- 1Department of Stomatology, The First Hospital of Jilin University, Changchun, China
- 2Department of Stomatology, China-Japan Union Hospital, Beijing, China
- 3Nanyang Medical College, Nanyang, China
Dental bonding materials are widely used in dentistry and there are several available kinds. However, in recent years, there has been no further research into dental bonding materials, with most dentists focusing on dental implants and orthodontics. In this paper, we systematically review the technology of tooth bonding in recent years and summarize the existing literature for potential innovation and direction of future research. First, the theoretical research on dental assembly materials and bonding mechanism was reviewed. Then, we reviewed the bonding of teeth, the bonding of metal alloy prosthesis, and the measurement of bond strength. Finally, the future development of dental bonding technology was evaluated. It is hoped that more dentists will be able to treat patients and update research on bonding materials in the field of teeth bonding to bring a new era to the restoration of teeth in the future.
Introduction
As we all know, when our teeth are broken by force or because of dental caries, we need to use bonding materials or filling materials. Over the years, a lot of changes have taken place in the dental materials. We aim to tell you how these materials have improved and restored teeth through such a review.
Adhesion is defined as the sticking of two surfaces to one another. Dental adhesion depends on the properties of components: assembly materials such as cement and adhesive, the tooth, and prosthesis. The mode of adhesion can be divided into two main categories: mechanical and chemical. Mechanical adhesion relies on mechanical interlocking which provides retention and a durable form of bonding. Chemical adhesion involves the modification of the surface etch, and two dissimilar surfaces are connected by an active monomer. This article will have a global review of dental assembly materials, mechanism of adhesion, adhesive bonding to teeth, and prosthesis.
Dental Assembly Materials
Cement
The definition of cement or adhesive is mainly defined by its curing methods. Cement includes acid base reaction curing materials. The most common forms are acidic liquids and alkaline powders. The liquid is composed of an aqueous solution of polyacrylic acidor a copolymer of acrylic acid and other unsaturated carboxylic acids. The powder is fluoroaluminosilicate glass. Their properties depend on the nature of liquid and powder.
Table 1 shows the classification of dental cements. Silicate cement is discarded due to its low mechanical properties, high cytotoxicity, and high solubility. Zinc polycarboxylate has the worst cohesion. Because of their poor mechanical properties, they are used less. The compressive strength of zinc orthophosphate is slightly lower than that of glass ionomer cement (Sofan et al., 2017).
In dental application, both glass ionomer cement (GIC) and zinc polycarboxylate cement provide direct adhesion to tooth and can provide fluoride release, but the latter is more radiopaque and biocompatible than GIC (Wetzel et al., 2020).
Polycarboxylate cement also sets according to an acid–base reaction. The powder is composed of mainly zinc oxide (ZnO), magnesium oxide (MgO), bismuth (Bi2O3) and aluminum oxide (Al2O3). It may also contain stannous fluoride (SnF2) where disappearance of fluoride improves the shear bond strength (SBS) of the orthodontic bracket (Khargekar et al., 2019).
Zinc oxide (ZnO) particles have been used for temporal or permanent luting cement because of their excellent mechanical strength and biocompatibility. ZnO nanoparticles have distinct optical and antibacterial properties and a high surface-to-volume ratio (Nguyen et al., 2019).
Typically, GICs are reacted by fluoride-containing silicate glass and polyalkenoic acids. Figure 1 shows the manufacturing method of GIC. Fluoride is one of ionic constituents that are released from the glass during the setting reaction. Polyacid-modified resin composites (compomers) claim to combine the mechanical and aesthetic properties of composites with the fluoride-releasing advantages of conventional GICs so the resultant material is a low-fluoride-releasing resin composite that contains vinyl groups that can be polymerized by visible light-activated initiators. Polyacrylic acid pretreatment significantly increases the micro-SBS (μSBS) of all cements (Paing et al., 2020). All these cements have relatively low cohesion and are relatively fragile. Although these weak properties limit the marking of conventional cement, they indicate that one of the main characteristics of cement is the ease of removal of excess cement (Shahid et al., 2010).
Adhesives
The adhesive is composed of powder and liquid and is mostly an organic polymer that comprises methacrylate matrix, wherein the matrix does or does contain mineral filler (Table 2).
The resin matrix is made of two methacrylic acid monomers. The most common ones are bis-GMA, UDMA, or methyl methacrylate (MMA). Ethylene glycol (TEGDMA) derivatives are usually added to reduce the high viscosity provided by bis-GMA. The filler includes colloidal particles of glass silicon such as SiO2, B2O3, Na2O3, Al2O3, which is obviously sub-micron size (particle size: 10–40 nm). They account for 30–65% of the adhesive volume. The volume ratio of filler to matrix has a major effect on adhesive properties. Therefore, the increase of this ratio will reduce the removal of polymerization and increase viscosity. To form a cohesive structure, a strong chemical bond must be formed between the matrix and the filler. Otherwise, each interface will be the preferred location for the initiation of cracks.
After the redox reaction, the polymerization initiator activates the curing phenomenon. The initiation always takes place after the redox reaction. The reaction produces free radicals that attack the double bond of the methacrylic group, which leads to polymerization. When the adhesive is chelating, it is usually triggered by a pair of amine peroxide. Figure 2 shows the progress of initiation of amine peroxide.
The adhesive is then packaged into two pastes, each paste containing one element and in contact with each other when mixed.
Mechanism of Adhesion
To choose the proper adhesive, it is important to understand the mechanism of adhesion and the surface energies of all the substrates, and how well the adhesive will wet out. Surface energy is a physical property of the surface of a material that determines whether an adhesive will make intimate contact and provide a bond. The adhesive interactions between an adhesive and a substrate not only concern the actual area of contact (adhesion zone) of the adhesive and substrate but also concern the state of the adhesive in the vicinity of the surface of the substrate (transition zone). Figure 3 shows the structure of the adhesive joint and effect of surface energy.
The Concept of Surface State, Surface, Surface Energy, and Adsorption
To better understand the mechanism of adhesion, it is necessary to redefine some concepts of surface state that include all the interactions that help to connect two main bodies. A good distinction must be made between the notion of cohesion, which includes all interactions that help to bind the two bodies, and adhesion, which corresponds to the force or energy of separation of an assembly.
The components (atoms, ions, molecules) between the surface and the internal do not have the same number. This result in an imbalance: the distance between atoms is greater than that of equilibrium; different levels of electronics; and an energetic state higher than that inside the body.
These characteristics explain the particular reactivity of the surface to its environment. Therefore, the surface energy must be defined, which is equivalent to the work required (W) to form a new area unit of the material (A).
where γ is represented by J/m2.
At the liquid/gas interface, the surface energy corresponds to surface tension. In accordance with the law of thermodynamics, the surface will naturally seek to reduce its energy levels. The components that make up the solid surface do not have the same freedom of movement as the liquid surface. These interface connections are matched with adsorption and reduce the energy of the surface by exchanging connections with the external environment.
In this case, physical adsorption includes weak bonds (2–42 kJ/mol) such as van der Waals or hydrogen bond; whereas, chemisorption includes strong bond by electronic transfers at the interfaces such as ionic and covalent bonds (100–1,000 kJ/mol).
All the chemical, structural, and topographic properties of the material surface are combined into the surface state. In practice, the surface is always full of defects and heterogeneity.
(1) Chemical heterogeneity is linked not only to the nature of different species that constitute the structure or material but also to the existence of adsorbed hetero atoms.
(2) Structural heterogeneity with some amorphous or crystalline characteristics and sequences of related defects.
(3) Morphological and topographical heterogeneity of substrates that have never been ideally smoothed can have a porous and absorbent appearance.
Adhesion and Wetting
When a material is deposited in the liquid phase on a substrate, it is spread and linked to the two materials to form bonds. Physical adhesion is only possible when the intermolecular distance is ≤7 Ǻ.
The spreading of liquid corresponds to wetting and depends on three interfacial energies:
(1) The surface energy of the substrate (γS)
(2) The surface energy in saturation of liquid vapor (γL)
(3) The solid-liquid interfacial energy which corresponds to the bonds (γSL)
Wetting for the liquid and wettability for the substrate are inversely proportional to γL and γS, respectively.
The adhesion, linked to γL and γS, is given by Dupré’s law (Figure 4):
By setting the equation of the quality of the forces at equilibrium, we find Young’s law:
where θ is the contact angle
The contact angle must be defined at equilibrium. This will be recorded by the contact angle according to time. The curve is hyperbolic and its limit gives us the value of θ.
Contrary to expectation, viscosity does not affect the wettability but only the spreading kinetics.
where v is the spreading speed, γL is the surface tension of the liquid, and η is the viscosity.
Liquid Surface Tension and/or Viscosity
It is obvious that a liquid adhesive will moisten the surface faster than a high-viscosity adhesive. The importance of this extension kinetics is relative, because the flow rate of most adhesive resins on an etched enamel is several centimeters per second. Therefore, the viscosity only affects a very fast kinetics.
Therefore, for two liquids of the same nature but different viscosity applied on the same surface and when t → 0, the contact angle of the most viscous contact angle is greater than that of the other, but when t → ∞, the angle is the same.
The roughness has a positive influence on interlocking when contact angle (θ) is <90° and has a negative influence when θ > 90° (Figure 5).
However, since all the real surfaces are not ideal, the Wenzel model was developed to describe the contact angles on the real surface where the new angle provides a roughness index (ρ) which corresponds to the real contact provided by the rough topography—Wenzel equation:
Where θ′ is the apparent contact angle and θ is the equilibrium contact angle on an ideal solid without roughness.
The capillary rise represents a special case of adhesive spreading, which is affected by capillary pressure.
where γL is the surface tension of the adhesive and r is the capillary radius.
This characteristic is largely responsible for the quality of the resin/etched enamel interface, particularly for a transverse section of prisms.
Adhesion Theory
There is no single theory to explain adhesion, but it is commonly divided into mechanical interlocking, physical bonding, and chemical bonding (Figure 6).
Mechanical Interlocking
Mechanical interlocking happens when two dissimilar phases attach to one another by mechanical force only. This typically requires a rough surface where the other material can penetrate.
Physical Bonding
Physical adhesion means a very small interaction distance. The liquid expansion on the substrate becomes a basic component. This theory is associated with the micro or macro bond of mechanical anchors through low link (hydrophilic or hydrophobic) physical adsorption. When the liquid is wetted, the interaction may be considered a response to the theory of physical adhesion.
Biomaterial surface properties including chemical, topographic, and wettability regulate the cell response. Control of inflammation will inform the design of surface modification procedures to direct the immune response and enhance the success of implanted materials (Hotchkiss et al., 2016; Qiu et al., 2020; Sang et al., 2020).
Intern-Diffusion Theory
The model is illustrated by polymer-polymer adhesion. The diffusion of one polymer into another polymer increased the degree of adhesion. Brown emphasized this interaction, which is considered to be the “Velcro” of molecules. There are two basic criteria for this diffusion:
(1) Thermodynamic criteria: molecules must be compatible in terms of hydrophobicity and hydrophobicity (van der Spoel et al., 2006).
(2) Dynamic criteria: macromolecules must have sufficient mobility. In addition, the molecule diameter and the distance of intermolecular space must be compatible with internal diffusion. Even if the thermodynamic criteria are valid, a 200 Ǻ polymer will not diffuse the membrane protein with intermolecular spaces of 150 Ǻ (Ando and Skolnick, 2010).
Therefore, the importance of diffusion medium and solvent and the viscosity of the material are also discussed.
This is the theory of the principle of dental hybrid layer.
Chemical Bonding
Chemical bonding which is much stronger than physical bonding includes covalent, ionic, and metallic bonding. Chemical bonding is responsible for cohesive forces inside the material itself.
Electrostatic Theory and Acid-Base Theory
The basis of the electrostatic theory of adhesion is the difference in electronegativity of adhering materials. The surface has its structural defects and heterogeneity in electronic community generating a low range (1–2 nm) but very strong field (>108 V m−1). Adhesive force is attributed to the transfer of electrons across the interface creating positive and negative charges that attract one another. The electrostatic forces at the interface account for resistance to separation of the adhesive and the substrate.
The surface of a substrate has plurality of polar sites, which have the acidity of the electron donor and/or the alkalinity of the electron acceptor. These sites can be linked to the reverse polarity activity of adhesives. Therefore, the polar connection will result from the load transfer between the base donor position and the adhesive acceptor position, and vice versa.
Adhesion to Teeth
Paradoxically, the vast majority of adhesives do not have adhesive ability to the surfaces. Only a few adhesives have adhesive ability because of active monomers such as 4-methacryl ethyl triacrylate (4-META) or 10-methacryl decyl phenyl phosphate (10-MDP).
Therefore, a coupling agent is used to adhere to the dental surface. The coupling agent involves a reactive polar group, which can be connected with dental tissue on one hand and an adhesive material on the other hand (HEMA).
Adhesion to Enamel
The conventional resins that are highly hydrophobic have no adhesive ability to dental surface.
The solution for bonding these surfaces is by creating favorable surface topography for anchorage, finding a coupling agent to bind to the dental tissues and the adhesive.
Phosphoric acid etching can clean the enamel surface, improve the surface energy of the substrate, and improve the wettability of the adhesive. On the other hand, acid etching produces a roughness (up to 50 microns) where resin can penetrate. In fact, this highly mineralized tissue has a composite structure which leads to selective etching of certain hydroxyapatite phases (Sato et al., 2018).
The depth and quality of etching will depend on some parameters as listed below:
(1) Acid properties
Phosphoric acid treatment seems to be the best for enamel etching. However, if we want to bond to enamel and dentin, the choice of acid is affected by dentin treatment, and the dentin must also be etched. Compared with the enamel, the complexity of the dentin adhesion has led to the development of other adhesives based on other acids, minerals (2.5% nitric acid), or organic compounds (10% maleic acid and 10% citric acid). It is used to etch enamel and dentin at the same time (total etch). However, the effectiveness of these acids does not seem equal to that of phosphoric acid (Benetti et al., 2019; Markovic et al., 2019).
(2) Concentration
Generally, 10–60% of the phosphoric acid solution seems to have similar adhesiveness. However, the acid concentration affects the depth of relief. In addition, the white appearance after etching is only obtained from a concentration of 20% (Ajaj et al., 2020). The concentration of 35% appears to be optimal.
(3) Consistency
A study showed that gels are as effective as liquid solutions. Therefore, we prefer colored gels that allow precise operation and good visual control (Cardenas et al., 2018).
(4) Application time
It seems the same bonding value between 5 and 37% phosphoric acid etching in the range of 15 s to 1 min. It proves good tolerance of enamel etching with different prosthesis (Cardenas et al., 2018; Sai et al., 2018).
It should be noted that contamination of the etched substrate with salivary or blood proteins can affect the quality of the bonding. Because the molecules are adsorbed on the surface, a simple rinsing is insufficient to eliminate these molecules. Therefore, it seems reasonable to have a new etching. Clinically, this has proved the importance of using the oral rubber dam to avoid the contamination.
Therefore, adhesion to enamel responds to two mechanisms: the first is physicochemical by increasing the wettability and surface area, and the second is the micromechanical, where the adhesive has penetrated and hardened the roughness produced by the acid.
Dentin Adhesion
Because the structure of dentin is complex, there are many problems regarding bonding with dentin.
(1) Dentin are hydrophilic and saturated oxygen.
(2) Dentin continues to form throughout life.
(3) Mineral phase (70%), organic phase (20%).
(4) Its complex structure varies from the depth of the tubule. In fact, near the dentinoenamel junction, only 1% dentinal tube is present on the dental surface and 22% dentinal tube is near the pulp.
(5) Dentin is covered by dentin mud. It should be noted that the removal of dentin by milling creates a deposit smear layer.
Therefore, these obstacles make it difficult to develop reliable dental adhesive systems.
General Principles
To implement the hybridization protocol, three basic components are needed:
(1) A conditioner
The application of the conditioner corresponds to acid etching. This reaction is acid-based with hydroxyapatite and smear layer, resulting in dissolution. It allows to eliminate the dentin mud and contaminants, open the tubules, demineralize the peri- and inter-tubular dentins over a thickness (1–5 µm) and form a dense collagen network. Many types of acids have been proposed to etch dentin, such as Maleic acid (C4H4O4HOOCCH=CHCOOH), Oxalic acid (C2H2O4 HOOC-COOH), Tartaric acid (HOOC-CHOH-CHOH-COOHC4H6O6), or Ethylenediaminetetraacetic acid (EDTA).
Glutaraldehyde (C5H8O2) is known for its ability to interconnect with collagen and is sometimes incorporated into the conditioner.
Hybridization involves infiltration of the collagen network with adhesive resin. The problem is to find a way to infiltrate this hydrophobic resin into this highly hydrophilic network.
(2) A primer or coupling agent.
The primer permits the opening of the inter-fibrillar space because of the hydrophilic monomers dissolved in a specific solvent which fix the collagen in a humid environment. These monomers have a methacrylate group at one end and a reactive group at the other end. The reactive group can interact with the polar molecules of the dentin, such as the hydroxyapatite of the apatite or the amine of collagen. The most commonly used is HEMA which has a positive effect on dentin adhesion. It is necessary to infiltrate the primer because the drying dentin surface would cause collage collapse.
The solvent can be alcohol or acetone, which can help the active monomer penetrate into the network and also eliminate the water.
(3) An adhesive resin
The last step is to apply the adhesive resin, which must penetrate into the tubules and infiltrate into intercellular protein networks.
Application of Bonding System
Paradoxically, the vast majority of adhesives do not have adhesive ability to the surfaces. Only a few adhesives have this ability because of the active monomers, such as 4-methacryl ethyl triacrylate (4-META) or 10-methacryl decyl phenyl phosphate (10-MDP).
Therefore, a coupling agent is used to adhere to the dental surface. The coupling agent involves a reactive polar group, which can be connected with dental tissue on one end and with adhesive material on the other end (HEMA).
Since the vast majority of adhesives do not have adhesive ability, the bonding is done by forming a mixing layer between the teeth or the porcelain and the adhesive (HEMA).
Nevertheless, there exist active monomers such as (4-META, 10-MDP, and 11-methacrylic oxygen-1, 1-eleven alkane two carboxylic acid (MAC 10) with adhesive ability.
On using 4-META, the dentin was treated by 10% citric acid and 3% ferric chloride. Citric acid allows opening of the dentinal tubules and collagen network. Ferric chloride can help form polar sites and enhance affinity to 4-META. It is forbidden to use H3PO4 on dentin because it creates low bonding value with 4 META-MMA resin, changes collagen nature, and prevents the penetration of monomers. Polymerization is initiated by three-N-butyl borane (TBB). This initiation mode is unique, because unlike the peroxide amine, dibromobenzene tribromide is not inhibited by oxygen and water, rather activated.
PMMA provides good viscoelastic properties and provides stress relaxation ability through reversible deformation. Therefore, the interfacial stress is reduced.
Adhesion to Metal Alloy Prosthesis
In order to fix a dental prosthesis to teeth, it is necessary to obtain a strong and durable adhesion on both sides. Adhesives based on hydrophobic monomers such as bis-GMA and UDMA do not have durable adhesion to alloy and to ceramic. Adhesives with reactive monomers such as 4-META or 10-MDP have the ability to spontaneously adhere to metal surfaces, especially to non-precious alloys. However, the adhesion value seems to be sensitive to long-term degradation, especially hydrolysis. Therefore, we show different technologies on metal and ceramic, both for conventional and reactive adhesives.
Surface Mechanical Reconstruction
Micro Mechanical Maintenance
Rochette suggested in 1973 that metal splint should be bonded on etched enamel. The metal splint was punched so that the resin can be mechanically fixed and maintained. However, the disadvantage of this technology was that on the one hand, the surface of the exposed adhesives increased, resulting in wear and penetration, while on the other hand, the metal surface greatly reduced. Therefore, with the improvement of adhesive quality and development of metal surface preparation, the signs of macromechanical technology gradually disappeared.
Micro Mechanical Retention
The principle is to change the topography of the metal surface without changing its chemical composition.
Alumina sandblasting (Al2O3, 50–250 micron) is a simple treatment method, which on the one hand can purify the surface contaminants of the possible adsorbents, thereby enhancing the surface tension. On the other hand, it can create a kind of roughness i.e., favorable for micromechanical retention.
A study showed that alumina sandblasting achieves bonding performance for non-precious metal alloy. For those adhesives without adhesive ability, the sandblasting cannot provide enough retention.
Electrolytic or Acid Etching
This technology was developed in the early 1980s, providing substrate compatible with conventional adhesives. It only applies to non-noble alloys (Ni-Cr, Cr-Co) with eutectic microstructure, allowing one part of the two phases to dissolved to produce high roughness surfaces. Therefore, owing to the micromechanical bonding provided by the surface, the method obtained high strength resin adhesion. However, it has been pointed out that the efficiency of Cr-Co alloy is lower than that of Ni-Cr alloy. In addition, some studies also showed that the results of Ni-Cr were poor.
Because electrolysis etching requires special equipment and high skill, acid etching has become a popular method. This method simply includes the application of high concentration hydrogen fluoride hydrogel (HF).
Because of the lack of effective electrolytic etching, a fine salt crystal layer is deposited on the metal surface before making the resin model. Once the resin is polymerized, the salt crystals were dissolved away from the resin, leaving a reproductible cap.
Chemical Changement for Noble Alloy
The poor bonding quality between the noble alloy and the adhesive is related to the low surface reactivity. We can modify the surface by chemical method and make it suitable for bonding.
Tin Deposit
The principle is to depose tin microcrystals onto the alloy surface, giving it a gray appearance. Tin is chemically linked to the surface to create micromechanical retention and forms polar bonds with the reactive groups of the adhesive.
However, the coupling between tin deposition (electronegativity) and the noble alloy substrate (electropositivity) may lead to corrosion and degradation of the joint.
Silica Deposit
The following two methods have been worked out:
(1) Thermochemical deposition
The Silicoater MD (Heraeus Kulzer) system consists of siloxane application: tetramethoxy silane, thermal dissolving in propane air flame or in an oven to deposit 0.5-micron siloxane.
(2) Tribochemical deposit
The surface is sanded with special powder containing alumina covered with colloidal silica under high pressure. The energy generated by the impact of pressurized silica particles on the metal surface leads to the binding of silica. These systems include the ROCATEC the COJET 3M ESPE system.
After silica deposition, the surface is treated with silane. It can be connected to the free hydroxyl (-OH) group and play an intermediate role between the silica layer and the adhesive resin. Regardless of the alloy and adhesive used, this method improves both the bonding value and the resistance to degradation.
Chemical Improvement
The poor bonding quality between the noble alloy and the self-etching adhesive is related to the low surface reactivity. We can chemically alter the surface and make it more suitable for bonding adhesives.
After silicon deposition, the surface is treated with silane. It can be connected to the free hydroxyl group (-OH) and play an intermediate role between the silica layer and the resin.
As explained earlier, regardless of the alloy and adhesive used, these methods improve both the bonding value and provide better resistance to degradation.
Metal Primers
Active monomers, such as those listed in Table 2, have the ability to react with the surface oxide of non-noble alloys such as Ni-Cr, Cr-Co, or steel, thereby providing strong and stable adhesion (Ikemura et al., 2011; Al Bakkar et al., 2016). The MDP seemed to be the most effective monomer. For example, for Superbond, metal primer is a liquid (MMA monomer +5% 4-META) activated by TBB, with a significantly improved bonding value.
Because noble alloy surfaces have little or no oxide, the primer based on acid monomers will be ineffective. Similarly, adhesives based on 4-META, 10-MDP have poor adhesion to precious alloys. The adhesion can be improved by applying specific primers.
These are based on a coupling agent using bifunctional monomers. The bifunctional monomer has methacrylic groups at one end and thiol groups (-SH) at the other end. On the sanded metal surface, the sulfide group (S2−) reacts with the noble alloy to form a strong chemical combination.
It is worth noting that these preparations are developed for specific types of adhesives and are not always able to withstand penetration. The metal primer 6- (4-vinylbenzyl-n-propyl) amino-1,3,5-triazine-2,4-dithione (VBATDT) is suitable for 4-META resin, but not for conventional methyl-based low-temperature adhesives. On the contrary, the AMPS monomer (C7H13NO4S) designed for conventional resin is very poor in bonding with 4-META resin.
Ultraviolet irradiation (UVI) processing efficiently increases the bond strength between porcelain and the Ti surface (Kumasaka et al., 2018). Because of the high strength of noble alloy surface, the acid monomer will not be effective. It can be concluded that alloy primer containing both the vinyl-thione monomer (VBATDT) and hydrophobic phosphate monomer (MDP) is effective for bonding the Ag-Zn-Sn-In alloy and pure Ag, Zn, and Sn (Imamura et al., 2018).
For highly translucent zirconia, chemical pre-treatment with an MDP-containing primer improved bonding efficacy of 4-META/MMA-TBB resin (Shimizu et al., 2018).
The polymerization kinetics of composites is material dependent which affects their contraction stress and clinical performance (Wang et al., 2019).
Ceramic Bonding
Traditional resins have no natural affinity to ceramics because ceramics are hydrophilic like resins, especially due to surface hydroxyl groups. By combining the technology with the application of coupling agents, the film surface can be adhered with ceramics.
However, improving the mechanical and optical quality of adhesives and ceramics is very important to improve the reliability of ceramic bonding.
Therefore, the adhesion of ceramics is controlled by combining the acid retaining micro-computer retention agent and the chemical retention agent through the coupling agent (Blatz et al., 2018). For computer-aided design (CAD)/computer-aided manufacturing (CAM)-fabricated, high-strength ceramics, proper pretreatment of the bonding surfaces and application of primers or composite resins that contain special adhesive monomers are necessary (Yoshihara et al., 2016; Dapieve et al., 2020).
Ceramic surface treatment by primer containing monobond can promote micromechanical interlocking and chemical bonds for lithium disilicate (Li2O5Si2) (vitroceramique) restorations.
The application of fluorofluoric acid for ceramic will greatly increase the roughness. In fact, in an enhanced Feldspathic ceramic with highly heterogeneous structure, the acid will preferably attack the crystalline phase. Hydrofluoric acid etching changes the pore pattern, crystal structure, roughness, and wettability of silica-based glass ceramics (Figure 7) (Ramakrishnaiah et al., 2016; El-Damanhoury and Gaintantzopoulou, 2018). Another study proved that the ceramic primer promoted the fatigue performance of lithium silicate ceramic (Dapieve et al., 2020; Fonzar et al., 2020). Phosphoric acid is completely ineffective for ceramic etching, but it can be used as a cleaning agent.
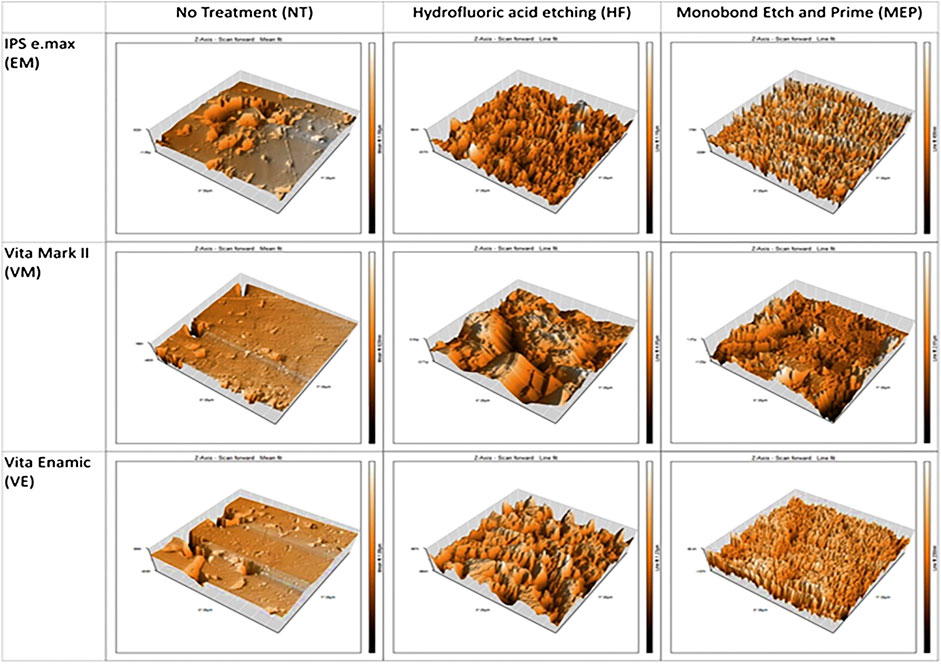
FIGURE 7. Micrographs of the materials tested after no treatment (NT), hydrofluoric acid etching/Monobond Plus (HFMP) or self-etching ceramic primer Monobond Etch and Prime (MEP) pretreatment.
Some defects of hydrogen fluoride should be noted:
(1) This is a very toxic substance and needs to be handled with great care.
(2) If the residue is not completely neutralized before bonding, the residue can spread after the event and cause tissue damage.
(3) Even in gel form, it tends to burn because it may not bite the edges, which may have an adverse effect on the sealing of the edges.
(4) On the other hand, the bite mark beyond the boundary is a marginal factor of plate maintenance and inflammation.
When the two materials are chemically incompatible, the cured solution is a coupling agent that has intermediate properties between the two substrates-silane (SiH4). As a result, condensation reaction occurs between the silane and the hydroxyl groups of the substrate on the surface of the ceramic surface, and the water molecules are released. Therefore, silane molecules are linked to the ceramic surface by covalent bonding.
Theoretically, the aim is to provide a monolayer of silane, but there is actually a gap composed of several silane and oligomer layers, which are basically connected with ceramic or resin that may damage the hydrolytic stability of the adhesive bonding. This method has greatly improved the hydrolytic stability of seals. Before applying silane, the prosthetic components must be carefully cleaned with alcohol, acetone, or phosphoric acid. Some silanes are packed in methyl form, which need acid activation, and then mixed in two liquids shortly before application.
Measurement of Bond Strength
Among the various methods used to test the bond strength of a joint, the shear and tensile tests are common. Regardless of the test used, many variable parameters influence the bond strength, including the interface geometry (Thoppul et al., 2009), force loading (Souza et al., 2015), and speed of the test (Abreu et al., 2011). This variability, which makes comparison among studies virtually impossible, may be due to sensitivity to the geometry of the interface (Thoppul et al., 2009), the loading of the force (Souza et al., 2015), and the solicitation speed of the test (Abreu et al., 2011). Additionally, the clinical and laboratory performances of dental adhesives rarely coincide, because dental adhesion tests are incapable of separating the effects of adhesive composition, substrate properties, joint geometry, and loading type on the measured bond strength (Jancar, 2011). Therefore, to evaluate the inherent material properties of an adhesive joint, tests measuring the adherence energy have been proposed. The concept behind these tests is to initiate and propagate a crack through the bonded interface in a stable manner (Bechtle et al., 2010).
The double cantilever beam (DCB) test and the notchless triangular prism (NTP) are two approaches that may be used to evaluate the adherence energy of a bonded joint. Theoretically, the data obtained by these tests are independent of the specimen geometry and provide a real, characteristic value of the bonding joints. The mechanics and principles of the DCB test have been described in detail in a previous study (Blaysat et al., 2015). The DCB test is a cleavage test that measures the value of Gc, which is an energy value that reflects the durability of the metal/resin interface. The DCB method provides valuable insights into how the microstructure enhances the toughness of the dental composite (De Souza et al., 2011). The DCB test was recently successfully applied to evaluate the mode I fracture energy of hydrated and thermally dehydrated cortical bone tissues of the young bovine femur, which has a composition similar to that of human dentine (Kageyama et al., 1987). Moreover, results from the DCB test have shown a good correlation with clinical findings (Dastjerdi et al., 2013).
In 1965, Irwin (1958) reported that the stress field around a sharp crack in a linear-elastic material could be uniquely defined by the stress intensity factor K, and that fracture occurs when the value of K exceeds some critical value of the fracture toughness, Kc. Thus, K is a stress field parameter i.e., material-independent, whereas KC reflects an inherent material property. K1C is the critical stress intensity fracture value for crack growth in the material during mode I. The NTP was derived from the chevron-notched short rod (CNSR, ASTM E1304-89) to develop a new method for evaluating the fracture property of materials. Bubsey detailed the mechanics of the NTP test in 1982 (Bubsey et al., 1982), and the test was first applied to dental biomaterials by Ruse in 1996 (Ruse and Feduik, 1996). The NTP test measures the toughness of the interface in bond joints (K1C). According to finite element analysis, the K1C values of poly (methyl methacrylate) obtained by the NTP correlate well with values obtained by the CNSR (Bubsey et al., 1982). These results suggest that the NTP test may be used to determine the fracture mechanics of bulk materials and adhesive interfaces.
Conclusion and Future Prospects
Though dental bonding system are becoming simplified and more efficacy, dentists still must follow manufacturer’s direction, adhere to the correct sequence of steps and observe the proper application times of each step. Though fewer bottles are needed, dentists should understand that not all structure is the same and not all material, be it composite, porcelain, zirconia or metal, can be treated similarly.
It is hoped that more dentists will be able to preserve natural teeth and update research on bonding materials in the field of dental bonding in the future. Perhaps the innovative technology of dental bonding materials will bring great impetus to the field of dental bonding research.
Author Contributions
ZZ and QW wrote the manuscript with support from JZ, BZ, ZM, and CZ. All authors contributed to the general discussion.
Funding
This work was supported by the Program of Scientific Development of Jilin Province (20180414043GH and 20190304112YY), the program of Jilin University (3R2201543428), and the program of the First Hospital of Jilin University (01032140001, 04023720002, and JDYYJC002).
Conflict of Interest
The authors declare that the research was conducted in the absence of any commercial or financial relationships that could be construed as a potential conflict of interest.
References
Abreu, C. W., Santos, J. F. F., Passos, S. P., Michida, S. M. A., Takahashi, F. E., and Bottino, M. A. (2011). The influence of cutting speed and cutting initiation location in specimen preparation for the microtensile bond strength test. J. Adhesive Dent 13 (3), 221–226. doi:10.3290/j.jad.a21540
Ajaj, M. T., Al-Khateeb, S., and Al-Batayneh, O. B. (2020). Effect of different acid etchants on the remineralization process of white-spot lesions: an in vitro study. Am. J. Dent 33 (1), 43–47.
Al Bakkar, H., Spintzyk, S., Schille, C., Schweizer, E., Geis-Gerstorfer, J., and Rupp, F. (2016). Influence of a bonding agent on the bond strength between a dental Co–Cr alloy and nine different veneering porcelains. Biomed. Tech 61 (5), 509–517. doi:10.1515/bmt-2015-0101
Ando, T., and Skolnick, J. (2010). Crowding and hydrodynamic interactions likely dominate in vivo macromolecular motion. Proc. Natl. Acad. Sci. U.S.A 107 (43), 18457–18462. doi:10.1073/pnas.1011354107
Bechtle, S., Fett, T., Rizzi, G., Habelitz, S., Klocke, A., and Schneider, G. A. (2010). Crack arrest within teeth at the dentinoenamel junction caused by elastic modulus mismatch. Biomaterials 31 (14), 4238–4247. doi:10.1016/j.biomaterials.2010.01.127
Benetti, A. R., Michou, S., Larsen, L., Peutzfeldt, A., Pallesen, U., and van Dijken, J. W. V. (2019). Adhesion and marginal adaptation of a claimed bioactive, restorative material. Biomater. Investig. Dent 6 (1), 90–98. doi:10.1080/26415275.2019.1696202
Blatz, M. B., Vonderheide, M., and Conejo, J. (2018). The effect of resin bonding on long-term success of high-strength ceramics. J. Dent. Res 97 (2), 132–139. doi:10.1177/0022034517729134
Blaysat, B., Hoefnagels, J. P. M., Lubineau, G., Alfanob, M., and Geersa, M. G. D. (2015). Interface debonding characterization by image correlation integrated with Double Cantilever Beam kinematics. Int. J. Solid Struct 55, 79–91. doi:10.1016/j.ijsolstr.2014.06.012
Bubsey, R. T., Munz, D., Pierce, W. S., and Shannon, J. L. (1982). Compliance calibration of the short rod chevron-notch specimen for fracture-toughness testing of brittle materials. Int. J. Fract 18 (2), 125–133. doi:10.1007/BF00019637
Cardenas, A. F. M., Siqueira, F. S. F., Bandeca, M. C., Costa, S. O., Lemos, M. V. S., Feitora, V. P., et al. (2018). Impact of pH and application time of meta-phosphoric acid on resin-enamel and resin-dentin bonding. J. Mech. Behav. Biomed. Mater 78, 352–361. doi:10.1016/j.jmbbm.2017.11.028
Dapieve, K. S., Machry, R. V., Pilecco, R. O., Kleverlaan, C. J., Pereira, G. K. R., Venturini, A. B., et al. (2020). One-step ceramic primer as surface conditioner: effect on the load-bearing capacity under fatigue of bonded lithium disilicate ceramic simplified restorations. J. Mech. Behav. Biomed. Mater 104, 9. doi:10.1016/j.jmbbm.2020.103686
Dastjerdi, A. K., Tan, E., and Barthelat, F. (2013). Direct measurement of the cohesive law of adhesives using a rigid double cantilever beam technique. Exp. Mech 53 (9), 1763–1772. doi:10.1007/s11340-013-9755-0
De Souza, J. A., Goutianos, G., Skovgaard, M., and Sørensen, B. F. (2011). Fracture resistance curves and toughening mechanisms in polymer based dental composites. J. Mech. Behav. Biomed. Mater 4 (4), 558–571. doi:10.1016/j.jmbbm.2011.01.003
El-Damanhoury, H. M., and Gaintantzopoulou, M. D. (2018). Self-etching ceramic primer versus hydrofluoric acid etching: etching efficacy and bonding performance. J. Prosthodont. Res 62 (1), 75–83. doi:10.1016/j.jpor.2017.06.002
Fonzar, R. F., Goracci, C., Carrabba, M., Louca, C., Ferrari, M., and Vichi, A. (2020). Influence of acid concentration and etching time on composite cement adhesion to lithium-silicate glass ceramics. J. Adhes. Dent 22 (2), 175–182. doi:10.3290/j.jad.a44282
Hotchkiss, K. M., Reddy, G. B., Hyzy, S. L., Schwartz, Z., Boyan, B. D., and Navarrete, R. O. (2016). Titanium surface characteristics, including topography and wettability, alter macrophage activation. Acta Biomater 31, 425–434. doi:10.1016/j.actbio.2015.12.003
Ikemura, K., Fujii, T., Negoro, N., Endo, T., and Kadoma, Y. (2011). Design of a metal primer containing a dithiooctanoate monomer and a phosphonic acid monomer for bonding of prosthetic light-curing resin composite to gold, dental precious and non-precious metal alloys. Dent. Mater. J 30 (3), 300–307. doi:10.4012/dmj.2010-163
Imamura, N., Kawaguchi, T., Shimizu, H., and Takahashi, Y. (2018). Effect of three metal priming agents on the bond strength of adhesive resin cement to Ag-Zn-Sn-In alloy and component metals. Dent. Mater. J 37 (2), 301–307. doi:10.4012/dmj.2017-139
Irwin, G. R. (1958). “Fracture,” in Elasticity and Plasticity/Elastizität und Plastizität. Editor S. Flügge (Berlin, Heidelberg, Germany: Springer Berlin Heidelberg), 551–590.
Jancar, J. (2011). Bond strength of five dental adhesives using a fracture mechanics approach. J. Mech. Behav. Biomed. Mater 4 (3), 245–254. doi:10.1016/j.jmbbm.2010.09.004
Kageyama, K., Kobayashi, T., and Chou, T. W. (1987). Analytical compliance method for mode-I interlaminar fracture-toughness testing of composites. Composites 18 (5), 393–399. doi:10.1016/0010-4361(87)90364-8
Khargekar, N. R., Kalathingal, J. H., Sam, G., Elpatal, M. A., Hota, S., and Bhushan, P. (2019). Evaluation of different pretreatment efficacy with fluoride-releasing material on shear bond strength of orthodontic bracket: an in vitro study. J. Contemp. Dent. Pract 20 (12), 1442–1446. doi:10.5005/jp-journals-10024-2697
Kumasaka, T., Ohno, A., Hori, N., Hoshi, N., Maruo, K., and Kuwabara, A. (2018). Influence of ultraviolet irradiation treatment on porcelain bond strength of titanium surfaces. Dent. Mater. J 37 (3), 422–428. doi:10.4012/dmj.2016-417
Markovic, D. L., Petrovic, B. B., Peric, T. O., Trisic, D., Kojic, S., and Kuljic, B. L. (2019). Evaluation of sealant penetration in relation to fissure morphology, enamel surface preparation protocol and sealing material. Oral Health Prev. Dent 17 (4), 349–355. doi:10.3290/j.ohpd.a42689
Nguyen, T. M. T., Wang, P. W., Hsu, H. M., Cheng, F. Y., Shieh, D. B., Wong, T. Y., Chang, H. J., et al. (2019). Dental cement’s biological and mechanical properties improved by ZnO nanospheres. Mater. Sci. Eng. C Mater. Biol. Appl 97, 116–123. doi:10.1016/j.msec.2018.12.007
Paing, S. Y., Tichy, A., Hosaka, K., Nagano, D., Nakajima, M., and Tagami, J. (2020). Effect of smear layer deproteinization with HOCl solution on the dentin bonding of conventional and resin-modified glass-ionomer cements. Eur. J. Oral Sci 128 (3), 255–262. doi:10.1111/eos.12694
Qiu, X. H., Xu, S., Hao, Y., Peterson, B., Li, B., Yang, K., et al. (2020). Biological effects on tooth root surface topographies induced by various mechanical treatments. Colloids Surf. B Biointerfaces 188, 110748. doi:10.1016/j.colsurfb.2019.110748
Ramakrishnaiah, R., Alkheraif, A. A., Divakar, D. D., Matinlinna, J. P., and Vallittu, P. K. (2016). The effect of hydrofluoric acid etching duration on the surface micromorphology, roughness, and wettability of dental ceramics. Int. J. Mol. Sci 17 (6), 17. doi:10.3390/ijms17060822
Ruse, N. D., and Feduik, D. (1996). Notchless triangular prism (NTP) fracture toughness testing of dental luting cements. J. Dent. Res 75, 23.
Sai, K., Takamizawa, T., Imai, A., Tsujimoto, A., Ishii, R., and Barkmeier, W. W. (2018). Influence of application time and etching mode of universal adhesives on enamel adhesion. J. Adhes. Dent 20 (1), 65–77. doi:10.3290/j.jad.a39913
Sang, T., Ye, Z., Fischer, N. G., Skoe, E. P., Echeverría, C., Wu, J., et al. (2020). Physical-chemical interactions between dental materials surface, salivary pellicle and Streptococcus gordonii. Colloids Surf. B Biointerfaces 190, 10. doi:10.1016/j.colsurfb.2020.110938
Sato, T., Takagaki, T., Ikeda, M., Nikaido, T., Burrow, M. F., and Tagami, J. (2018). Effects of selective phosphoric acid etching on enamel using “no-wait” self-etching adhesives. J. Adhes. Dent 20 (5), 407–415. doi:10.3290/j.jad.a41359
Shahid, S., Billington, R. W., Hill, R. G., and Pearson, G. J. (2010). The effect of ultrasound on the setting reaction of zinc polycarboxylate cements. J. Mater. Sci. Mater. Med 21 (11), 2901–2905. doi:10.1007/s10856-010-4146-x
Shimizu, H., Inokoshi, M., Takagaki, T., Uo, M., and Minakuchi, S. (2018). Bonding efficacy of 4-META/MMA-TBB resin to surface-treated highly translucent dental zirconia. J. Adhes. Dent 20 (5), 453–459. doi:10.3290/j.jad.a41330
Sofan, E., Sofan, A., Palaia, G., Tenore, G., Romeo, U., and Migliau, G. (2017). Classification review of dental adhesive systems: from the IV generation to the universal type. Ann. Stomatol 8 (1), 1–17. doi:10.11138/ads/2017.8.1.001
Souza, A. C. D., Gonçalves, F. C. P., Anami, L. C., de Melo, R. M., Bottino, M. A., and Valandro, L. P. (2015). Influence of insertion techniques for resin cement and mechanical cycling on the bond strength between fiber posts and root dentin. J. Adhes. Dent 17 (2), 175–180. doi:10.3290/j.jad.a33993
Thoppul, S. D., Finegan, J., and Gibson, R. F. (2009). Mechanics of mechanically fastened joints in polymer-matrix composite structures–a review. Compos. Sci. Technol 69 (3–4), 301–329. doi:10.1016/j.compscitech.2008.09.037
van der Spoel, D., van Maaren, P. J., Larsson, P., and Tîmneanu, N. (2006). Thermodynamics of hydrogen bonding in hydrophilic and hydrophobic media. J. Phys. Chem. B 110 (9), 4393–4398. doi:10.1021/jp0572535
Wang, R., Liu, H., and Wang, Y. (2019). Different depth-related polymerization kinetics of dual-cure, bulk-fill composites. Dent. Mater 35 (8), 1095–1103. doi:10.1016/j.dental.2019.05.001
Wetzel, R., Eckardt, O., Biehl, P., Brauer, D. S., and Schacher, F. H. (2020). Effect of poly(acrylic acid) architecture on setting and mechanical properties of glass ionomer cements. Dent. Mater 36 (3), 377–386. doi:10.1016/j.dental.2020.01.001
Keywords: adhesion, tooth, dental, restoration, bonding, prosthesis
Citation: Zhao Z, Wang Q, Zhao J, Zhao B, Ma Z and Zhang C (2021) Adhesion of Teeth. Front. Mater. 7:615225. doi: 10.3389/fmats.2020.615225
Received: 08 October 2020; Accepted: 26 November 2020;
Published: 11 January 2021.
Edited by:
Junchao Wei, Nanchang University, ChinaCopyright © 2021 Zhao, Wang, Zhao, Zhao, Ma and Zhang. This is an open-access article distributed under the terms of the Creative Commons Attribution License (CC BY). The use, distribution or reproduction in other forums is permitted, provided the original author(s) and the copyright owner(s) are credited and that the original publication in this journal is cited, in accordance with accepted academic practice. No use, distribution or reproduction is permitted which does not comply with these terms.
*Correspondence: Congxiao Zhang, zhangcongxiao@jlu.edu.cn
†These authors have contributed equally to this work