- Electric Power Science Research Institute of Yunnan Power Grid Co., Ltd., Kunming, China
MoS2 has been considered a potential novel material in various fields due to its large specific surface area, high carrier mobility, and tunable electronic properties. However, with the increasing demand for sensor substrates, different strategies have been made to achieve its high performance, usually by adopting the method of controlling the microstructure. SF6 gas-insulated electrical equipment has gained considerable attention in electric systems with the advantages of small volume, high security, strong breaking performance, and high-pressure fracture tolerance. Nevertheless, in the process of equipment operation, the SF6 gas will occur inevitably decompose due to partial discharge, resulting in the deterioration of the insulation performance of the equipment. Therefore, detecting SF6 decomposition products is significant for the safe and stable operation of power systems. In this mini review, we start from the synthesis of various MoS2 morphological structures. Then, the beneficial characteristics of the unique synthesized nanostructures at present are analyzed. Besides, we focus on the gas-sensing mechanisms and applications of MoS2-based sensors for detecting SF6 decomposition products. Finally, the future development in this field is proposed.
Introduction
Sulfur hexafluoride (SF6) is a non-color, tasteless, and non-flammable insulating gas, which is widely known for applications in gas insulated switchgear (GIS) (Lu et al., 2018; Lu et al., 2019). Although GIS has advantage of high stability, insulation faults such as partial discharge, breakdown discharge, and spark discharge inevitably occur during a long running process (Zeng et al., 2015; Liu et al., 2017), which will lead to the decomposition of the SF6 gas into various sulfur fluorides, including H2S, SO2, SO2F2, and SOF2 etc. (Wei et al., 2020). Previous research has indicated that these characteristic gases can accelerate the corrosion rate of facilities and increase the probability of system paralysis (Zhang X. X. et al., 2017a; Zhou et al., 2018b). Therefore, it is of great necessity to evaluate the operational status of GIS equipment by effectively detecting these typically decomposed products of SF6 (Zhang X. X. et al., 2017b; Zhou et al., 2018d). In this respect, bidimensional nanomaterials have captured widespread attention for its multiple physical and chemical properties in the detection of SF6 decomposed gas (Zhou et al., 2018a; Chen et al., 2018).
Given this, various 2-D nanomaterials such as carbon nanotube (CNT), graphene, and molybdenum disulfide (MoS2) have been synthesized by different methods (Zhou et al., 2018e; Choi et al., 2020). As an n-type semiconductor material with wide band gap, MoS2 receives the most interest because of its high surface activity and chemical stability (Fan et al., 2017; Zhang et al., 2019). Up to now, a large number of studies have been carried out on the various nanostructures of MoS2, including nanoflakes (Johari et al., 2020), nanotubes (Zhong et al., 2020), nanospheres (Li Y. X. et al., 2019) and other complex hierarchical nanostructures (Zhang et al., 2018b; Agrawal et al., 2020), to realize more effective methods of detecting SF6 decomposed products. Besides, the evident correlation has been confirmed between unique structures and performances (Barzegar et al., 2019; Wang et al., 2020). Therefore, the morphology synthesis and analysis of MoS2 nanostructures are of great significant to discuss. This mini review summarizes the morphological features and sensing applications of MoS2, especially for detecting SF6 decomposed products.
Morphology and analysis of MoS2
MoS2 is deemed as a viable and effective material owing to its stable semiconducting property and high thermal stability (Sahoo et al., 2016; Sangeetha and Madhan, 2020). Until now, the diverse morphology of MoS2 nanostructures have been designed by investigators through various methods to achieve excellent sensing properties in the aspects of chemical, optical, and gas sensors (Zhang et al., 2018a; Bhakhar et al., 2019). Kang et al. demonstrated vertical MoS2 nanoflakes (shown in Figure 1A) fabricated on SiO2/Si substrates by the deposition and thermal evaporation. They found the vertical flakes supplied an effective surface area and sufficient oxygen vacancies for the adsorption of NO2 gas. The minimum concentration of the NO2 detection was 0.15 ppm at room temperature (Kang et al., 2017). Tu et al. synthesized hierarchical MoS2 spheres with flower-like structures (shown in Figure 1B) presented efficient aluminum storage properties. These flower-like microstructures for aluminum storage possessed open and well-defined hierarchical structures, leading to a higher specific capacity and prominent cycling stability. The as-prepared MoS2 electrodes delivered reversible capacities of 112.2 mAh g−1 at 153.6 mAh g−1 after 100 cycles (Tu et al., 2019).
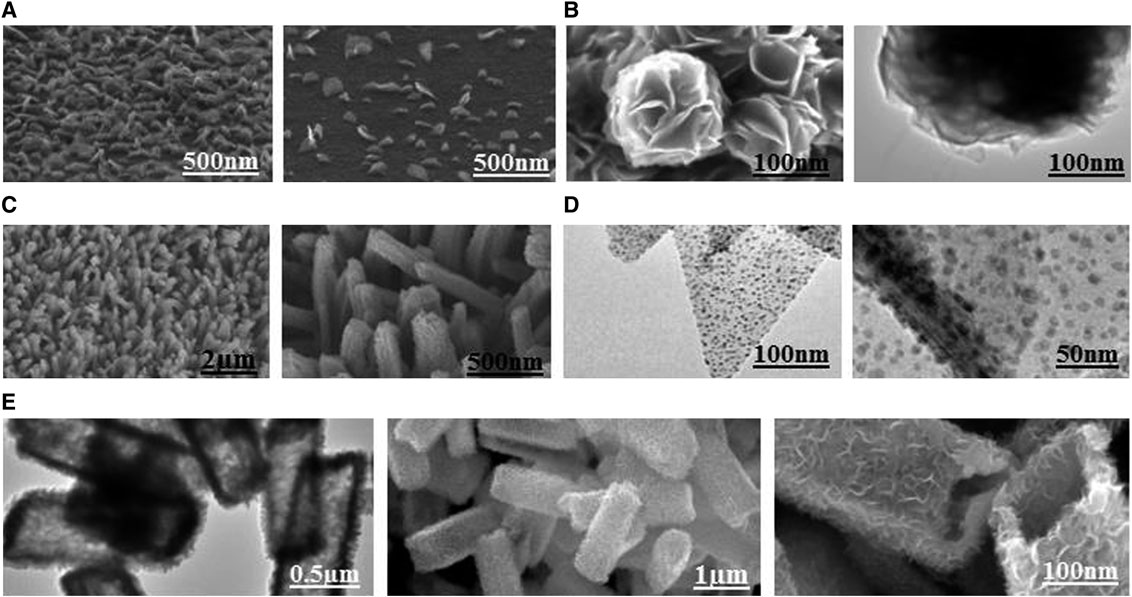
FIGURE 1. MoS2 with different hierarchical structures: (A) Vertical nanoflakes. Reprinted with permission from Kang et al. (2017). Copyright (2017) American Chemical Society. (B) Flower-like spheres. Reprinted with permission from Tu et al. (2019). Copyright (2019) American Chemical Society. (C) Nanorods. Reprinted with permission from Sun et al. (2018). Copyright (2018) American Chemical Society. (D) Porous film. Reprinted with permission from Li et al. (2019). Copyright (2019) American Chemical Society. (E) Porous hollow rhomboids. Reprinted with permission from Han et al. (2020). Copyright (2020) American Chemical Society.
Sun et al. developed MoS2 nanorods (shown in Figure 1C) by the hydrothermal and ammonia annealing approach and found that the fabricated nanorods exhibited excellent catalytic performances due to a more specific surface area and a sufficient ion transportation channel (Sun et al., 2018). Li et al. fabricated a three-dimensional porous MoS2 film of surface-grown Pt nanocrystals (shown in Figure 1D) via the CVD-TA method, and found that higher Pt loading yields improved the performances of the hydrogen evolution reaction with a smaller specific surface area. Besides, these special porous constructions could effectively solve the stacking and agglomeration problems owing to the electrochemical reaction, guarding remarkable cycling stability (Li et al., 2019). Han et al. reported electrode material performance of hierarchically porous MoS2-Carbon hollow rhomboids (shown in Figure 1E) prepared by a self-templated solvothermal reaction. The as-prepared electrodes delivered reversible capacities of 506 mAh g−1 at 0.1 A g−1 after 3,000 cycles. The authors ascribed the excellent storage energy properties of MoS2-C rhomboids structures to the distinct internal void structure (Han et al., 2020).
MoS2 sensor for SF6 decomposing products
Theoretical calculations about MoS2-based sensors
In order to analyze and investigate the adsorption process between SF6 decomposed gases and MoS2-based material, corresponding adsorbing factors containing total adsorption energies (
where
The adsorption procedures between gas sensing materials and SF6 decomposing products were studied based on the density functional theory (DFT) (Singh et al., 2018; Cui et al., 2019). For instance, Abbasi et al. studied the adsorptions properties of SO2 molecules on MoS2 monolayers in the aspects of charge transfer, band structures, adsorption energy, and charge density differences. They found that SO2 gas molecules were adsorbed on the surface monolayer by physisorption. The S-O bonds of the adsorption gas molecules were lengthened after the adsorbed process. Furthermore, elongation of the bond lengths was mainly owed to the transfer of charge density from the original to the new bonds between the MoS2 monolayers and target gas molecules, and the adsorption of SO2 changed the electronic properties of MoS2 monolayer (Abbasi and Sardroodi 2019). Chen et al. theoretically discussed the adsorption behavior of five types of SF6 decomposing products (H2S, HF, SO2, SOF2, and SO2F2) on the MoS2 monolayer by employing the NEGF combined with DFT method. Among the five types of gas molecules, MoS2 monolayer had the best adsorption performance for SO2 caused by the special electronegativity (Chen et al., 2019a).
In another instance, Qian et al. researched the adsorption property of H2S and SO2 on the Pt-decorated MoS2 and found that Pt-MoS2 showed a strong interaction with gas molecules due to the strong chemical activity of with Pt atom. The adsorption energy of H2S and SO2 molecules adsorbed on Pt-MoS2 was −1.465 and −1.584 eV, respectively, suggesting the occurrence of strong chemisorption in the contact surfaces. The charge transfer amount between H2S and Pt-MoS2 was up to 0.302 e, which is much higher than that of SO2 (0.036 e). The results indicated that Pt-MoS2 exhibited an excellent adsorption property for H2S gas (Qian et al., 2019). Wei et al. reported the response behavior of Ni-MoS2 towards SF6 typical decomposition products: SO2 and H2S. The different adsorption sites for the SO2 and H2S on Ni-MoS2 surface were designed to find the site of optimal adsorption energy. The result of SO2 and H2S was −1.382 and −1.319 eV, respectively, indicating the strong chemical interaction between the Ni-doped MoS2 monolayer and two kinds of gases (Wei et al., 2018). Gui et al. reported that Si-doped MoS2 were used to investigate its adsorptive properties to H2S and SOF2, and found that the most stable doping site were above the S atom. The adsorption energy was −0.68 eV when H2S adsorbed on Si-MoS2, while pristine MoS2 was only −0.17 eV. The adsorption energy of SOF2 adsorbed on Si-MoS2 reached −3.63 eV, which was much higher than the −0.01 eV of the pristine MoS2 adsorption system. The results provided that the adsorption capacities of pristine MoS2 for H2S and SOF2 gases could be greatly improved by doping them with a nonmetallic atom (Gui et al. 2019). Li et al. selected the Pd atom as the dopant to modify the surface of the MoS2 monolayer in the latest study, and an adsorbent with higher properties for the adsorption of SOF2 and SO2F2 gases was obtained on this basis. In addition, the double gas molecules could still be stably adsorbed on the surface of Pd-MoS2, indicating the feasibility of the adsorbent (Li et al. 2020).
In the above, the adsorption of H2S and SO2 molecules in different systems have been discussed in detail. The simulation results of H2S and SO2 molecules adsorption are in Table 1. These values may be obtained by different DFT functions. Compared with the adsorption construction of a pristine MoS2 system, the introduction of novel elements promotes the surface chemical activity of the MoS2 system. In addition, the addition of dopants enhances the affect of orbital hybridization between the MoS2 monolayer and gas molecules, and facilitates the electronic transfer. The adsorption capacity of the modified materials to the goal gas will be further improved, when the dopant is suitable (Zhang et al., 2017a).
Experimental analysis about MoS2-based sensors
At present, there are few experimental studies on the detection of SF6 decomposed byproducts, the few that do mainly focus on the H2S, SO2, and CO gases (Wang et al., 2019a; Zhou et al., 2019). Park et al. prepared a MoS2 gas sensor modified with Pt nanoparticles with a low detection limit and high sensitivity for H2S gas. The Pt-MoS2-based sensor reduced the minimum detection concentration of H2S gas to 5 ppm, which was much lower than that of the pure MoS2 sensor (30 ppm) (Park et al., 2018). The Pt-MoS2 film structures were formed by the introduction of Pt nanoparticles with ultra-small diameters, which could distinctly improve the performance of sensors. The Ni-doped MoS2 nanoflower synthesized by Zhang et al. displayed a faster and higher response to 5 ppm SO2 gas (Ra/Rg = 14.75) at room temperature. The main reason was that the richer porosity in the nanoflower structures provided sufficient adsorption sites for SO2 gas (Zhang et al., 2017b). Hierarchical MoS2 nanospheres assembled from nanosheets fabricated by Zhou et al. exhibited high properties to CO. The response value of the gas sensor to 500 ppm CO reached 92.6 at 230°C. Besides, this sensor had almost no response to other gases when the operation temperature was higher than 200°C, indicating the outstanding selectivity toward CO gas (Zhou et al., 2018c). The experimental results show that these unique nanostructures can enhance the gas-sensing performance, which is attributed to the increase of oxygen vacancies and the promotion of gas adsorption-desorption efficiency. Although some advancements have been made in the detection of SF6 decomposed products, more high-accuracy experiments are needed for other decomposition gases such as SOF2 and SO2F2.
Gas sensing mechanism
The gas sensing mechanism of the MoS2-based sensors to SF6 decomposed products can be explained by the change of conductivity caused by the interaction between oxyanions (mainly O-) on the material surface and target gas molecules. Firstly, the oxygen molecules in air will adsorb on the surface of semiconductor materials and capture electrons from the adsorption sites to form oxyanions. The formation of an electron depletion layer and the increase of resistance are caused by oxygen adsorption. Then, when sensors are placed in an environment of reductive gas, the test gas molecules react with oxyanions to release the electrons back to the surface of the material, resulting in the decrease of the electron depletion layer and the occurrence of conductivity change. The relevant reactions are depicted as follows (take H2S gas as an example):
Conclusion
In this paper, we focused on the morphological characteristics and application of MoS2 materials for the detection of SF6 decomposed products in GIS. Firstly, partial reports of sensing properties of MoS2 with various morphologies were concluded. Diverse MoS2 structures presented relatively different sensing properties at specific operating temperatures, so it was possible to promote sensing performances of MoS2 by simple surface morphology modification. With the increasing demand for sensor materials, simples and more convenient synthetic routes and more favorable morphology structures should be proposed. Next, it was shown that the selectivity and sensitivity of MoS2 gas sensors could also be enhanced by the enlargement of the active surface area and the introduction of metal or non-metal elements based on formerly theoretical and experimental investigations. Then, the gas-sensing mechanism of the MoS2 based sensors to SF6 decomposed products was described by comparing the characteristics of material substrate and gas molecules. Although some developments have been obtained in theoretical research into SF6 decomposed products, a large number of fundamental experiments are needed to further prepare MoS2 sensors for practical industrial applications.
Conflict of Interest
Authors GQ, QP, DZ, SW, and BY were employed by the Electric Power Science Research Institute of Yunnan Power Grid Co., Ltd.
Data Availability Statement
The raw data supporting the conclusions of this article will be made available by the authors, without undue reservation, to any qualified researcher.
Author Contributions
All authors listed have made a substantial, direct, and intellectual contribution to the work, and approved it for publication.
References
Abbasi, A., and Sardroodi, J. J. (2019). Adsorption of O3, SO2 and SO3 gas molecules on MoS2 monolayers: a computational investigation. Appl. Surf. Sci. 469, 781–791. doi:10.1016/j.apsusc.2018.11.039.
Agrawal, A. V., Kumar, R., Yang, G., Bao, J. M., Kumar, M., and Kumar, M. (2020). Enhanced adsorption sites in monolayer MoS2 pyramid structures for highly sensitive and fast hydrogen sensor. Int. J. Hydrog. Energy 45 (15), 9268–9277. doi:10.1016/j.ijhydene.2020.01.119.
Azofra, L. M., Sun, C. H., Cavallo, L., and MacFarlane, D. R. (2017). Feasibility of N2 binding and reduction to ammonia on Fe-deposited MoS2 2D sheets: a DFT study. Chem. Eur. J. 23 (24), 8275–8279. doi:10.1002/chem.201701113.
Barzegar, M., Zad, A. I., and Tiwari, A. (2019). On the performance of vertical MoS2 nanoflakes as a gas sensorformance of vertical MoS2 nanoflakes as a gas sensor. Vacuum 167, 90–97. doi:10.1016/j.vacuum.2019.05.033.
Bhakhar, S. A., Patel, N. F., Zankat, C. K., Tannarana, M., Solanki, G. K., and Patel, K. D.etal (2019). Sonochemical exfoliation and photodetection properties of MoS2 nanosheets. Mater. Sci. Semicond. Process. 98, 13–18. doi:10.1016/j.mssp.2019.03.017.
Chen, D. C., Tang, J., Zhang, X. X., Li, Y., and Liu, H. J. (2019a). Detecting decompositions of sulfur hexafluoride using MoS2 monolayer as gas sensor. IEEE Sens. J. 19, 39–46. doi:10.1109/JSEN.2018.2876637.
Chen, D. C., Zhang, X. X., Tang, J., Cui, H., and Li, Y. (2018). Noble metal (Pt or Au)-doped monolayer MoS2 as a promising adsorbent and gas-sensing material to SO2, SOF2, and SO2F2: a DFT study. Appl. Phys. A-Mater. 124 (2), 194. doi:10.1007/s00339-018-1629-y.
Choi, G. J., Mishra, R. K., and Gwag, J. S. (2020). 2D layered MoS2 based gas sensor for indoor pollutant formaldehyde gas sensing applications. Mater. Lett. 264, 127385. doi:10.1016/j.matlet.2020.127385.
Cui, H., Zhang, X. X., Zhang, G. Z., and Tang, J. (2019). Pd-doped MoS2 monolayer: a promising candidate for DGA in transformer oil based on DFT method. Appl. Surf. Sci. 470, 1035–1042. doi:10.1016/j.apsusc.2018.11.230.
Fan, C., Liu, G. Z., Zhang, Y. H., and Wang, M. J. (2017). Synthesis and gas-responsive characteristics to methanol and isopropanol of bean-sprout-like MoS2. Mater. Lett. 209, 8–10. doi:10.1016/j.matlet.2017.07.092.
Gui, Y. G., Liu, D. K., Li, X. D., Tang, C., and Zhou, Q. (2019). DFT-based study on H2S and SOF2 adsorption on Si-MoS2 monolayer. Results Phys. 13, 1–8. doi:10.1016/j.rinp.2019.102225.
Han, L. F., Wu, S. D., Hu, Z., Chen, M. Z., Ding, J. W., and Wang, S. W.etal (2020). Hierarchically porous MoS2-carbon hollow rhomboids for superior performance of the anode of sodium-ion batteries. ACS Appl. Mater. Interfaces 12 (9), 10402–10409. doi:10.1021/acsami.9b21365.
Johari, M. H., Sirat, M. S., Mohamed, M. A., Nasir, S. N. F. M., Teridi, M. A. M., and Mohmad, A. R. (2020). Effects of Mo vapor concentration on the morphology of vertically standing MoS2 nanoflakes. Nanotechnology 31 (30), 305710. doi:10.1088/1361-6528/ab8666.
Kang, M. A., Han, J. K., Cho, S. Y., Bu, S. D., Park, C. Y., and Myung, S.etal (2017). Strain-gradient effect in gas sensors based on three-dimensional hollow molybdenum disulfide nanoflakes. ACS Appl. Mater. Interfaces 9 (50), 43799–43806. doi:10.1021/acsami.7b14262.
Li, S., Lee, J. K., Zhou, S., Pasta, M., and Warner, J. H. (2019). Synthesis of surface grown Pt nanoparticles on edge-enriched MoS2 porous thin films for enhancing electrochemical performance. Chem. Mat. 31 (2), 387–397. doi:10.1021/acs.chemmater.8b03540.
Li, T., Gui, Y. G., Zhao, W. H., Tang, C., and Dong, X. C. (2020). Palladium modified MoS2 monolayer for adsorption and scavenging of SF6 decomposition products: a DFT study. Physica E 123, 114178. doi:10.1016/j.physe.2020.114178.
Li, Y. X., Song, Z. X., Li, Y. N., Chen, S., Li, S., and Li, Y. H.etal (2019). Hierarchical hollow MoS2 microspheres as materials for conductometric NO2 gas sensors. Sens. Actuators, B-Chem. 282, 259–267. doi:10.1016/j.snb.2018.11.069.
Liu, H. C., Zhou, Q., Zhang, Q. Y., Hong, C. X., Xu, L. N., and Jin, L. F.etal (2017). Synthesis, characterization and enhanced sensing properties of a NiO/ZnO p-n junctions sensor for the SF6 decomposition byproducts SO2, SO2F2, and SOF2. Sensors 17 (4), 913. doi:10.3390/s17040913.
Lu, Z. R., Zhou, Q., Wang, C. S., Wei, Z. J., Xu, L. N., and Gui, Y. G. (2018). Electrospun ZnO-SnO2 composite nanofibers and enhanced sensing properties to SF6 decomposition byproduct H2S. Front. Chem. 6, 540. doi:10.3389/fchem.2018.00540.
Lu, Z. R., Zhou, Q., Wei, Z. J., Xu, L. N., Peng, S. D., and Zeng, W. (2019). Synthesis of hollow nanofibers and application on detecting SF6 decomposing products. Front. Mater. 6, 183. doi:10.3389/fmats.2019.00183.
Park, J., Mun, J. H., Shin, J. S., and Kang, S. W. (2018). Highly sensitive two-dimensional MoS2 gas sensor decorated with Pt nanoparticles. R. Soc. Open Sci. 5 (12), 181462. doi:10.1098/rsos.181462.
Qian, H., Lu, W. H., Wei, X. X., Chen, W., and Deng, J. (2019). H2S and SO2 adsorption on Pt-MoS2 adsorbent for partial discharge elimination: a DFT study. Results Phys. 12, 107–112. doi:10.1016/j.rinp.2018.11.035.
Sahoo, M. P. K., Wang, J., Zhang, Y. J., Shimada, T., and Kitamura, T. (2016). Modulation of gas adsorption and magnetic properties of monolayer-MoS2 by antisite defect and strain. J. Phys. Chem. C. 120 (26), 14113–14121. doi:10.1021/acs.jpcc.6b03284.
Sangeetha, M., and Madhan, D. (2020). Ultra sensitive molybdenum disulfide (MoS2)/graphene based hybrid sensor for the detection of NO2 and formaldehyde gases by fiber optic clad modified method. Opt. Laser Technol. 127, 106193. doi:10.1016/j.optlastec.2020.106193.
Singh, A. K., Kumar, P., Late, D. J., Kumar, A., Patel, S., and Singh, J. (2018). 2D layered transition metal dichalcogenides (MoS2): synthesis, applications and theoretical aspects. Appl. Mater. Today 13, 242–270. doi:10.1016/j.apmt.2018.09.003.
Sun, T., Wang, J., Chi, X., Lin, Y. X., Chen, Z. X., and Ling, X.etal (2018). Engineering the electronic structure of MoS2 nanorods by N and Mn dopants for ultra-efficient hydrogen production. ACS Catal. 8 (8), 7585–7592. doi:10.1021/acscatal.8b00783.
Tu, J. G., Xiao, X., Wang, M. Y., and Jiao, S. Q. (2019). Hierarchical flower-like MoS2 microspheres and their efficient Al storage properties. J. Phys. Chem. C. 123 (44), 26794–26802. doi:10.1021/acs.jpcc.9b07509.
Wang, J. X., Zhou, Q., Lu, Z. R., Gui, Y. G., and Zeng, W. (2019b). Adsorption of H2O molecule on TM (Au, Ag) doped-MoS2 monolayer: a first-principles study. Physica E 113, 72–78. doi:10.1016/j.physe.2019.05.006.
Wang, J. X., Zhou, Q., Lu, Z. R., Wei, Z. J., and Zeng, W. (2019c). Gas sensing performances and mechanism at atomic level of Au-MoS2 microspheres. Appl. Surf. Sci. 490, 124–136. doi:10.1016/j.apsusc.2019.06.075.
Wang, J. X., Zhou, Q., Xu, L. N., Gao, X., and Zeng, W. (2020). Gas sensing mechanism of dissolved gases in transformer oil on Ag-MoS2 monolayer: a DFT study. Physica E 118, 113947. doi:10.1016/j.physe.2019.113947.
Wang, J. X., Zhou, Q., and Zeng, W. (2019a). Competitive adsorption of SF6 decompositions on Ni-doped ZnO (100) surface: computational and experimental study. Appl. Surf. Sci. 479, 185–197. doi:10.1016/j.apsusc.2019.01.255.
Wei, H. L., Gui, Y. G., Kang, J., Wang, W. B., and Tang, C. (2018). A DFT study on the adsorption of H2S and SO2 on Ni doped MoS2 monolayer. Nanomaterials 8 (9), 646. doi:10.3390/nano8090646.
Wei, Z. J., Zhou, Q., and Zeng, W. (2020). Hierarchical WO3-NiO microflower for high sensitivity detection of SF6 decomposition byproduct H2S. Nanotechnology 31 (21), 215701. doi:10.1088/1361-6528/ab73bd.
Zeng, F., Ju, T., Zhang, X., Pan, J., Qiang, Y., and Hou, X. (2015). Influence regularity of trace H2O on SF6 decomposition characteristics under partial discharge of needle-plate electrode. IEEE T. Dielect. El. In. 22 (1), 287–295. doi:10.1109/TDEI.2014.004217.
Zhang, D. Z., Jiang, C. X., Li, P., and Sun, Y. (2017a). Layer-by-layer self-assembly of Co3O4 nanorod-decorated MoS2 nanosheet-based nanocomposite toward high-performance ammonia detection. ACS Appl. Mater. Inter. Interfaces 9 (7), 6462–6471. doi:10.1021/acsami.6b15669.
Zhang, D. Z., Wu, J. F., Li, P., and Cao, Y. H. (2017b). Room temperature SO2 gas-sensing properties based on a metal-doped MoS2 nanoflower: an experimental and density functional theory investigation. J. Mater. Chem. Am. 5 (39), 20666–20677. doi:10.1039/c7ta07001b.
Zhang, R. Y., Fu, D., Ni, J. M., Sun, C. B., and Song, S. X. (2018). Adsorption for SO2 gas molecules on B, N, P and Al doped MoS2: the DFT study. Chem. Phys. Lett. 715, 273–277. doi:10.1016/j.cplett.2018.11.054.
Zhang, S., Zhang, W., Nguyen, T. H., Jian, J., and Yang, W. (2019). Synthesis of molybdenum diselenide nanosheets and its ethanol-sensing mechanism. Mater. Chem. Phys. 222, 139–146. doi:10.1016/j.matchemphys.2018.08.062.
Zhang, X. X., Chen, D., Cui, H., Dong, X., Xiao, S., and Tang, J. (2017a). Understanding of SF6 decompositions adsorbed on cobalt-doped SWCNT: A DFT study. Appl. Surf. Sci. 420, 371–382. doi:10.1016/j.apsusc.2017.05.163.
Zhang, X. X., Cui, H., and Gui, Y. G. (2017b). Synthesis of graphene-based sensors and application on detecting SF6 decomposing products: a review. Sensors 17 (2), 363. doi:10.3390/s17020363.
Zhang, Y. J., Zeng, W., and Li, Y. Q. (2018a). Hydrothermal synthesis and controlled growth of hierarchical 3D flower-like MoS2 nanospheres assisted with CTAB and their NO2 gas sensing properties. Appl. Surf. Sci. 455, 276–282. doi:10.1016/j.apsusc.2018.05.224.
Zhang, Y. J., Zeng, W., and Li, Y. Q. (2018b). The hydrothermal synthesis of 3D hierarchical porous MoS2 microspheres assembled by nanosheets with excellent gas sensing properties. J. Alloys Compd. 749, 355–362. doi:10.1016/j.jallcom.2018.03.307.
Zhao, B., Li, C. Y., Liu, L. L., Zhou, B., Zhang, Q. K., and Chen, Z. Q.etal (2016). Adsorption of gas molecules on Cu impurities embedded monolayer MoS2: a first-principles study. Appl. Surf. Sci. 382, 280–287. doi:10.1016/j.apsusc.2016.04.158.
Zhong, Y. L., Liu, D., Wang, L. T., Zhu, H. G., and Hong, G. (2020). Controllable synthesis of hierarchical MoS2 nanotubes with ultra-uniform and superior storage potassium properties. J. Colloid Interface Sci. 561, 593–600. doi:10.1016/j.jcis.2019.11.034.
Zhou, Q., Chen, W. G., Li, J., Peng, S. D., Lu, Z. R., and Yang, Z.etal (2018b). Highly sensitive hydrogen sulfide sensor based on titanium dioxide nanomaterials. J. Nanoelectron. Optoelectron. 13 (12), 1784–1788. doi:10.1166/jno.2018.2417.
Zhou, Q., Chen, W. G., Xu, L. N., Kumarc, R., Gui, Y. G., and Zhao, Z. Y.etal (2018a). Highly sensitive carbon monoxide (CO) gas sensors based on Ni and Zn doped SnO2 nanomaterials. Ceram. Int. 44 (4), 4392–4399. doi:10.1016/j.ceramint.2017.12.038.
Zhou, Q., Hong, C. X., Yao, Y., Hussain, S., Xu, L. N., and Zhang, Q. Y.etal (2018c). Hierarchically MoS2 nanospheres assembled from nanosheets for superior CO gas-sensing properties. Mater. Res. Bull. 101, 132–139. doi:10.1016/j.materresbull.2018.01.030.
Zhou, Q., Umar, A., Sodki, E. M., Amine, A., Xu, L. N., and Gui, Y. G.etal (2018d). Fabrication and characterization of highly sensitive and selective sensors based on porous NiO nanodisks. Sens. Actuator B-Chem. 259, 604–615. doi:10.1016/j.snb.2017.12.050.
Zhou, Q., Xu, L. N., Umar, A., Chen, W. G., and Kumar, R. (2018e). Pt nanoparticles decorated SnO2 nanoneedles for efficient CO gas sensing applications. Sens. Actuator B-Chem. 256, 656–664. doi:10.1016/j.snb.2017.09.206.
Keywords: molybdenum disulfide, morphological characteristics, SF6 decomposing products, sensing application, gas insulated switchgear
Citation: Qian G, Peng Q, Wang H, Wang S and Dai W (2020) Morphological Characteristics of Molybdenum Disulfide and Current Application on Detecting SF6 Decomposing Products. Front. Mater. 7:580245. doi: 10.3389/fmats.2020.580245
Received: 05 July 2020; Accepted: 02 September 2020;
Published: 06 November 2020.
Edited by:
Wen Zeng, Chongqing University, ChinaReviewed by:
Yanqiong Li, Chongqing University of Arts and Sciences, ChinaJianbo Yin, Northwestern Polytechnical University, China
Copyright © 2020 Qian, Peng, Wang, Wang and Dai. This is an open-access article distributed under the terms of the Creative Commons Attribution License (CC BY). The use, distribution or reproduction in other forums is permitted, provided the original author(s) and the copyright owner(s) are credited and that the original publication in this journal is cited, in accordance with accepted academic practice. No use, distribution or reproduction is permitted which does not comply with these terms.
*Correspondence: Guochao Qian, MTY0Njg4ODQ3QHFxLmNvbQ==