- 1Institute of Engineering Thermophysics (CAS), Beijing, China
- 2College of Engineering Sciences, University of Chinese Academy of Sciences, Beijing, China
- 3Nanjing Institute of Future Energy System, Institute of Engineering Thermophysics, Chinese Academy of Sciences, Nanjing, China
Mil-101 (Cr) material is considered to be one of the most potential thermochemical energy storage materials in recent years. It has the advantages of a typical S-type water adsorption isotherm. Has low working temperature and large water adsorption amount. However, the adsorption properties of the material need to be improved under low water pressure. To improve the performance, the acidified MWCNTs were added before the hydrothermal reaction of mil-101 (Cr) materials to optimize the micropore structure. After the preparation, the new composite thermochemical energy storage materials were prepared by impregnation with a certain concentration of calcium chloride aqueous solution. The effects of multi-walled carbon nanotubes and calcium chloride on the physical and chemical properties of the materials were discussed. Through X-ray diffraction experiment, scanning electron microscope, microstructure analysis, nitrogen adsorption capacity test, water adsorption capacity test, and other means, the micro characteristics, pore structure, crystal morphology, and chemical composition of the materials were characterized, and the water adsorption performance of the materials was synthesized. The experimental results show that the addition of carbon nanotubes can improve the pore properties of the materials so that the porous organic skeleton can accommodate more calcium chloride particles. The composite materials with calcium chloride can provide excellent hydrophilicity and high water adsorption capacity. Also, the water absorption rate and adsorption-desorption cycle capacity of the material have been significantly improved. The experimental results show that when the mass fraction of calcium chloride reaches 30% when 90 mg acidified MWCNTs are added into every four chromium nitrate crystals, the hydrophilicity and adsorption capacity of the composite can reach a high equilibrium state. At the same time, the experimental yield of the material is high, the product is easy to obtain, the environmental friendliness is also reflected, so it is expected to become the adsorption heat pump thermochemical energy storage material with research potential.
Introduction
Thermochemical energy storage is an energy storage process where heat energy is converted into chemical energy through reversible heat absorption and release chemical reaction, and then the stored energy is released as much as possible in the required time and space at a given temperature. This energy storage mode has the functions of energy storage and heat pump, and can also be used for refrigeration (Abedin, 2011; N’Tsoukpoe et al., 2014). It is one of the most advanced energy storage modes. Among the thermochemical energy storage devices, the adsorption heat pump is a kind of device that can use low-grade energy, such as waste heat, solar energy, etc., to achieve the purpose of refrigeration and heating (Henninger et al., 2012; Demir et al., 2013). The core component of the adsorption heat pump, namely the corresponding adsorbent and adsorbate, is the main focus of attention in extensive researches. Among the common adsorbates, formaldehyde and ammonia are volatile and highly toxic, they are prone to thermal decomposition reaction under adsorption conditions; however, ethanol and water are relatively safe. Compared with ethanol, water is safer and more pollution-free, as it is non-flammable and non-toxic. Besides, the high adsorption heat and high thermal stability of water make it an outstanding adsorbate (Meunier, 1985; Pons and Grenier, 1987).
In a broad sense, thermochemical energy storage materials include absorption thermochemical energy storage materials, adsorption thermochemical energy storage materials, and chemical reaction thermochemical energy storage materials. In recent years, the research focuses on adsorption thermochemical energy storage materials, including physical adsorption, chemical adsorption, and composite adsorption (Neumann et al., 2016; Ousaleh et al., 2020). Silica gel is a typical physical adsorption material. Deshmukh et al. (2016). In 2016, the energy density of silica gel water adsorption pair was 42 kWh / m3, and the effective energy was 73%. This kind of physical adsorption can adsorb and desorb the adsorbate through the macro and microstructure of the material itself and its crystal structure. With these porous materials as the matrix, the composite materials are formed by adding some chemical adsorption salt materials. It can obtain high-performance adsorption energy storage materials by directionally changing the chemical properties of the adsorption salt and the pore properties of the porous materials.
Recently, a new type of porous material called organometallic framework material has received much attention all over the world (Stock and Biswas, 2012a, b). Such as MIL-125(Ti), CAU-1(Al), UiO-66, and MIL-101(Cr). Compared with other materials, MOF materials generally have high water absorption, strong hydrophilicity, high stability, and Superb specific water absorption isotherms (Simonova and Aristov, 2005; Leng et al., 2016). According to the literature reports, mil-101 (Cr) is a potential material with high water absorption and well water absorption stability. However, the hydrophilicity of the material is not high under low water pressure, it is an important research direction to improve its hydrophilicity so that it can do adsorption and desorption under more suitable conditions. The hydrophilicity of mil-101 (Cr) can be improved by introducing hydrophilic functional groups into the molecular structure and using the affinity of functional groups to water molecules. Andreas Sauer et al. reported that by adding 2,6-naphylenedicarboxylic acid to the hydrothermal reaction, the overall pore volume of the porous material can be expanded in Sonnauer et al. (2009). so that the performance of the composite material can be greatly improved without a large loss of total water absorption. The molecular structure can also be optimized by doping alkaline metal ion salt into the reactant metal salt of the synthetic material, to improve the performance of the material. Zhou et al. (2016), reported that the hydrophilicity of the material is greatly improved by doping metal magnesium ion salt into the material. However, the above methods can improve the hydrophilicity, the preparation process is complex, the yield is low, the thermal stability of the product is not high. In recent years, it is very popular to improve the hydrophilicity of MOFs materials by doping inorganic salts with high water absorption (Permyakova, 2016). Many researchers have also proved that the super absorbent inorganic salt has an obvious effect on improving the hydrophilicity of materials. This kind of inorganic salt can be attached to the pores of porous materials, changing the pore structure and improving the water adsorption. At the same time, the hydrophilic inorganic salt itself has good water adsorption under low water pressure. It is worth mentioning that the cost of this inorganic salt is low, which can greatly reduce the cost of composite materials preparation, and is conducive to the production and application of adsorption heat pumps. Qasem et al. (2017) showed that the combination of mil-101 (Cr) and MWCNTs form a composite material which greatly improves the adsorption properties of related gasses (Qasem et al., 2017). Through the adhesion of MWCNTs on the microstructure, the pore diameter of the material is improved dramatically. Under relevant conditions, the adsorption capacity of the gas can be increased by about 35%. In recent years, adsorption thermochemical energy storage materials have been widely studied (Iguchi and Kato, 2016), such as zeolite, molecular sieve, silica gel, and other materials, and related materials have a certain degree of application in some practical industrial production. However, the potential of improving their ultimate adsorption performance is poor. However, the amount of researches on MOF materials is not enough, and there are not enough researches on the modification of pore properties by adding inorganic salts and multi-walled carbon nanotubes based on MOFs materials. There is no comparative study on the water adsorption properties of the materials. Besides, the study on the relationship between the amount of MOFs material mixed with other materials and the material properties is not enough, which may lead to the failure of the original microstructure due to insufficient improvement or excessive doping. At the same time, the experimental yield of material modification and product availability are also worthy of consideration. If it can be better solved, it will be conducive to the application of materials in energy storage and environmental protection in the future, ultimately benefit production and life.
In this study, we use the MOFs material with good performance, mil-101 (Cr) as the basis to prepare new composite materials. By adding a variety of multi-walled carbon nanotubes before the preparation of the matrix metal-organic skeleton material, after the completion of the preparation of the material with a variety of concentrations of CaCl2 solution impregnated with composite materials. Then, the effects of different amounts of carbon nanotubes and different concentrations of salt solution on the properties and structures of the materials were discussed by X-ray diffraction, nitrogen adsorption isotherm, water vapor adsorption isotherm, and SEM. Analyze the data of water vapor dynamics of materials, understand the adsorption performance and corresponding microstructure of materials, analyze the adsorption/desorption cycle capacity of materials, and explore the methods and experiments to improve the adsorption performance of metal-organic framework materials.
Experimental Section
Synthesis of the Composite
Five groups of 4 g chromium nitrate and 3.32 g terephthalic acid were dissolved in 48 ml distilled water and then injected into a flask. The samples were stirred by a magnetic stirrer for 30 min. Acidified multi-walled carbon nanotubes (MWCNTs) of 0 mg, 30 mg, 60 mg, 90 mg, and 120 mg were added to five groups of samples, respectively, stirred for 1 h (Tan et al., 2019). The stirred solution was transferred to the PTFE reactor, sealed, and placed in a thermostat, heated at 220°C for 8 h. Then the reactor was cooled down to atmosphere temperature. The reaction products were separated into 4 ml centrifugal tubes for centrifugal separation in a high-speed centrifuge. The centrifugal speed was 9000 r/min and the single centrifugal time was about 6-10 min. After centrifugation, the supernatant was separated, washed with absolute ethanol. Then washed in an ultrasonic cleaner for 30 min, centrifuged again, and repeated three times. After the last centrifugation, the supernatant was taken out, N, N-dimethylformamide (DMF) was added, and then the suspension was heated and stirred in a water bath in a flask for 8 h. Then the suspension heated by the water bath was separated into a centrifugal tube. After high-speed centrifugation, the supernatant was removed, anhydrous alcohol was added, ultrasonic dispersion was carried out, the vacuum was 140°C. After drying for 8 h, the solid powder was ground and added to the qualitative filter paper, wrapped in a filter paper bag, put into a beaker, and soaked in methanol for 5 days. Methanol was replaced once a day. The beaker is placed at room temperature and covered with fresh-keeping film. After the immersion, filter the methanol, transfer the filter paper bag to the crucible, put it in the drying box at 140°C for 8 h prepare 100 ml, 30 mmol/L ammonia fluoride solution, transfer the dried powder from the filter paper bag to ammonia fluoride solution, and heat it in the water bath at 60°C for 5 h. After heated in the water bath, the supernatant was removed by high-speed centrifugation, the suspension was washed five times by water-ultrasonic centrifugation. Finally, the suspension was vacuum-dried at 140°C for 8 h. The grinding powder was added in two centrifugal tubes to prepare for the characterization experiment. Then the prepared materials were divided into two groups, one was the materials without carbon nanotubes, the other was the composite materials with carbon nanotubes. It was placed in a drying oven at 140°C for 8 h to remove impurities and residual water at a low boiling point, which may be the residual water in the preparation or the water in the air after the preparation. Then it was sealed and cooled to 25°C after drying. 4 ml solution was prepared with 0.5 g, 1 g, 1.5 g, 2 g, 3 g, and 4 g calcium chloride crystals, respectively, the composite materials of mil-101 (Cr) and mil-101 (Cr) and carbon nanotubes were soaked in 100 mg equal amount, respectively. The material was then centrifuged and dried at high temperatures to maintain its constant weight. After the preparation, the degree of calcium chloride adsorbed by mil-101 (Cr) was measured by the balance weighing method, the precision of electronic balance used to measure the weight was 0.001 g. The weight measurement method is shown in Table 1. According to the data, at a certain concentration, with the increase of the concentration of calcium chloride solution, the total weight of the composite increases to a certain extent, the mass fraction of calcium chloride also increases. However, when the concentration of calcium chloride reaches a certain level, the mass fraction of calcium chloride in the composite does not increase with the increase of the concentration of calcium chloride solution.
Characterization of Composite Materials
The X-ray diffraction experiment uses the X-ray diffractometer of Bruker company in Germany, with the model of d8 focus. The power of the X-ray generator is 2.2 kW, Cu Target ceramic X-ray tube. The range of X-ray scanning is -110 to 168 and the angle measurement accuracy is 0.0001° with the maximum scanning speed of 1500°per min. The scanning electron microscope is the s-4800 scanning electron microscope of Hitachi company in Japan. The electron gun and high voltage are cold field guns, and the accelerating voltage is 0.5-30 kv. The secondary electron resolution is 1.0 nm (15 kV) and 2.0 nm (1KV), the backscatter electron resolution, and 3.0 nm (15 kV). The element composition identified in the energy spectrum is be-u with a resolution of 127 ev. Use a 3 h-2000pw adsorption instrument to measure nitrogen adsorption, specific surface area, and pore diameter. The relative pressure range of specific surface area (P / P 0) is 0.01-0.1, and the relative pressure of total pore volume is 0.95. The T-plot method was used to measure micro pore data, and the DFT method was used to measure pore size distribution.
Measurement of Vapor Adsorption and Desorption Capacity of Composite Materials
The static adsorption of water vapor was carried out at 25°C. At the same time, the adsorption kinetics of water vapor can be measured. The adsorption and desorption experiments were carried out at several points between 0.1 and 0.9 relative pressure. The precision of the high precision balance can reach 0.0001 mg by using the weight method. The weight of the sample at different times was measured to analyze the adsorption capacity. Using Fick’s first law of diffusion, the apparent water diffusivity of the sample was estimated. The relative particle radius is 0.001 cm. According to the results of water vapor adsorption, when the time weight stability is 0.1 mg/30 min, the adsorption equilibrium can be determined, the adsorption-desorption isotherm can be drawn. The adsorption temperature was 30°C, the relative pressure was 0.9, and the desorption temperature was 80°C. The results of adsorption and desorption were plotted repeatedly, and the stability image of the adsorption-desorption cycle was obtained.
Naming of Samples in This Study
Because of the large number of samples in this study, we will now give detailed names to the samples.
Materials without any modifier: Mil-101 (Cr).
Materials with carbon nanotubes only:
MWCNT30 mg,MWCNT60 mg,MWCNT90 mg, and MWCNT120 mg.
Materials with calcium chloride only: 0.5 gCacl2, 1 gCacl2, 1.5 gCacl2, and 2 gcacl2.
Materials added to calcium chloride and carbon nanotubes: 30+0.5, 30+1, 30+1.5, and 30+2.
Experimental Results and Discussion
Analysis of Apparent Properties and Vapor Condensation of Carbon Nanotubes Calcium Chloride Mil 101 (Cr) Composite
Generally speaking, the preparation of salt immersion adsorption thermochemical energy storage materials is realized by saturated salt solution and porous material skeleton for a long time. The doping preparation of carbon nanotubes and porous metal-organic framework materials is realized by doping a certain amount of the reactant before hydrothermal reaction. In general, there will be crystal condensation on the surface of porous metal-organic framework materials under the condition of too high a concentration of salt solution (Bernini et al., 2012). At the same time, due to the influence of multi-walled carbon nanotubes, the porous properties of porous materials will change. Therefore, different concentrations of the salt solution should be respectively, impregnated in porous metal-organic materials to study the best concentration. The results show that the salt concentration of the composite increases with the increase of the salt concentration. However, when the salt concentration is high to a certain extent, the mass fraction of salt in the composite decreases inversely (see Table 1). At the same time, it can be seen from the apparent observation that there are more white sand-like substances in the composite material. According to the analysis, we can know that salt crystal condensation occurs at this time. At this time, porous materials can not completely prevent the formation of salt crystals. According to the data analysis, when the concentration of the salt solution is less than 27.3%, there is a positive correlation between the salt absorption rate and the salt concentration, and there is no obvious crystal condensation. At the same time, the analysis is carried out in the thermogravimetric analysis dimension. According to the thermogravimetric analysis experiment at different temperatures, different weight loss mass can be obtained to analyze the mass fraction of salt. According to the results, the mass fraction obtained by thermogravimetric analysis is less than that obtained by the weighing method. At the same time, after adding carbon nanotubes, the mass fraction of calcium chloride increased significantly. The reason is that after washing, the ability of calcium chloride to immerse into the porous materials of mil-101 (Cr) is limited. After adding multi-walled carbon nanotubes, the matrix materials form an excellent pore structure, which expands the pore volume and pore size, which is conducive to accommodate more salt ions to invade the porous matrix. The mass fraction calculated by ICP element analysis can still be seen that the mass fraction of calcium chloride increases greatly after adding carbon nanotubes, which also proves this viewpoint. Therefore, the improvement scheme of CNTs-CaCl2-Mil-101 (Cr) composite has great potential for improving the water absorption performance of the material and improving its chemical heat pump underwater adsorption conditions.
Crystal Properties of Carbon Nanotubes Calcium Chloride Mil 101 (Cr) Composite
Figure 1 shows the XRD of acidified MWCNTs with different quality and mil-101 (Cr) composite, and Figure 2 shows the XRD of mil-101 (Cr) composite after immersion in different concentrations of salt solution. The peaks of mil-101 (Cr) in the above XRD patterns can be seen in the diffraction peaks of carbon nanotubes (Permyakova et al., 2017), indicating that the matrix material has no effect on its structure and related properties under the influence of carbon nanotubes. However, compared with the diffraction peak of mil-101 (Cr) material, the height of the diffraction peak decreases obviously. The XRD peak height of the composite impregnated with calcium chloride also decreases obviously, and with the increase of the concentration of the salt solution, the height of the diffraction peak decreases more and more obvious. For other diffraction peaks, there is no typical diffraction peak of calcium chloride when the salt concentration is less than 30%, but when the salt concentration is higher, there is an obvious diffraction peak of calcium chloride, which indicates that the adsorption capacity of the composite material is saturated, which also corresponds to the analysis of the first part of the apparent characteristics. The decrease of the diffraction peak confirmed the relevant research of others. The first reason is that the mass fraction of porous organic framework decreases due to the addition of salt doping in the composite, and the decrease of the relative content will naturally lead to the nonobvious diffraction peak. The second reason may be that the skeleton structure of mil-101 (Cr) was destroyed by calcium chloride or carbon nanotubes. Before hydrothermal reaction, the addition time of CNTs may affect the synthesis yield of mil-101 (Cr), but it will not destroy the reference structure. In the analysis of calcium chloride, we can wash the composite, and find that there is no obvious difference in the apparent properties or XRD diffraction peak, which shows that the effect of calcium chloride on the composite is reversible. The third possibility is that the structural disorder of composite materials is higher than that of reference materials. This hypothesis needs to be verified by scanning electron microscope images. According to the SEM images (see Figure 3), it is found that the cubic crystal of mil-101 (Cr) material is very clear and pure. Whether it is carbon nanotubes doped or calcium chloride solution impregnated, there are more or less small gritty particles or adhesion phenomenon on the surface of the cubic crystal, the structure becomes fuzzy, the crystal shape deforms and the microstructure becomes chaotic (Ponomarenko et al., 2010). When the amount of carbon nanotubes reaches a certain level, we can see that long needle like carbon nanotubes exist in the crystal in SEM. At the same time, with the addition of carbon nanotubes, the crystal size and the clarity of crystal edge are improved obviously. At the same time, carbon nanotubes and calcium chloride mainly affect the pore properties of the reference frame material. The change of pore properties may lead to the decrease of X-ray diffraction results to the illuminance, and the change of material diffraction characteristics is not obvious. In conclusion, the reason for the decrease of the XRD diffraction peak may be the change of pore structure and properties or the disorder of crystal structure.
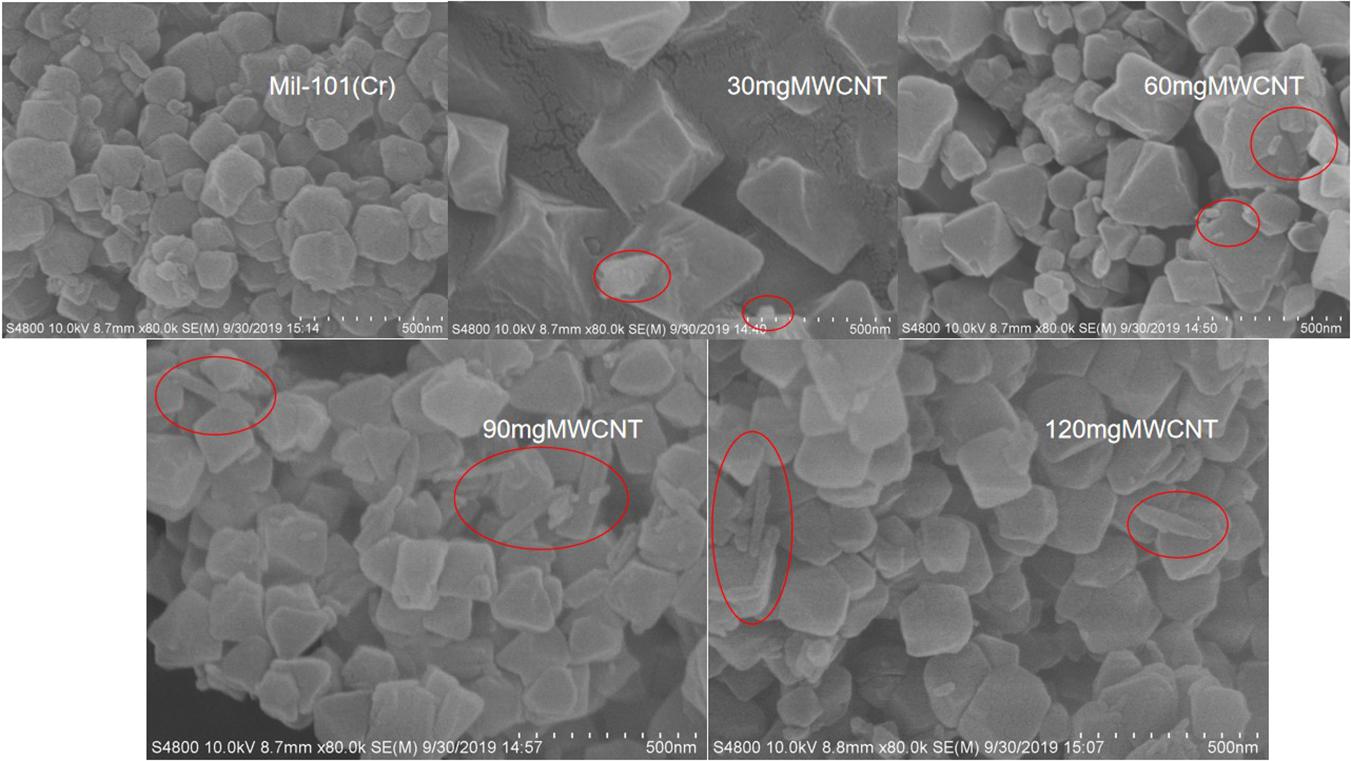
Figure 3. SEM of crystal analysis of carbon nanotubes calcium chloride mil 101 (Cr) composite (The red circle shows the free state of the carbon nanotubes).
Pore Structure Analysis of Carbon Nanotubes Calcium Chloride Mil 101 (Cr) Composite
Supplementary Figures 1, 2 show the adsorption-desorption isotherm and pore size distribution curve of nitrogen. According to the nitrogen adsorption isotherm, compared with the original mil-101 (Cr) nitrogen adsorption isotherm, the nitrogen adsorption curve of all the composites with carbon nanotubes is slightly lower, the more the carbon nanotubes are added, the lower the curve is, but the trend isn’t obvious. Combined with bet and pore volume data, it can be seen that the specific surface area (BET) (see Tables 2–4) increased significantly after adding carbon nanotubes, while the pore size also expanded to a certain extent, but the pore volume reduced relatively in a certain range. According to the pore size distribution diagram, the pore size increases, and the pore size distribution moves to the left to some extent. For analysis, carbon nanotubes are likely embedded in the pore structure, leading to the improvement of the pore properties of porous materials, but at the cost of occupying a certain pore volume. It has a certain improvement effect, but the pore volume occupies too much, so it needs some other means to improve the adsorption performance of the material to make up for the loss of pore volume. In the experiment of calcium chloride doping, no matter how much concentration of calcium chloride solution is impregnated, the nitrogen adsorption isotherm of the composite material is decreased. Bet data, pore size data, and pore volume data are all significantly reduced, the pore size distribution is greatly shifted to the left. Because porous materials have a strong affinity for calcium chloride, most of the micropores are filled with a large number of calcium chloride particles. It can be seen from the XRD results that the specific surface area, pore diameter, and pore volume data all confirm this point. With the increase of the concentration of the solution, the amount of calcium chloride particles entering the pore increased significantly. When the capacity of the pore reached saturation, the absorption is not obvious. Secondly, the particle radius of calcium ion in calcium chloride is large, which occupies the pore volume more obviously. At the same time, with the invasion of large particles, the influence of the contrast surface area shows a cliff-like decline. Considering the characteristics of calcium chloride, high water absorption, and excellent hydrophilicity. The honeycomb-like structure is formed by the calcium chloride particles and the porous material. In the porous structure of the porous metal-organic framework materials, the water absorption capacity, the dynamic property of water absorption, and the total amount of water adsorption increase when the relative pressure is low. However, the pore properties of porous materials will be Compromised when salt particles invade. Similar to the honeycomb structure, it provides more capacity. Excellent porous reference materials and excellent hydrophilic materials embedded together constitute excellent thermochemical energy storage materials. There is another problem here. The water adsorption performance of porous metal-organic framework materials is determined by the specific surface area of pore diameter, while the water absorption performance is determined by the hydrophilic absorbent salt and content. However, the contradiction is that a high concentration of salt will greatly affect the improvement of pore performance. Therefore, a balance should be found to optimize the water adsorption performance of the composite material for the contradiction between the porous property and the content of water-absorbing salt. After adding different amounts of calcium chloride, the structure of MOFs materials will not change. Because calcium chloride ions are attached to the organic porous framework in the composite material. The addition of carbon nanotubes is to optimize the pore structure in order to provide a better adsorption environment for calcium chloride. However, the maximum amount of calcium chloride in this study has reached the maximum pore carrying capacity of MOFs. Excessive free calcium chloride will cause large deviations in the characterization data of the material. At the same time, the environment where excess calcium chloride reacts with water under multiple water adsorption environments will destroy the organic porous framework material and destroy the stability and balance of the material. At the same time, since the affinity of calcium chloride for water and nitrogen is much greater than the pore structure of MOFs, the structure shown in the characterization experiment will change greatly.
Micro Crystal Morphology Analysis of Carbon Nanotubes Calcium Chloride Mil 101 (Cr) Composite
Figure 3 shows the scanning electron microscope images (SEM) of each group of composite materials as described above. According to the analysis of the images, it is concluded that the base version of mil-101 (Cr) material is a cubic crystal with a clear outline, pure crystal, similar octahedral shape, and clear boundary between crystals. There is no obvious bonding phenomenon, and the contour can be distinguished. The cubic crystal of mil-101 (Cr) material can still be seen in the MWCNT doped composite, and small particles can be found around the cube. With the addition of CNTs, the volume of grains increases. At the same time, with the increase of the number of carbon nanotubes added, the morphology of SEM images did not change significantly. It can be seen from the observation of the composite impregnated with calcium chloride that the clear crystal profile can still be seen for the composite with low salt solution concentration. A small number of salt particles can be seen but not obvious. However, with the increase of salt concentration, the crystal conglutinates, the crystal outline is not clear, the crystal is deformed and the shape is irregular. A large number of other particles are seen on the crystal surface. After analysis, we know that the calcium chloride particles enter into the pore structure of the porous metal-organic skeleton base, and some of them attach to the skeleton surface, resulting in the above changes in the microstructure.
Analysis of Water Adsorption Properties of Carbon Nanotubes Calcium Chloride Mil 101 (Cr) Composite
Figures 4–6 shows the adsorption-desorption isotherms of the above composite materials. For the water adsorption properties, whether it is hydrophilicity or total adsorption capacity, the material modified by carbon nanotubes doping alone has no obvious effect (Zhang et al., 2017). Generally, the adsorption isotherm is divided into three parts. The first part is that under the low water pressure level, the water adsorption capacity of the material is low and the increase of water adsorption capacity is not obvious with the increase of water pressure at this stage. The second stage is the stage in which the water adsorption suddenly increases with the increase of water pressure. In the image, there is a climbing curve with a large slope. The higher the climbing curve to the left, the better the hydrophilicity of the material. Good hydrophilicity means that under low water pressure, thermochemical energy storage materials have strong applicability and mild conditions, which is conducive to practical application. The third stage is the stage of high water pressure, in which the water absorption remains at a high level and the water absorption does not increase significantly with the increase of water pressure. On the image, there is a high horizontal curve. The image height of this part means the total water absorption of the material. The larger the total water absorption is, the greater the potential of the material to be used as an adsorption thermochemical energy storage material, and the more worthy of improvement. Indicated by the images, it is found that the high total amount of water adsorption and the good hydrophilicity of the reference plate mil-101 (Cr) indicate that this kind of porous material is an excellent material, and the improvement of adding carbon nanotubes does not significantly improve the water adsorption performance. However, we can see that the desorption curve shows that the separation between the adsorption-desorption curves of the material modified by carbon nanotubes is significantly smaller than that of the reference material. This shows that the water adsorption-desorption cycle capacity of the modified material is significantly improved, which is conducive to the use of the material as a circulating heat pump. The reason may be that the micropore structure of the material has been improved. Although the addition of other substances will reduce the affinity and adsorption of water, the greatly improved pore performance is conducive to the improvement of water absorption by adding superabsorbent salt.
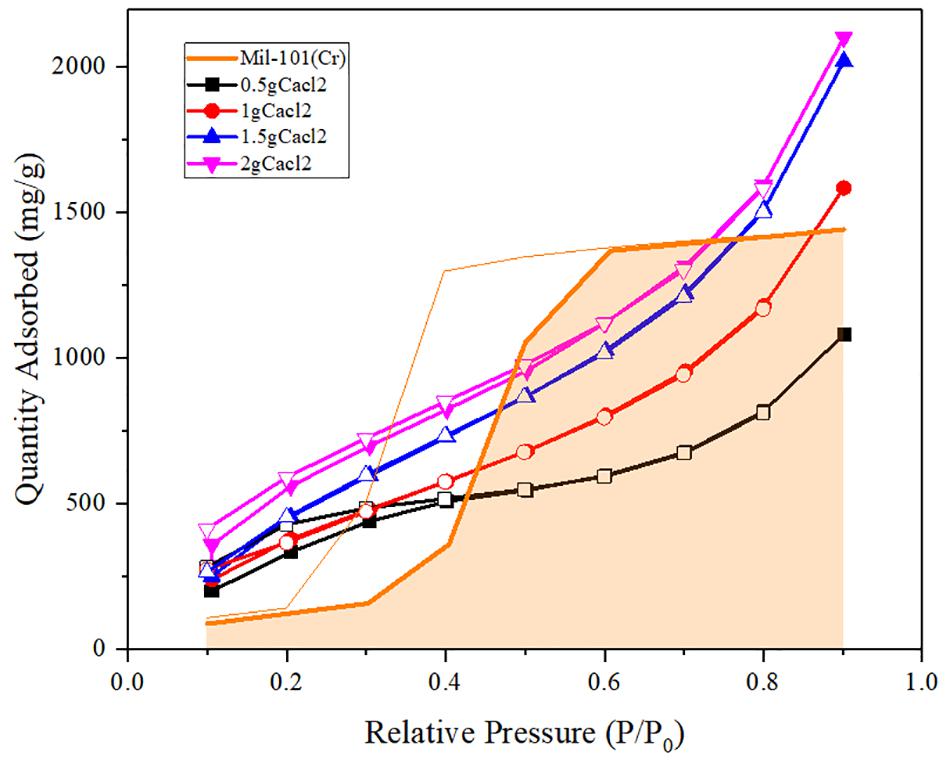
Figure 4. Water adsorption isotherms of calcium chloride mil-101 (Cr) composite compared with mil-101 (Cr) composite.
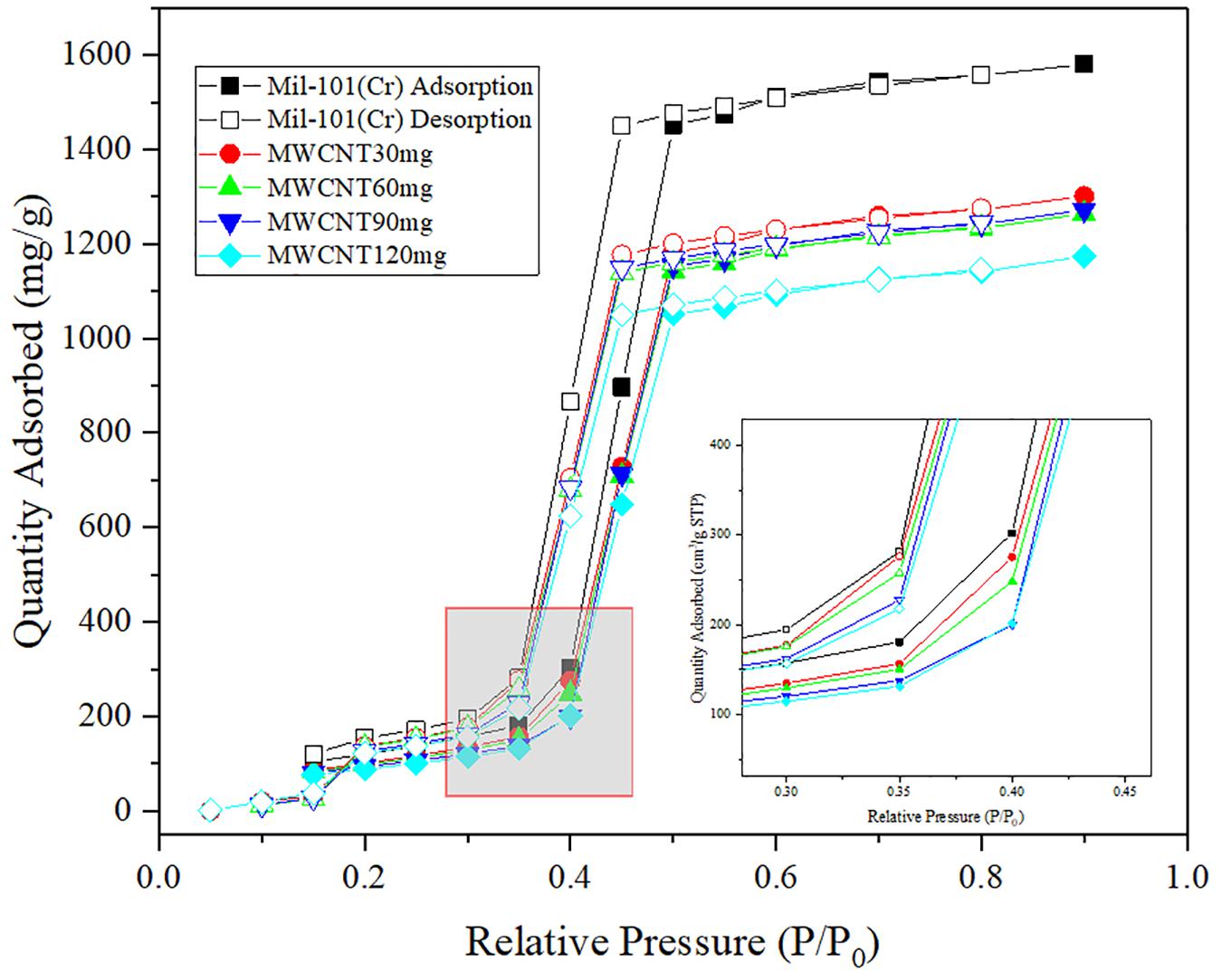
Figure 5. Comparison of water adsorption isotherms of carbon nanotube mil-101 (Cr) composite with mil-101 (Cr) composite.
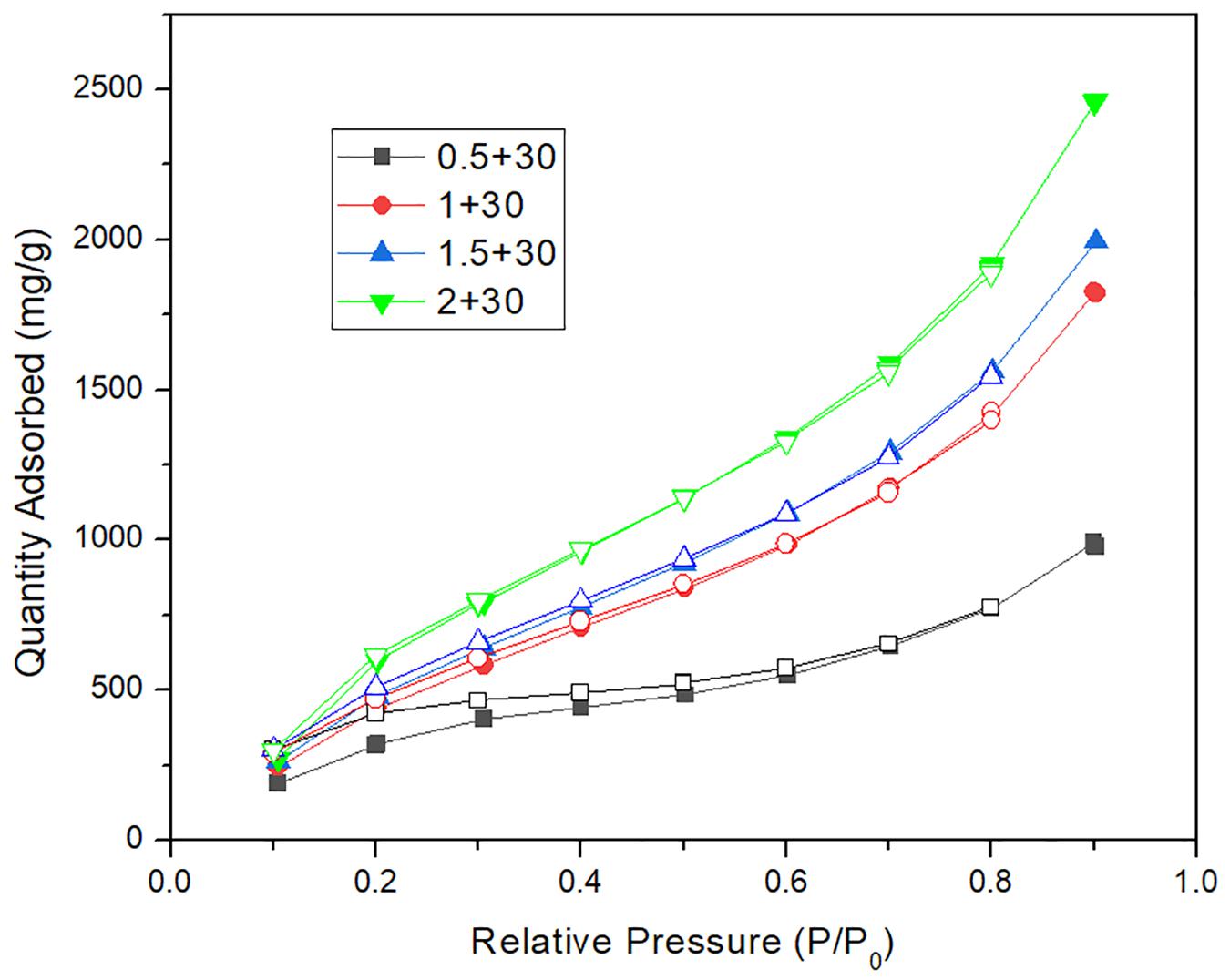
Figure 6. The comparison of water adsorption isotherms between calcium chloride carbon nanotubes mil-101 (Cr) composite and mil-101 (Cr) composite.
It can be seen from the analysis of the composite impregnated with calcium chloride that the adsorption capacity of the material in low and medium partial pressure water is significantly increased after adding calcium chloride, which may be due to the hydrophilic effect of calcium chloride itself. However, when the concentration of the salt solution used for impregnation is low, and the water pressure is about 0.5, the water adsorption capacity of impregnated calcium chloride is not as large as that of the reference porous material itself. Combined with the specific surface area, pore size, and pore volume data, we analyzed that under low concentration immersion, there were not enough calcium chloride particles in the pores of porous materials. Although the water adsorption capacity of calcium chloride itself is large, the amount of calcium chloride in the pore is small. At the same time, the invasion of calcium chloride leads to the degradation of pore properties, resulting in the total adsorption less than the reference mil-101 (Cr) material. When the concentration of the salt solution is higher than a certain degree, the pore diameter will decrease and capillary force will appear, which will increase the siphon force on the water. Besides, a large number of calcium chloride itself has a great absorption capacity for water, therefore, the total adsorption capacity is significantly increased. To sum up, increasing calcium chloride has a significant effect on improving the water adsorption capacity of the composite under low water pressure. At the same time, when the concentration of calcium chloride reaches a certain degree, adding calcium chloride can also significantly increase the total water adsorption capacity. It is worth noting that although calcium chloride itself has strong hydrophilicity and large water adsorption capacity, its water adsorption-desorption cycle capacity is poor. adsorbed and desorbed process of water will make the properties of the material unable to return to the original state. However, we found that the coincidence between the water adsorption-desorption curves was very high in the improved composite, which indicated that the water adsorption-desorption cycle ability of the material was strong. In our analysis, the addition of calcium chloride may cause a capillary effect in the pores, which improves the cycling ability of mil-101 (Cr) material itself. At the same time, the porous framework of porous material is conducive to the water desorption of calcium chloride material, and the effect of the framework finalization is better in the desorption experiment. The two materials interact with each other during desorption, improving jointly improve the cycle capacity of water adsorption-desorption of the composite material and make the heat pump cycle potential of the material greater.
Water Adsorption Rate and Kinetics of Carbon Nanotubes Calcium Chloride Mil 101 (Cr) Composite
In addition to the angle of water adsorption capacity and adsorption isotherm, it is also important to evaluate the adsorption efficiency and kinetics of water vapor. According to the adsorption rate and adsorption kinetic curve drawn by water adsorption capacity and adsorption time (Yu et al., 2011), the ratio of instantaneous absorption rate and equilibrium absorption rate (Mt / Me) and t0.5 were drawn after data processing. It can be seen from the image that when the reference plate mil-101 (Cr) is modified by carbon nanotubes, the adsorption rate of the composite with carbon nanotubes is always lower than that of the reference plate porous skeleton material. In the early stage, the adsorption rate of the composite with calcium chloride is generally higher than that of the reference plate, and in the later stage, the adsorption rate is slowly reversed by the reference plate. The reason for this phenomenon may be that carbon nanotubes improve the material by improving the pore properties, which is a kind of degradation of the hydrophilicity of the material itself but is more conducive to loading more absorbent salts. In the early stage, the material with water-absorbent salt can take advantage of the super high hydrophilicity of calcium chloride itself to lead the unmodified material in speed. However, due to a large amount of water adsorption of calcium chloride, the saturated adsorption rate of the material is lower than that of the reference organic metal skeleton material.
Using Fick’s first law of diffusion mentioned above, the data for calculating the diffusion coefficient is obtained by mathematical linear fitting. The diffusion data values are shown in Tables 5, 6. According to the data, the total diffusion coefficient of the two kinds of modified materials decreased significantly (Valencia and González, 2011). For carbon nanotubes, the modification itself does not change the main adsorption principle of the composite for water. The adsorption is still carried out through the surface and pore characteristics of the skeleton material. This modification did not change the adsorption properties of the material mainly through physical adsorption. The addition of carbon nanotubes is not conducive to the diffusion of water, and the water diffusion coefficient of natural materials is reduced. The material modified by calcium chloride results in the change of adsorption principle from physical adsorption to chemical adsorption. Analysis of the adsorption rate curve shows that the high rate in the early stage of adsorption is mainly due to the water adsorption of calcium chloride. In the late stage of adsorption, the adsorption rate of calcium chloride does not play a leading role (Khutia et al., 2013; Yu et al., 2014). The important factors affecting the rate are the capillary siphonage in the pore space of porous materials and the capillary condensation. From the microscopic point of view, the intermolecular force of hydrogen bonding between water molecules is smaller than that between water vapor and composite materials. This explains why the diffusion coefficient changes correspondingly.

Table 5. Water adsorption diffusion coefficient of calcium chloride mil-101 (Cr) composite and mil-101 (Cr) material.
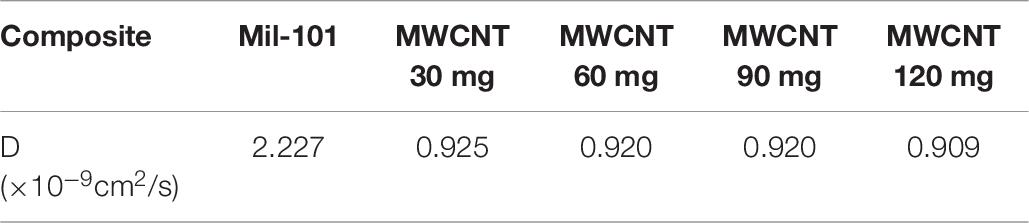
Table 6. Water adsorption diffusion coefficient of carbon nanotube mil-101 (Cr) composite and mil-101 (Cr) material.
Desorption Properties of Carbon Nanotubes Calcium Chloride Mil 101 (Cr) Composite
The desorption kinetic data of adsorbent can be obtained by TGA (Yu et al., 2014). Through DTG curves at different temperatures, the activation energy of desorption can be calculated by using the Kissinger equation (see Supplementary Figures 3–6). The peak value of desorption value was calculated by the equation, and the desorption activation energy was obtained by linear fitting and integrated calculation. The heating rate can be obtained by thermogravimetry experiment, and the temperature at this time can be obtained by reading the maximum desorption rate. According to the results, the activation energy of the composite is higher than that of the basic mil-101 (Cr) material (see Supplementary Tables 1, 2). The desorption activation energy of the material will increase to a certain extent when the carbon nanotubes are added alone. When the calcium chloride is added alone, the desorption activation energy will increase significantly, and when the salt is added too much, the desorption activation energy of the material will decrease. When carbon nanotubes are added to the composite composed of calcium chloride and mil-101 (Cr), the desorption activation energy increases significantly. However, the desorption activation energy of the composite is lower than that of the composite without carbon nanotubes. Through our analysis and literature review, the reason for this phenomenon may be related to the existence of superabsorbent salts in composite materials. In general, the adsorption of water by metal-organic framework materials mainly depends on the interaction between water molecules and metal-organic ligands and the interaction of hydrogen bonds between water molecules. When some ionic salts infiltrate into porous materials, the adsorption water molecular correlation potential of porous materials will be affected by the infiltrated materials, which will affect the interaction between materials and water molecules and the interaction between materials and hydrogen bonds. Therefore, in the desorption activation energy data, because the related force and interaction tendency of materials to water is disturbed, the desorption activation energy of composite materials is reduced. At the same time, the addition of carbon nanotubes will promote the absorption of calcium chloride, and the existence of calcium chloride will improve the porous structure of carbon nanotubes. However, if the doping ratio of the two materials is not reasonable, the performance of the two materials will be degraded. Combined with the above, the composite material after the modification has high activation energy, large adsorption capacity, high adsorption rate, and more reasonable operating temperature, so such modification becomes more promising for the application of materials in the adsorption heat pump.
Element Content Analysis of Carbon Nanotubes Calcium Chloride Mil 101 (Cr) Composite
Based upon the above analysis, it is concluded that the reasonable combination of carbon nanotubes calcium chloride mil-101 (Cr) composite material can be conducive to the adsorption improvement of the adsorbent for water. At the same time, the hydrophilicity of the material can be increased. At the same time, the material has strong water adsorption capacity, good thermal stability, and adjustable desorption capacity. The yield of the material is improved obviously. To explore the interaction of composite materials and their doping ratio, the ICP experiment of element content ratio analysis was carried out for the materials prepared in the front. The impurities in the material are removed by strong acid treatment, heated quickly and fully dissolved, cooled to room temperature, added with the appropriate amount of dilute hydrochloric acid to adjust the pH value, and finally sent to the ICP experimental instrument for quantitative analysis of elements. See Table 7 for the results of element analysis. To explore the effect of carbon nanotubes on the doping of calcium chloride, the element ratio of Ca and Cr were analyzed to explore their interaction. The results show that the mass fraction of the two elements is lower than that of the carbon nanotubes. The addition of carbon nanotubes can promote the stable attachment of calcium chloride, which has a stronger water adsorption capacity, to porous materials, thus significantly improving the hydrophilicity of the maximum water adsorption capacity. The addition of CNTs increased the stability of the composite, and the mass fraction of effective elements was significantly improved after acid treatment. Moreover, the upper saturation limit of calcium chloride doping increases after adding carbon nanotubes, which is beneficial to improve the properties of the materials. In the future, it has great potential to be used in the adsorption heat pump.
Dynamic Water Adsorption Properties of Carbon Nanotubes Calcium Chloride Mil-101 (Cr) Composite
To understand the water adsorption performance of CNT-CaCl2-mil-101 (Cr) composite under the actual working condition, this paper simulates the actual working condition and uses the water vapor nitrogen mixture composed of 32% water vapor to dynamically purge the completely dehydrated material. The vacuum dehydration temperature is 150°C, the purging temperature is 30°C, and Figure 7 shows the 0-550 min dynamic water adsorption capacity time curve of calcium chloride mil-101 (Cr) composite. Supplementary Table 3 shows the maximum dynamic water adsorption capacity of calcium chloride mil-101 (Cr) composite. Figure 8 shows the dynamic water adsorption capacity time curve of carbon nanotube calcium chloride mil-101 (Cr) composite, and Supplementary Table 4 shows the maximum dynamic water adsorption capacity of carbon nanotube calcium chloride mil-101 (Cr) composite. It is worth noting that the experimental data of dynamic adsorption simulated by the actual working conditions are affected by the macro morphology and quality of the material. The curve is used to analyze the dynamic adsorption rate to determine the expected working capacity of the composite under the actual low partial pressure working conditions. The maximum dynamic adsorption capacity can reflect the dynamic adsorption potential of the material. Analysis of various curves and tables shows that the dynamic maximum water adsorption capacity and hydrophilicity are not as good as the static adsorption experiment under the ideal state. At the same time, it is worth noting that in the sample only added with calcium chloride crystal, when the addition amount of calcium chloride is too small, the excellent water affinity of calcium chloride and the water affinity of the porous structure of metal-organic framework material itself are combined, and the two are superposed It is consistent with the excellent hydrophilicity of the material in the dynamic water adsorption test. However, its maximum water adsorption capacity is not enough, which is not conducive to the application of composite materials in actual conditions. When the concentration of calcium chloride reaches a certain amount, the chemical affinity between calcium chloride and water is obvious. The water affinity of the porous structure is not obvious due to the invasion of calcium chloride. Therefore, the greater the amount of calcium chloride, the greater the water affinity and the total amount of water adsorption. When carbon nanotubes are added, the improvement of the pore structure by carbon nanotubes and the invasion of calcium chloride to the pore structure are combined. We can see that 1 g of calcium chloride with 30 mg of carbon nanotubes form the best water affinity and water adsorption capacity. In addition to 0.5 g calcium chloride, the hydrophilicity and the maximum water adsorption capacity of the other groups of samples increased after adding carbon nanotubes. Because the addition of carbon nanotubes provides a better pore structure for calcium chloride, there are more calcium chloride ions in the pore structure. Therefore, the composite material has better water adsorption performance. The results of dynamic adsorption show that the applicable type of composite is worthy of expectation.
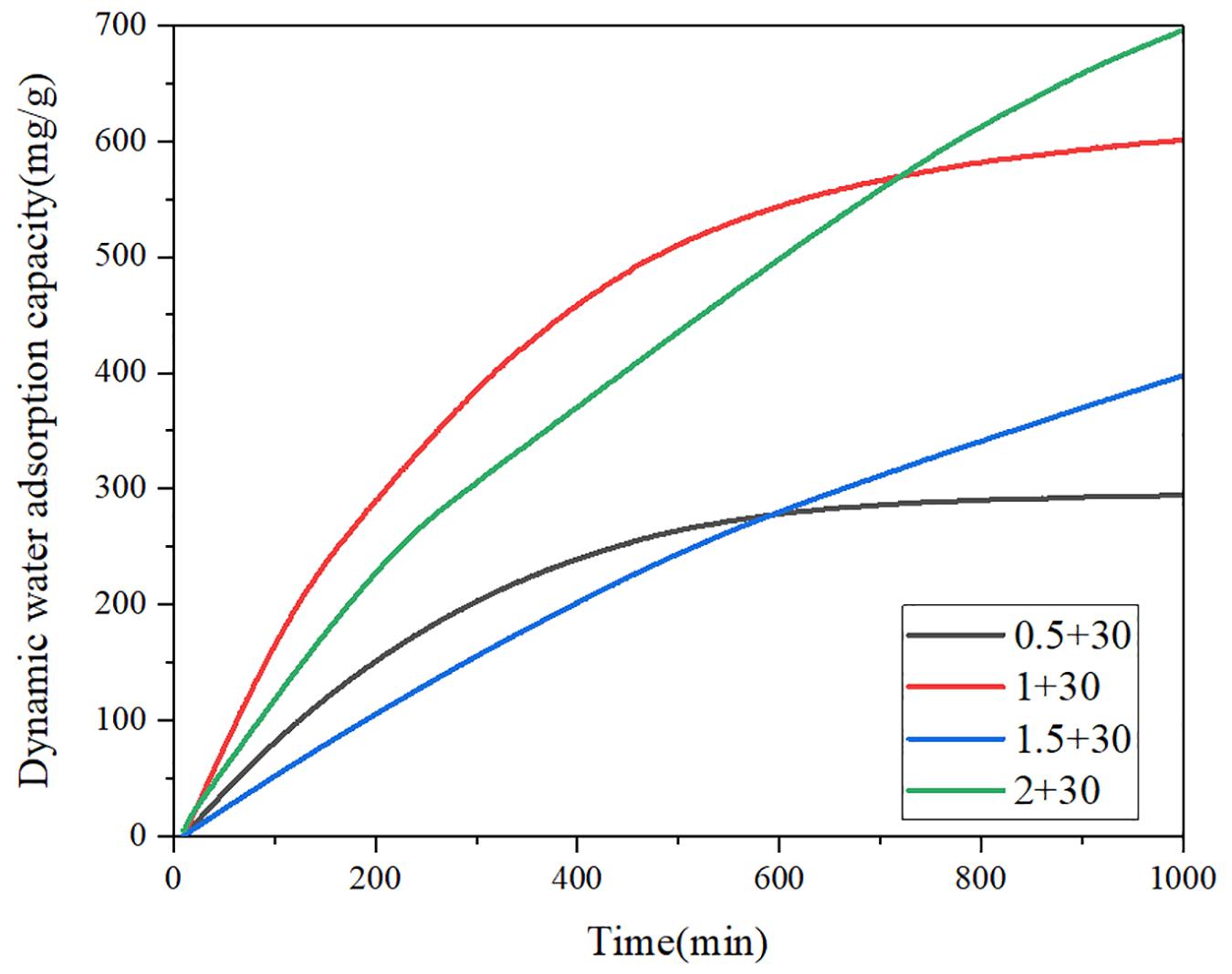
Figure 8. Dynamic water adsorption curve of carbon nanotubes calcium chloride mil 101 (Cr) composite.
Water Adsorption Cycle Stability of Carbon Nanotubes Calcium Chloride-Mil-101 (Cr) Composites
Figures 9, 10 show the variation of maximum adsorption capacity and the comparison of adsorption rate after 10 water adsorption and desorption cycles. As can be seen from the figure, after about 7 water cycles, the maximum water absorption of the composite reaches a peak value, but when the number of cycles continues to increase, the maximum water adsorption capacity decreases significantly. At the same time, we can observe that the shape of the cycle rate curve of the second cycle is similar to that of the tenth cycle, but the height of the curve decreases with the increase of the number of cycles, and the starting point of the curve increases greatly with the increase of the number of cycles. Observing the experimental samples, we can see that white powder appears with the increase of the number of cycles. By analyzing the reasons, it can be seen that the water adsorption cycle stability of NMCNTs-CaCl2-mil-101 (Cr) composite is good, and the automatic optimization phenomenon of materials appears in the initial cycle. Under the limit condition, the maximum water adsorption capacity decreased slightly after 7 cycles, and the shape of the curve is stable and the morphology of the material is stable. This kind of composite material has good adsorption stability underwater adsorption condition, which can meet the technical requirements of the heat pump in adsorption thermochemical energy storage conditions. It is a thermochemical energy storage material with the potential for automatic circulation. The practical application prospect of this material is broad. Figure 11 shows the simulation of actual heat pump conditions, adsorption at 0.32 partial pressure and 30°C, desorption at 0.32 partial pressure, and 80°C. The curve of each working condition is simulated for 100 min. Figure 12 shows the histogram of maximum adsorption capacity and adsorption times under the above actual conditions. Figure 13 shows the adsorption curves of the first adsorption and the tenth adsorption under the above actual conditions. According to the above experimental results, the heat pump cycle stability of the composite material is good under the simulated actual working conditions. With the increase of adsorption times, the cycle capacity decreases slightly, but it is not obvious. The results show that the composite material has good stability under actual working conditions. This material is an excellent alternative material for adsorption heat pumps underwater adsorption condition.
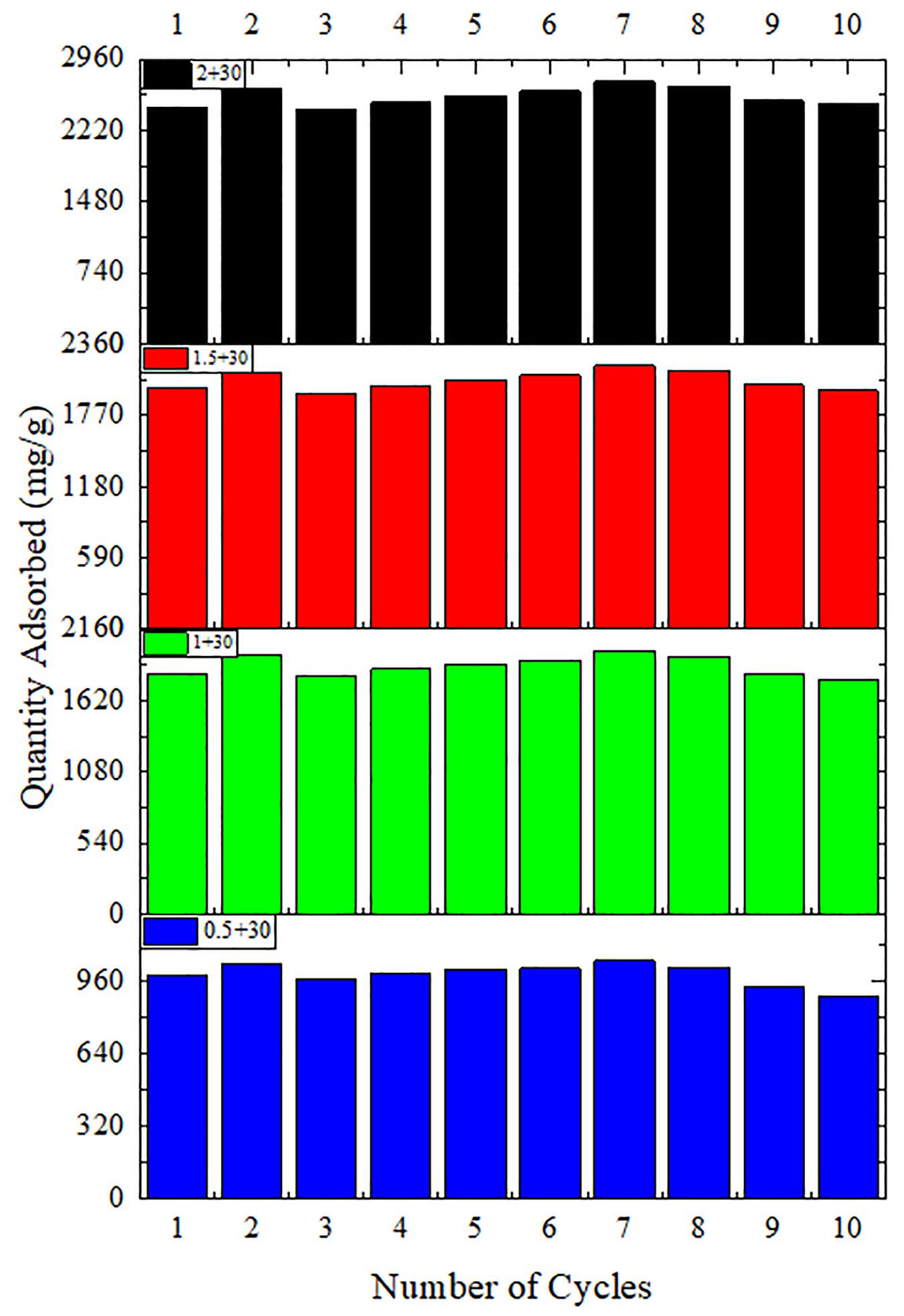
Figure 9. Histogram of maximum water adsorption capacity of composites in 10 adsorption desorption cycles.
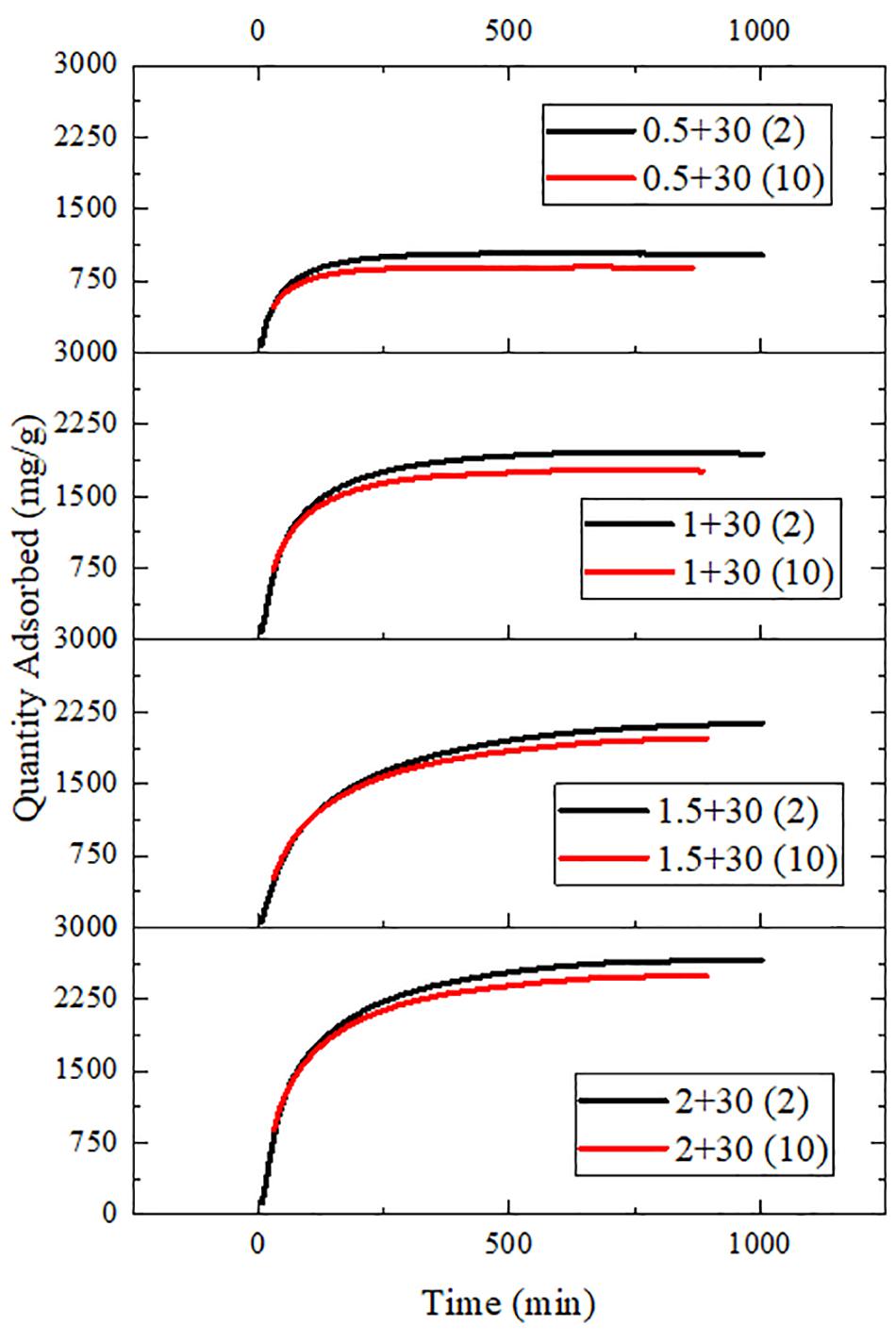
Figure 10. Comparison of adsorption rate of composites in the second and tenth water adsorption cycles.
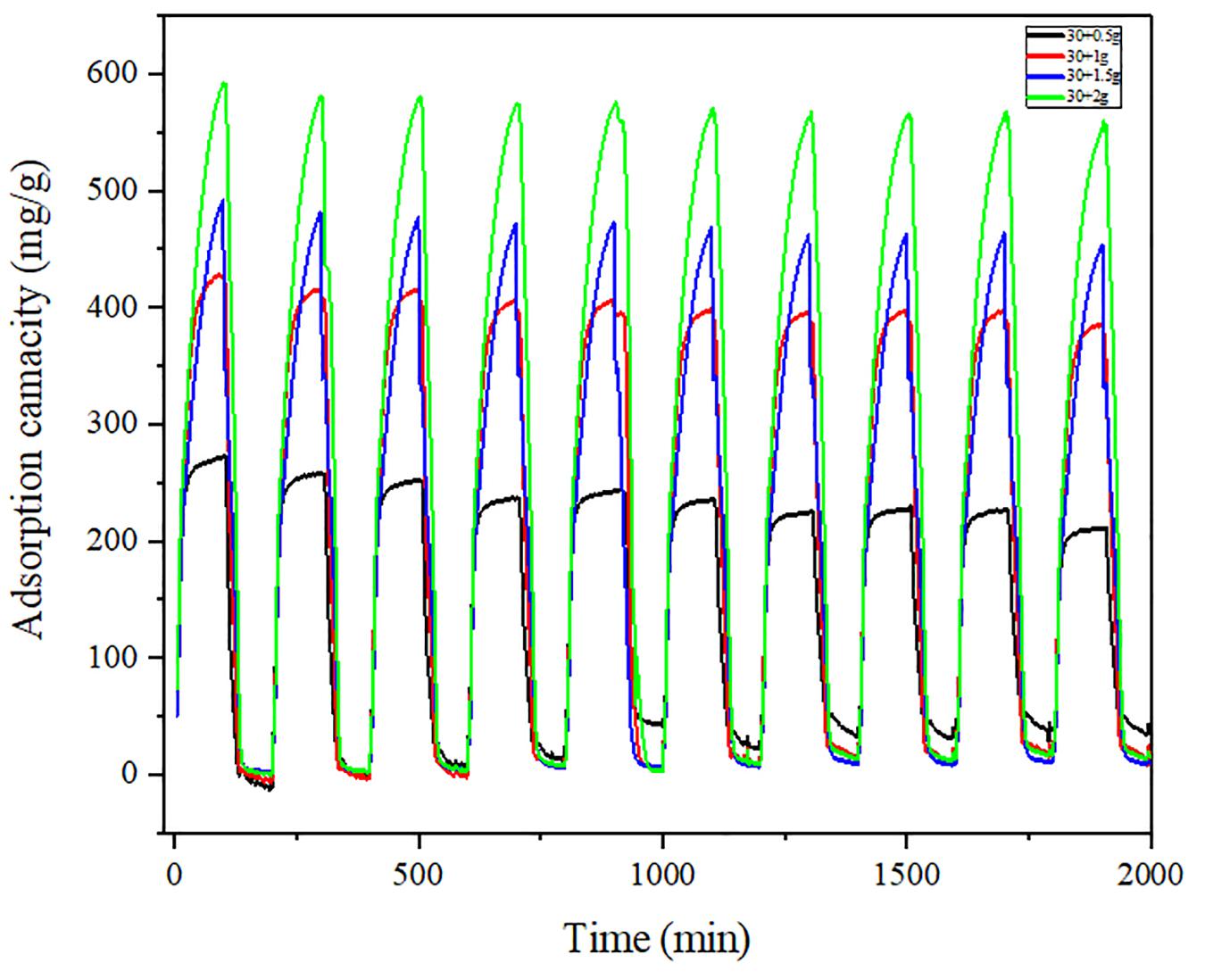
Figure 11. Adsorption desorption cycle curve under actual working conditions (100 min for each process).
Total Energy Storage Capacity and Energy Storage Density of Carbon Nanotubes Calcium Chloride-Mil-101 (Cr) Composites
The heat storage density of the material is the heat storage capacity per unit volume of material. Its value depends on the difference of enthalpy of adsorption, the ability of endothermic and exothermic cycles, and the macroscopic bulk density of materials. The value depends on an integral function of the three. Macroscopically, this parameter depends on the difference of enthalpy of adsorption and the lift force of cyclic loading. The energy storage capacity of materials is also the energy storage capacity per unit mass of materials. This value only depends on the difference of enthalpy of adsorption and the capacity of materials to absorb and release heat. In the calculation of theoretical energy storage density and total heat storage capacity, the process of calcium chloride crystal water quantity from 6 to 1 and the physical adsorption of MOFs materials constitute the theoretical heat storage capacity. According to the phase diagram of calcium chloride, calcium chloride crystal lost crystal water three times underrated condition (30-80°C). The adsorption heat value of the material was measured by the endothermic and exothermic cycling ability of the material. It can also be converted into the average value of the integral of enthalpy change corresponding to unit adsorbent mass adsorption and enthalpy change integral corresponding to unit adsorbent mass desorption. It includes chemical enthalpy of chemical reaction with adsorbate and phase change enthalpy of physical change, endothermic and exothermic circulation capacity of materials, which is the total exchange mass of materials in the cycle. The final energy storage density is the product of heat storage and material bulk density. The results show that the heat storage density of the composites is higher than that of the composites without CNTs. At the same time, it should be noted that after the addition of carbon nanotubes, the amount of calcium chloride and heat storage density increase threshold value, which can accommodate more salt, is more conducive to the use of adsorption heat pump in water adsorption condition.
It should be noted that the difference between the first cycle and the tenth cycle decreases with the increase of salt content when calcium chloride is added into the organic framework material alone, and the difference is huge at low salt content. When carbon nanotubes were added to immobilize inorganic salts, the cycle difference at low salt content was significantly improved. The analysis shows that the loading capacity and stability of the organic framework for inorganic salt particles are enhanced after adding carbon nanotubes. At low concentration, the loss performance becomes lower, which indicates that the addition of carbon nanotubes can significantly reduce the adsorption loss of inorganic salts. This method is conducive to the stable composite of organic framework materials and inorganic materials, and is conducive to the application of materials in the actual conditions of thermochemical energy storage (Figure 14).
Conclusion
In this study, we used carbon nanotubes and calcium chloride to impregnate and mix mil-101 (Cr) to form a new composite. Through the preparation of materials, analysis of the composition of materials, observation of the structure and micro morphology of materials, and determination of the properties of materials, the following conclusions are drawn.
(1) Adding proper amount of acidified MWCNTs and calcium chloride crystals to mil-101 (Cr) material can significantly improve the water adsorption performance of the material, including water affinity, total water adsorption amount, water adsorption cycle stability, etc. In this study, the optimal salt concentration is about 27.3%. Theoretically, each mole of mil-101 (Cr) material doped with about 3 g of acidified MWCNTs can achieve excellent water adsorption materials.
(2) After adding calcium chloride crystal, the diffraction peak intensity of mil-101 (Cr) decreases obviously, and no obvious diffraction peak of calcium chloride is observed. After adding calcium chloride, the specific surface area, pore size and pore volume of the material decreases significantly, and the micro morphology becomes irregular. After adding carbon nanotubes, the diffraction peak intensity of mil-101 (Cr) has little effect, no obvious diffraction peak of carbon nanotubes is seen, the specific surface area, pore diameter and volume of the material increase significantly, the micro morphology changes little, the crystal size decreases, the shape is more regular. Carbon nanotubes can significantly improve the pore properties of porous materials, the invasion of calcium chloride requires more pore resources. The combination of the two can obviously increase the doping amount of calcium chloride in the composite, greatly improve the performance of the material by using the excellent water adsorption property of the hygroscopic salt.
(3) Because of the common force of the siphon effect of the water affinity of calcium chloride, the curve climbing point of the water vapor adsorption isotherm of the composite moved to the left and the maximum value of the curve increased significantly. After adding a certain amount of carbon nanotubes, the adsorption capacity of the material is further improved. Much higher than the basic version of mil-101 (Cr) material.
(4) The pore size and pore size distribution of the composite are significantly improved, the water adsorption rate is increased. However, the diffusion coefficient of the material decreases. The diffusion coefficient of the material is 1.112 × 10–9cm2/s after adding calcium chloride, and 0.925 × 10–9cm2/s after adding carbon nanotubes. It shows that the main reason for the adsorption mechanism of the composite material is that the main adsorption of the siphon hole at the physical level is the combination of pure physical adsorption and chemical adsorption. The water adsorption capacity of the material is greatly improved.
(5) The addition of appropriate amount of calcium chloride makes the material exhibit stronger affinity for water molecules to some extent. However, the addition of excessive calcium chloride leads to the deterioration of material properties and the weakening of water affinity. At the same time, the addition of carbon nanotubes makes the material more water compatible by addition of calcium chloride. Adding calcium chloride can increase the water affinity by 58.6% in micro theory, adding carbon nanotubes can further increase the water affinity of calcium chloride composite to 77.4% in theory.
(6) The addition of carbon nanotubes can promote the stable attachment of calcium chloride, which has stronger water adsorption capacity, to the porous materials, thus significantly improving the hydrophilicity of the maximum water adsorption capacity. Moreover, the stability of the composite increases with the addition of CNTs. The results show that the stable doping amount of calcium chloride can be increased by 78.2% with the addition of carbon nanotubes.
Carbon nanotube calcium chloride mil-101 (Cr) composite has excellent water adsorption capacity under low water pressure, and has excellent maximum water adsorption capacity. The preparation cost is low, the preparation difficulty is low, the stability is good. This kind of material can be an excellent alternative material for adsorption heat pump in the future. At the same time, this kind of multi doping composite will improve the pore properties of porous materials and increase the content of hydrophilic materials, which will provide valuable ideas for the future research of new adsorption energy storage materials.
Data Availability Statement
The raw data supporting the conclusions of this article will be made available by the authors, without undue reservation.
Author Contributions
LL wrote the manuscript, the experiments, and the data processing. MX directed the study. XH provided the project and the funding. CH and ZL helped with the study. All authors contributed to the article and approved the submitted version.
Funding
This work was supported by the National Natural Science Foundation of China (No. 51836009).
Conflict of Interest
The authors declare that the research was conducted in the absence of any commercial or financial relationships that could be construed as a potential conflict of interest.
Supplementary Material
The Supplementary Material for this article can be found online at: https://www.frontiersin.org/articles/10.3389/fmats.2021.653933/full#supplementary-material
References
Abedin, A. H. (2011). A critical review of thermochemical energy storage systems. Open Renew. Energy J. 4, 42–46. doi: 10.2174/1876387101004010042
Bernini, M. C., Platero-Prats, A. E., Snejko, N., Gutiérrez-Puebla, E., Labrador, A., Sáez-Puche, R., et al. (2012). Tuning the magnetic properties of transition metal MOFs by metal–oxygen condensation control: the relation between synthesis temperature, SBU nuclearity and carboxylate geometry. Crystengcomm 14:5493. doi: 10.1039/c2ce06563k
Demir, H., Mobedi, M., and UElkue, S. (2013). A review on adsorption heat pump: problems and solutions. Renew. Sustain. Energy Rev. 12, 2381–2403. doi: 10.1016/j.rser.2007.06.005
Deshmukh, H., Maiya, M. P., and Srinivasa Murthy, S. (2016). Study of sorption based energy storage system with silica gel for heating application. Appl. Therm. Eng. 111, 1640–1646. doi: 10.1016/j.applthermaleng.2016.07.069
Henninger, S. K., Jeremias, F., Kummer, H., and Janiak, C. (2012). MOFs for use in adsorption heat pump processes. Eur. J. Inorg. Chem. 2012, 2625–2634. doi: 10.1002/ejic.201101056
Iguchi, H., and Kato, Y. (2016). Ammonia Adsorption Ability of Calcium Chloride and Expanded Graphite Composite for Thermochemical Energy Storage. Tokyo: The Japan Society of Mechanical Engineers.
Khutia, A., Rammelberg, H. U., Osterland, T., Henninger, S. K., and Janiak, C. (2013). Water sorption cycle measurements on functionalized MIL-101Cr for heat transformation application. Chem. Mater. 25, 790–798. doi: 10.1021/cm304055k
Leng, K., Sun, Y., Xiaolin, L., Sun, S., and Xu, W. (2016). Rapid synthesis of metal–organic frameworks MIL-101(Cr) without the addition of solvent and hydrofluoric acid. Cryst. Growth Des. 16, 1168–1171. doi: 10.1021/acs.cgd.5b01696
Meunier, F. (1985). Second law analysis of a solid adsorption heat pump operating on reversible cascade cycles: application to the zeolite-water pair. J. Heat Recov. Syst. 5, 133–141. doi: 10.1016/0198-7593(85)90045-1
Neumann, N., Zhao, D., Wokon, M., and Linder, M. (2016). Particle stability investigation of Mn-Fe oxides supported by TiO2, ZrO2 or CeO2 as thermochemical energy storage materials. Mater. Sci. Eng. Cong. 2016, 27–29.
N’Tsoukpoe, K. E., Schmidt, T., Rammelberg, H. U., Watts, B. A., and Ruck, W. K. L. (2014). A systematic multi-step screening of numerous salt hydrates for low temperature thermochemical energy storage. Appl. Energy 124, 1–16. doi: 10.1016/j.apenergy.2014.02.053
Ousaleh, H. A., Sair, S., Zaki, A., Faik, A., Igartua, J. M., and El Bouari, A. (2020). Double hydrates salt as sustainable thermochemical energy storage materials: evaluation of dehydration behavior and structural phase transition reversibility. Solar Energy 201, 846–856. doi: 10.1016/j.solener.2020.03.067
Permyakova, A. (2016). Metal Organic Frameworks Based Materials for Long Term Solar Energy Storage Application. Gif-sur-Yvette: University of Paris-Saclay.
Permyakova, A., Wang, S., Courbon, E., Nouar, F., Heymans, N., D’Ans, P., et al. (2017). Design of salt–metal organic framework composites for seasonal heat storage applications. J. Mater. Chem. A 5, 12889–12898. doi: 10.1039/c7ta03069j
Ponomarenko, I. V., Glaznev, I. S., Gubar, A. V., Aristov, Y. I., and Kirik, S. D. (2010). Synthesis and water sorption properties of a new composite “CaCl2 confined into SBA-15 pores”. Micropor. Mesopor. Mater. 129, 243–250. doi: 10.1016/j.micromeso.2009.09.023
Pons, M., and Grenier, P. (1987). Experimental data on a solar-powered ice maker using activated carbon and methanol adsorption pair. J. Solar Energy Eng. 109:303. doi: 10.1115/1.3268222
Qasem, N. A. A., Qadir, N. U., Ben-Mansour, R., and Said, S. A. M. (2017). Synthesis, characterization, and CO 2 breakthrough adsorption of a novel MWCNT/MIL-101(Cr) composite. J. Co2 Util. 22, 238–249. doi: 10.1016/j.jcou.2017.10.015
Simonova, I. A., and Aristov, Y. I. (2005). Sorption properties of calcium nitrate dispersed in silica gel: the effect of pore size. Russ. J. Phys. Chem 79, 1307–1311.
Sonnauer, A., Hoffmann, F., Fröba, M., Kienle, L., Duppel, V., Thommes, M., et al. (2009). Giant pores in a chromium 2,6-Naphthalenedicarboxylate open-framework structure with MIL-101 topology. Angew. Chem. 48, 3791–3794. doi: 10.1002/anie.200805980
Stock, N., and Biswas, S. (2012b). Synthesis of metal-organic frameworks (MOFs): routes to various MOF topologies, morphologies, and composites. Cheminform 43, 933–969. doi: 10.1021/cr200304e
Stock, N., and Biswas, S. (2012a). ChemInform abstract: synthesis of metal–organic frameworks (MOFs): routes to various MOF topologies, morphologies, and composites. Chem. Rev. 112, 933–969.
Tan, B., Luo, Y., Liang, X., Wang, S., Gao, X., Zhang, Z. Z., et al. (2019). Mixed-solvothermal synthesis of MIL-101(Cr) and its water adsorption/desorption performance. Ind. Eng. Chem. Res. 58, 2983–2990. doi: 10.1021/acs.iecr.8b05243
Valencia, D. P., and González, F. J. (2011). Understanding the linear correlation between diffusion coefficient and molecular weight. A model to estimate diffusion coefficients in acetonitrile solutions. Electrochem. Commun. 13, 129–132. doi: 10.1016/j.elecom.2010.11.032
Yu, C., Han, J., Wang, T., and Mu, T. (2011). Determination of absorption rate and capacity of CO2in ionic liquids at atmospheric pressure by thermogravimetric analysis. Energy Fuels 25, 5810–5815. doi: 10.1021/ef201519g
Yu, N., Wang, R. Z., Lu, Z. S., and Wang, L. W. (2014). Development and characterization of silica gel–LiCl composite sorbents for thermal energy storage. Chem. Eng. Sci. 111, 73–84. doi: 10.1016/j.ces.2014.02.012
Zhang, K. D., Tsai, F. C., Ma, N., Xia, Y., Liu, H. L., Zhan, X. Q., et al. (2017). Adsorption behavior of high stable Zr-based MOFs for the removal of acid organic dye from water. Materials 10:205. doi: 10.3390/ma10020205
Keywords: material synthesis, MOFs, water adsorption, adsorption-desorption cycle, thermochemical energy storage
Citation: Lou L, Xu M, Huai X, Huang C and Liu Z (2021) Composite MWCNT/ MIL-101 (Cr)/CaCl2 as High-Capacity Water Adsorbent for Long-Term Thermal Energy Storage. Front. Mater. 8:653933. doi: 10.3389/fmats.2021.653933
Received: 18 January 2021; Accepted: 19 March 2021;
Published: 12 April 2021.
Edited by:
Peter Reiss, Commissariat à l’Energie Atomique et aux Energies Alternatives, FranceReviewed by:
Alessio Sapienza, Istituto di Tecnologie Avanzate per l’Energia “Nicola Giordano”, ItalyJinliang Li, Jinan University, China
Copyright © 2021 Lou, Xu, Huai, Huang and Liu. This is an open-access article distributed under the terms of the Creative Commons Attribution License (CC BY). The use, distribution or reproduction in other forums is permitted, provided the original author(s) and the copyright owner(s) are credited and that the original publication in this journal is cited, in accordance with accepted academic practice. No use, distribution or reproduction is permitted which does not comply with these terms.
*Correspondence: Min Xu, xumin@iet.cn; Xiulan Huai, hxl@iet.cn