- 1Key Laboratory of Green Construction and Intelligent Maintenance for Civil Engineering of Hebei Province, Yanshan University, Qinhuangdao, China
- 2College of Civil Engineering and Architecture, Hebei University, Baoding, China
- 3Shijiazhuang Institution of Railway Technology, Shijiazhuang, China
In order to analyze the influence mechanism of delay period on the mechanical properties of reactive powder concrete (RPC), the compressive strength of RPC with delay periods of 18, 24, and 30 h was tested at the age of 7, 28, and 90 days, respectively. The results show that compared with the RPC with delay period of 18 h, the compressive strength of the RPC with delay periods of 24 and 30 h increases by 3.2 and 4.2%, respectively, and the long-term strength reduction ratio decreases by 22.8 and 71.9%, respectively. The constitutive model curves of RPC under different delay period show that the initial elastic modulus E increases with the delay period and the strength and rigidity of RPC increase with the extension of delay period. According to the non-evaporation water quantity test, it could be speculated that the quantities of hydration products of the RPC with delay periods of 24 and 30 h slightly increase compared with the RPC with delay period of 18 h. X-ray diffraction (XRD) analysis show that the delay periods of 24 and 30 h consume more 3CaO·SiO2 (C3S) and 2CaO·SiO2 (C2S) compared with delay period of 18 h. Seen from the scanning electron microscope (SEM) image, the structures of the three groups of samples are relatively dense and have no significant difference. Through energy dispersive X-ray spectroscopy (EDS) analysis, the calcium-silicon ratios of hydration products of the RPC with delay periods of 18, 24, and 30 h are 1.81, 1.56, and 1.54, respectively. The existence of C-S-H gel and Ca(OH)2 in hydration products is confirmed by thermogravimetric-differential scanning calorimetry (DSC-TG) analysis. An appropriate delay period (30 h in this paper) generates more hydration products, then improves the compactness of the internal structure and reduces the calcium-silicon ratio of hydration products, and it is conducive to the growth of RPC compressive strength and the stability of long-term compressive strength.
Introduction
Reactive powder concrete (RPC) is a new type of concrete prepared by steam curing, which has removed the coarse aggregate to improve uniformity of the matrix (Abid et al., 2017). The fineness and reactivity of the components of RPC are increased by steam curing (Cheyrezy et al., 1995). According to the most compact principle, the initial defects such as voids and micro-cracks in the structure are greatly reduced (Chan and Chu, 2004).
Compared to common cement-based materials, RPC has excellent mechanical properties and durability (Huynh et al., 2015; Mostofinejad et al., 2016; Song and Liu, 2016). By now, it has not been widely used for two reasons. First, the cost is high (Yazici et al., 2008), and the maintenance conditions are complex. Steam curing is only suitable for preparing smaller prefabricated components, and it is difficult to prepare RPC in construction site. Secondly, the strength of RPC will decrease in the long term, which is not negligible for structures that need to bear long-term load. The long-term strength reduction of concrete refers to the condition that the strength in the later age (90 days) is lower than that in the earlier age (7, 28 days) under the normal mix proportion and curing conditions (Soroka, et al., 1978). Some scholars have found that the long-term strength of RPC decreases gradually (Zhang et al., 2007; Yazici et al., 2010; Wang et al., 2014). Zhang et al. (2007) showed that the strength of RPC would be decreased in the middle and later age due to steam curing. It was speculated that the early hydration was too fast and too many hydration products hindered the later strength improvement. Wang et al. (2014) tested the uniaxial compressive strength of RPC at the age of 7 days, 3 months, and 3 years after hot water curing. It was found that the strength of RPC did not decrease after 3 months, but it did after 3 years.
High and stable compressive strength could better ensure the quality of concrete structure design, thereby improving the safety and stability of the structure (Nadiger et al., 2018). Zhao (2010) showed that changing the delay period may reduce the strength reduction ratio of steam cured cement paste. Delay period refers to the stage of standard maintenance from specimens molding to steam curing (Erdem et al., 2003). There are many studies on the effect of delay period on ordinary concrete (Soroka et al., 1978; Talakokula et al., 2015). Many researchers have indicated that a suitable delay period is beneficial to concrete properties, such as strength and durability (Shideler and Chamberlin, 1949; Hanson, 1963). Shideler and Chamberlin (1949) showed that the concrete with delay periods of 2–6 h had 15–40% higher strengths than the concrete with steam curing immediately after casting. Hanson (1963) proved that compressive strengths of concrete increased at all ages, as the delay period increased from 1 to 5 h. Erdem et al. (2003) tested the compressive strength of ordinary concrete at different ages under the steam curing of 80 °C with the delay periods of 1, 2, and 3 h. It was found that when the paste was hardened before steam curing, concrete tended to obtain higher strength. Taylor et al. (2001) showed that if delay periods are not chosen properly, thermal stresses can cause micro-cracks, and affect the strength of concrete. However, there are few studies related to the effect of delay period on RPC. Liu et al. (2020) investigated the effects of steam curing parameters on the capillary water absorption of concrete, and it was found that long delay period is benefificial to the development of concrete structure. Zdeb (2017) explored the strength of RPC under the delay periods of 0, 3, 6, 12, and 24 h at the steam curing of 90 °C. The results showed that the strength of RPC was the highest when the delay period was 6 h, and the length of the delay period obviously affected the mechanical properties of RPC.
The function of the delay period is to make the harden paste form a certain plastic structure strength to prevent the heat damage during the steam curing (Yang et al., 2003). Previous studies had proved that the delay period has an effect on the mechanical properties and internal microstructure of concrete, but the mechanism of the effect of delay period on the long-term mechanical properties of RPC had not been discussed. Wu et al. (2019) studied the effect of delay period under steam curing on high strength mortar. It was found that the different delay period will result in the difference of the composition of the paste, and insufficient delay period (6 h) will cause the strength of the specimen under steam curing to be lower than the standard curing.
Previous studies had proved that the delay period has an effect on the mechanical properties and internal microstructure of concrete, but the mechanism of the effect of delay period on the long-term mechanical properties of RPC had not been discussed. Based on this, in order to analyze the influence of delay period on the mechanical properties of RPC, the compressive strength of RPC at the age of 7, 28, and 90 days were tested under the delay periods of 18, 24, and 30 h, and the long-term compressive strength reduction ratio was calculated. Meanwhile, in order to study the mechanism of the effect of delay period on the long-term mechanical properties of RPC from microstructure, the composition and microstructure was explored by non-evaporative water volume, X-ray diffraction (XRD), the scanning electron microscope (SEM), energy dispersive X-ray spectroscopy (EDS) and thermogravimetric-differential scanning calorimetry (DSC-TG).
Experimental
Raw Materials
P. II 52.5 Portland cement, encrypted silica fume (SF) and S115 ground slag (GS) were used as cementitious materials, with the main chemical composition and specific surface area were shown in Table 1. The method used to obtain the specific surface area was Blaine method. The aggregate was natural river sand with particle size ranging from 0.16 to 2.36 mm, and steel fibers were copper-plated round straight steel fibers with a length of 12–13 mm and a diameter of 0.15–0.20 mm. Polycarboxylic acid (standard high performance water reducer) was used as superplasticizer. The performance index of polycarboxylate superplasticizer was shown in Table 2.
Methods
Preparation of RPC specimens
The cement mortar was stirred evenly and the RPC specimens of 40 × 40 × 160 mm were prepared according to the mix proportion in Table 3. The prepared process of RPC was shown as Figure 1.
Non-evaporated Water Volume Test of Cement-Silica Fume-Slag System
The test results of non-evaporated water under different delay periods were compared, and the amount of hydration products under different delay period were measured by measuring the amount of non-evaporated water in the paste from hydration to the specified age. Testing methods for non-evaporated water were shown as Figure 2.
X-Ray Diffraction, SEM Image, EDS and DSC-TG Analysis of the Paste
At the age of 90 days, the internal non-carbonated zone of the hardened paste was taken under different delay periods, and the appropriate amount of fine powder filtered and dried by Non-evaporated Water Volume Test of Cement-Silica Fume-Slag System method was used for X-ray diffraction (XRD) analysis. The 2θ values ranged from 10° to 80° and the scanning rate applied was 1°min for all specimens.
The microstructure of the hardened paste prepared by Non-evaporated Water Volume Test of Cement-Silica Fume-Slag System method was observed by scanning electron microscope (SEM) image after the vacuum and gold spray treatment. And the energy dispersive X-ray spectroscopy (EDS) analysis of the hydration products was carried out.
DSC-TG analysis of the paste prepared by Non-evaporated Water Volume Test of Cement-Silica Fume-Slag System method was carried out by the STA 449F5 integrated thermal analyzer (Zhao et al., 2016). The temperature rising rate was 10 °C/min, and the Ar was used as the protective gas to prevent the carbonization of the specimens during the heating process.
Results and Discussion
Effect of Delay Period on Compressive Strength and Long-Term Strength Reduction Ratio of RPC
The compressive strength of RPC specimens under different age were shown in Table 4. Results in Table 4 were expressed as mean value ± 1x standard deviation. The difference of compressive strength under different delay period was analyzed by one-way analysis of variance. The test value of F was 5.272, p = 0.007 < 0.05. It was shown that the compressive strength of RPC had statistically significant difference with the prolongation of delay period. Compared with the maximum values of 7 and 28 days compressive strength, the reduction ratio of RPC long-term compressive strength at the age of 90 days was calculated. The results were shown in Figure 3.
According to the compressive strength from Table 4, it could be seen that the compressive strength of RPC at the age of 90 days was 179.28, 184.95, and 186.71 MPa under the delay periods of 18, 24, and 30 h respectively. Compared with delay period of 18 h, the compressive strength of RPC with delay periods of 24 and 30 h increased by 3.2 and 4.2%, respectively. It could be seen that the compressive strength of RPC increased with delay periods.
Table 4 showed that the maximum compressive strength of RPC under the delay periods of 18, 24, and 30 h was 184.46, 189.05, and 188.20 MPa, respectively. The maximum compressive strength of 7 and 28 days was taken as the reference value, and the compressive strength of 90 days was compared with it to calculate the long-term reduction rate. Figure 3 showed that the long-term strength reduction ratio of 24 and 30 h was 22.8 and 71.9% lower than that of 18 h. It could be seen that the long-term strength reduction ratio of RPC decreases gradually with the increase of delay periods.
Effect of Delay Period on Initial Elastic Modulus of RPC
At the age of 90 days, the stress-strain curves of RPC specimens were obtained by uniaxial compression test. The stress-strain curves and error bars were shown in Figure 4. The difference of compressive strength under different delay period at the age of 90 days was analyzed by one-way analysis of variance. The test value of F was 3.852, p = 0.03 < 0.05. It was shown that the compressive strength of RPC at the age of 90 days had statistically significant difference with the prolongation of delay period.
Based on Weibull distribution phenomenological method and equivalent strain hypothesis theory (Lemaitre, 1983), Wang et al. (2006) established the constitutive model of damage of steel fiber concrete as Eq. 1.
In the formula,
It could be seen from Table 5 that the values of m under the delay periods of 18, 24, and 30 h was 2.5748, 2.5585, and 2.4798, respectively. The difference between them were small. In order to analyze the influence of delay period on initial elastic modulus E, the average value 2.5377 of m was taken and parameter E was deduced by least square method again based on the experimental data. The results and constitutive model expressions were shown in Table 6. The constitutive model curves of RPC under different delay period were shown in Figure 6.
It could be seen from Table 6 that the values of E under the delay periods of 18, 24, and 30 h was 9,933, 10,306, and 10,548 MPa, respectively. The initial elastic modulus E in the constitutive model increased with the delay period. As could be seen from Figure 6, the strength and rigidity of RPC increased with the extension of delay period. It was conformed that the delay period was helpful to improve the mechanical properties of RPC, generated more hydration products, then improved the compactness of the internal structure. And it was conducive to the growth of RPC compressive strength and rigidity.
Effect of Delay Period on Non-evaporated Water Volume of the Paste
The amount of non-evaporative water in the paste at the age of 7, 28, and 90 days were measured. The results were shown in Figure 7. It could be seen from Figure 7 that at the age of 7 days, the non-evaporative water of 18, 24, and 30 h was 7.8, 7.8, and 7.9%, respectively. The non-evaporated water volume at the age of 28 days increased by 0.3, 0.4, and 0.4%, respectively compared with that of 7 days. The non-evaporated water of 90 days increased by 0.2, 0.3, and 0.3%, respectively compared with 28 days. The difference of non-evaporative water under different delay period was analyzed by one-way analysis of variance. The test value of F was 5.190, p = 0.013 < 0.05. It was shown that the content of non-evaporative water had statistically significant difference with the prolongation of delay period. It could be seen that the amount of non-evaporated water increases slightly with the prolongation of delay period. The difference of non-evaporative water at different age was analyzed by one-way analysis of variance. The test value of F was 9.497, p = 0.001 < 0.05. It was shown that the content of non-evaporative water had statistically significant difference with the age of RPC.
As the age progresses, hydration products were continuously separated out, which made the space for new hydration products to be contained in the paste become smaller and smaller. When there was no room to accommodate, the new hydration products produce compressive stress on the surrounding raw hydration products, resulting in micro-cracks in the structure, and consequently strength retrogression. At the same time, compared with the early hydration stage, the increase of hydration products decreased gradually during the 90-day period. The reason was that the hydration rate of cement was very fast during the steam curing period, and the hydration products gradually formed a layer of film on the surface of un-hydrated particles. As the age progressed, the hydration product films accumulated and they gradually wrapped up the surface, which hindered the internal water entry and the outward precipitation of hydration products, thus affecting the further hydration in the later age (Zhao et al., 2010).
XRD Analysis of Hydration Products
A suitable delay period could not only make cement hydration more fully and produce more hydration products, but also improve the compactness of RPC structure. The amount of hydration products also changed with the delay period. In order to analyze the mechanism of the effect of delay period on the compressive strength and long-term strength reduction of RPC from the micro-structure level, XRD analysis was used to verify the relationship between hydration degree of the paste and the delay period at the age of 90 days. The results were shown in Figure 8, and the pdf number of C2S, C3S, and Ca(OH)2 was 83-0460, 73-0599, and 72-0156, respectively. Determination of content of C2S, C3S, and Ca(OH)2 by internal standard method, the result was shown in Figure 9.
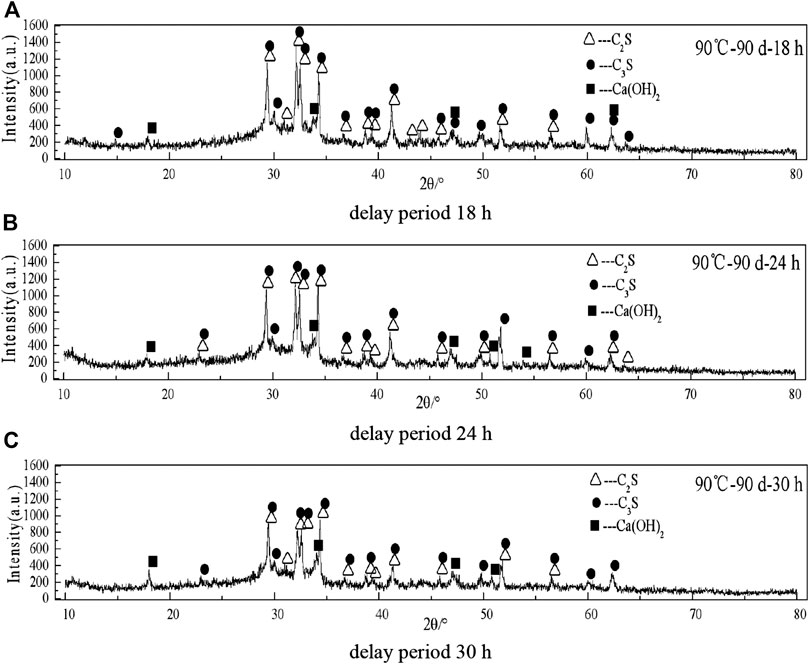
Figure 8. XRD pattern of the paste sample under different delay period. (A) delay period 18 h. (B) delay period 24 h. (C) delay period 30 h.
Figure 8 indicated that the main phase composition of RPC hydration products were basically the same under different delay periods. They were mainly composed of hydrated calcium silicate, Ca(OH)2 crystals, un-hydrated cement particles 3CaO·SiO2 (C3S) and 2CaO·SiO2 (C2S). Calcium silicate hydrate was amorphous and cannot be recognized by XRD. The diffraction peaks of C3S and C2S were more obvious in Figure 8, mainly because the water-cement ratio of RPC was very low, the hydration speed was very fast at 90 °C, the internal structure was compact, and the cement cannot be completely hydrated due to a lack of water and space. Compared with the intensity of the diffraction peaks of C3S and C2S under different delay periods at 90 days, the relationship was: 18 h > 24 h > 30 h. The results showed that at the age of 90 days, the consumption of un-hydrated particles was greater, the degree of hydration was higher and the hydration products were more when the paste was delayed by 30 h. The conclusion was consistent with that of Effect of Delay Period on Non-evaporated Water Volume of the Paste about the quantity of non-evaporative water. At the same time, the diffraction peaks of Ca(OH)2 crystals at 2θ = 18° and 2θ = 34° were observed for three groups of paste specimens.
It could be seen from Figures 8, 9 that the corresponding peak of Ca(OH)2 crystals at 90 days was relatively weak under the three delay periods. Because the reaction rate between the admixture and Ca(OH)2 was greatly accelerated by steam at 90 °C, and the consumption of Ca(OH)2 was very large.
SEM Image Analysis of Hydration Products
A certain delay period was conducive to the formation of finer hydration product particles in the cement, so that RPC can obtain higher strength in the steam curing stage. In order to elucidate the mechanism of the effect of delay period on hydration products, the micro-morphology characteristics of hydration products of the paste at the age of 90 days were observed by SEM.
Figure 10 showed that the hydration products were closely connected to each other and formed a dense continuous phase. The internal structure of RPC remained complete and compact. The hydrated calcium silicate cured by steam at 90°C were amorphous and continuous. Flake crystals were Ca(OH)2 crystals and spherical particles were un-hydrated particles (Cheyrezy et al., 1995; Liu and Song, 2010). It could be seen from Figure 10 that there was no significant difference in the morphology of hydration products among the three groups of samples, and no complete crystalline Ca(OH)2 crystal was found. Because the reaction activity of silica fume was fully stimulated and a large amount of Ca(OH)2 crystals were consumed under steam curing at 90°C. The cementation between hydration products was relatively dense.
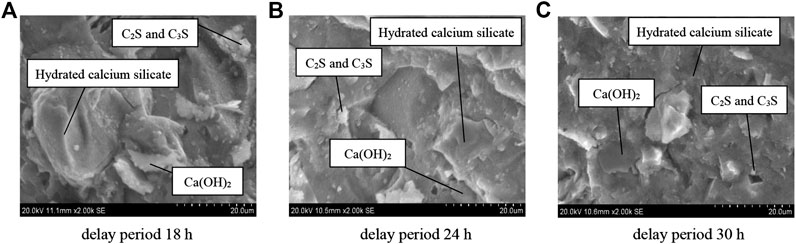
Figure 10. SEM image of hydration products at 90 days age under different delay period. (A) delay period 18 h. (B) delay period 24 h. (C) delay period 30 h.
Energy Dispersive Spectrum Analysis of Hydration Products
Figure 10 indicated that there was no obvious difference in the morphological characteristics of hydration products under different delay periods, but the development of long-term compressive strength of RPC specimens were obviously different. Based on this difference, the chemical composition and elemental composition of hydration products were determined through the analysis of the back-scattered-electron (BSE) images and energy dispersive spectrum analysis (EDS). The results were shown in Figure 11 and Table 7. The ratio of calcium to silicon of hydration products was calculated, and the hydrated calcium silicate was analyzed qualitatively to prove the influence mechanism of delay period on the long-term mechanical properties of RPC.
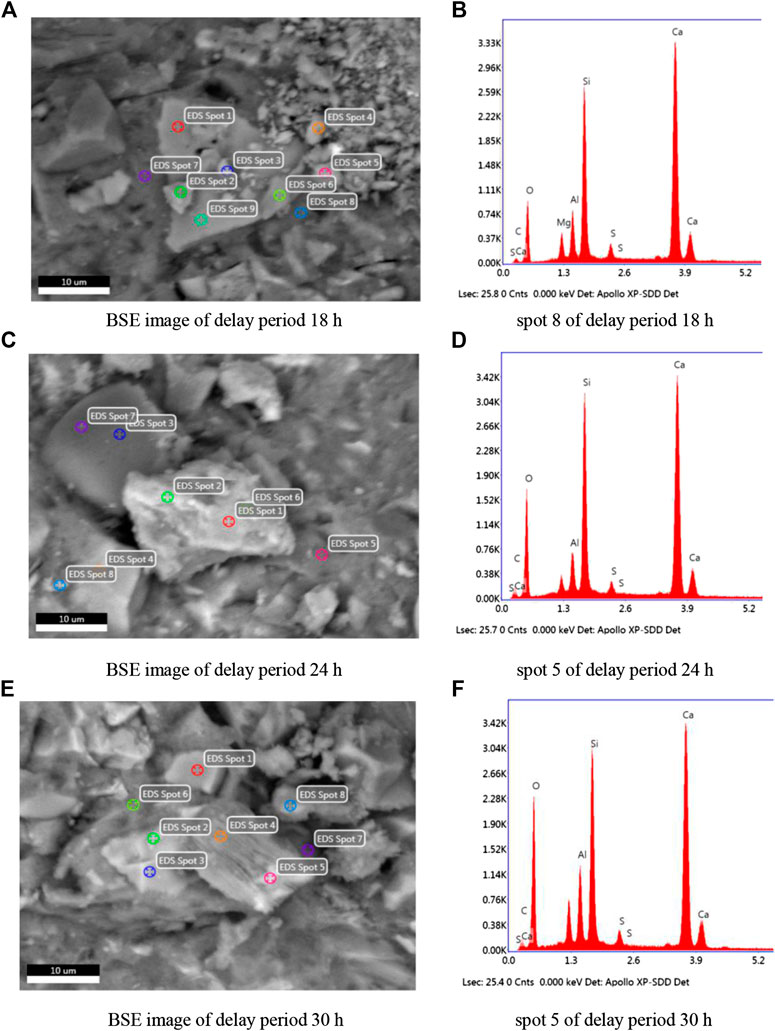
Figure 11. Elemental energy spectrum of hydration products under different delay period. (A) BSE image of delay period 18 h. (B) spot eight of delay period 18 h. (C) BSE image of delay period 24 h. (D) spot five of delay period 24 h. (E) BSE image of delay period 30 h. (F) spot five of delay period 30 h.
It could be seen from Figure 11 and Table 7 that the Ca/Si ratio of hydration products under the delay period of 18 h was 1.81, which belonged to needle hydrated calcium silicate (C2SH crystal) with relatively high Ca/Si ratio. The Ca/Si ratio of hydration products under the delay period of 24 h was 1.56, which belonged to columnar hydrated calcium silicate (C3S2H crystal). The Ca/Si ratio of hydration products under the delay period of 30 h was 1.54, which belonged to C3S2H crystal as well. Documents have shown that the compressive strength of hydration products was higher when the calcium-silicon ratio of hydration products was lower under certain conditions (Wang et al., 2007), and the lower the calcium-silicon ratio was, the denser the morphology of hydration products was, which was consistent with the strength law of Effect of Delay Period on Compressive Strength and Long-Term Strength Reduction Ratio of RPC.
DSC-TG Analysis of Hydration Products
The DSC-TG patterns of the paste at the age of 90 days under different delay period were shown in Figures 12, 13. It could be seen from Figure 12 that the TG curves of the paste under the delay period of 18, 24, and 30 h were relatively close, the hydration products increased slightly with the prolongation of delay period, which was consistent with the results of non-evaporated water. The endothermic peak at 110 °C was the result of dehydration reactions because of the loss of water from C-S-H (Sha et al., 1999; Yang and Yue, 2000). By the analyzation of DSC-TG, the existence of C-S-H gel in hydration products was evidenced, which was consistent with the results of SEM and EDS analysis. The second major peak which occurred between 400 and 500 °C corresponds to the dehydroxylation of Ca(OH)2 (Mohammed et al., 2020). The existence of Ca(OH)2 in the paste specimens was showed in the XRD patterns. The enthalpy (ΔH) of second major peak under the delay period of 18, 24, and 30 h was 4.156 J/g, 6.627 J/g and 9.089 J/g respectively. It can be seen that the degree of hydration was higher with the prolongation of delay period, and the hydration products were more when the paste was delayed by 30 h. That was consistent with the results of XRD analysis.
Mechanism Analyses
Relevant literature (Erdem et al., 2003) had shown that during the delay period, the hydration of cement was uniform and the crystalline particles of hydration products were finer, which was conducive to more adequate hydration reaction during steam curing and the rehydration of the paste in later age. Within the suitable range, the hydration reaction was more sufficient and the long-term strength of the paste was more stable with the prolongation of delay period.
The delay period had two effects on the mechanical properties of RPC. Compared with the delay period of 18 h, the delay period of 24 and 30 h could not only improve the compressive strength of RPC, but also reduce the damage of high temperature steam to the internal structure of RPC in the initial stage of hydration. It could be seen that the appropriate delay period made the early hydration degree of RPC higher, the initial strength higher, and the calcium-silicon ratio of hydration products relatively lower. The dual effects of structure compactness and protection of hydration products on RPC enhanced the compressive strength and long-term strength stability of RPC.
Conclusion
From the test and the analytical results, the following conclusions could be drawn.
1) Compared with the delay period of 18 h, the compressive strength of RPC specimens delayed by 24 and 30 h increased 3.2 and 4.2% respectively. The difference of compressive strength under different delay period was analyzed by one-way analysis of variance. It was shown that the compressive strength of RPC had statistically significant difference with the prolongation of delay period. The long-term strength reduction ratio of RPC specimens under the delay periods of 24 and 30 h decreased 22.8% and 71.9% respectively compared with the delay period of 18 h. Therefore, the appropriate delay period was conducive to increasing the compressive strength of RPC and reducing the reduction ratio of long-term compressive strength.
2) Based on the delay period, there was more adequate hydration reaction during steam curing and the rehydration of the paste in later age. The strength and rigidity of RPC increased with the extension of delay period. The initial elastic modulus E in the constitutive model increased with the delay period.
3) The hydration products of the paste under the delay periods of 24 and 30 h increased compared with the delay period of 18 h. The calcium-silicon ratio of hydration products under the delay periods of 24 and 30 h decreased significantly compared with the delay period of 18 h. And the structure compactness increased gradually with the prolongation of delay periods. Therefore, the suitable delay period could make RPC produce more hydration products with low Ca/Si ratio, improve the compactness of internal structure, and enhance the compressive strength and long-term strength stability of RPC.
Data Availability Statement
The original contributions presented in the study are included in the article/Supplementary Material, further inquiries can be directed to the corresponding author.
Author Contributions
XW: Experimental design, investigation, methodology and writing. QZ: Funding acquisition, investigation, methodology, project administration and writing. XH: Conceptualization, data analysis, supervision, validation, writing and revising. SZ: Data analysis, methodology and writing.
Funding
Financial support from the National Natural Science Foundation of China under the grants of 51578477, the Key Research and Development Project of Hebei Province under the grant of 19211505D.
Conflict of Interest
The author declares that the research was conducted in the absence of any commercial or financial relationships that could be construed as a potential conflict of interest.
References
Abid, M., Hou, X. M., Zheng, W. Z., and Waqar, G. Q. (2017). Mechanical properties of steel fiber-reinforced reactive powder concrete at high temperature and after cooling. Procedia Eng. 210, 597–604. doi:10.1016/j.proeng.2017.11.119
Chan, Y. W., and Chu, S. H. (2004). Effect of silica fume on steel fiber bond characteristics in reactive powder concrete. Cem. Concr. Res. 34 (7), 1167–1172. doi:10.1016/j.cemconres.2003.12.023
Cheyrezy, M., Maret, V., and Frouin, L. (1995). Microstructural analysis of RPC (reactive powder concrete). Cem. Concr. Res. 25 (7), 1491–1500. doi:10.1016/0008-8846(95)00143-Z
Erdem, T. K., Turanli, L., and Erdogan, T. Y. (2003). Setting time: an important criterion to determine the length of the delay period before steam curing of concrete. Cem. Concr. Res. 33 (5), 741–745. doi:10.1016/s0008-8846(02)01058-x
Hanson, J. A. (1963). Optimum steam curing procedure in precasting plants. J. ACI Journal Proceedings. 60 (1), 75–100. doi:10.14359/7843
Huynh, L., Foster, S., Valipour, H., and Randall, R. (2015). High strength and reactive powder concrete columns subjected to impact: experimental investigation. Constr Build Mater. 78, 153–171. doi:10.1016/j.conbuildmat.2015.01.026
Lemaitre, J. (1983). How to use damage mechanics. Nucl. Eng. Des. 80 (2), 233–245. doi:10.1016/0029-5493(84)90169-9
Liu, B. J., Shi, J. Y., Zhou, F., Shen, S., Ding, Y. B., and Qin, J. L. (2020). Effects of steam curing regimes on the capillary water absorption of concrete: prediction using multivariable regression models. Constr. Build. Mater. 256, 119426. doi:10.1016/j.conbuildmat.2020.119426
Liu, J. H., and Song, S. M. (2010). Effects of curing systems on properties of high volume fine mineral powder RPC and appearance of hydrates. J. Wuhan Univ. Technol.-Mater. Sci. Ed. 25 (4), 619–623. doi:10.1007/s11595-010-0056-5
Mohammed, A., Rafiq, S., Mahmood, W., Noaman, R., AL-Darkazali, H., Ghafor, K., et al. (2020). Microstructure characterizations, thermal properties, yield stress, plastic viscosity and compression strength of cement paste modified with nanosilica. J. Mater. Res. 9 (5), 10941–10956. doi:10.1016/j.jmrt.2020.07.083
Mostofinejad, D., Nikoo, M. R., and Hosseini, S. A. (2016). Determination of optimized mix design and curing conditions of reactive powder concrete (RPC). Constr. Build. Mater. 123, 754–767. doi:10.1016/j.conbuildmat.2016.07.082
Nadiger, A., Harinath Reddy, C., Vasudevan, S., and Mini, K. M. (2018). “Fuzzy logic modeling for strength prediction of reactive powder concrete.” in 3rd International Conference on Intelligent Computing and Applications, ICICAAkurdi, Pune, India, Singapore: Springer, 632, 375–386. doi:10.1007/978-981-10-5520-1_35
Sha, W., O’Neill, E. A., and Guo, Z. (1999). Differential scanning calorimetry study of ordinary Portland cement. Cem Concr Res. 29 (9), 1487–1489. doi:10.1016/S0008-8846(99)00128-3
Shideler, J. J., and Chamberlin, W. H. (1949). Early strength of concrete as affected by steam curing temperatures. J. ACI Journal Proceedings. 46 (12), 273–283. doi:10.14359/12057
Song, J. W., and Liu, S. H. (2016). Properties of reactive powder concrete and its application in highway bridge. Adv. Mater Sci. Eng., 2016, 1–7. doi:10.1155/2016/5460241
Soroka, I., Jaegermann, C. H., and Bentur, A. (1978). Short-term steam-curing and concrete later-age strength. Mater Struct. 11 (62), 93–96. doi:10.1007/BF02478955
Talakokula, V., Singh, R., and Vysakh, K. (2015). “Effect of delay time and duration of steam curing on compressive strength and microstructure of geopolymer concrete.” Advances in Structural Engineering., New Delhi, India: Springer, 1635–1641. doi:10.1007/978-81-322-2187-6_124
Taylor, H. F. W., Famy, C., and Scrivener, K. L. (2001). Delayed ettringite formation. Cem. Concr. Res. 31 (5), 683–693. doi:10.1016/S0008-8846(01)00466-5
Wang, C. L., Xu, B. G., Li, S. L., and Tang, H. Y. (2006). Study on a constitutive model of damage of SFRC under uniaxial compression. Rock and Soil Mech. 27 (1), 151–154. doi:10.16285/j.rsm.2006.01.030
Wang, X. F., Wang, Y. P., and Wu, L. C. (2014). Degradation phenomenon of basic mechanical properties of plain reactive powder concrete with time. J. Adv. Mater. Res. 1065–1069, 1871–1874. doi:10.4028/www.scientific.net/AMR.1065-1069.1871
Wang, Z., Yang, Y. Z., and Li, J. H. (2007). Preparation of C-S-H-Phase nuclei and its effects on compressive strength of cement. J. Mater. Sci. Technol. 15 (6), 789–796. doi:10.3969/j.issn.1005-0299.2007.06.013
Wu, Y. J., Ren, S. R., Zhang, H., Shao, Z., and Wang, J. F. (2019). Effect of pre-curing time under steam curing on high strength mortar. J. Bulletin of The Chinese Ceramic Society. 38 (8), 2397–2402. doi:10.16552/j.cnki.issn1001-1625.2019.08.009
Yang, N. R., and Yue, W. H. (2000). The handbook of inorganic matalloid materials atlas. Wuhan, China: Wuhan University of Technology Press, 245–267.
Yang, Q. B., Yang, Q. R., and Zhu, P. R. (2003). Scaling and corrosion resistance of steam-cured concrete. Cem. Concr. Res. 33 (7), 1057–1061. doi:10.1016/S0008-8846(03)00010-3
Yazici, H., Yardimci, M. Y., Yigiter, H., Aydin, S., and Turkel, S. (2010). Mechanical properties of reactive powder concrete containing high volumes of ground granulated blast furnace slag. Cem. Concr. Compos. 32 (8), 639–648. doi:10.1016/j.cemconcomp.2010.07.005
Yazici, H., Yigiter, H., Karabulut, A. S., and Baradan, B. (2008). Utilization of fly ash and ground granulated blast furnace slag as an alternative silica source in reactive powder concrete. J. Fuel. 87 (12), 2401–2407. doi:10.1016/j.fuel.2008.03.005
Zdeb, T. (2017). An analysis of the steam curing and autoclaving process parameters for reactive powder concretes. Constr. Build. Mater. 131, 758–766. doi:10.1016/j.conbuildmat.2016.11.026
Zhang, S., Zhou, X. L., Xie, Y. J., and Wang, G. J. (2007). Study on the effect of curing system on the strength and microstructure of reactive powder concrete. J. Concrete. 28, (6), 16–18. doi:10.3969/j.issn.1002-3550.2007.06.006
Zhao, Q. X., He, X. J., Zhang, J. R., and Jiang, J. Y. (2016). Long-age wet curing effect on performance of carbonation resistance of fly ash concrete. J. Constr Build Mater. 127, 577–587. doi:10.1016/j.conbuildmat.2016.10.065
Zhao, X. Y., Liu, B. J., and Jiang, N. N. (2010). Effect of the steam curing paramaters on the hydration characteristics of hardened cement paste. MS dissertation. Changsha (China):Central South University of China.
Keywords: reactive powder concrete, delay period, compressive strength, long-term strength reduction ratio, mechanism analysis
Citation: Wang X, Zhao Q, He X and Zhang S (2021) Mechanism Analysis of the Influence of Delay Period on Mechanical Properties of Reactive Powder Concrete. Front. Mater. 7:563234. doi: 10.3389/fmats.2020.563234
Received: 18 May 2020; Accepted: 15 December 2020;
Published: 20 January 2021.
Edited by:
Dongshuai Hou, Qingdao University of Technology, ChinaReviewed by:
Anatolijus Eisinas, Kaunas University of Technology, LithuaniaNeven Ukrainczyk, Darmstadt University of Technology, Germany
Copyright © 2021 Wang, Zhao, He and Zhang. This is an open-access article distributed under the terms of the Creative Commons Attribution License (CC BY). The use, distribution or reproduction in other forums is permitted, provided the original author(s) and the copyright owner(s) are credited and that the original publication in this journal is cited, in accordance with accepted academic practice. No use, distribution or reproduction is permitted which does not comply with these terms.
*Correspondence: Qingxin Zhao, emhhb3F4MjAwMkAxNjMuY29t