- 1School of Materials and Architectural Engineering, Guizhou Normal University, Guiyang, China
- 2Guizhou Provincial Water Conservancy Research Institute, Guiyang, China
The transformation law of autogenous volume deformation of MgO-admixed concrete with specimen size was revealed through continuous observation on standard-size (Φ200 mm × 500 mm), medium-size (Φ250 mm × 500 mm), and large-size specimens (Φ250 mm × 600 mm) over 6 years. Besides, the pore parameters of concrete core samples obtained from autogenous volume deformation specimens in the 1st, 3rd, and 6th years of age were investigated. The results show that the autogenous volume deformation of MgO-admixed concrete increases with the increase in the MgO content or age. The expansion rate of the concrete specimen decreases after the age of 360 days, and the autogenous volume expansion deformation of the specimen tends to be stable after about 2 years. When the size of the specimen changes, the autogenous volume deformation of MgO-admixed concrete decreases with the increase of specimen size. During the age of 2–6 years, the expansion of medium- and large-size specimens is reduced by 6–10 and 15–20%, respectively, compared with the standard-size specimens under the same MgO content. With the condition of an appropriate MgO content, regardless of the size of the specimen, the pore structure of the concrete becomes better and better with the growth of age, the concrete becomes denser and denser, and the expansion caused by MgO hydration will not cause damage to concrete structures.
Introduction
It is well known that cracks produced by concrete during service endanger the safety and durability of structures. To this end, scientists and technicians have carried out fruitful research on the causes of cracks, cracking mechanisms, anti-cracking measures, and evaluation of anti-cracking effect from the aspects of raw materials, mix proportion, structural design, construction methods, and service environment (Nagataki and Gomi, 1998; Wang et al., 2021a; Kabir and Hooton, 2020; Chen et al., 2011b; Li and Burrows, 2013; Qureshi et al., 2018; Qureshi et al., 2019; Wang et al., 2020b; Wang et al., 2021b). In particular, scientific and technical personnel have conducted a large number of pioneering creative studies on the performance and production of magnesium oxide expansion agent (MgO) used in cement mortar and concrete (Liu et al., 1991; Gao et al., 2008; Li et al., 2010; Cao et al., 2018b), the performance of cement-based materials containing MgO (Li 1999a; Chen et al., 2010; Lu et al., 2011; Sherir et al., 2016; Sherir et al., 2017; Chen et al., 2017a; Liu et al., 2019; Wang et al., 2020a; Cao and Yan, 2019), and the expansion mechanism of MgO mortar and concrete (Mehta and Pirtz, 1980; Chatterji 1995; Deng et al., 1990; Cao et al., 2018b). The results show that MgO concrete, which is formed by mixing MgO expansion agent with concrete, can produce excellent delayed micro-expansion deformation. This expansion deformation can compensate for volume shrinkage of the large volume dam during the long temperature drop process. MgO has already been employed in cement clinker or as an expansive additive, particularly in dam concrete, with almost 40 years’ experience in both research activities and industrial applications in China (Mo et al., 2014). In China, it has been almost 40 years since MgO was employed in cement clinker or used as an expansive additive, particularly in dam concrete. So far, MgO-admixed concrete has been used in more than 50 small- and medium-sized hydraulic and hydroelectric engineering projects in China to simplify the temperature control measures for dam concrete, accelerate the construction schedule, and save the project investment (Li and Yuan, 2003; Chen et al., 2011a; Yuan et al., 2012; Mo et al., 2014; Zhao 2014; Chen et al., 2017b). However, only a few experimental studies about MgO-admixed mortar and concrete in the laboratory have been done in other countries (Mehta and Pirtz, 1980; Chatterji 1995; Ali and Mullick, 1998; Vandeperre et al., 2008; Seul-Woo et al., 2014; Sherir et al., 2016; Sherir et al., 2017; Sherir et al., 2017; Gonçalves et al., 2019; Khalil and Celik, 2019; Nguyen et al., 2019).
Before the MgO concrete is applied, an important task is to test the volume deformation of the concrete caused only by the hydration of cementitious materials under constant temperature and isolated humidity, that is, the autogenous volume deformation. This indicator is the essential basic information for the temperature control design, creep stress calculation, and simulation design of MgO-admixed concrete dams. For MgO-admixed concrete, due to its delayed expansion characteristics, engineers and technicians pay much more attention to the stability of its long-term autogenous volume expansion deformation because the instability of long-term autogenous volume deformation will endanger the safety of dam operation. However, there are many factors affecting the autogenous volume deformation of the MgO concrete. A comprehensive study must be carried out to accurately design the dam structure. (Li, 1999b) studied the effect of temperature on the autogenous volume deformation of MgO-admixed concrete with an age of 1,500 days. (Chen et al., 2015a) analyzed the effect of MgO expansion agent types, dosages, and cement types on the autogenous volume deformation of MgO-admixed concrete with an age of 1,400 days. (Chen et al., 2015b; Chen et al., 2018) investigated the influence of the test specimen’s forming method on the autogenous volume deformation of MgO-admixed concrete with an age of 1,400 days. In addition, they studied the correlation between the autogenous volume deformation of the MgO concrete with an age of 2,190 days and the autoclaved expansion of cement-based materials. Zhou et al. (2014) investigated the effect of the fly ash content, water–binder ratio, and MgO content on the autogenous volume deformation of MgO-admixed concrete with an age of 1,200 days. (Yan and Chen, 2016) studied the effect of fly ash on the autogenous volume deformation of MgO-admixed concrete with an age of 1,500 days. These research results have played an important role in both analyzing the influencing factors for the autogenous volume deformation of long-aged MgO concrete and promoting the popularization of MgO-admixed concrete. However, in the study of concrete autogenous volume deformation, the concrete specimen’s size was the standard size specified by the Chinese Water Conservancy Industry Standard test code for hydraulic concrete SL352-2006Chinese Water Conservancy Industry Standard, 2006, that is, a cylinder with a diameter of 200 mm and a height of 500 mm. It is worth noting that it is necessary to screen out the large aggregate of more than 40 mm in the concrete mixture beforehand, which is obviously not consistent with the actual situation of concrete. Engineers hope to use undisturbed concrete mixtures to test the autogenous volume deformation of concrete under the condition of appropriately increasing the size of specimens, but this still needs a lot of supporting research results. However, except for (Yan and Chen, 2015), who have studied the influence of specimen size on the autogenous volume deformation of MgO-admixed concrete with an age of 365 days, there are few studies about the influence of specimen size on the autogenous volume deformation of MgO-admixed concrete with a long age, based on the delayed micro-expansion characteristics of MgO concrete, which shows that the size of specimens with self-generated volume deformation of concrete is not allowed to be increased rashly. In this study, autogenous volume deformation specimens of concrete with different sizes were molded, and their autogenous volume deformation was continuously observed for more than 6 years. In addition, the micropore structure of these long-aged specimens was investigated. The results can be used to explore the size effect of the autogenous volume deformation of long-aged MgO concrete and provide basic information for the amendment of experimental method on autogenous volume deformation of concrete in future.
Materials and Methods
Experimental Materials and mix Proportion
The cement used in the test was PO 42.5 ordinary Portland cement, with 3.05 g/cm3 density, 311 m2/kg specific surface area, and 26.3% water requirement of standard consistency. The fly ash used was grade II according to the Chinese Standard, whose water demand ratio was 91.2%, fineness was 16.55% (0.045 mm sieve residue), and density was 2.46 g/cm3. MgO used was specialty for dam concrete on water resources and hydropower engineering whose fineness was 200 mesh and activity index was 215 s. Aggregates were machine-made limestone blocks or particles, whose grain size 5–20 mm is small stone, 20–40 mm is medium stone, and 40 ∼ 80 mm is large stone. The sand used was medium sand, and its fineness modulus was 2.98. The chemical component of cement, fly ash, and MgO is shown in Table 1.
The design strength grade of concrete is C9020 (i.e., the compressive strength of concrete reaches 20 MPa or 2900PSI at the age of 90 days). The fundamental concrete design adopted for specimens is shown in Table 2. In addition, the content of MgO was calculated according to the percentage of the total amount of cement and fly ash, taking 0, 6, 8, and 10%, respectively. The slumps of the fresh concrete confined between 30–50 mm.
Specimen Preparation
Three sizes of specimens, namely, the standard-size cylinder specimen (Φ200 mm × 500 mm) (standard-size specimen for short) specified by SL352-2006, medium-size specimen (Φ250 mm × 500 mm) (medium-size specimen for short), and large-size specimen (Φ250 mm × 600 mm) (large-size specimen for short), were used to observe the autogenous volume deformation of concrete. In this study, a total of 11 groups of specimens were prepared. The standard-, medium-, and large-size test specimens are represented by S, M, and L, respectively, and the number behind S, M, and L represents the MgO content in concrete. The MgO content used in this study was 0, 6, 8, and 10%.
The standard autogenous volume deformation specimen was molded completely in accordance with SL352–2006. First, the aggregates with the particle size over 40 mm in the concrete mixture were removed by wet sieving, and then the mixtures were put into the test barrel with a standard size. At the same time, the strain gauge was vertically fixed in the center of the specimen. Then, the specimen was compacted by vibrating, and the test barrel was sealed. Finally, the specimens were cured under a constant temperature of (20 ± 2)°C and isolated humidity, as shown in Figure 1. Obviously, the mix proportion of the standard-size specimen of concrete autogenous volume deformation was no longer the original mix proportion of concrete. In order to avoid the situation that the concrete specimen does not coincide with the actual project, the aggregates with particle size over 40 mm were not taken out when preparing the medium-size and large-size specimens, but the preparation process was the same as that of the standard-size specimens. In order to observe the hydration products of MgO and fine cracks around them, we performed energy spectrum analysis. The samples used for energy spectrum analysis were first drilled from the autogenous volume deformation specimens of concrete mixed with 10% MgO at the age of 6th year, and then ground into smooth and flat samples with a diameter of 15 mm and a thickness of 3 mm.
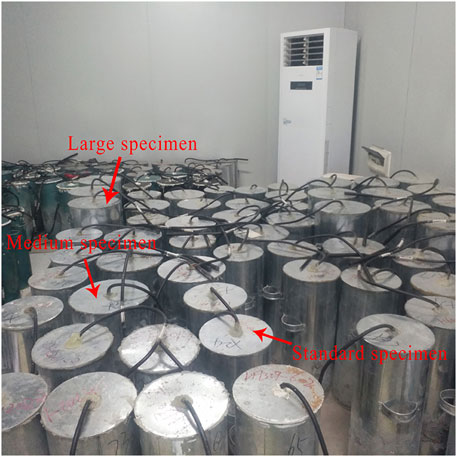
FIGURE 1. Concrete autogenous volume deformation specimens placed in the lab with constant temperature and isolated humidity.
The samples used to determine the pore parameters of the concrete were drilled from autogenous volume deformation specimens of concrete in the 1st, 3rd, and 6th year of age. The diameter and thickness of the core sample were 20 and 8 mm, respectively.
Test
There were three aspects of the test. First, the autogenous volume deformation of concrete was tested in accordance with the provisions of SL352–2006. Hydraulic proportional bridge was used in this test. Second, the hydration products of MgO and whether there are fine cracks around them were observed. An Oxford INCA-350 X-ray energy spectrometer was used in this test. Third, the pore parameters of concrete core samples were tested, including pore size distribution, average pore diameter, and porosity. An AutoPore IV 9520 mercury intrusion porosimeter produced by American Mike Instruments Company was used in this test. This mercury intrusion porosimeter has a maximum pressure of 60,000 PSI and a measured aperture range of 3.2 nm–360 μm. Before the mercury intrusion test, the samples were dried at 50°C for 24 h. This mercury intrusion test was conducted in accordance with the provisions of Chinese (National standards of the People's Republic of China, 2008) pore size distribution and porosity of solid materials by mercury porosimetry and gas adsorption—Part: Mercury porosimetry (ISO 15901-1:2005, IDT).
Results
The autogenous volume deformation curves obtained in the lab over 6 years are shown in Figures 2–5. The autogenous volume deformation values of various specimens at typical ages and the deformation values of medium- and large-size specimens mixed with 6 and 10% MgO relative to the standard-size specimens are shown in Table 3. At the age of the 6th year, the energy spectrum analysis diagrams of concrete core samples mixed with 10% MgO are shown in Figures 6–8. In addition, the results of porosity parameters of core samples of MgO concrete at typical ages are shown in Table 4; Figures 9–14.
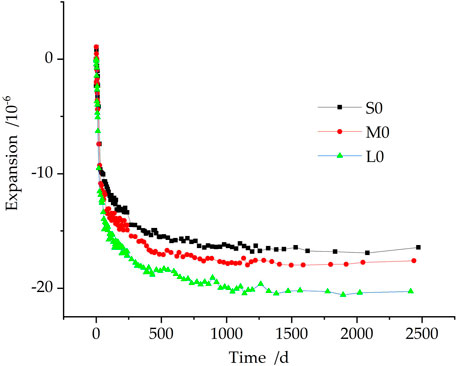
FIGURE 2. Autogenous volume deformation of long-aged concrete without the MgO content at different specimen sizes.
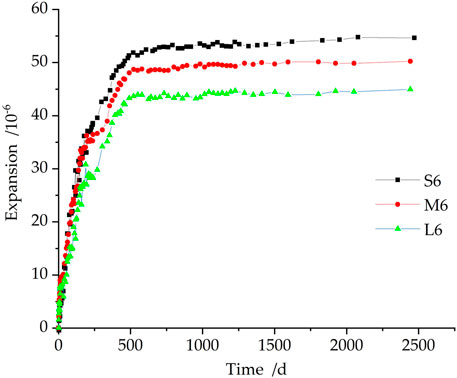
FIGURE 3. Autogenous volume deformation of long-aged concrete mixed with 6% MgO at different specimen sizes.
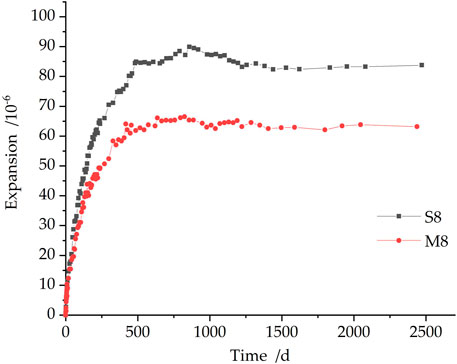
FIGURE 4. Autogenous volume deformation of long-aged concrete mixed with 8% MgO at different specimen sizes.
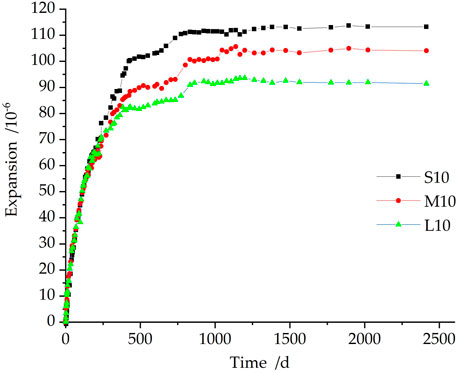
FIGURE 5. Autogenous volume deformation of long-aged concrete mixed with 10% MgO at different specimen sizes.
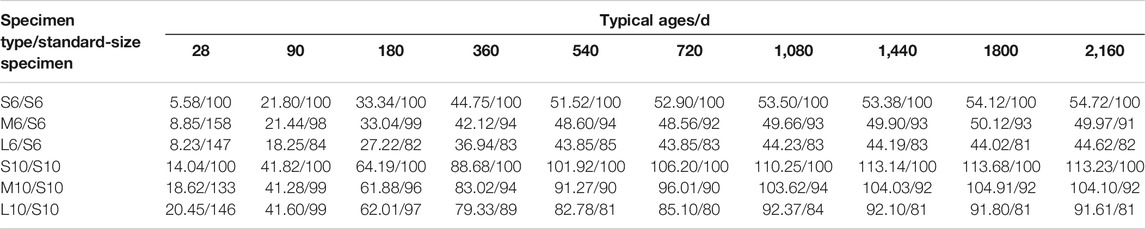
TABLE 3. The autogenous volume deformation values of various specimens at typical ages and the deformation values of medium- and large-size specimens relative to the standard-size specimens/(× 10−6/%).
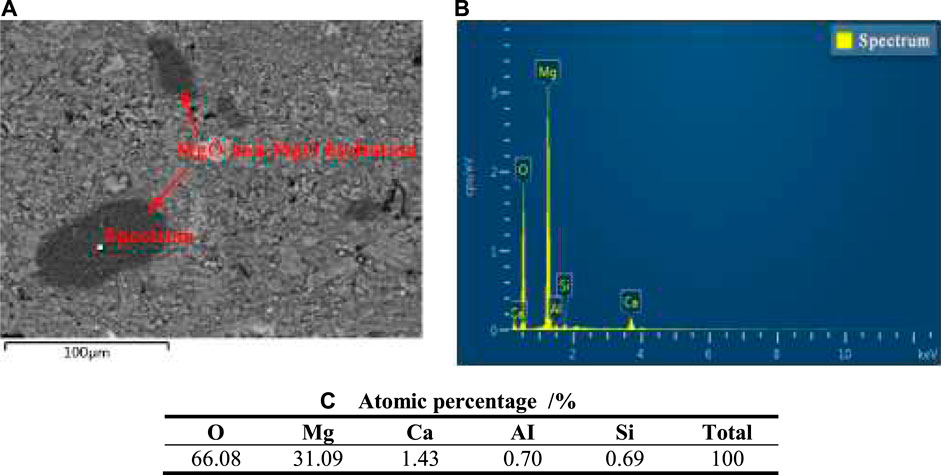
FIGURE 6. Energy spectrum analysis diagram of a standard-size concrete specimen mixed with 10% MgO. (A) Backscattered electron image. (B) Micro-area composition of energy spectrum. (C) Atomic percentage.
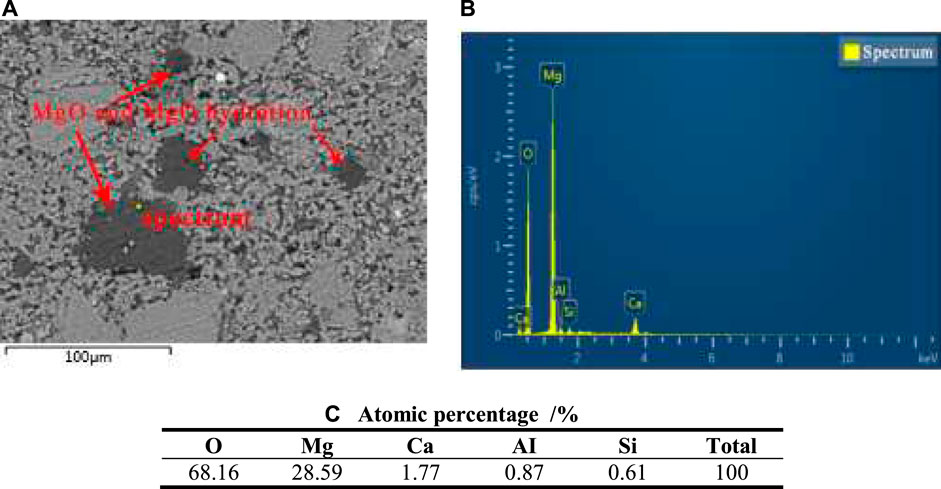
FIGURE 7. Energy spectrum analysis diagram of a medium-size concrete specimen mixed with 10% MgO. (A) Backscattered electron image. (B) Micro-area composition of energy spectrum. (C) Atomic percentage.
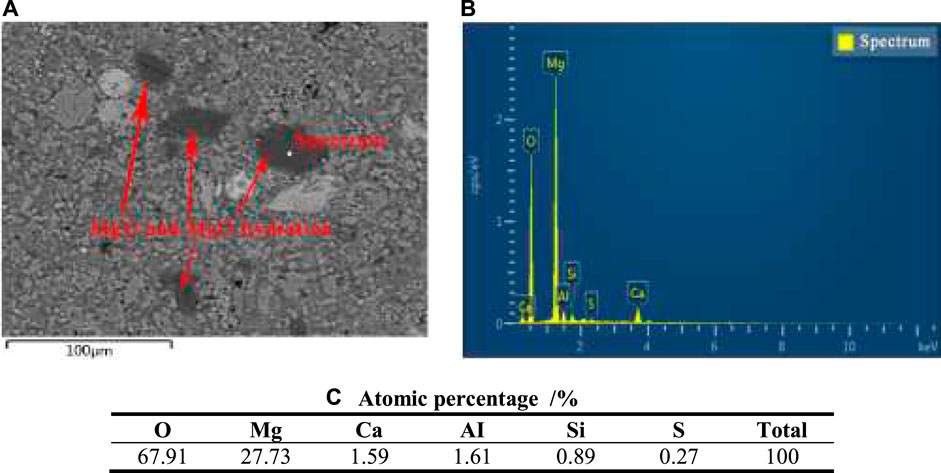
FIGURE 8. Energy spectrum analysis diagram of a large-size concrete specimen mixed with 10% MgO. (A) Backscattered electron image. (B) Micro-area composition of energy spectrum. (C) Atomic percentage.
Discussion
According to the above test results, the analysis is as follows.
(1) The autogenous volume deformation of the MgO-admixed concrete increases with the increase of the MgO content or the age.
As demonstrated in Figure 2, no matter what size the specimens are, autogenous volume deformation of the concrete without the MgO content is all at shrinkage state. Within the age of 180 days, the shrinkage rate of the specimens is high, and all three kinds of specimens show a sharp shrinkage. At the age of 180 days, the shrinkage values of the large-, medium-, and standard-size specimens are −16 × 10−6, −14 × 10−6, and −12.5 × 10−6, respectively. After the age of 180 days, the shrinkage rate of specimens decreases rapidly. After the age of 1,000 days, the autogenous volume shrinkage of the concrete tends to be stable. In general, the shrinkage value of the standard-size specimen is smaller than those of the medium- and large-size specimens. However, the shrinkage value of the standard-size specimen is only about 3.5 × 10−6 smaller than that of the large specimen. It can be seen from Table 4 that, for the concrete without MgO, the porosity at the age of 6th year can be ranked from high to low as follows: large-size specimen (9.07%), medium-size specimen (7.42%), and standard-size specimen (4.53%). This indicates that when the porosity inside the concrete is higher, the autogenous volume shrinkage is more significant.
However, from Figures 3Figures 4Figures 5, for the concrete with 6–10% MgO, the autogenous volume deformation of the standard-, medium-, and large-size specimens is all in an expansive state. In addition, the autogenous volume expansion increases with the increase of the MgO content or age. Within the age of 180 days, the expansion rate of test specimens is faster, and all three kinds of specimens show a rapid expansion. For example, at the age of 180 days, for the concrete specimens with 6% MgO, the expansion values of the large-, medium-, and standard-size specimens are 27 × 10−6, 32.5 × 10−6, and 36 × 10−6, respectively. When 10% MgO was added into the concrete, the expansion values increased to 58 × 10−6, 62 × 10−6, and 78 × 10−6, respectively. After the age of 360 days, the expansion rate of the concrete specimens slows down. After about 2 years, the autogenous volume expansion of the specimens tends to be stable. At the age of the 6th year, the expansion values of large-, medium-, and standard-size specimens of the concrete specimens with 10% MgO are 91.7 × 10−6, 104.2 × 10−6, and 113.2 × 10−6, respectively, and are 33.7 × 10−6, 42.2 × 10−6, and 35.2 × 10−6 more than those at 180 days of age. The expansion rate is about 6.1 × 10−6–7.7 × 10−6/a. (Deng et al., 1990) pointed out that the volume expansion of MgO-admixed concrete originates from the water-absorption swelling force and crystal growth pressure in the hydration process of MgO to Mg(OH)2. At the early stage of hydration, the crystal of Mg(OH)2 is very small, and the expansion of slurry is mainly caused by water-absorption swelling force. With the growth of Mg(OH)2 crystal, the crystal growth pressure becomes the main driving force of expansion. Therefore, in the early hydration stage, due to the obvious water-absorption swelling force, the expansion rate is high. At the later stage of hydration, the expansion rate is low because of the slow growth of Mg(OH)2 crystal. (Cao et al., 2018a) pointed out that the expansion caused by MgO expansive agents is due to the growth of brucite crystals mainly on the wall of inferior pores in the confined zone and slightly on the surface of the MgO particles. The growth of brucite crystal is controlled by the supersaturation of Mg2+ in the pore solution. The growth of brucite crystals expands the gaps inside the matrix, thus causing the expansion of the paste. These findings indicate that crystal growth is the main driving force for the expansion of MgO-containing slurry. When the MgO content in the concrete increases or the age of the MgO concrete is longer, more Mg(OH)2 crystals are generated by the hydration of MgO, and the corresponding crystal growth pressure will increase, resulting in more volume expansion of the concrete.
At the same time, as shown in the energy spectrum diagram in Figures 6–8, the quantity of Mg and O is significantly higher than that of Si, Al, and Ca, which indicates that the observation point selected in the sample is indeed the location of MgO and its hydration products. For the concrete specimens with the MgO content up to 10%, until the 6th year of age, the atom number ratio (Mg: O) was close to 1:2, higher than that in MgO (1:1), and very close to that in Mg(OH)2 (1:2), no matter the standard-, medium-, or large-size specimens, which indicates that MgO in concrete had been basically hydrated to Mg(OH)2 after 6 years. However, no radioactive micro-cracks caused by MgO hydration expansion are observed around the concrete particles containing MgO hydration products. This indicates that under the condition of the appropriate MgO content, expansion caused by MgO hydration will not cause damage to concrete structures.
(2) Autogenous volume deformation of MgO-admixed concrete decreases with the increase of specimen size.
It can be seen from Figures 3; Table 3 that, with the same MgO content, the autogenous volume expansion of MgO concrete is from high to low as follows: standard-, medium-, and large-size specimens. In other words, the autogenous volume expansion of MgO concrete decreases with the increase of specimen size. This trend becomes obvious especially after the age of 180 days. In addition, during the age of 2–6 years, the expansion of the medium-size specimen is reduced by 6–10% compared with that of the standard-size specimen, and the expansion of the large-size specimen is reduced by 15–20% compared with that of the standard-size specimen under the same MgO content. This phenomenon should be related to the difference of pore structure among standard-, medium-, and large-size specimens.
As shown in Table 4; Figures 9–12, after 6 ∼ 10% MgO was added into the standard-, medium-, or large-size specimens, the volume percent of harmless pores with the diameter smaller than 20 nm (Wu and Lian, 1999) increases obviously with age. Meanwhile, the average pore diameter and porosity of the concrete core reduce with age. Therefore, after an appropriate amount of MgO is added into the concrete, the pore structure of the concrete becomes finer with age, and the density of the concrete gets higher. At the same time, it can be seen from Table 4; Figures 13, 14 that the porosity of medium- and large-size specimens is generally close in the 1st, 3rd, and 6th year of age, and both obviously higher than that of standard-size specimen. When the standard-size specimens were prepared in the laboratory, the coarse aggregates with the particle size over 40 mm were removed. However, in order to be consistent with the actual engineering concrete, the coarse aggregates with the particle size over 40 mm were not removed when the large- and medium-size specimens were prepared. Under the same vibrating effect, concrete with a relatively large amount of coarse aggregate is not easy to be compacted and has relatively poor compactness, so their porosity is higher than that of the standard-size specimens without coarse aggregates. Compared with the concrete with lower porosity, concretes with large porosity have a smaller elastic modulus, and these pores can easily be squeezed by the hydration expansion generated by MgO particles. In particular, the hydration expansion generated by the MgO particles close to the internal pores can also grow into the pores, which are equivalent to the absorbing part of the MgO particles to cause hydration expansion, and eventually lead to the reduction of the effective expansion amount. Because the concrete with larger internal porosity can absorb more expansion caused by MgO, the standard-size specimen has larger expansion deformation than the large- and medium-size specimens.
Engineering practice also shows that the measured value of autogenous volume deformation of standard-size specimens is higher than that of engineering solid concrete (Li and Yuan, 2003; Chen et al., 2011b; Yuan et al., 2012; Zhao 2014; Chen et al., 2017a), which is consistent with the trend that the measured value of standard-size specimens is higher than those of large- and medium-size specimens. Therefore, when testing the autogenous volume deformation value of concrete indoors and revising the test standard for autogenous volume deformation of concrete in the future, it is suggested to appropriately increase the size of the specimen to reflect the engineering practice as much as possible.
Conclusion
Through continuous observation on standard-size (Φ200 mm × 500 mm), medium-size (Φ250 mm × 500 mm), and large-size specimens (Φ250 mm × 600 mm) over 6 years, and observing pore size distribution, average pore diameter, and porosity of concrete core samples by drilling from autogenous volume deformation specimens in the 1st, 3rd, and 6th year of age, the main findings are as follows.
(1) The autogenous volume deformation of MgO-admixed concrete increases with the increase of the MgO content or the age, no matter how the specimen size is. After the ages of 360 days, the expansion rate of the concrete specimen slows down. After about 2 years, the autogenous volume expansion of the specimen tends to be stable.
(2) When the size of the specimen changes, the autogenous volume deformation of MgO-admixed concrete decreases with the increase of specimen size. During the age of 2–6 years, the expansion amount of medium- and large-size specimens is reduced by 6–10 and 15–20% sequentially compared with that of the standard-size specimens under the same MgO content.
(3) Regardless of the size of the specimen, under the condition of proper MgO content, the pore structure of the concrete becomes better with the growth of age, the density of the concrete gets higher, and expansion generated by MgO hydration will not cause damage to concrete structures.
Data Availability Statement
The raw data supporting the conclusions of this article will be made available by the authors, without undue reservation.
Author Contributions
CC designed the experiments and wrote the manuscript. CC, RC, ZZ, WL, SY, and HY performed the experiments and contributed to the discussion part. RC processed data and made charts. XD and HY did the review and editing, and contributed to the discussion part. All authors contributed to the article and approved the submitted version.
Funding
This research is supported by the National Natural Science Foundation of China (grant nos. 51869005 and 51269003).
Conflict of Interest
The authors declare that the research was conducted in the absence of any commercial or financial relationships that could be construed as a potential conflict of interest.
References
Ali, M. M., and Mullick, A. K. (1998). Stabilisation of high MgO cement: effect of curing conditions and fly ash addition. Cem. Concr. Res. 28, 1585–1594. doi:10.1016/s0008-8846(98)00140-9
Cao, F., Miao, M., and Yan, P. (2018a). Effects of reactivity of MgO expansive agent on its performance in cement-based materials and an improvement of the evaluating method of MEA reactivity. Constr. Build. Mater. 187, 257–266. doi:10.1016/j.conbuildmat.2018.07.198
Cao, F., Miao, M., and Yan, P. (2018b). Hydration characteristics and expansive mechanism of MgO expansive agents. Constr. Build. Mater. 183 (2), 234–242. doi:10.1016/j.conbuildmat.2018.06.164
Cao, F., and Yan, P. (2019). The influence of the hydration procedure of MgO expansive agent on the expansive behavior of shrinkage-compensating mortar. Constr. Build. Mater. 202, 162–168. doi:10.1016/j.conbuildmat.2019.01.016
Chatterji, S. (1995). Mechanism of expansion of concrete due to the presence of dead-burnt CaO and MgO. Cem. Concr. Res. 25, 51–56. doi:10.1016/0008-8846(94)00111-b
Chen, X., Yang, H. Q., Zhou, S. H., and Yan, J. J. (2010). Crack resistance of concrete with incorporation of light-burnt MgO. Adv. Mater. Res. 168–170, 1348–1352. doi:10.4028/www.scientific.net/amr.168-170.1348 |
Chen, C. L., Tang, C. L., and Zhao, Z. H. (2011a). Application of MgO concrete in China Dongfeng arch dam foundation. Adv. Mater. Res. 168–170, 1953–1956. doi:10.4028/www.scientific.net/AMR.168-170.1953 |
Chen, X., Yang, H., Zhou, S., and Li, W. (2011b). Sensitive evaluation on early cracking tendency of concrete with inclusion of light-burnt MgO. J. Wuhan Univ. Technol. Mater. Sci. Ed. 26, 1018–1022. [in Chinese, with English summary]. doi:10.1007/s11595-011-0354-6
Chen, C. L., Zhao, Z. H., Li, W. W., and Li, L. C. (2015a). Analysis of autogenic deformation of MgO concrete at long age. Hydro-Sci. Eng. 54–59. [in Chinese, with English summary].
Chen, X., Yang, H. Q., Zhang, J. F., and Yan, J. J. (2015b). Analysis of influential factors on autogenous deformation of MgO concrete. Yangtze River 46, 70–73. [in Chinese, with English summary].
Chen, C., Li, W., Yang, D., Zhao, Z., and Yang, H. (2017a). Study on autoclave expansion deformation of MgO-admixed cement-based materials. Emerg. Mater. Res. 6, 422–428. doi:10.1680/jemmr.16.00090
Chen, C. L., Shen, X. P., and Chen, X. M. (2017b). Application of damming technology with MgO admixed concrete used in the whole dam in Guizhou’s arch dam. Adv. Sci. Technol. Water Resour. 37, 84–88. [in Chinese, with English summary]. doi:10.3880/j.issn.1006-7647.2017.05.015
Chen, C. L., Chen, R. F., Yan, S. L., Lei, P., and Li, W. W. (2018). Relationship between autogenous deformation of long-age MgO-mixed concrete and autoclave-treated expansive deformation of cement-based materials. Adv. Sci. Technol. Water Resour. 38, 90–94. [in Chinese, with English summary]. doi:10.3880/j.issn.1006-7647.2018.02.015
Chinese Water Conservancy Industry Standard (2006). Chinese water conservancy industry standard test code for hydraulic concrete SL352-2006. Beijing, China: China Water Resources and Hydropower Press, 12 [in Chinese, with English summary].
Deng, M., Cui, X. H., Liu, Y. Z., and Tang, M. S. (1990). Expansive mechanism of magnesia as an additive of cement. J. Nanjing Univ. Chem. Technol. 12, 1–11. [in Chinese, with English summary].
Gao, P., Lu, X., Geng, F., Li, X., Hou, J., Lin, H., et al. (2008). Production of MgO-type expansive agent in dam concrete by use of industrial by-products. Build. Environ. 43 (4), 453–457. doi:10.1016/j.buildenv.2007.01.037
Gonçalves, T., Silva, R. V., de Brito, J., Fernández, J. M., and Esquinas, A. R. (2019). Hydration of reactive MgO as partial cement replacement and its influence on the macroperformance of cementitious mortars. Adv. Mater. Sci. Eng. 2019, 1–12. doi:10.1155/2019/9271507
Kabir, H., and Hooton, R. D. (2020). Evaluating soundness of concrete containing shrinkage-compensating MgO admixtures. Constr. Build. Mater. 253, 1–11. doi:10.1016/j.conbuildmat.2020.119141
Khalil, A., and Celik, K. (2019). Optimizing reactivity of light-burned magnesia through mechanical milling. Ceram. Int. 45 (17), 22821–22828. doi:10.1016/j.ceramint.2019.07.324
Li, C. M. (1999a). An experimental study of the autogenous deformation of the MgO concrete. J. Hydroelectr. Eng. 11–20. [in Chinese, with English summary].
Li, C. M. (1999b). Temperature effect test of self deformation of MgO concrete and its application. Adv. Sci. Technol. Water Resour. 19, 33–37. [in Chinese, with English summary].
Li, C. M., and Yuan, M. D. (2003). Micro-expansion of technology of concrete dam mixed with MgO. Adv. Sci. Technol. Water Resour. 23, 57–63. [in Chinese, with English summary]. doi:10.1007/bf02829450
Li, F. X., Chen, Y. Z., Long, S. Z., Wang, B., and Li, G. G. (2010). Research on the preparation and properties of MgO expansive agent. Adv. Cement Res. 22 (1), 37–44. doi:10.1680/adcr.2008.22.1.37
Li, W. W., and Burrows, R. W. (2013). Observation and consideration of concrete cracking. Beijing, China: China Water Resources and Hydropower Press, 9 [in Chinese, with English summary].
Liu, P., Chen, Z., and Deng, M. (2019). Regulating the expansion characteristics of cementitious materials using blended MgO-type expansive agent. Materials 12 (6), 976. doi:10.3390/ma12060976
Liu, Z., Cui, X. H., and Tang, M. S. (1991). MgO-type delayed expansive cement. Cem. Concr. Res. 21 (6), 1049–1057. doi:10.1016/0008-8846(91)90065-p
Lu, X., Geng, F., Zhang, H., and Chen, X. (2011). Influence of MgO-type expansive agent hydration behaviors on expansive properties of concrete. J. Wuhan Univ. Technol.-Mat. Sci. Edit. 26, 344–346. doi:10.1007/s11595-011-0227-z
Mehta, P. K., and Pirtz, D. (1980). “Magnesium oxide additive for producing self-stress in mass concrete,” in proceedings of the 7th international congress on the chemistry of cement. Paris, France: Septima, 3, 6–9.
Mo, L., Deng, M., Tang, M., and Al-Tabbaa, A. (2014). MgO expansive cement and concrete in China: past, present and future. Cem. Concr. Res. 57, 1–12. doi:10.1016/j.cemconres.2013.12.007
Nagataki, S., and Gomi, H. (1998). Expansive admixtures. Cem. Concr. Compos. 21 (11), 163–170. doi:10.1016/s0958-9465(97)00064-4
National standards of the People's Republic of China (2008). Pore size distribution and porosity of solid materials by mercury porosimetry and gas adsorption-Part, Mercury porosimetry. 1st Edn. 18 [in Chinese, with English summary].
Nguyen, V. C., Tong, F. G., and Nguyen, V. N. (2019). Modeling of autogenous deformation process of RCC mixed with MgO based on concrete expansion experiment. Constr. Build. Mater. 210, 650–659. doi:10.1016/j.conbuildmat.2019.03.226
Qureshi, T., Kanellopoulos, A., and Al-Tabbaa, A. (2018). Autogenous self-healing of cement with expansive minerals-I: impact in early age crack healing. Constr. Build. Mater. 192, 768–784. doi:10.1016/j.conbuildmat.2018.10.143
Qureshi, T., Kanellopoulos, A., and Al-Tabbaa, A. (2019). Autogenous self-healing of cement with expansive minerals-II: impact of age and the role of optimised expansive minerals in healing performance. Constr. Build. Mater. 194, 266–275. doi:10.1016/j.conbuildmat.2018.11.027
Seul-Woo, C., Bong-Seok, J., Joo-Hyung, K., and Kwang-Myong, L. (2014). Durability characteristics of fly ash concrete containing lightly-burnt MgO. Constr. Build. Mater. 58, 77–84. doi:10.1016/j.conbuildmat.2014.01.080
Sherir, M. A. A., Hossain, K. M. A., and Lachemi, M. (2016). Self-healing and expansion characteristics of cementitious composites with high fly ash and MgO-type expansive agent. Constr. Build. Mater. 127, 80–92. doi:10.1016/j.conbuildmat.2016.09.125
Sherir, M. A. A., Hossain, K. M. A., and Lachemi, M. (2017). The influence of MgO-type expansive agent incorporated in self-healing system of engineered cementitious composites. Constr. Build. Mater. 149, 164–185. doi:10.1016/j.conbuildmat.2017.05.109
Vandeperre, L. J., Liska, M., and Al-Tabbaa, A. (2008). Hydration and mechanical properties of magnesia, pulverized fuel ash, and portland cement blends. J. Mater. Civ. Eng. 20, 375–383. doi:10.1061/(asce)0899-1561(2008)20:5(375)
Wang, L., He, T. S., Zhou, Y. X., Tang, S. W., Tan, J. J., Liu, Z. T., et al. (2021b). The influence of fiber type and length on the cracking resistance, durability and pore structure of face slab concrete. Constr. Build. Mater. 272, 121952. doi:10.1016/j.conbuildmat.2021.122706
Wang, L., Guo, F., Yang, H., Wang, Y., and Tang, S. (2020a). Comparison of fly ash, PVA fiber, MgO and shrinkage-reducing admixture on the frost resistance of face slab concrete via pore structural and fractal analysis. Fractals 29 (2), 2140002. doi:10.1142/S0218348X21400028
Wang, L., Jin, M., Guo, F., Wang, Y., and Tang, S. (2020b). Pore structural and fractal analysis of the influence of fly ash and silica fume on the mechanical property and abrasion resistance of concrete. Fractals 29 (2), 2140003. doi:10.1142/s0218348x2140003x
Wang, L., Jin, M. M., Wu, Y. H., Zhou, Y. X., and Tang, S. W. (2021a). Hydration, shrinkage, pore structure and fractal dimension of silica fume modified low heat Portland cement-based materials. Constr. Build. Mater. 272, 1–13. doi:10.1016/j.conbuildmat.2020.121952
Wu, Z. W., and Lian, H. Z. (1999). High performance concrete. Beijing, China: China Railway Publishing House, 9 [in Chinese, with English summary].
Yan, S. L., and Chen, C. L. (2016). Influence of fly ash on the long-term autogenetic deformation of concrete mixed with magnesium oxide. South-to-North Water Transf. Water Sci. Technol. 14, 153–157. [in Chinese, with English summary].
Yan, S. L., and Chen, C. L. (2015). Influence of specimen size on autogenous deformation of concrete with magnesium oxide. Mater. Sci. Eng. 46 (12), 100–104. doi:10.1088/1757-899X/103/1/012001
Yuan, M. D., Xiao, M., and Yang, G. H. (2012). Long-term prototype observation data analysis of autogenous deformation of MgO concrete for Changsha arch dam. J. Hydroelectr. Eng. 31, 168–174. [in Chinese, with English summary]. doi:10.1016/j.proeng.2012.01.1103
Zhao, Q. X. (2014). Review on design of MgO concrete arch dam for 10 years in Guizhou. Water Resour. Hydr. Eng. 45, 53, 2014. [in Chinese, with English summary]. doi:10.13928/j.cnki.wrahe.2014.02.017
Keywords: MgO-admixed concrete, autogenous volume deformation, size effect, pore parameters, long age
Citation: Chen C, Chen R, Zhao Z, Li W, Yan S, Du X and Yang H (2021) Influence of Specimen Size on Autogenous Volume Deformation of Long-Aged MgO-Admixed Concrete. Front. Mater. 8:639838. doi: 10.3389/fmats.2021.639838
Received: 10 December 2020; Accepted: 15 February 2021;
Published: 22 April 2021.
Edited by:
E Chen, Chalmers University of Technology, SwedenReviewed by:
Zhengwu Jiang, Tongji University, ChinaHuamei Yang, Wuchang University of Technology, China
Lei Wang, Xi’an University of Architecture and Technology, China
Copyright © 2021 Chen, Chen, Zhao, Li, Yan, Du and Yang. This is an open-access article distributed under the terms of the Creative Commons Attribution License (CC BY). The use, distribution or reproduction in other forums is permitted, provided the original author(s) and the copyright owner(s) are credited and that the original publication in this journal is cited, in accordance with accepted academic practice. No use, distribution or reproduction is permitted which does not comply with these terms.
*Correspondence: Changli Chen, gzgyccl@tom.com