- 1Ph.D. Program in Biomedical Sciences, University of Maryland School of Dentistry, Baltimore, MD, United States
- 2Department of Restorative Dental Sciences, Imam Abdulrahman Bin Faisal University, College of Dentistry, Dammam, Saudi Arabia
- 3Department of Preventive Dental Sciences, Imam Abdulrahman Bin Faisal University, College of Dentistry, Dammam, Saudi Arabia
- 4Department of Dental Materials, School of Dentistry, Federal University of Rio Grande do Sul, Porto Alegre, Brazil
- 5Biomaterials and Tissue Engineering Division, Department of Advanced Oral Sciences and Therapeutics, University of Maryland School of Dentistry, Baltimore, MD, United States
- 6Division of Operative Dentistry, Department of General Dentistry, University of Maryland School of Dentistry, Baltimore, MD, United States
Resin composites are the material of choice for dental restorative treatment in oral health care. However, the inherent composition of this class of material commonly results in microbial adherence and colonization, which carries the potential risk of recurrent carious lesions around dental restorations. The high risk of resin composites failure complicates the treatment of root caries, defined as the onset of tooth decay over the prone root surface of a tooth. The restorative treatment of root caries among high caries risk individuals, especially for senior patients, is a challenging, painful, and costly. The dysbiotic microbiota colonizes the composite’s surfaces and forms polymicrobial biofilms that are difficult to be dislodged by regular tooth brushing. This study assesses the antibiofilm performance of a surface contact killing antibacterial dental resin composites on the growth of microcosm biofilms using dental plaque sampled from patients with active root carious lesions as an inoculum. The designed formulations contain dimethylaminohexadecyl methacrylate (DMAHDM), a tailored quaternary ammonium monomer with an alkyl chain length of 16, at 3–5 wt.% in a base resin with and without 20 wt.% nanoparticles of amorphous calcium phosphate (NACP). Biofilms were grown on the tested resin composites using a 48 h plaque-derived microcosm biofilm model. Dental plaque collected from active root carious lesions was used as an inoculum to emulate the microbiota present in those lesions. The biofilm growth was assessed via the colony-forming unit (CFU) counts in four culture media, metabolic behavior, lactic acid production, and confocal microscopy. The percentage of reacted double bonds of the formulations was also investigated. The dental resin composites formulated with 3–5 wt.% DMAHDM and 20 wt.% NACP were effective at eradicating surface-attached biofilms from the total microbial load and each relevant cariogenic group: total streptococci, mutans streptococci, and lactobacilli. The metabolic activities and lactic acid production of the plaque-derived microcosm biofilms were reduced by 80–95%, respectively. Fewer viable microorganisms were observed over resin composites containing DMAHDM and NACP. Besides, all the experimental formulations demonstrated an acceptable degree of conversion values. This new strategy fits with ongoing dental caries preventive and minimally invasive approaches by preventing biofilm growth over-restored carious root lesions and improving the lifespan of dental restorations.
Introduction
Dysbiotic biofilm-triggered mineral loss causes tooth cavitation, and it is the primary reason for the loss of tooth structure (Takahashi and Nyvad, 2016; Damé-Teixeira et al., 2017). When it is located at the root of a tooth, noted as “root caries,” the onset of carious lesions could be accelerated due to multiple risk factors (Takahashi and Nyvad, 2016; Damé-Teixeira et al., 2017). Root caries is a preventable dental disease that affects a growing number of adults. The annual incidence of root caries ranged from 10.1 to 40.6%, with the highest-burden observed among elderly patients (Hayes et al., 2017a).
Root surfaces are at higher risk of biofilm-triggered mineral loss compared to enamel, as the cement and dentin, constituents of the root, hold lower mineral contents compared to the enamel. As a result, microorganisms involved in root caries are less dependent on carbohydrates, and less amount of pH drop is required to induce demineralization (Takahashi and Nyvad, 2016; Damé-Teixeira et al., 2017). Moreover, the location of the root surface close to the gingival margin and cementoenamel junction is considered a plaque-stagnation area, making it more difficult to be cleaned by brushing (Vandana and Haneet, 2014). As the present demographic shifts, the population of the United States over the age of 65 years is projected to increase from 13.5 to 20% in 2030 (The United States census bureau, 2019). Therefore, there is a demographic and oral health imperative to understand how to better care for senior dental patients who are at high risk of root caries (Gati and Vieira, 2011; Tonetti et al., 2017).
Currently, the standard of care on the management of root caries cavitation relies on restorative treatment by placing a circumferential “dental filling” to replace the missing root structure (Tan et al., 2017). Resin composite restorations can perform better in restoring root surfaces compared to glass ionomer and resin-modified glass ionomer restorations (Meyer-Lueckel et al., 2019). However, the survival rate of resin composite restorations in replacing the root surface is poor, as the annual failure rates reach 15% (Meyer-Lueckel et al., 2019).
One of the main reasons for restoration failure is secondary caries, especially around resin composite surfaces, which are more susceptible to plaque accumulation (Bourbia and Finer, 2018). Biofilms have specialized physiology where cells aggregate together and become encased in a self-produced polysaccharide and protein matrix that protects the cells from several oral environmental conditions (Khatoon et al., 2018). Resin composite restorations have no bioactivity and undergo several degradative effects caused by bacterial acids as well as salivary esterase (Delaviz et al., 2014). It is no longer sufficient for dental restorations to simply repair esthetics and function without providing protection against microorganisms and their associated biofilms. In this sense, dentists and dental material researchers worldwide have been perseveringly searching for restorative approaches that can contribute to the longevity of root caries restorative treatment (Gavriilidou and Belibasakis, 2019).
There is currently no approved dental composite that can effectively contribute to the protection of restorations against biofilm accumulation. However, several investigations were conducted to design a bioactive resin composite with antibacterial or ion-releasing properties (Cheng et al., 2012). The use of quaternary ammonium methacrylate, such as 12-methacryloyloxydodecylpyridinium bromide (MDPB) or bis(2-methacryloyloxyethyl) dimethylammonium bromide (QADM) was associated with slightly reduced growth of total microorganisms and caries-related pathogens (Melo et al., 2018). This class of monomers has a positive-charge surface that can interact with the negatively charged bacterial membrane causing cell wall disturbance and lysis (Zubris et al., 2017).
Importantly, these antibacterial monomers can copolymerize with other dental monomers to provide a long-lasting effect with no leaching adverse effects (Yuan et al., 2013). Holding the contact killing property based on bacterial membrane interactions, the recently developed antibacterial monomer, DMAHDM, had its synthesis tailored with a 16-alkyl chain to allow robust antibacterial action against cariogenic biofilms (Ibrahim et al., 2019); covalent bond with the commonly used Bis-GMA/TEGDMA dental resin system (Liang et al., 2014) and high surface charge density (Balhaddad et al., 2020).
In the course of investigating anti-caries approaches via dental materials, the understanding of caries as a dynamic process that shifts toward de- or remineralization has encouraged the incorporation of a potential resource for ion-depleted tooth surfaces around dental restorations (Melo et al., 2017). Nano-sized amorphous calcium phosphate (NACP) fillers may provide the release of calcium and phosphate ions under acidic pH as ion-enriched source incorporated in the dental material resulting in buffering capacity and remineralization effect (Weir et al., 2012).
Clinically, patients with active root carious lesions are assessed at a severely high risk of caries (Hayes et al., 2017b). They very often present several risk-indicators, such as hyposalivation, gingival recession, high cariogenic diet intake, and low fluoride exposure (Ritter et al., 2010). In view of the intended use for root restorations, the developed bioactive resin composite must present maximum anti-biofilm performance to confront high plaque build-up inside the mouth. Considering the bacterial diversity within the biofilms, it would be conceivably preferable to use natural inoculum to facilitate the development of in vitro biofilms to more broadly resemble the in vivo community for testing new antibiofilm biomaterials (Signori et al., 2016). Plaque-derived inoculum can represent the environmental diversity related to the root surface in a site-dependent and niche-dependent manner, making the inoculum more clinically relevant for in vitro models testing new antibacterial compounds (Marsh, 2006; Fontana et al., 2009)
In this study, we examined for the first time the antibiofilm performance of a surface contact killing antibacterial dental resin composites on the growth of microcosm biofilms using dental plaque sampled from patients with active root carious lesions as an inoculum. It was hypothesized that: 1) The DMAHDM -NACP composites would substantially reduce biofilm activities, including colony-forming units (CFU), metabolic activity, and acid production, and 2) Adding DMAHDM and NACP into composite would not compromise the degree of conversion (DC).
Material and Methods
Participants Selection and Plaque Collection
Ethical Considerations
The participants were ongoing patients who visited university dental clinics for regular dental treatment. All participants consented to donate samples of dental plaque after getting comprehensive information about the study. The study protocol and written informed consent from all participants were approved by The University of Maryland Baltimore Institutional Review Board (HP-00083485).
Participants
Each participant had at least one exposed root with a primary active root carious lesion in order to collect the plaque. The average years of age of the participants were 56.8 ± 22.6 (N = 10; 40% female; 60% male). Ten adult volunteers presenting active root carious lesions at clinical oral examination, as shown in Figure 1, were recruited for the plaque collection. The lesions are presented as discoloration and loss of surface continuity or cavitation below the cement-enamel junction (Figures 1A). They were located on the root surface of a tooth, usually close to or below the gingival margin (Figures 1B). The activity of root caries was determined based on the texture (smooth, rough), appearance (shiny or glossy, matte or non-glossy), and location in a plaque-stagnation area (Figures 1C). The inclusion and exclusion criteria of the participants are described in Table 1.
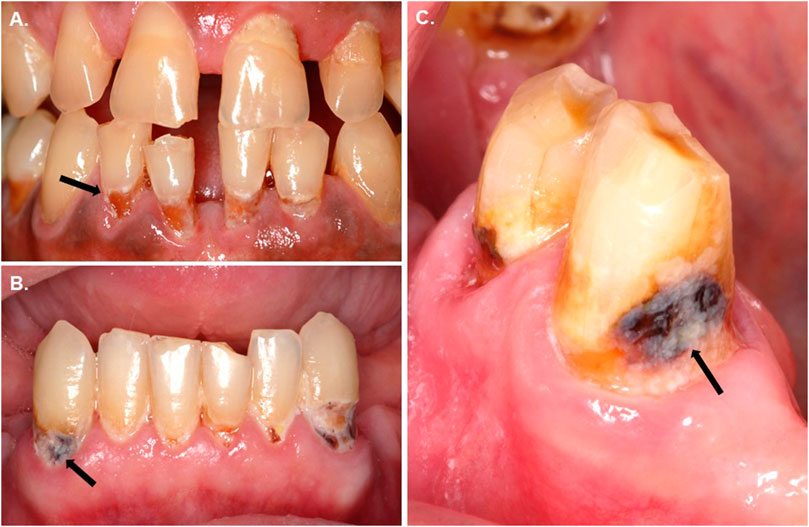
FIGURE 1. Clinical appearance of the carious root lesions showing a massive plaque build-up over exposed root surfaces. The lesions are presented as discoloration and loss of surface continuity or cavitation below the cementoenamel junction (A). Root carious lesions are mainly located on the root surface of a tooth, usually close to or below the gingival margin (B). The activity of such lesion can be determined based on the texture (smooth, rough), appearance (shiny or matte), and location in a plaque-stagnation area (C).
Plaque sampling from patients with active root carious lesions.
The plaque sampling was undertaken at carious root sites. To assure proper sampling, the teeth presenting the lesions were isolated with cotton rolls and slightly air-dried. The dental plaque (supragingival biofilm) was removed using a sterile ultrafine micro-brush applicator (head size: 0.5 mm; MUT400; Microbrush international, Grafton, WI, United States) with a circular motion parallel to the gingival margin, as illustrated in Figure 2 by the sequential images (2A-C). The samples were placed inside a liquid dental transport medium composed of a buffered mineral salt-based liquid medium with reducing agents (Anaerobe System, Morgan Hill, CA, United States) and transferred to the laboratory settings.
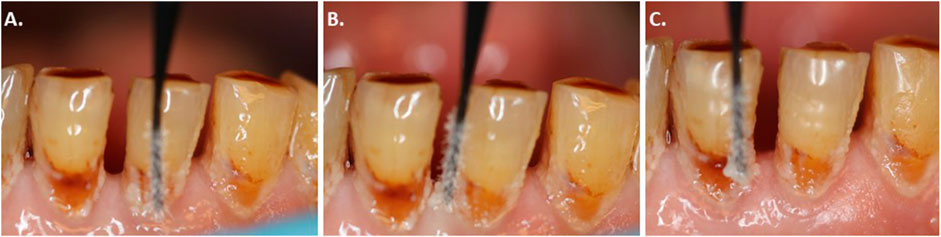
FIGURE 2. (A) Clinical photographic images illustrating how the plaque was scraped and collected from the carious root lesions; (B) The dental plaque (supragingival biofilm) was removed using a sterile ultrafine micro-brush applicator; and (C) with a circular motion parallel to the gingival margin.
The collected plaque samples were mixed, stored in a solution of brain heart infusion, and glycerol at the ratio of 7:3, and stored at −80°C for further plaque-derived microcosm biofilm assays. The addition of glycerol stabilizes the frozen bacteria, preventing damage to the cell membranes and keeping the cells alive (Fontana et al., 2009).
Experimental Design
An in vitro study was conducted using a validated plaque-derived microcosm assay model (Melo et al., 2013). For the purpose of studying the antibacterial activity and DC of the designed formulations, a flowchart of the experimental process was intended, as shown in Figure 3. The factors under study were the DMAHDM concentration at three levels (0, 3, and 5%) and incorporation of NACP at two levels [absence and presence of 20% NACP], generating six groups as described in Table 2. For microbiological analyses derived from in vitro biofilms, four independent experiments were carried out, and the data were statistically analyzed according to the design of this study, considering the biofilm grown over the composite disk as a statistical unit (n = 4 × 3 replicates). The dependent variables were: 1) CFUs counting for total microorganisms, 2) total streptococci, 3) mutans streptococci, and 4) total lactobacilli, 5) metabolic activity, 6) and lactic acid production. For DC, the statistical unit was the cured composite samples (n = 5).
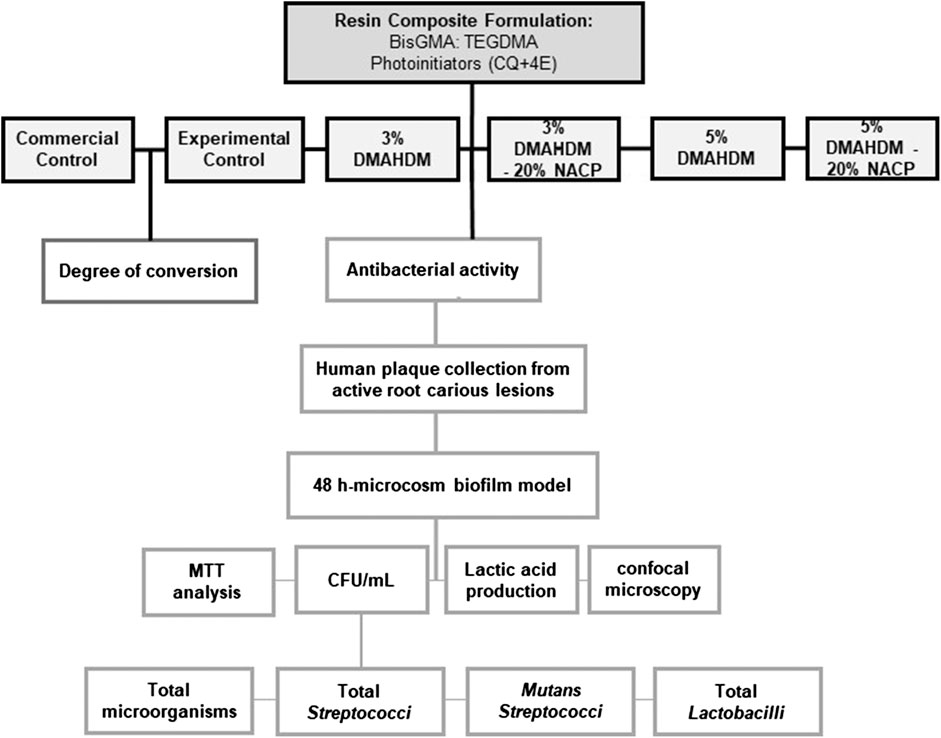
FIGURE 3. Schematic drawing showing the design of the study. Antibacterial resin composite formulations were optimized containing the different mass fraction of DMAHDM with and without NACP. Microbiological analysis and the degree of conversion of the designed formulations were assessed.
Synthesizing the Dimethylaminohexadecyl Methacrylate Monomer and Nano-Sized Amorphous Calcium Phosphate Fillers
A modified Menschutkin reaction was performed to synthesize DMAHDM following previous studies (Zhou et al., 2013). Briefly, 10 mmol of 2-(dimethylamino)ethyl methacrylate (DMAEMA; Sigma-Aldrich, St. Louis, MO, United States), 10 mmol of 1-bromohexadecane (BHD, TCI America, Portland, OR, United States), and 3 g of ethanol were placed in a 20 ml-scintillation vial and mixed very well and stirred at 70°C for 24 h. Then, the solvent was evaporated, and DMAHDM was obtained.
The NACP fillers were synthesized by a spray-drying technique, as reported previously (Xu et al., 2011). The final molar ratio of Ca/P was 1.5, the same as that for ACP [Ca3(PO4)2]. The full characterization of the NACP particles, including transmission electron microscopy and X-ray diffraction patterns, was described by previous study (Xu et al., 2011).
Resin Composite Formulations
At a mass ratio of 1:1, bisphenol glycidyl dimethacrylate (BisGMA; Esstech, Essington, PA, United States) and TEGDMA (Esstech, Essington, PA, United States) were mixed (referred to as “BT resin”). 0.2 wt.% camphorquinone, and 0.8 wt.% ethyl 4-N, N- dimethylaminobenzoate photoinitiators were added as photoinitiators. In the base formulation, the resin matrix mass to the fillers was designed at the ratio of 35:65 with BT as a resin matrix, and barium boroaluminosilicate glass particles with a median size of 1.4 μm (Caulk/Dentsply; Milford, DE, United States) silanized with 4 wt.% three- methacryloxypropyltrimethoxysilane as fillers. For the experimental formulations, DMAHDM was introduced to achieve the final mass fractions of 3 wt.% and 5 wt.% in the dental composite with and without 20 wt.% of NACP. All the investigated formulations are described in Table 2.
Plaque-Derived Microcosm Biofilm Assays
Sample Preparation
Circular molds with a diameter of 8 mm and a thickness of 1 mm were used to fabricate resin composite discs. The samples were cured (60 s; 1,000 mW/cm2; VALO Cordless, Ultradent Products, South Jordan, UT, United States) and kept in a dry incubator for 24 h at 37°C followed by 1 h stirring in distilled water at 100 rpm to remove uncured monomers. Ethylene oxide (AnproleneAN 74i; Andersen, Haw River, NC, United States) gas was used to sterilize the samples, followed by seven days of de-gassing to assure the release of entrapped ethylene oxide (Farrugia et al., 2015).
Preparation of the Plaque-Derived Microcosm Assay
A McBain artificial saliva growth medium was prepared as the following: mucin (Type II, porcine, gastric), 2.5 g/L; bacteriological peptone, 2.0 g/L; tryptone, 2.0 g/L; yeast extract, 1.0 g/L; NaCl, 0.35 g/L, KCl, 0.2 g/L; CaCl2, 0.2 g/L; 50 mM pipes, 15 g/L; hemin, 0.001 g/L; vitamin K1, 0.0002 g/L, at pH 7. 0.2% sucrose was added to this medium (McBain et al., 2003). The plaque-brain heart infusion-glycerol solution containing the dispersed bacteria was added into the McBain artificial saliva growth medium to have approximately 1.5 × 106 cells/ml in each well of the 24-well plate (Fontana et al., 2009) containing the resin composite samples.
The plates containing the samples were incubated at 37°C supplemented with 5% CO2. Fresh McBain medium supplemented with 0.2% of sucrose was added after 8 and 24 h of the incubation. At a total of 48 h incubation, the samples were transferred to conduct the CFUs count, metabolic activity assay, lactic acid measurement, or confocal laser scanning microscopy.
Biofilm Disruption Procedure
To quantify bacterial viability in biofilms grown over the composite, each specimen (n = 12) containing 2-day-old microcosm biofilms was washed and transferred to a vial containing 1 ml of cysteine peptone water. The sessile cells were harvested from the composites by sonication (Branson 3510, Branson Ultrasonics Corp., Danbury, CT) (5 min; 1x) followed by two rounds of 30 s vortexing (900 rpm, Vortex-Genie 2, Scientific Inc., Bohemia, NY). The volume of each disrupted biofilm was ten-fold serially diluted using buffered peptone water.
CFU counts of biofilms on resin composites.
The suspensions of the bacterial biofilms (10 µl) were plated in triplicate onto each agar plate using the drop-counting technique. The agar plates were incubated at 37°C in 5% CO2 for 48 h, except for rogosa plates, which were incubated for 96 h. The number of CFU per ml of disrupted biofilm over each disk was determined by counting the colonies grown in the media. The colonies on plates were enumerated using a colony counter (Quebec Darkfield 3328; American Optical Corp, New York, United States). The detection limit with the methods used was 100 CFU/ml of disrupted biofilm. Four solid culture media, including non-selective and selective agar growth media, were used for selective isolation of the cariogenic bacteria (Wan et al., 2002):
(1) Tryptic soy blood agar (TSA) plates to estimate the biofilm growth of the total microorganisms.
(2) Mitis salivarius agar plates containing 15% sucrose to estimate the biofilm growth of total streptococci. Potassium tellurite at 1% was added to the agar to inhibit most gram-negative bacilli and most gram-positive bacteria.
(3) Mitis salivarius agar culture plates with the addition of 0.2 units of bacitracin per ml (MSB) were used to estimate the biofilm growth mutans streptococci.
(4) Rogosa agar culture plates to determine the growth of lactobacilli. Sodium acetate and ammonium citrate at a low pH were added at a high level to allow only the total lactobacilli to grow.
The number of CFU/specimen was calculated and converted to log10 for the statistical analysis.
MTT Assay for Quantification of Metabolic Activity of Biofilms
A colorimetric assay was performed to evaluate the enzymatic reduction of MTT (3-[4,5-dimethylthiazol-2-yl]-2,5-diphenyltetrazolium bromide) as previously described (Melo et al., 2013). Briefly, resin composite samples (n = 4 × 3 replicates) subjected to the 48-h plaque-derived biofilm were washed with phosphate-buffered saline (PBS) and immersed with 1 ml of tetrazolium dye. The plate was incubated at 37°C in 5% CO2 for 1 h. Then, the samples were soaked with 1 ml of dimethyl sulfoxide in the dark for 20 min. The absorbance of the dimethyl sulfoxide at 540 nm was measured using a microplate reader (SpectraMax M5; Molecular Devices, Sunnyvale, CA, United States).
Lactic Acid Production by Biofilms
The lactic acid production was determined via an enzymatic (lactate dehydrogenase) method following a previous study (Balhaddad et al., 2020). Resin composite discs (n = 4 × 3 replicates) subjected to 48 h biofilms were washed with cysteine peptone water solution, immersed with 1.5 ml of buffered-peptone water plus 0.2% sucrose, and incubated for 3 h to allow the biofilms to produce acid. 1 M of glycine and 0.8 M of hydrazine sulfate were mixed at a ratio of 1:1. 170 μl of this mix was placed inside the wells of a 96-well plate followed by 20 μl of 26 mM of ß-nicotinamide adenine dinucleotide and 10 μl of each sample. The microplate reader (SpectraMax M5; Molecular Devices, Sunnyvale, CA, United States) was used to measure the absorbance of the buffered-peptone water solution of each well at 340 nm (optical density OD340) before and after the addition of 10 μl of L-lactic dehydrogenase bovine heart (1,000 units/ml; Sigma-Aldrich, St. Louis, MO, United States). The standard curve was created using a lactic acid standard (Sigma-Aldrich, St. Louis, MO, United States).
Confocal Laser Scanning Microscopy
Confocal laser scanning microscopy was used to visualize the biofilm organization using BacLight live/dead kit (Molecular Probes, Eugene, OR, United States), and the images were three-dimensional reconstructed as previously described (Ibrahim et al., 2020c). Three random areas were selected and scanned using a ×20 air objective. To obtain the Z-series, vertical optical sectioning with a thickness of 1 μm was utilized. The images were processed using ZEN Black 2.3 SP1 (Bitplane, Zürich, Switzerland).
Degree of Conversion
Fourier-transform infrared spectroscopy with attenuated total reflectance device (FTIR-ATR) was used to investigate the DC of the resin composite formulations, as previously described (Collares et al., 2013; Monteiro et al., 2020). Briefly, resin composites (n = 5) were placed inside a mold of polyvinylsiloxane mold over the ATR crystal (Nicolet 6700; Thermo Fisher Scientific, Waltham, MA, United States) to standardize the thickness of each sample (thickness = 1 mm). A polyester strip was used to cover the resin composites. Then, a light-curing tip (VALO Cordless; Ultradent Products, South Jordan, UT, United States) was placed as close as possible to the sample to achieve photoactivation of 20 s with a radiant emittance of 1,000 mW/cm2.
The spectra of each sample were captured before and after the light-curing procedure. OMNIC Series Software (Thermo Fisher Scientific), from 4,000 to 400 cm−1, with 32 scans and 4 cm−1 resolution, was used to evaluate the recorded data. The intensity of the aliphatic carbon-carbon double bond (1,638 cm−1) and the aromatic carbon-carbon double bond (1,608 cm−1) from the resin composite samples were studied before and after the curing to estimate the DC percentage using the equation:
Statistical Analysis
Data normality and distribution were evaluated via the Shapiro-Wilk test. Data were analyzed using one-way ANOVA and Tukey’s post hoc test. All tests were conducted using the statistical software package Sigma Plot 12.0 (SYSTAT, Chicago, IL, United States), and the statistical significance was set at p < 0.05.
Results
Colony-forming Unit Counts of the Plaque-Derived Microcosm Assay
Figure 4 summarizes the CFU results for the plaque-derived microcosm assays. Overall, all the experimental resin composites resulted in a significant bacterial reduction. Figures 4A illustrates the dental plaque-derived microcosm biofilm model and the respective agar media used in the study. Figures 4B displays the percentage of the cariogenic bacterial groups (in Log10) in relation to the total microorganisms grown over the same surface containing DMAHDM and NACP. Compared to the experimental control, incorporating DMAHDM and NACP in the resin composite formulation reduced the prevalence of cariogenic species involved in root caries.
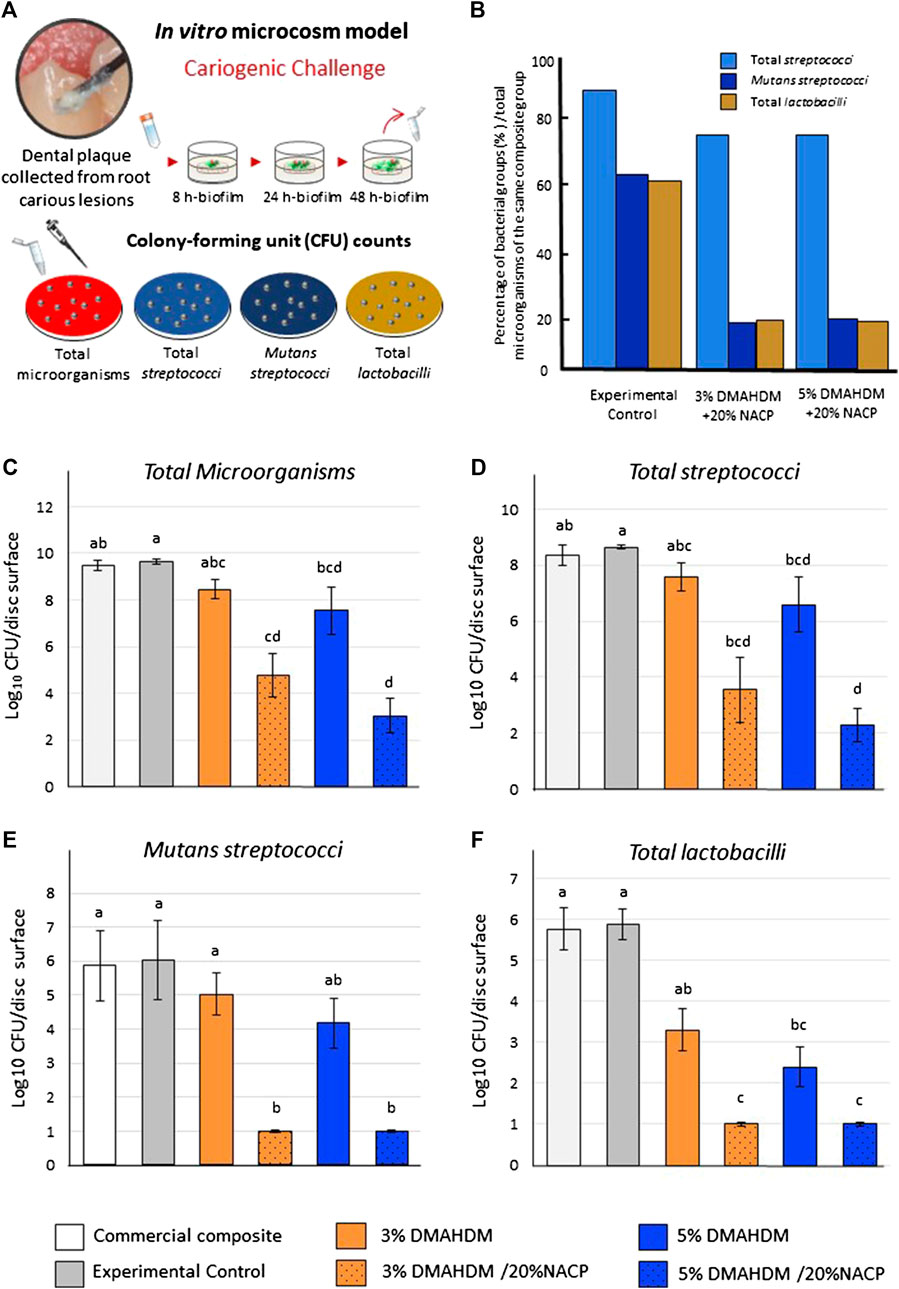
FIGURE 4. Colony-forming unit (CFU) counts for biofilms grown over the resin composite discs. (A) The plaque samples isolated from active root carious lesions were used to initiate plaque-derived microcosm biofilms in vitro. (B) The percentage of the bacterial groups after biofilm inhibition, compared to total microorganisms, quantified over the same resin composite disk. the experimental control. (C) Total microorganisms, (D) total streptococci, (E)mutans streptococci, and (F) total lactobacilli. Values indicated by different letters are statistically different from each other (p < 0.05).
In Figures 4C, the growth of total microorganisms was significantly different among the tested groups (p < 0.001; power of analysis = 100%). The massive amount of reduction was observed with the DMAHDM-NACP resin composites where the CFU counts were reduced by around five and 6.5-log in the 3% DMAHDM-20% NACP and 5% DMAHDM-20% NACP resin composites, respectively, compared to the controls (p < 0.001). For the total streptococci biofilms (Figures 4D), the combinatorial addition of 3 and 5 wt.% of DMAHDM and 20% NACP reduced the growth by approximately 5 to 6-log (p < 0.001; power of analysis = 100%).
Figures 4E displays the counts for mutans streptococci in a selective agar medium. Adding 3 wt.% and 5 wt.% of DMAHDM to the resin composite formulations reduced the growth of mutans streptococci by around 1 and 2-log, respectively. The NACP-DMAHDM resin composites significantly reduced the mutans streptococci biofilms by 5-log compared to the control groups (p < 0.001; power of analysis = 100%). Resin composite with 3 wt.% of DMAHDM inhibited the CFU growth of the total lactobacilli by around 2.5-log, and by increasing the concentration to 5 wt.%, 3.5-log reduction was observed (p < 0.05). The NACP-DMAHDM resin composites significantly inhibited the lactobacilli biofilm growth (p < 0.001; power of analysis = 100%), resulting in 5-log reduction compared to the commercial and experimental controls (Figures 4F).
Metabolic Activities
Resin composites containing 3 wt.% DMAHDM did not significantly reduce the metabolic activities of the biofilms compared to the commercial (0.43 ± 0.14) and experimental (0.31 ± 0.08) control samples (p > 0.05; power of analysis = 100). However, incorporating 5 wt.% DMAHDM into the base formulation resulted in significant inhibition (0.16 ± 0.06) (Figures 5A). Formulations containing 20 wt.% NACP with 3 wt.% or 5 wt.% of DMAHDM significantly reduced the metabolic activities compared to the control groups (p < 0.001).
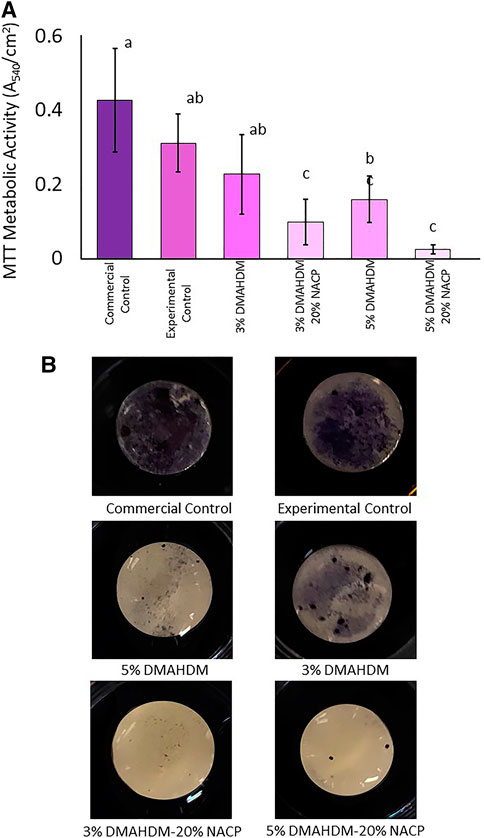
FIGURE 5. (A) Metabolic activities absorbance values, higher absorbance values are associated with higher metabolic activities. Values indicated by different letters are statistically different from each other (p < 0.05). (B) Several resin composite discs showing high adherence of tetrazolium dye to the control groups indicate highly metabolic microorganisms. Resin composite with DMAHDM and NACP were associated with low adherence indicating a lower quantity of highly metabolic microorganisms.
In Figures 5B, commercial and experimental control discs are associated with the most substantial stains indicating a noticeable bond between the tetrazolium dye and a considerable amount of highly metabolic microorganisms. The addition of 3 wt.% and 5 wt.% of DMAHDM resulted in reduced staining. The least amount of staining can be observed among the NACP-DMAHDM resin composites as the surfaces look shiny with a small extent of the stain.
Lactic Acid Production
The concentration of lactic acid production in (mmol/L) was calculated among resin composite samples (Figures 6A). The incorporation of 3 wt.% and 5 wt.% of DMAHDM into the resin composite formulations did not significantly reduce the lactic acid production compared to the controls (p > 0.05). However, the addition of NACP to DMAHDM resulted in a significant reduction (p < 0.001; power of analysis = 100%). In this assay, a commercially obtained lactic acid standard was used to validate the L-lactic dehydrogenase solution and create the standard curve using different lactic acid concentrations. As shown in Figures 5, 6, DMAHDM alone prevented lactic acid production by 36.5–58.5%. However, the combination of NACP and DMAHDM in the resin composite formulations reduced the production by more than 90% compared to the experimental control.
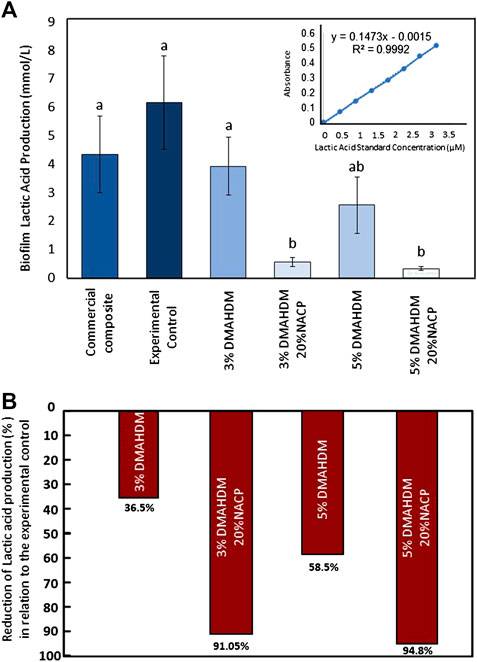
FIGURE 6. (A) Lactic acid production values, higher readings are associated with higher lactic acid production. Values indicated by different letters are statistically different from each other (p < 0.05). (B) The percentages of lactic acid reduction among the experimental groups compared to the experimental control.
Confocal Laser Scanning Microscopy
Representative live/dead images using a confocal microscope are shown in Figures 7A–C. A high visualization of viable microorganisms with thick biofilm was observed over the commercial control resin composite. The incorporation of 3 wt.% and 5 wt.% of DMAHDM resulted in less viable microorganisms. In the 3% DMAHDM-20% NACP samples, a reduced quantification of viable microorganisms and a considerable amount of dead colonies were observed (Figures 7D–F). Almost no viable microorganisms were observed over the 5% DMAHDM-20% NACP resin composites (Figures 7G–I).
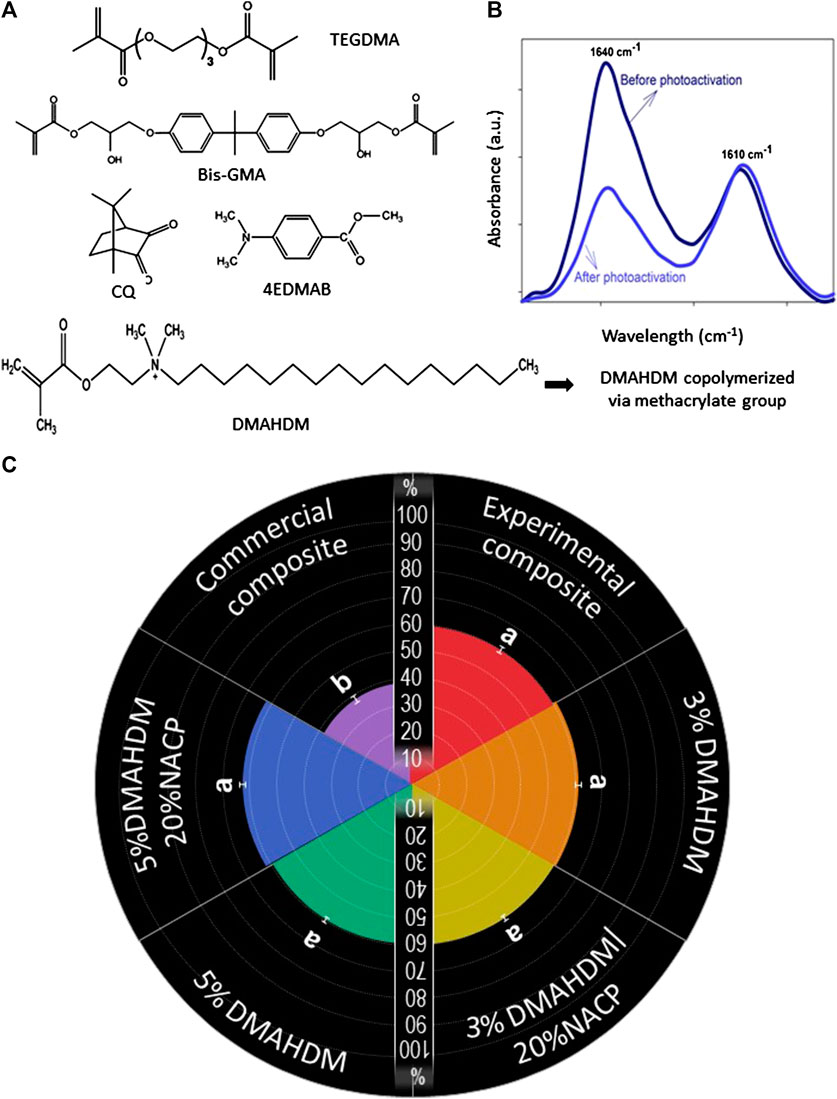
FIGURE 7. (A) The chemical structures of the monomers used in the study to formulate the base resin, the antibacterial monomer, and photoinitiators. (B) A schematic illustration showing the spectra of FTIR of uncured and cured resin, evidencing that the peak of C=C in the aliphatic chain (1,640 cm−1) decreases after the photoactivation. (C) Degree of conversion of the composite resins. Values indicated by different letters are statistically different from each other (p < 0.05).
Degree of Conversion
Figure 8 exhibits the results for the monomer conversion of the formulations. Figures 8A illustrates the chemical composition of the monomers and photoinitiators used in the tested formulations. Figures 8B illustrates the representative image of the FTIR spectra of monomer and polymer during the DC analysis. The DC was determined by the variation of peaks corresponding to aliphatic (1,638 cm−1) and aromatic (1,608 cm−1) C=C bonds of the monomer and polymer. All the experimental formulations demonstrated a higher DC compared to the commercial control (p < 0.001; power of analysis = 100%).
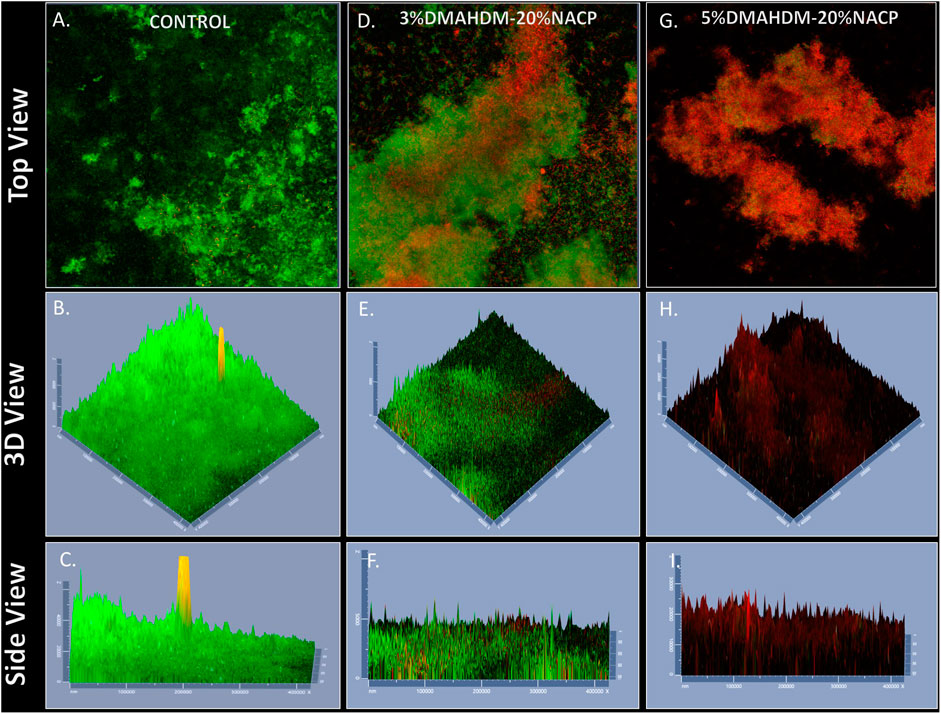
FIGURE 8. Representative live/dead staining images of biofilms using confocal microscopy; (A–C) commercial control, (D–F) 3%DMAHDM-20%NACP%, (G–I) 5%DMAHDM-20%NACP%. Live bacteria were stained green, and compromised bacteria were stained red.
In Figures 8C, the radial chart displays the percentage of monomer conversion for each formulation. The addition of 3% wt.% and 5% wt.% of DMAHDM led to values of 59.9 ± (1.3) % and 60.5 ± (1.2) %. Likewise, the addition of 20% wt.% of NACP to the formulation did not compromise the DC (p= >0.05), as the values were 60.2 ± (1.0)% and 62.3 ± (2.6)% in the 3%DMAHDM-20%NACP and 5%DMAHDM-20%NACP resin composites, respectively.
Discussion
There is currently a great need to develop effective antibacterial dental restorative material that can be used in the clinical setting. Since root caries is a significant and growing oral health concern. It is anticipated that root caries increases in prevalence and severity with the increased rate of aging globally. In this study, we tested the ability of a resin composite formulated with an antibacterial monomer and remineralizing fillers to modulate bacterial pathogens isolated from carious root lesions.
Often, secondary caries around root caries restorations is a burden for patients and a source of frustration for dentists in routine dental practice (Askar et al., 2020). In geriatric patients, the survival rate of composites due to secondary caries is at a low level (Meyer-Lueckel et al., 2019). Patients with prevailing risk factors such as hyposalivation, mental and physical disabilities, and areas of root surface exposure are at a high risk of developing the disease. Considering that the dentin located at the root is more susceptible to caries progression than enamel, managing these lesions at the root surfaces is even more complicated. As a result, the newly developed bioactive resin composites discussed, if successfully translated to the dental clinics, could assist the survival rate of root caries restorations.
In the current work, we assessed for the first time the new dental material using biofilm models that most closely resemble in vivo microbial communities by applying inoculum harvested from root lesions with whole ecosystem diversity. Previous studies have investigated the potential antibiofilm performance using in vitro biofilm models inoculated only with saliva from healthy patients (Balhaddad et al., 2020). Here, we carefully screened patients who presented active carious root lesions to improve microbial representativity of intraoral challenges faced by the restorative material applied on root carious lesions. It is well established that under planktonic growth, individual bacterium behavior dramatically differs from its behavior within complex or multi-species oral biofilms (Fernández et al., 2017).
The assessment of the new bioactive materials may reveal different antibacterial performance, depending on the susceptibilities of the bacteria/biofilm target used for the evaluation (Ibrahim et al., 2020b). The survival mechanisms of clinically relevant microorganisms grown in biofilm inside the mouth are known to be highly resistant to antibacterial therapy delivered by dental products (Sharma et al., 2019). Besides, bacterial species isolated from plaque are quantitatively different from saliva (Georgios et al., 2015; Ruparell et al., 2020). Also, the location where the dental plaque has grown matters. A highly diverse microbial species can be isolated from plaque grown over the lesions compared to other oral niches found inside the mouth (Ruparell et al., 2020). More aciduric and cariogenic species can also be isolated from caries-active individuals than their caries-free counterparts (Georgios et al., 2015; Johansson et al., 2016; Zhou et al., 2016). Highly cariogenic species are often associated with a thicker exopolysaccharide matrix and increased lactic acid production (Wu et al., 2018).
In this study, we have demonstrated the ability of DMAHDM-NACP resin composites to inhibit the growth and activities of plaque-derived microcosm biofilms. The clinically accepted standard of bacterial reduction by an antimicrobial agent is at least a 3-log reduction in cell numbers (Pankey and Sabath, 2004). However, there is no evidence that a somewhat more or less stringent number might not be equally useful in predicting clinical use. Here, the DMAHDM-NACP resin composite reduced the CFUs of highly cariogenic plaque-derived biofilms by around 4-6-log (Figure 4), two to three times higher than what have has reported previously against non-cariogenic inoculum. The DMAHDM-NACP significantly decreased the metabolic activities and lactic acid production compared to DMAHDM solely and control groups (Figures 5, 6).
Additionally, the confocal microscope revealed less and thinner biofilms growing over the DMAHDM-NACP resin composite (Figure 7). The great antibacterial action of our formulations could be attributed to the increased DMAHDM concentration and the use of NACP to convey a multifunctional approach to target dental pathogens. When the DMAHDM concentration was increased up to 5%, an additional reduction was achieved, indicating the ability of DMAHDM to kill pathogens in a dose-dependent manner.
Increasing the DMAHDM concentration up to 5% reveals significant challenges as increasing the concentration may compromise structural stability and low mechanical properties. Our previous report demonstrated the capability of designing and optimizing a resin composite formulation containing 5% DMAHDM and 20% NACP with acceptable mechanical properties, high surface-charge density, and clinically acceptable surface roughness (Balhaddad et al., 2020).
The dimethylaminohexadecyl methacrylate is an antibacterial monomer with a contact-killing mechanism. The positively-charged quaternary ammonium embedded within its structure can interact with microorganisms composed of negatively charged-membrane (Ibrahim et al., 2020c). Short-chain quaternary ammonium induces antibacterial killing solely through the quaternary ammonium by disrupting the bacterial membrane and compromising the balance of some essential ions such as K+, Na+, Ca2+, and Mg2+ (Cheng et al., 2017). Therefore, the antibacterial properties of the synthesized antibacterial monomers are indeed influenced by their alkyl chain length, as suggested by published literature (Li et al., 2013; Ibrahim et al., 2019)
While several other additives were found to impart bioactivity to resin composite formulations, some additives had a disappointing long-term performance for promoting limited or lack of action after depleting ion release (Young, 2010). Opposingly, the use of quaternary ammonium monomers affords a long-lasting effect without leaching or release (Zhang et al., 2018).
In developing bioactive dental materials, the combinatorial approach aims to incorporate agents to target multiple risk factors for disease development. Many researchers, including our group, have sequentially investigated the addition of calcium/fluoride-ions source fillers into an antibacterial resin blend to combine remineralizing and antibacterial actions in one formulation. Several reports support the ability of NACP fillers in resin-based materials to release calcium and phosphate ions under low pH acidic challenge (Melo et al., 2017; Ibrahim et al., 2020a). With a combinatorial approach of antibacterial and bioactivity responses, the resin composite intended to restore root caries lesions could afford different functionality against acidic biofilm attack. The DMAHDM-NACP resin composites could be a promising strategy to assist in preventing secondary caries around root restorations.
Even though we are facing here exciting results, more studies are needed to understand better what signals trigger biofilm development over the dental materials in vivo and to learn how to extrapolate these signals to the in vitro environment (Velsko and Shaddox, 2018). As limitations, many of the influencing factors for the robust growth of patient’s isolates derived biofilms are difficult to control in the laboratory setting (Papadimitriou et al., 2016; Cattò and Cappitelli, 2019). As we have done here, researchers often characterize dental plaque-derived microcosm biofilms and make the assumption that they are representative of the entire microbiome of the root caries. It should also be noted that quantitation of in vitro biofilms suffers from inherent flaws since methodological steps such as sonication that only removes a proportion of bound cells from the composite disk or the sucrose concentration and frequency used in the models can lead to an inadequate translation of microbial profiling (Luo et al., 2015; Williams et al., 2019).
Other expressive findings in this study were the achievement of a suitable percentage of conversion of C=C to C-C in the aliphatic chains during the polymerization reaction for the tested formulations. Studies found that resin composite materials usually accept a DC from 40 to 80% (Noronha Filho et al., 2010). Materials with a low DC are expected to have poor mechanical properties with subsequent high susceptibility to degradation and release of residual unreacted monomers, that may induce toxic reaction to the surrounding tissues and stimulate the biofilm growth (Drummond, 2008; Collares et al., 2013; Maktabi et al., 2018; Fujioka-Kobayashi et al., 2019). Therefore, it is essential when designing a new formulation to assess this chemical property once the monomer conversion can control several properties of the cured material, including mechanical strength, polymerization shrinkage, wear behavior and monomer release (Fujioka-Kobayashi et al., 2019).
In our work, the degrees of conversion for the designed bioactive formulations were comparable to commercially available resin composites. Neither the addition of NACP nor DMAHDM had negatively affected this property. The lack of high rates of uncured monomers may indicate that these formulations are biocompatible and can achieve the required amount of polymerization, enhancing the long-term performance of these restorations. Future studies may involve long-term evaluation of the mechanical and antibacterial properties of the DMAHDM-NACP resin composites. Moreover, investigations on the DMAHDM-NACP composites using a translational model of dental caries can provide evidence of efficacy and the bases for clinical trials.
Conclusion
In summary, we demonstrated, for the first time, a pronounced anti-biofilm performance of DMAHDM-NACP resin composites against plaque-derived microcosm biofilms from carious root lesions. An antibacterial action was imparted in the newly designed bioactive formulations without adversely affecting the monomer conversion. This study presents a practicable strategy to assist in the preventive measures against the onset of secondary caries at the tooth-restoration interface, especially in a highly challenging clinical situation as restoring carious root lesions in high caries-risk patients.
Data Availability Statement
All datasets presented in this study are included in the article/supplementary material.
Ethics Statement
The studies involving human participants were reviewed and approved by The University of Maryland Baltimore Institutional Review Board (HP-00083485). The patients/participants provided their written informed consent to participate in this study.
Author Contributions
AB, MI, FC, and IG contributed to the design and the writing of the manuscript. MW, HX, and MM contributed to the critical review of the manuscript. All authors have read and agreed to the published version of the manuscript.
Funding
This work was supported by the award of the University of Maryland, Baltimore, Institute for Clinical and Translational Research (ICTR) (MM), and the University of Maryland School of Dentistry departmental funds (MM, HX).
Conflicts of Interest
The authors HX and MW have patents (US20150299345A1; US8889196B2) for the manufacture and use of antibacterial monomers and amorphous calcium phosphate used in this study. The funders had no role in the design of the study; nanoparticles in the collection, analyses, or interpretation of data; in the writing of the manuscript, or in the decision to publish the results.
Acknowledgments
The authors are grateful to the volunteers for their participation in this study. The authors thank the faculty and staff of the Department of General Dentistry at the University of Maryland School of Dentistry for their assistance and use of their clinical facilities. We are grateful to Esstech (Essington, PA) for kindly donating the BisGMA and TEGDMA monomers. MM acknowledges the award support of the University of Maryland, Baltimore, Institute for Clinical & Translational Research (ICTR); the Research Center for Innovative Biomedical Resources (CIBR)-Confocal Microscopy Facility on behalf Mauban for the assistance with confocal microscopy. AB and MI acknowledge the scholarship during their Ph.D. studies from the Imam AbdulRahman bin Faisal University, Dammam, Saudi Arabia, and the Saudi Arabia Cultural Mission. IG acknowledge the scholarship during their Ph.D. studies from the Coordenação de Aperfeiçoamento de Pessoal de Nível Superior - Brasil (CAPES) - Finance Code 001 – scholarship.
References
Askar, H., Krois, J., Göstemeyer, G., Bottenberg, P., Zero, D., Banerjee, A., et al. (2020). Secondary caries: what is it, and how it can be controlled, detected, and managed? Clin Oral Invest. 24, 1869–1876. doi:10.1007/s00784-020-03268-7
Bourbia, M., and Finer, Y. (2018). Biochemical stability and interactions of dental resin composites and adhesives with host and bacteria in the oral cavity: a review. J. Can. Dent. Assoc. 84, i1
Cattò, C., and Cappitelli, F. (2019). Testing anti-biofilm polymeric surfaces: where to start? Int. J. Mol. Sci. 20, 2–60. doi:10.3390/ijms20153794
Cheng, L., Weir, M. D., Zhang, K., Xu, S. M., Chen, Q., Zhou, X., et al. (2012). Antibacterial nanocomposite with calcium phosphate and quaternary ammonium. J. Dent. Res. 91, 460–466. doi:10.1177/0022034512440579
Cheng, L., Zhang, K., Zhang, N., Melo, M. A. S., Weir, M. D., Zhou, X. D., et al. (2017). Developing a new generation of antimicrobial and bioactive dental resins. J. Dent. Res. 96, 855–863. doi:10.1177/0022034517709739
Collares, F. M., Portella, F. F., Leitune, V. C. B., and Samuel, S. M. W. (2013). Discrepancies in degree of conversion measurements by FTIR. Braz. Oral Res. 27, 453–454. doi:10.1590/S1806-83242013000600002
Damé-Teixeira, N., Parolo, C. C. F., and Maltz, M. (2017). Specificities of caries on root surface. Monogr. Oral Sci. 26, 15–25. doi:10.1159/000479303
Delaviz, Y., Finer, Y., and Santerre, J. P. (2014). Biodegradation of resin composites and adhesives by oral bacteria and saliva: a rationale for new material designs that consider the clinical environment and treatment challenges. Dent. Mater. 30, 16–32. doi:10.1016/j.dental.2013.08.201
Drummond, J. L. (2008). Degradation, fatigue, and failure of resin dental composite materials. J. Dent. Res. 87, 710–719. doi:10.1177/154405910808700802
Farrugia, C., Cassar, G., Valdramidis, V., and Camilleri, J. (2015). Effect of sterilization techniques prior to antimicrobial testing on physical properties of dental restorative materials. J. Dent. 43, 703–714. doi:10.1016/j.jdent.2015.03.012
Fernández, C. E., Aspiras, M. B., Dodds, M. W., González-Cabezas, C., and Rickard, A. H. (2017). The effect of inoculum source and fluid shear force on the development of in vitro oral multispecies biofilms. J. Appl. Microbiol. 122, 796–808. doi:10.1111/jam.13376
Fontana, C. R., Abernethy, A. D., Som, S., Ruggiero, K., Doucette, S., Marcantonio, R. C., et al. (2009). The antibacterial effect of photodynamic therapy in dental plaque-derived biofilms. J. Periodont. Res. 44, 751–759. doi:10.1111/j.1600-0765.2008.01187.x
Fujioka-Kobayashi, M., Miron, R. J., Lussi, A., Gruber, R., Ilie, N., Price, R. B., et al. (2019). Effect of the degree of conversion of resin-based composites on cytotoxicity, cell attachment, and gene expression. Dent. Mater. 35, 1173–1193. doi:10.1016/j.dental.2019.05.015
Gati, D., and Vieira, A. R. (2011). Elderly at greater risk for root caries: a look at the multifactorial risks with emphasis on genetics susceptibility. Int J Dent. 2011, 647168. doi:10.1155/2011/647168
Gavriilidou, N. N., and Belibasakis, G. N. (2019). Root caries: the intersection between periodontal disease and dental caries in the course of ageing. Br. Dent. J. 227, 1063–1067. doi:10.1038/s41415-019-0973-4
Georgios, A., Vassiliki, T., and Sotirios, K. (2015). Acidogenicity and acidurance of dental plaque and saliva sediment from adults in relation to caries activity and chlorhexidine exposure. J. Oral Microbiol. 7, 26197. doi:10.3402/jom.v7.26197
Hayes, M., Burke, F., and Allen, P. F. (2017a). Incidence, prevalence and global distribution of root caries. Monogr. Oral Sci. 26, 1–8. doi:10.1159/000479301
Hayes, M., Da Mata, C., McKenna, G., Burke, F. M., and Allen, P. F. (2017b). Evaluation of the Cariogram for root caries prediction. J. Dent. 62, 25–30. doi:10.1016/j.jdent.2017.04.010
Ibrahim, M. S., Balhaddad, A. A., Garcia, I. M., Collares, F. M., Weir, M. D., Xu, H. H. K., et al. (2020a). pH-responsive calcium and phosphate-ion releasing antibacterial sealants on carious enamel lesions in vitro. J. Dent. 97, 103323. doi:10.1016/j.jdent.2020.103323
Ibrahim, M. S., Garcia, I. M., Kensara, A., Balhaddad, A., Collares, F. M., Williams, M. A., et al. (2020b). How we are assessing the developing antibacterial resin-based dental materials? A scoping review. J. Dent. 99, 103369. doi:10.1016/j.jdent.2020.103369
Ibrahim, M. S., Garcia, I. M., Vila, T., Balhaddad, A. A., Collares, F. M., Weir, M. D., et al. (2020c). Multifunctional antibacterial dental sealants suppress biofilms derived from children at high risk of caries. Biomater. Sci. 8 (Suppl. 18). doi:10.1039/D0BM00370K
Ibrahim, M. S., Ibrahim, A. S., Balhaddad, A. A., Weir, M. D., Lin, N. J., Tay, F. R., et al. (2019). A novel dental sealant containing dimethylaminohexadecyl methacrylate suppresses the cariogenic pathogenicity of Streptococcus mutans biofilms. Int. J. Mol. Sci. 20 (14), 3491. doi:10.3390/ijms20143491
Johansson, I., Witkowska, E., Kaveh, B., Lif Holgerson, P., and Tanner, A. C. R. (2016). The microbiome in populations with a low and high prevalence of caries. J. Dent. Res. 95, 80–86. doi:10.1177/0022034515609554
Khatoon, Z., McTiernan, C. D., Suuronen, E. J., Mah, T.-F., and Alarcon, E. I. (2018). Bacterial biofilm formation on implantable devices and approaches to its treatment and prevention. Heliyon. 4, e01067. doi:10.1016/j.heliyon.2018.e01067
Li, F., Weir, M. D., and Xu, H. H. K. (2013). Effects of quaternary ammonium chain length on antibacterial bonding agents. J. Dent. Res. 92, 932–938. doi:10.1177/0022034513502053
Liang, X., Söderling, E., Liu, F., He, J., Lassila, L. V. J., and Vallittu, P. K. (2014). Optimizing the concentration of quaternary ammonium dimethacrylate monomer in bis-GMA/TEGDMA dental resin system for antibacterial activity and mechanical properties. J. Mater. Sci. Mater. Med. 25, 1387–1393. doi:10.1007/s10856-014-5156-x
Luo, X., Jellison, K. L., Huynh, K., and Widmer, G. (2015). Impact of bioreactor environment and recovery method on the profile of bacterial populations from water distribution systems. PloS One. 10, e0133427. doi:10.1371/journal.pone.0133427.
Maktabi, H., Balhaddad, A. A., Alkhubaizi, Q., Strassler, H., and Melo, M. A. S. (2018). Factors influencing success of radiant exposure in light-curing posterior dental composite in the clinical setting. Am. J. Dent. 31, 320–328
Marsh, P. D. (2006). Dental plaque as a biofilm and a microbial community – implications for health and disease. BMC Oral Health. 6, S14. doi:10.1186/1472-6831-6-S1-S14.
McBain, A. J., Bartolo, R. G., Catrenich, C. E., Charbonneau, D., Ledder, R. G., and Gilbert, P. (2003). Growth and molecular characterization of dental plaque microcosms. J. Appl. Microbiol. 94, 655–664. doi:10.1046/j.1365-2672.2003.01876.x.
Melo, M. A. S., Cheng, L., Zhang, K., Weir, M. D., Rodrigues, L. K. A., and Xu, H. H. K. (2013). Novel dental adhesives containing nanoparticles of silver and amorphous calcium phosphate. Dent. Mater. 29, 199–210. doi:10.1016/j.dental.2012.10.005.
Melo, M. A. S., Weir, M. D., Passos, V. F., Powers, M., and Xu, H. H. K. (2017). Ph-activated nano-amorphous calcium phosphate-based cement to reduce dental enamel demineralization. Artif. Cells Nanomed. Biotechnol. 45, 1778–1785. doi:10.1080/21691401.2017.1290644.
Melo, M. A. S., Weir, M. D., Passos, V. F., Rolim, J. P. M., Lynch, C. D., Rodrigues, L. K. A., et al. (2018). Human in situ study of the effect of bis(2-methacryloyloxyethyl) dimethylammonium bromide immobilized in dental composite on controlling mature cariogenic biofilm. Int. J. Mol. Sci. 19 (11), 3443. doi:10.3390/ijms19113443
Meyer-Lueckel, H., Machiulskiene, V., and Giacaman, R. A. (2019). How to intervene in the root caries process? Systematic review and meta-analyses. Caries Res. 53, 599–608. doi:10.1159/000501588
Monteiro, J. C., Stürmer, M., Garcia, I. M., Melo, M. A., Sauro, S., Leitune, V. C. B., et al. (2020). Dental sealant empowered by 1,3,5-tri acryloyl hexahydro-1,3,5-triazine and α-tricalcium phosphate for anti-caries application. Polymers (Basel). 12 (4), 895. doi:10.3390/polym12040895
Noronha Filho, J. D., Brandão, N. L., Poskus, L. T., Guimarães, J. G. A., and Silva, E. M. (2010). A critical analysis of the degree of conversion of resin-based luting cements. J. Appl. Oral Sci. 18, 442–446. doi:10.1590/s1678-77572010000500003
Pankey, G. A., and Sabath, L. D. (2004). Clinical relevance of bacteriostatic versus bactericidal mechanisms of action in the treatment of Gram-positive bacterial infections. Clin. Infect. Dis. 38, 864–870. doi:10.1086/381972
Papadimitriou, K., Alegría, Á., Bron, P. A., de Angelis, M., Gobbetti, M., Kleerebezem, M., et al. (2016). Stress physiology of lactic acid bacteria. Microbiol. Mol. Biol. Rev. 80, 837–890. doi:10.1128/MMBR.00076-15
Ritter, A. V., Shugars, D. A., and Bader, J. D. (2010). Root caries risk indicators: a systematic review of risk models. Community Dent. Oral Epidemiol. 38, 383–397. doi:10.1111/j.1600-0528.2010.00551.x
Ruparell, A., Inui, T., Staunton, R., Wallis, C., Deusch, O., and Holcombe, L. J. (2020). The canine oral microbiome: variation in bacterial populations across different niches. BMC Microbiol. 20, 42. doi:10.1186/s12866-020-1704-3
Sharma, D., Misba, L., and Khan, A. U. (2019). Antibiotics versus biofilm: an emerging battleground in microbial communities. Antimicrob. Resist. Infect. Contr. 8, 76. doi:10.1186/s13756-019-0533-3
Signori, C., van de Sande, F. H., Maske, T. T., de Oliveira, E. F., and Cenci, M. S. (2016). Influence of the inoculum source on the cariogenicity of in vitro microcosm biofilms. Caries Res. 50, 97–103. doi:10.1159/000443537
Takahashi, N., and Nyvad, B. (2016). Ecological hypothesis of dentin and root caries. Caries Res. 50, 422–431. doi:10.1159/000447309 | Google Scholar
Tan, H., Richards, L., Walsh, T., Worthington, H. V., Clarkson, J. E., Wang, L., et al. (2017). Interventions for managing root caries. Cochrane Database Syst. Rev. 2017 (8). doi:10.1002/14651858.CD012750
The United States census bureau (2019). By 2030, All baby boomers will Be age 65 or older. Available at: https://www.census.gov/library/stories/2019/12/by-2030-all-baby-boomers-will-be-age-65-or-older.html (Accessed June 1, 2020).
Tonetti, M. S., Bottenberg, P., Conrads, G., Eickholz, P., Heasman, P., Huysmans, M.-C., et al. (2017). Dental caries and periodontal diseases in the ageing population: call to action to protect and enhance oral health and well-being as an essential component of healthy ageing - consensus report of group 4 of the joint EFP/ORCA workshop on the boundaries between caries and periodontal diseases. J. Clin. Periodontol. 44 (Suppl. 18), S135–S144. doi:10.1111/jcpe.12681
Vandana, K. L., and Haneet, R. K. (2014). Cementoenamel junction: an insight. J. Indian Soc. Periodontol. 18, 549–554. doi:10.4103/0972-124X.142437
Velsko, I. M., and Shaddox, L. M. (2018). Consistent and reproducible long-term in vitro growth of health and disease-associated oral subgingival biofilms. BMC Microbiol. 18 (1), 70. doi:10.1186/s12866-018-1212-x
Wan, A. K. L., Seow, W. K., Walsh, L. J., and Bird, P. S. (2002). Comparison of five selective media for the growth and enumeration of Streptococcus mutans. Aust. Dent. J. 47, 21–26. doi:10.1111/j.1834-7819.2002.tb00298.x
Weir, M. D., Moreau, J. L., Levine, E. D., Strassler, H. D., Chow, L. C., and Xu, H. H. K. (2012). Nanocomposite containing CaF2 nanoparticles: thermal cycling, wear and long-term water-aging. Dent. Mater. 28, 642–652. doi:10.1016/j.dental.2012.02.007
Williams, D. L., Smith, S. R., Peterson, B. R., Allyn, G., Cadenas, L., Epperson, R. T., et al. (2019). Growth substrate may influence biofilm susceptibility to antibiotics. PLoS One. 14, e0206774. doi:10.1371/journal.pone.0206774
Wu, H., Zeng, B., Li, B., Ren, B., Zhao, J., Li, M., et al. (2018). Research on oral microbiota of monozygotic twins with discordant caries experience - in vitro and in vivo study. Sci. Rep. 8, 7267. doi:10.1038/s41598-018-25636-w
Young, A. M. (2010). “11 - antibacterial releasing dental restorative materials,” in Drug-device combination products woodhead publishing series in biomaterials. Editos Lewis, A. (Cambridge, UK: Woodhead Publishing), 246–279
Yuan, Y., Sun, F., Zhang, F., Ren, H., Guo, M., Cai, K., et al. (2013). Targeted synthesis of porous aromatic frameworks and their composites for versatile, facile, efficacious, and durable antibacterial polymer coatings. Adv. Mater. 25, 6619–6624. doi:10.1002/adma.201301955
Zhang, N., Zhang, K., Xie, X., Dai, Z., Zhao, Z., Imazato, S., et al. (2018). Nanostructured polymeric materials with protein-repellent and anti-caries properties for dental applications. Nanomaterials (Basel). 8 (6),393. doi:10.3390/nano8060393
Zhou, C., Weir, M. D., Zhang, K., Deng, D., Cheng, L., and Xu, H. H. K. (2013). Synthesis of new antibacterial quaternary ammonium monomer for incorporation into CaP nanocomposite. Dent. Mater. 29, 859–870. doi:10.1016/j.dental.2013.05.005
Zhou, J., Jiang, N., Wang, S., Hu, X., Jiao, K., He, X., et al. (2016). Exploration of human salivary microbiomes--insights into the novel characteristics of microbial community structure in caries and caries-free subjects. PLoS One. 11, e0147039. doi:10.1371/journal.pone.0147039
Keywords: amorphous calcium phosphate, antibacterial agents, dental caries, polymerization, quaternary ammonium compounds, Amorphous calcium, Biomaterials, Antibacterial
Citation: Balhaddad AA, Ibrahim MS, Garcia IM, Collares FM, Weir MD, Xu HH and Melo MAS (2020) Pronounced Effect of Antibacterial Bioactive Dental Composite on Microcosm Biofilms Derived From Patients With Root Carious Lesions. Front. Mater. 7:583861. doi: 10.3389/fmats.2020.583861
Received: 15 July 2020; Accepted: 28 October 2020;
Published: 23 November 2020.
Edited by:
Hae-Won Kim, Institute of Tissue Regeneration Engineering (ITREN), South KoreaReviewed by:
Sergey V. Dorozhkin, Independent Researcher, Moscow, RussiaJoanna Mystkowska, Bialystok University of Technology, Poland
Copyright © 2020 Balhaddad, Ibrahim, Garcia, Collares, Weir, Xu and Melo. This is an open-access article distributed under the terms of the Creative Commons Attribution License (CC BY). The use, distribution or reproduction in other forums is permitted, provided the original author(s) and the copyright owner(s) are credited and that the original publication in this journal is cited, in accordance with accepted academic practice. No use, distribution or reproduction is permitted which does not comply with these terms.
*Correspondence: Mary Anne S. Melo, bW1lbG9AdW1hcnlsYW5kLmVkdQ==; Hockin H. Xu, SFh1QHVtYXJ5bGFuZC5lZHU=