wang, X., Wang, Y., Yang, D., Yao, F., Zhang, W., et al. (2020a). Additive Manufacturing of Piezoelectric Materials. Adv. Funct. Mater. 30, 2005141. doi:10.1002/adfm.202005141
Chen, Y., Bao, X., Wong, C.-M., Cheng, J., Wu, H., Song, H., et al. (2018). PZT Ceramics Fabricated Based on Stereolithography for an Ultrasound Transducer Array Application. Ceramics Int. 44, 22725–22730. doi:10.1016/j.ceramint.2018.09.055
Chen, Y., Xi, Z., Guo, F., Fang, P., Li, X., Long, W., et al. (2020b). Electric and Photoluminescence Properties of Eu3+-Doped PZN-9PT Single crystal. J. Mater. Sci. Mater. Electron. 31, 11295–11302. doi:10.1007/s10854-020-03678-z
Chen, Y., Xi, Z., Guo, F., Fang, P., Li, X., Long, W., et al. (2020c). Pb(Er1/2Nb1/2)O3-Pb(Zn1/3Nb2/3)O3-PbTiO3 Single Crystals with High Curie Temperature. Crystals 10, 22–43. doi:10.3390/cryst10010022
Choi, S. W., Shrout, R. T. R., Jang, S. J., and Bhalla, A. S. (1989). Dielectric and Pyroelectric Properties in the Pb(Mg1/3Nb2/3)O3-PbTiO3 system. Ferroelectrics 100, 29–38. doi:10.1080/00150198908007897
Dang, S., Sun, L.-N., Zhang, H.-J., Guo, X.-M., Li, Z.-F., Feng, J., et al. (2008). Near-Infrared Luminescence from Sol−Gel Materials Doped with Holmium(III) and Thulium(III) Complexes. J. Phys. Chem. C 112, 13240–13247. doi:10.1021/jp8041632
de Camargo, A. S. S., Botero, É. R., Garcia, D., Eiras, J. A., and Nunes, L. A. O. (2005). Nd3+-doped lead Lanthanum Zirconate Titanate Transparent Ferroelectric Ceramic as a Laser Material: Energy Transfer and Stimulated Emission. Appl. Phys. Lett. 86, 152905. doi:10.1063/1.1899752
de Camargo, A. S. S., de O. Nunes, L. A., Santos, I. A., Garcia, D., and Eiras, J. A. (2004). Structural and Spectroscopic Properties of Rare-Earth (Nd3+, Er3+, and Yb3+) Doped Transparent lead Lanthanum Zirconate Titanate Ceramics. J. Appl. Phys. 95, 2135–2140. doi:10.1063/1.1642285
Deng, G., Yin, Q., Ding, A., Zheng, X., Cheng, W., and Qiu, P. (2010). High Piezoelectric and Dielectric Properties of La-Doped 0.3Pb(Zn1/3Nb2/3)O3-0.7Pb(ZrxTi1−x)O3 Ceramics Near Morphotropic Phase Boundary. J. Am. Ceram. Soc. 88, 2310–2314. doi:10.1111/j.1551-2916.2005.00391.x
Ding, G., Gao, F., Wu, G., and Bao, D. (2011). Bright Up-Conversion green Photoluminescence in Ho3+-Yb3+ Co-doped Bi4Ti3O12 Ferroelectric Thin Films. J. Appl. Phys. 109, 123101. doi:10.1063/1.3596597
Du, J., Xu, Z., Chu, R., Hao, J., Li, W., Jiang, G., et al. (2017). Rare-earth Doped (K0.5Na0.5)NbO3 Multifunctional Ceramics. J. Mater. Sci. Mater. Electron. 28, 5288–5294. doi:10.1007/s10854-016-6186-6
Fan, G. F., Shi, M. B., Lu, W. Z., Wang, Y. Q., and Liang, F. (2014). Effects of Li2CO3 and Sm2O3 Additives on Low-Temperature Sintering and Piezoelectric Properties of PZN-PZT Ceramics. J. Eur. Ceram. Soc. 34, 23–28. doi:10.1016/j.jeurceramsoc.2013.07.028
Fang, Z., Jiang, X., Tian, X., Zheng, F., Cheng, M., Zhao, E., et al. (2021). Ultratransparent PMN‐PT Electro‐Optic Ceramics and its Application in Optical Communication. Adv. Opt. Mater. 2021, 2002139. doi:10.1002/adom.202002139
Gao, F., Wu, G., Zhou, H., and Bao, D. (2009). Strong Upconversion Luminescence Properties of Yb3+ and Er3+ Codoped Bi4Ti3O12 Ferroelectric Thin Films. J. Appl. Phys. 106, 126104–126698. doi:10.1063/1.3273477
Geng, Z., Li, K., Li, X., and Shi, D. (2017). Fabrication and Photoluminescence of Eu-Doped KNN-Based Transparent Ceramics. J. Mater. Sci. 52, 2285–2295. doi:10.1007/s10853-016-0521-4
Guo, J., Zhou, H., Fan, T., Zhao, B., Shang, X., Zhou, T., et al. (2020). Improving Electrical Properties and Toughening of PZT-Based Piezoelectric Ceramics for High-Power Applications via Doping Rare-Earth Oxides. J. Mater. Res. Tech. 9, 14254–14266. doi:10.1016/J.JMRT.2020.10.022
Guo, Q., Hou, L., Li, F., Xia, F., Wang, P., Hao, H., et al. (2019a). Investigation of Dielectric and Piezoelectric Properties in Aliovalent Eu3+ ‐modified Pb(Mg1/3 Nb2/3 )O3 ‐PbTiO3 Ceramics. J. Am. Ceram. Soc. 102, 7428–7435. doi:10.1111/jace.16653
Guo, Q., Li, F., Xia, F., Gao, X., Wang, P., Hao, H., et al. (2019b). High-Performance Sm-Doped Pb(Mg1/3Nb2/3)O3-PbZrO3-PbTiO3-Based Piezoceramics. ACS Appl. Mater. Inter. 11, 43359–43367. doi:10.1021/acsami.9b15424
Hagemann, H.-J., and Hennings, D. (1981). Reversible Weight Change of Acceptor-Doped BaTiO3. J. Am. Ceram. Soc. 64, 590–594. doi:10.1111/j.1151-2916.1981.tb10223.x
Hao, J., Xu, Z., Chu, R., Li, W., and Du, J. (2015). Bright Reddish-orange Emission and Good Piezoelectric Properties of Sm2O3-Modified (K0.5Na0.5)NbO3-Based lead-free Piezoelectric Ceramics. J. Appl. Phys. 117, 194104. doi:10.1063/1.4921451
He, C., Zhang, Y., Liang, S., Wang, J., Tong, W., Feng, X., et al. (2013). Electrical and Optical Properties of Nd3+-Doped Na0.5Bi0.5TiO3 Ferroelectric Single crystal. J. Phys. D. 46, 245104. doi:10.1088/0022-3727/46/24/245104
Hiruma, Y., Nagata, H., Hidaka, Y., and Tsukada, S. (2010). Depolarization Temperatures and Piezoelectric Properties of (Bi0.5Na0.5)TiO3 Ceramics and Single crystal. Ferroelectrics 404, 815–827. doi:10.1080/00150193.2010.482854
Inaguma, Y., Miyaguchi, A., Yoshida, M., and Katsumata, T. (2004). High-pressure Synthesis and Ferroelectric Properties in Perovskite-type BiScO3-PbTiO3 Solid Solution. J. Appl. Phys. 95, 231–235. doi:10.1063/1.1629394
Jaeger, R. E. (2010). Hot Pressing of Potassium-Sodium Niobates. J. Am. Ceram. Soc. 45, 209–213. doi:10.1111/j.1151-2916.1962.tb11127.x
Jiang, L., Wang, Z., Chen, Y., Chen, P., Luo, L., and Chen, H. (2018). Bright Up-Conversion Emission of Er3+-Doped lead-free Ferroelectric Na0.5Bi0.5TiO3 Single crystal. Mater. Lett. 210, 158–160. doi:10.1016/j.matlet.2017.08.135
Jo, W., Daniels, J. E., Jones, J. L., Tan, X., Thomas, P. A., Damjanovic, D., et al. (2011). Evolving Morphotropic Phase Boundary in lead-free (Bi1/2Na1/2)TiO3-BaTiO3 Piezoceramics. J. Appl. Phys. 109, 14110. doi:10.1063/1.3530737
Kamzina, L. S., Wei, R., Li, G., Zeng, J., and Ding, A. (2010). Electro-optical Properties of PMN-xPT Compounds: Single Crystals and Transparent Ferroelectric Ceramic. Phys. Solid State 52, 2142–2146. doi:10.1134/S1063783410100203
Khazanchi, R., Sharma, S., and Goel, T. C. (2005). Effect of Rare Earth Europium Substitution on the Microstructure, Dielectric, Ferroelectric and Pyroelectric Properties of PZT Ceramics. J. Electroceram 14, 113–118. doi:10.1007/s10832-005-0871-9
Kim, K. B., Hsu, D. K., Ahn, B., Kim, Y. G., and Barnard, D. J. (2010). Fabrication and Comparison of PMN-PT Single crystal, PZT and PZT-Based 1-3 Composite Ultrasonic Transducers for NDE Applications. Ultrasonics 50, 790–797. doi:10.1016/j.ultras.2010.04.001
Kim, K. T., Kim, C. I., Kang, D. H., and Shim, I. W. (2002). The Effect of Eu Substitution on the Ferroelectric Properties of Bi4Ti3O12 Thin Films Prepared by Metal-Organic Decomposition. Thin Solid Films 422, 230–234. doi:10.1016/S0040-6090(02)00981-1
Kour, P., Kumar, P., Sinha, S. K., and Kar, M. (2015). Study of Dielectric and Impedance Spectroscopy of La Substituted Nanocrystalline Pb(Zr0.52Ti0.48)O3 Ceramics. J. Mater. Sci. Mater. Electron. 26, 1304–1310. doi:10.1007/s10854-014-2538-2
Kour, P., Pradhan, S. K., Kumar, P., Sinha, S. K., and Kar, M. (2016). Enhanced Ferroelectric and Piezoelectric Properties in La-Modified PZT Ceramics. Appl. Phys. A-mater. 122, 1–7. doi:10.1007/s00339-016-0122-8
Kumari, M., Yadav, A., and Sarun, P. M. (2020). Systematic Investigation of Structural, Optical and Dielectric Properties of 0.5 Mol% Eu: BaTiO3 Ceramics. Mater. Today Proc. 42, 2214–7853. doi:10.1016/j.matpr.2020.03.337
Kuwata, J., Uchino, K., and Nomura, S. (1982). Dielectric and Piezoelectric Properties of 0.91Pb(Zn1/3Nb2/3)O3-0.09PbTiO3 Single Crystals. Jpn. J. Appl. Phys. 21, 1298–1302. doi:10.1143/JJAP.21.1298
Lau, C. M., Xu, X. W., and Kwok, K. W. (2015). Photoluminescence, Ferroelectric, Dielectric and Piezoelectric Properties of Er-Doped BNT-BT Multifunctional Ceramics. Appl. Surf. Sci. 336, 314–320. doi:10.1016/j.apsusc.2014.12.105
Li, C., Xu, B., Lin, D., Zhang, S., and Li, F. (2020b). Atomic-scale Origin of Ultrahigh Piezoelectricity in Samarium-Doped PMN-PT Ceramics. Phys. Rev. B. 101, 140102. doi:10.1103/PhysRevB.101.140102
Li, F., Cabral, M. J., Xu, B., Cheng, Z., Dickey, E. C., Lebeau, J. M., et al. (2019b). Giant Piezoelectricity of Sm-Doped Pb(Mg1/3Nb2/3)O3-PbTiO3 Single Crystals. Science 364, 264. doi:10.1126/science.aaw2781
Li, F., Lin, D., Chen, Z., Cheng, Z., Wang, J., Li, C. C., et al. (2018). Ultrahigh Piezoelectricity in Ferroelectric Ceramics by Design. Nat. Mater. 17, 349–424. doi:10.1038/s41563-018-0034-4
Li, H., Feng, C., and Yao, W. (2004). Some Effects of Different Additives on Dielectric and Piezoelectric Properties of (Bi1/2Na1/2)TiO3-BaTiO3 Morphotropic-Phase-Boundary Composition. Mater. Lett. 58, 1194–1198. doi:10.1016/j.matlet.2003.08.034
Li, H., Zhang, Y., Zhou, J., Zhang, X., Liu, H., and Fang, J. (2015a). Phase Structure and Electrical Properties of xPZN-(1-X)PZT Piezoceramics Near the Tetragonal/rhombohedral Phase Boundary. Ceram. Int. 41, 4822–4828. doi:10.1016/j.ceramint.2014.12.038
Li, Q., Wei, S., Jiang, Y., Ying, P., Qiang, C., Xi, Y., et al. (2009). “Effect of Y-Doping on the Piezoelectric Properties of (1-x)BiScO3-xPbTiO3 High-Temperature Piezoelectric Ceramics,” in 2009 18th IEEE International Symposium on the Applications of Ferroelectrics (China: Xi’an), 1–4. doi:10.1109/ISAF.2009.5307539
Li, W., Hao, J., Du, J., Fu, P., and Chu, R. (2020a). Electrical Properties and Luminescence Properties of 0.96(K0.48Na0.52)(Nb0.95Sb0.05)-0.04Bi0.5(Na0.82K0.18)0.5ZrO3-xSm lead-free Ceramics. J. Adv. Ceram. 9, 72–82. doi:10.1007/s40145-019-0349-x
Li, X., Chen, C., Deng, H., Zhang, H., and Luo, H. (2015b). The Growth and Properties of lead-free Ferroelectric Single Crystals. Crystals 5, 172–192. doi:10.3390/cryst5020172
Li, Y., Xi, Z., Fang, P., Li, X., Long, W., He, A., et al. (2019c). Electric and Optical Properties of Tm3+/Yb3+ Co-doped PZN-9PT Crystals. J. Mater. Sci. Mater. Electron. 30, 3811–3819. doi:10.1007/s10854-019-00665-x
Li, Y. X., Yao, X., Wang, X. S., and Hao, Y. B. (2012). Studies of Dielectric Properties of Rare Earth (Dy, Tb, Eu) Doped Barium Titanate Sintered in Pure Nitrogen. Ceram. Int. 38, S29–S32. doi:10.1016/j.ceramint.2011.04.042
Li, Y., Zhao, J., Zhang, X., Jia, Q., and Hao, X. (2019a). Tuning the Ferroelectric, Dielectric and Photoluminescence Properties of 0.88(Na0.5Bi0.5)TiO3-0.12BaTiO3 Ceramics by Sm Ion Doping. J. A. D. 9, 1950041. doi:10.1142/S2010135X19500413
Liu, L., Fan, H., Ke, S., and Chen, X. (2008). Effect of Sintering Temperature on the Structure and Properties of Cerium-Doped 0.94(Bi0.5Na0.5)TiO3-0.06BaTiO3 Piezoelectric Ceramics. J. Alloys Compd. 458, 504–508. doi:10.1016/j.jallcom.2007.04.037
Liu, L. (2015). Progress on the Fabrication of lead-free Textured Piezoelectric Ceramics: Perspectives over 25years. J. Mater. Sci-mater El. 26 (7), 4425–4437. doi:10.1007/s10854-015-2920-8
Liu, Z., Jiang, G., Wang, R., Chai, C., Zheng, L., Zhang, Z., et al. (2016). Temperature and Concentration Effects on Up-Conversion Photoluminescence Properties of Ho3+ and Yb3+ Co-doped 0.67Pb(Mg1/3Nb2/3)O3-0.33PbTiO3 Multifunctional Ceramics. Ceram. Int. 45, 11309–11313. doi:10.1016/j.ceramint.2016.04.049
Lv, Z., Qin, Y., Zhang, Y., Fu, J., and Lu, C. (2019). Efficient Up-Conversion Photoluminescence in Transparent Pr3+/Yb3+ Co-doped 0.75Pb(Mg1/3Nb2/3)O3-0.25PbTiO3 Ferroelectric Ceramics. Ceram. Int. 45, 10924–10929. doi:10.1016/j.ceramint.2019.02.172
Ma, Z., Zhang, Y., Lu, C., Qin, Y., Lv, Z., and Lu, S. (2018). Synthesis and Properties of La-Doped PMN-PT Transparent Ferroelectric Ceramics. J. Mater. Sci-mater El. 29, 6985–6990. doi:10.1007/s10854-018-8685-0
Majumder, S. B., Dobal, P. S., Roy, B., Bhaskar, S., Katiyar, R. S., and Bhalla, A. (2001). Effect of Rare Earth Doping on Sol-Gel Derived PZT Thin Films. Ferroelectrics Lett. 28, 85–92. doi:10.1080/07315170108202952
Morrison, F. D., Coats, A. M., Sinclair, D. C., and West, A. R. (2001). Charge Compensation Mechanisms in La-Doped BaTiO3. J. Electroceramics 6, 219–232. doi:10.1023/A:1011400630449
Nakaki, H., Uchida, H., Okamoto, S., Yokoyama, S., Funakubo, H., and Koda, S. (2004). Improvement of Ferroelectric Properties of lead Zirconate Titanate Thin Films by Ion-Substitution Using Rare-Earth Cations. Mrs Proc. 830, D3. doi:10.1557/PROC-830-D3.3
Panda, P. K. (2009). Review: Environmental Friendly lead-free Piezoelectric Materials. J. Mater. Sci. 44, 5049–5062. doi:10.1007/s10853-009-3643-0
Pandey, S. K., Kumar, S., Chatterjee, S. N., Kumar, U., Prakash, C., Chatterjee, R., et al. (2007). Growth and Characterization of Sm3+-Substituted PZT Thin Films. Physica B 388, 404–411. doi:10.1016/j.physb.2006.06.167
Pandey, S. K., Thakur, O. P., Bhattacharya, D. K., Prakash, C., and Chatterjee, R. (2009). Structural and Electrical Properties of Sm3+ Substituted PZT Ceramics. J. Alloys Compd. 468, 356–359. doi:10.1016/j.jallcom.2008.01.006
Peng, F., Xu, Z., Chu, R., Wei, L., Zang, G., and Hao, J. (2010c). Piezoelectric, Ferroelectric and Dielectric Properties of La2O3-Doped (Bi0.5Na0.5)0.94Ba0.06TiO3 lead-free Ceramics lead-free Ceramics. Mater. Des. 31, 706–801. doi:10.1016/j.matdes.2009.07.056
Peng, F., Xu, Z., Chu, R., Wei, L., Zang, G., and Hao, J. (2010b). Piezoelectric, Ferroelectric and Dielectric Properties of Nd2O3-Doped (Bi0.5Na0.5)0.94Ba0.06TiO3 lead-free Ceramics. Mater. Sci. Eng. B. 167, 161–166. doi:10.1016/j.mseb.2010.01.057
Peng, F., Xu, Z., Chu, R., Wei, L., Zang, G., and Hao, J. (2010a). Piezoelectric, Ferroelectric and Dielectric Properties of Sm2O3-Doped (Bi0.5Na0.5)0.94Ba0.06TiO3 lead-free Ceramics. Mater. Sci. Eng. B. 124, 1065–1070. doi:10.1016/j.matchemphys.2010.08.033
Peng, G., Zheng, D., Hu, S., Zhao, H., and Cheng, C. (2016). Effects of Rare-Earth Sm2O3 Addition on Relaxation Behavior and Electric Properties of 0.5PNN-0.5PZT Ceramics. J. Mater. Sci. Mater. Electron. 27, 5509–5516. doi:10.1007/s10854-016-4577-3
Perumal, R. N., Sadhasivam, S., and Athikesavan, V. (2019). Structural, Dielectric, Ac Conductivity, Piezoelectric and Impedance Spectroscopy Studies on PbZr0.52Ti0.48O3:Re3+ (Re3+: La3+, Nd3+ and Dy3+) Ceramics. Results Phys. 15, 102729. doi:10.1016/j.rinp.2019.102729
Pieter, D. (2017). Charge Transfer Bands in Optical Materials and Related Defect Level Location. Opt. Mater. 69, 8–22. doi:10.1016/j.optmat.2017.03.061
Pieter, D. (2013). Lanthanide 4f-Electron Binding Energies and the Nephelauxetic Effect in Wide Band gap Compounds. J. Lumin. 136, 122–129. doi:10.1016/j.jlumin.2012.11.030
Politova, E. D., Egorova, B. V., Kaleva, G. M., Mosunov, A. V., and Zeng, J. (2011). Phase Transitions and the Dielectric and Piezoelectric Properties of Ceramic Solid Solutions Based on BiScO3-PbTiO3. Bull. Russ. Acad. Sci. Phys. 75, 1166–1169. doi:10.3103/S1062873811050418
Qiu, W., and Hng, H. H. (2002). Effects of Dopants on the Microstructure and Properties of PZT Ceramics. Mater. Chem. Phys. 75, 151–156. doi:10.1016/S0254-0584(02)00045-7
Rajan, K. K., Shanthi, M., Chang, W. S., Jin, J., and Lim, L. C. (2007). Dielectric and Piezoelectric Properties of [0 0 1] and [0 1 1]-poled Relaxor Ferroelectric PZN-PT and PMN-PT Single Crystals. Sensor Actuat A-Phys. 133, 110–116. doi:10.1016/j.sna.2006.03.036
Rath, M., Miryala, M., Murakami, M., and Rao, M. S. R. (2019). Controlled Piezotronic Properties on Recoverable Energy Storage Density in Rare-Earth Ions Doped Epitaxial PZT Thin Films. J. Phys. D. 52, 304001. doi:10.1088/1361-6463/ab1b08
Ruan, K., Chen, X., Liang, T., Wu, G., and Bao, D. (2008). Photoluminescence and Electrical Properties of Highly Transparent (Bi,Eu)4Ti3O12 Ferroelectric Thin Films on Indium-Tin-Oxide-Coated Glass Substrates. J. Appl. Phys. 103, 627. doi:10.1063/1.2903928
Saito, Y., Takao, H., Tani, T., Nonoyama, T., Takatori, K., Homma, T., et al. (2004). Lead-free Piezoceramics. Nature 432, 84–87. doi:10.1038/nature03028
Samad, R., Rather, M., Asokan, K., and Want, B. (2017). Structural, Dielectric and Ferroelectric Properties of Rare Earth Substituted lead Zirconate Titanate. J. Mater. Sci-mater El. 29, 4226–4237. doi:10.1007/s10854-017-8368-2
Shannigrahi, R. S., Tay, F. E. H., Yao, K., and Choudhary, R. N. P. (2004). Effect of Rare Earth (La, Nd, Sm, Eu, Gd, Dy, Er and Yb) Ion Substitutions on the Microstructural and Electrical Properties of Sol-Gel Grown PZT Ceramics Science Direct. J. Eur. Ceram. Soc. 24, 163–170. doi:10.1016/S0955-2219(03)00316-9
Shrout, T. R., and Zhang, S. J. (2007). Lead-free Piezoelectric Ceramics: Alternatives for PZT?. J. Electroceram 19, 113–126. doi:10.1007/s10832-007-9047-0
Singh, D. J., Ghita, M., Fornari, M., and Halilov, S. V. (2011). Role of A-Site and B-Site Ions in Perovskite Ferroelectricity. Ferroelectrics 338, 73–79. doi:10.1080/00150190600732694
Singh, N., Shyam, R., Upadhyay, N. K., and Dhar, A. (2015). Development of Rare-Earth Free Mn-Al Permanent Magnet Employing Powder Metallurgy Route. IOP Conf. Ser. Mater. Sci. Eng. 73, 012042. doi:10.1088/1757-899X/73/1/012042
Steudel, F., Loos, S., Ahrens, B., and Schweizer, S. (2015). Quantum Efficiency and Energy Transfer Processes in Rare-Earth Doped Borate Glass for Solid-State Lighting. J. Lumin. 170, 157–162. doi:10.1016/j.jlumin.2015.07.032
Sun, H., Peng, D., Wang, X., Tang, M., Zhang, Q., and Yao, X. (2012). Green and Red Emission for (K0.5Na0.5)NbO3: Pr Ceramics. J. Appl. Phys. 111, 2087. doi:10.1063/1.3686193
Sun, H., Peng, D., Wang, X., Tang, M., Zhang, Q., and Yao, X. (2011). Strong Red Emission in Pr Doped (Bi0.5Na0.5)TiO3 Ferroelectric Ceramics. J. Appl. Phys. 110, 2087. doi:10.1063/1.3606425
Sun, H. Q., Wang, X. S., and Yao, X. (2010). Structure and Electric Properties of Sm Doped BaTiO3 Ceramics. Ferroelectrics 404, 99–104. doi:10.1080/00150193.2010.482459
Sun, H., Zhang, Q., Wang, X., and Mu, G. (2014). Green and Red Up-Conversion Luminescence of Er3+-Doped K0.5Na0.5NbO3 Ceramics. Ceram. Int. 40, 2581–2584. doi:10.1016/j.ceramint.2013.10.089
Sun, L. N., Zhang, H. J., Peng, C. Y., Yu, J. B., Meng, Q. G., Fu, L. S., et al. (2006). Covalent Linking of Near-Infrared Luminescent Ternary Lanthanide (Er(3+), Nd(3+), Yb(3+)) Complexes on Functionalized Mesoporous MCM-41 and SBA-15. J. Phys. Chem. B 110, 7249–7258. doi:10.1021/jp060395u
Tsonev, L. (2008). Luminescent Activation of Planar Optical Waveguides in LiNbO3 with Rare Earth Ions Ln3+ a Review. Opt. Mater. 30, 892–899. doi:10.1016/j.optmat.2007.03.011
Uchino, K. (1995). Electro-optic Ceramics and Their Display Applications. Ceram. Int. 21, 309–315. doi:10.1016/0272-8842(95)96202-Z
Vittayakorn, N., Rujijanagul, G., Tan, X., He, H., Marquardt, M. A., and Cann, D. P. (2006). Dielectric Properties and Morphotropic Phase Boundaries in the xPb(Zn1/3Nb2/3)O3-(1-x)Pb(Zr0.5Ti0.5)O3 Pseudo-binary System. J. Electroceramic 16, 141–149. doi:10.1007/s10832-006-4927-2
Wang, N., Sun, Q., Ma, W., Yong, Z., and Liu, H. (2012). Investigation of La-Doped 0.25Pb(Zn1/3Nb2/3)O3-0.75Pb(ZrxTi1-x)O3 Ceramics Near Morphotropic Phase Boundary. J. Electroceramics 28, 15–19. doi:10.1007/s10832-011-9672-5
Wei, R., Li, G., Zeng, J., Bian, J., Kamzina, L. S., Zeng, H., et al. (2010). Large Electro-Optic Effect in La-Doped 0.75Pb(Mg1/3Nb2/3)O3-0.25PbTiO3 Transparent Ceramic by Two-Stage Sintering. Int. J. Appl. Ceram. Tec 93, 2128–2131. doi:10.1111/j.1551-2916.2010.03675.x
Wei, Z., Huang, Y., Tsuboi, T., Nakai, Y., Zeng, J., and Li, G. (2012a). Optical Characteristics of Er3+-Doped PMN-PT Transparent Ceramics. Ceram. Int. 38, 3397–3402. doi:10.1016/j.ceramint.2011.12.051
Wei, Z., Tsuboi, T., Nakai, Y., Huang, Y., Zeng, J., and Li, G. (2012b). The Synthesis of Er3+-Doped PMN-PT Transparent Ceramic and its Infrared Luminescence. Mater. Lett. 68, 57–59. doi:10.1016/j.matlet.2011.10.034
Wu, X., Chi, M. L., and Kwok, K. W. (2015a). Photoluminescence Properties of Er/Pr-Doped K0.5Na0.5NbO3 Ferroelectric Ceramics. J. Am. Ceram. Soc. 98, 2139–2145. doi:10.1111/jace.13605
Wu, X., Chung, T. H., and Kwok, K. W. (2015b). Enhanced Visible and Mid-IR Emissions in Er/Yb-Codoped K0.5Na0.5NbO3 Ferroelectric Ceramics. Ceram. Int. 41, 14041–14048. doi:10.1016/j.ceramint.2015.07.018
Wu, X., Kwok, K. W., and Li, F. L. (2013). Up-conversion Fluorescence Studies of Sol-Gel Derived Er-Doped KNN Ceramics. J. Alloys Compd. 580, 88–92. doi:10.1016/j.jallcom.2013.05.096
Wu, X., Lu, S., and Kwok, K. W. (2017). Photoluminescence, Electro-Optic Response and Piezoelectric Properties in Pressureless-Sintered Er-Doped KNN-Based Transparent Ceramics. J. Alloys Compd. 695, 3573–3578. doi:10.1016/j.jallcom.2016.11.409
Xi, Z., Han, A., Fang, P., Li, X., and Long, W. (2016a). Electrical Properties and Up-Conversion Luminescence of the Er3+-Modified PZN-9PT Crystals. Int. J. Mater. Res. 31, 3044–3049. doi:10.1557/jmr.2016.311
Xi, Z., Han, A., Fang, P., Long, W., Li, X., and Bu, Q. (2016b). Structural and Electrical Properties of Ho3+-Modified Pb(Zn1/3Nb2/3)O3-9PbTiO3 Single Crystals. Mater. Sci. Mater. Electron. 27, 4223–4229. doi:10.1007/s10854-016-4286-y
Xie, D., Zhang, Z., Ren, T., Liu, T., and Liu, L. (2006). Properties of Neodymium-Doped Bi4Ti3O12 Thin Films for Ferroelectric Random Access Memory. Integrated Ferroelectrics 84, 67–73. doi:10.1080/10584580601085230
Xu, J., Lu, Q., Lin, C., Zheng, X., Lin, T., and Wu, X. (2020). Enhanced Ferro-/piezoelectric Properties of Tape-Casting-Derived Er3+ -doped Ba0.85Ca0.15Ti0.9Zr0.1O3 Optoelectronic Thick Films. J. Adv. Ceram. 9, 693–702. doi:10.1007/s40145-020-0405-6
Yang, D., Yang, Z., Zhang, X., Wei, L., Chao, X., and Yang, Z. (2017). High Transmittance in lead-free Lanthanum Modified Potassium-Sodium Niobate Ceramics. J. Alloys Compd. 716, 21–29. doi:10.1016/j.jallcom.2017.04.236
Yao, Z. H., Liu, H. X., Li, Y. Q., Cao, Y. Q., and Hao, H. (2010). Morphotropic Phase Boundary of (Bi0.9La0.1)ScO3-PbTi)O3 Piezoelectric Ceramics for High-Temperature Application. Ferroelectrics 409, 21–26. doi:10.1080/00150193.2010.485886
Yu, Y. J., Chan, H. L. W., Wang, F. P., and Zhao, L. C. (2003). Effects of Rare Earth Eu Doping on Ferroelectric Properties of PbZr0.52Ti0.48O3 Thin Films by Sol-Gel Methods. Microelectron Eng. 66, 0167–9317. doi:10.1016/S0167-9317(02)00991-7
Zeng, X., He, X., Cheng, W., Qiu, P., and Xia, B. (2014). Effect of Dy Substitution on Ferroelectric, Optical and Electro-Optic Properties of Transparent Pb0.90La0.10(Zr0.65Ti0.35)O3 Ceramics. Ceram. Int. 40, 6197–6202. doi:10.1016/j.ceramint.2013.11.074
Zhai, Y., Feng, Y., Du, J., Xue, J., Shen, J., Lu, Y., et al. (2019). The Impedance, Dielectric and Piezoelectric Properties of Tb4O7 and Tm2O3 Doped KNN Ceramics. Mater. Sci. Mater. Electron. 30, 4352–4358. doi:10.1007/s10854-019-00748-9
Zhang, H., Zhao, X., Hao, D., Chao, C., Di, L., Li, X., et al. (2014c). Photoluminescence and Electrical Properties of Eu-Doped (Na0.5Bi0.5)TiO3 Ferroelectric Single Crystals. Appl. Phys. A-mater. 114, 357–361. doi:10.1007/s00339-013-8176-3
Zhang, J. R., Zhang, Y. C., Lu, C. J., Ye, W. N., and Su, J. (2014a). Effect of La-Doping Content on the Dielectric and Ferroelectric Properties of 0.88Pb(Mg1/3Nb2/3)O3-0.12PbTiO3 Ceramics. J. Mater. Sci. Mater. Electron. 25, 653–658. doi:10.1007/s10854-013-1688-y
Zhang, J. W., Zou, Y. K., Chen, Q., Zhang, R., Li, K. K., Jiang, H., et al. (2006). Optical Amplification in Nd3+ Doped Electro-Optic Lanthanum lead Zirconate Titanate Ceramics. Appl. Phys. Lett. 89, 411–426. doi:10.1063/1.2336216
Zhang, K., Li, L., Wang, M., and Luo, W. (2020a). Charge Compensation in Rare Earth Doped BaTiO3-Based Ceramics Sintered in Reducing Atmosphere. Ceram. Int. 46, 25881–25887. doi:10.1016/j.ceramint.2020.07.072
Zhang, Q., Chen, J., and Che, M. (2020b). Dielectric Properties of Barium Titanium Ceramics Doped by Lanthanum Oxide. Ferroelectrics 566, 30–41. doi:10.1080/00150193.2020.1762427
Zhang, Q., Sun, H., Wang, X., and Zhang, T. (2014b). Highly Efficient orange Emission (K0.5Na0.5)NbO3: Sm3+ lead Free Piezoceramics. Mater. Lett. 117, 283–285. doi:10.1016/j.matlet.2013.12.026
Zhang, R., Wang, X., Zhang, S., Yang, Z., and Zou, H. (2021). Effect of Dy Doping on the crystal Orientation, Microstructure, and Electrical Properties of PDZT Thin Films Prepared by Sol-Gel Method. J. Mater. Sci. Mater. Electron. 32, 3612–3620. doi:10.1007/s10854-020-05108-6
Zhang, S., Alberta, E. F., Eitel, R. E., Randall, C. A., and Shrout, T. R. (2005). Elastic, Piezoelectric, and Dielectric Characterization of Modified BiScO3-PbTiO3 Ceramics. IEEE Trans. Ultrason. Ferroelectrics Frequency Control. 52, 2131–2139. doi:10.1109/TUFFC.2005.1561684
Zhang, S. T., Zhang, X. J., Cheng, H. W., Chen, Y. F., Liu, Z. G., Ming, N. B., et al. (2003). Enhanced Electrical Properties of C-axis Epitaxial Nd-Substituted Bi4Ti3O12 Thin Films. Appl. Phys. Lett. 83, 4378–4380. doi:10.1063/1.1629372
Zhao, H., Zhang, K., Xu, L., Sun, F., Chen, X., Li, K. K., et al. (2014). Optical Amplification in Disordered Electro-Optic Tm3+ and Ho3+ Co-doped Lanthanum-Modified lead Zirconate Titanate Ceramics and Study of Spectroscopy and Communication between Cations. J. Appl. Phys. 115, 397–2971. doi:10.1063/1.4866000
Zheng, M., Hou, Y., Zhu, M., Zhang, M., and Yan, H. (2014). Shift of Morphotropic Phase Boundary in High-Performance fine-grained PZN-PZT Ceramics. J. Eur. Ceram. Soc. 34, 2275–2283. doi:10.1016/j.jeurceramsoc.2014.02.041
Zheng, T., Wu, J., Xiao, D., and Zhu, J. (2018). Recent Development in lead-free Perovskite Piezoelectric Bulk Materials. Prog. Mater. Sci. 98, 552–624. doi:10.1016/j.pmatsci.2018.06.002
Zhou, J., Ma, Q., Wang, P., Cheng, L., and Liu, S. (2014). Influence of Rare-Earth Nd, Dy, and Ho Doping on Structural and Electrical Properties of (Na0.53K0.47)0.942Li0.058NbO3 Based lead-free Piezoceramics. Ceram. Int. 40, 2451–2459. doi:10.1016/j.ceramint.2013.08.020
Keywords: piezoelectric materials, rare-earth doping, electric properties, photoluminescence properties, multifunctional materials
Citation: Chen Y, Zhang D, Peng Z, Yuan M and Ji X (2021) Review of Research on the Rare-Earth Doped Piezoelectric Materials. Front. Mater. 8:679167. doi: 10.3389/fmats.2021.679167
Received: 11 March 2021; Accepted: 24 May 2021;
Published: 08 July 2021.
Edited by:
Chunlong Fei, Xidian University, ChinaCopyright © 2021 Chen, Zhang, Peng, Yuan and Ji. This is an open-access article distributed under the terms of the Creative Commons Attribution License (CC BY). The use, distribution or reproduction in other forums is permitted, provided the original author(s) and the copyright owner(s) are credited and that the original publication in this journal is cited, in accordance with accepted academic practice. No use, distribution or reproduction is permitted which does not comply with these terms.
*Correspondence: Xuanrong Ji, xr.ji@gdut.edu.cn; Maodan Yuan, mdyuan@gdut.edu.cn