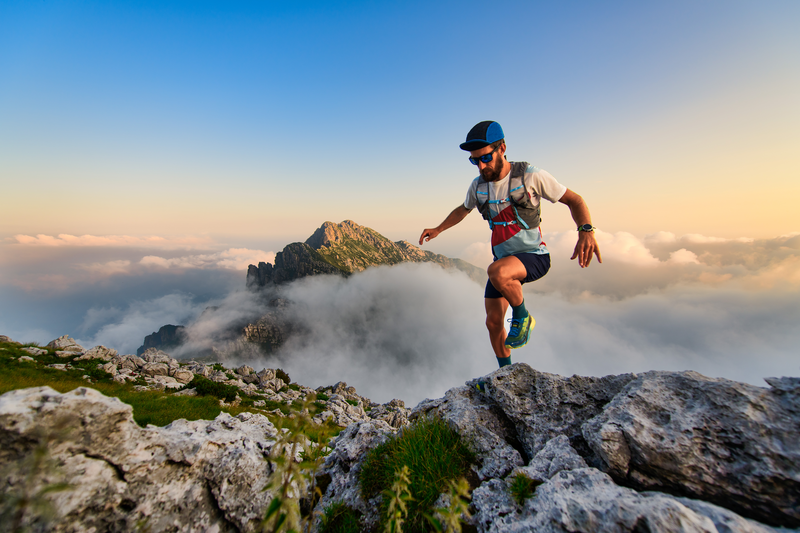
95% of researchers rate our articles as excellent or good
Learn more about the work of our research integrity team to safeguard the quality of each article we publish.
Find out more
ORIGINAL RESEARCH article
Front. Mater. , 30 April 2020
Sec. Ceramics and Glass
Volume 7 - 2020 | https://doi.org/10.3389/fmats.2020.00117
This article is part of the Research Topic Glasses for Energy Applications View all 9 articles
Layered double hydroxides (LDHs) are promising solid electrolytes for all solid-state alkaline fuel cells (AFCs) because of inorganic clay-like materials. However, LDHs were usually obtained as powder, and the formation of thin membranes as a separator of a fuel cell is rather difficult. In this study, a glass paper, non-woven fabric of fine glass fibers with more than 90% porosity, was used as a support for Mg-Al LDH powder, and self-standing, thin membranes for all solid-state AFCs were fabricated. Crystals of Mg-Al LDH were deposited in the inside of the glass paper by repeated immersion in Mg-Al LDH-dispersed solution and drying. Thickness of the obtained Mg-Al LDH thin membrane was about 150 μm, and LDH layer showed c-axis orientation because of the plate-like structure. The H2-O2 fuel cell using the Mg-Al LDH thin membrane as an electrolyte showed open circuit voltage of more than 0.9 V, indicating that the Mg-Al LDH thin membrane is dense and has high gas barrier property. The H2-O2 fuel cell using the Mg-Al LDH thin membrane showed higher power density compared with that using pelletized Mg-Al LDH powder as the electrolyte. The glass paper is proved to be very effective support for making thin Mg-Al LDH electrolyte membrane.
Alkaline fuel cells (AFCs) using electrolytes such as aqueous KOH solution are known to show higher performance compared with well-known fuel cells operable below 200°C such as polymer electrolyte membrane fuel cells and direct alcohol fuel cells because of the faster kinetics for oxygen reduction reaction (Strasser, 1990; Spendelow and Wieckowski, 2007; Cheng et al., 2015; Serov et al., 2018). The wider selection of fuels and electrode materials in AFCs is also well-known (Matsuoka et al., 2005; Kruusenberg et al., 2012). However, KOH aqueous solution used as the electrolyte is very sensitive to the presence of carbon dioxide. The formation of solid carbonate salts in the electrode/electrolyte interface causes the degradation of the cell performance (Gultekin et al., 1994; Gulzow and Schulze, 2004; Merle et al., 2011). Thus, AFCs using solid electrolytes such as anion exchange membranes (AEM) have attracted attention in recent years because AEM which contains no alkali-metal cation is insusceptible to carbon dioxide in air (Adams et al., 2008; Fujiwara et al., 2011; Cheng et al., 2015; Serov et al., 2018).
Layered double hydroxides (LDHs) have been studied as a hydroxide ion conducting material (Kim et al., 2010; Tadanaga et al., 2010; Furukawa et al., 2011; Kubo et al., 2012, 2013a,b; Sun et al., 2017; Ishiyama et al., 2019). LDHs are anionic clay-like material, and the general formula for LDHs is [ (OH)2][(A n−)x/nÂmH 2O], where MII is a divalent cation such as Mg 2+, Ni 2+, Zn 2+, etc., and MIII is a trivalent cation such as Al 3+, Cr3+, Fe3+, etc., and A n− is an anion such as , Cl −, , etc. The inorganic clay-like material LDHs are expected to have higher durability and thermal stability compared with organic polymer-based AEM (Tadanaga et al., 2010). We reported that LDHs intercalated with showed high hydroxide ion conductivity of the order of 10−3 S cm −1 under 80% relative humidity (Tadanaga et al., 2010; Furukawa et al., 2011; Kubo et al., 2012). We also reported that LDHs can be applied to the solid electrolyte of alkaline-type direct ethanol fuel cells (Tadanaga et al., 2010; Furukawa et al., 2011; Kubo et al., 2012), and aqueous ammonia fuel cell (Ishiyama et al., 2019). To improve the cell performance using LDHs as electrolyte, thin and large area membranes with LDHs must be prepared. However, LDHs are usually obtained as powder, and the formation of thin membrane as a separator of a fuel cell is rather difficult.
On the other hands, in our previous reports, we used a glass paper as a support for thin membrane for fuel cells (Tezuka et al., 2005a,b). Glass paper is non-woven fabric of fine glass fibers with high porosity. We reported that glass paper can be used as a support of proton conductive inorganic-organic hybrid electrolyte (Tezuka et al., 2005a,b). Self-standing membranes with a small thickness and a high mechanical strength were obtained, and improved fuel cell performances were shown.
In the present study, self-standing Mg-Al LDH thin membranes were prepared using a glass paper as a support, and the structure, morphology and electrochemical properties of the membrane were examined. In addition, the H2-O2 fuel cell using the prepared Mg-Al LDH thin membrane as electrolyte was fabricated. The cell performance of the H2-O2 fuel cell using Mg-Al LDH thin membrane as electrolyte and impedance spectra under an operating condition were evaluated.
Mg-Al LDH powder was prepared by using the co-precipitation process. Mg(NO3)2·6H2O and Al(NO3)3·9H2O were dissolved in deionized water in a Mg: Al ratio of 3: 1 (Furukawa et al., 2011). The mixture of 0.3 M Mg(NO3)2 solution and 0.1M Al(NO3)3 solution was dropped into 0.3 M Na2CO3 solution with stirring at 80°C. During dropping of the solution, pH of the reaction mixture was controlled to 10 by using 2 M NaOH solution. The obtained mixture was then aged at 80°C for 17 h. The white precipitates were separated with filtration, washed with distilled water, and dried at 80°C.
Thin Mg-Al LDH membrane was fabricated using the Mg-Al LDH re-dispersed solution and a glass paper. The obtained Mg-Al LDH powder by the co-precipitation method was re-dispersed into distilled water. Then, glass paper provided by Nippon Sheet Glass Co. Ltd., [C-glass (soda-lime-borosilicate glass Cameron and Rapp, 2001)] fibers, nominal thickness of 50 μm, about 90% porosity) was immersed into the Mg-Al LDH re-dispersed solution. Immediately after the most area of the glass paper was immersed, the glass paper was removed from the solution with withdrawal speed of 3 mm s−1, and the obtained glass paper with LDH precipitates was dried at 50°C for 1 h. This immersion-drying procedure was repeated five times because the dense Mg-Al LDH thin membrane was not obtained by the immersion less than five times. Consequently, the crystals were deposited in the inside and surface of the glass paper, and the self-standing Mg-Al LDH thin membranes were obtained. The thickness of the Mg-Al LDH thin membranes was measured by a micrometer with the error of 5% or less. The membranes were pressed from both sides before the conductivity and fuel cell evaluations. Pelletized Mg-Al LDH powder electrolyte was also prepared for comparison, by mixing Mg-Al LDH powder and 5 wt% polytetrafluoroethylene (PTFE) or 10 wt% PTFE as binder for pellet molding. The thickness of Mg-Al LDH thin membrane with the glass paper was about 150 μm and that of pelletized Mg-Al LDH powder was about 250 μm.
Powder X-ray diffraction (XRD) was used to characterize crystalline phases. The electrical conductivities of the pelletized Mg-Al LDH powder and LDH thin membrane were evaluated using impedance data in a frequency range of 1–8 × 106 Hz. The samples were kept for 1 h at each measurement condition in a temperature and humidity-controlled chamber to ensure that samples reached an equilibrium. The pellets of Mg-Al LDH were obtained by cold pressing under 200 MPa. Gold electrodes were sputtered on both sides of the pelletized Mg-Al LDH and the Mg-Al LDH thin membrane. To evaluate the fuel cell performance of the Mg-Al LDH thin membrane as electrolyte, a H2-O2 fuel cell was fabricated using the pelletized Mg-Al LDH powder or Mg-Al LDH thin membrane as electrolyte, as presented in Figure 1. The anode electrode catalyst was prepared by mixing Pt/C (EC-20-10-10; Electrochem Inc.), Mg-Al LDH and PTFE as a binder with a weight ratio of 1:1:0.6. The cathode electrode catalyst was prepared by mixing Pt/C, Ni-Al LDH and PTFE with the same ratio as anode electrode catalyst. Catalysts were loaded on a carbon sheet by painting the catalyst ink. In this study, the loading amount of Pt was 1.9 mg cm−2, the LDH loading amount as ionomer was the same amount as the Pt catalyst. Then, these electrolytes were sandwiched with the prepared catalyst layers on carbon sheet. The cell performance was measured at 60°C by exposing a flow of 25 mL/min humidified H2 gas to anode side and 50 mL/min humidified air to cathode side.
Figure 2 shows XRD pattern of the Mg-Al LDH powder obtained by the coprecipitation method. The XRD pattern shows characteristic 003, 006, 0012, etc. reflections attributed to the layered structure (brucite-like structure) of LDHs, and the formation of Mg-Al LDH single phase was confirmed.
Figure 3A shows cross-sectional scanning electron microscope (SEM) image of the glass paper used in this study. The cross-section of the membranes was obtained by mechanical cutting with a knife. The glass paper is non-woven fabric and consists of glass fibers with a diameter of about 5 μm and 0.5 μm. Figure 3B shows the image of the cross-section of the obtained Mg-Al LDH thin membrane (five-time immersion). Mg-Al LDH particles were confirmed to be deposited on the inside of the glass paper. The deposited Mg-Al LDH crystals in the inside of the glass papers are assumed to be weakly attached with the glass fibers.
Figure 3. Cross sectional SEM images of the glass paper (A) and the obtained Mg-Al LDH membrane (B).
Figure 4 shows surface SEM images of Mg-Al LDH thin membrane with the glass paper support and the pelletized Mg-Al LDH powder. The surface of the pelletized LDH powder (Figure 4B) is rather smooth. This may be because shape and size of LDH crystals are rather easy to be changed by pressing. On the surface of the pelletized sample, the presence of mixed PTFE binder are also confirmed. However, some cracks are present on the surface. On the other hand, in Mg-Al LDH thin membrane (Figure 4A), Mg-Al LDH powder are deposited on glass paper, and LDH hexagonal particles are well-oriented parallel to the plane of glass paper. The characteristic morphology of the Mg-Al LDH thin membrane electrolyte and the pressure applied in the fabrication of membrane-electrolyte assembly for fuel cell is expected to result in dense structure and high gas barrier property.
Figure 4. Surface SEM images of Mg-Al LDH thin membrane (A) and pelletized Mg-Al LDH powder (B) with 10% PTFE binder.
Electrical properties of the pelletized Mg-Al LDH powder and Mg-Al LDH thin membrane were determined using the impedance spectroscopy. The temperature dependence of the ionic conductivities under 80% relative humidity for the pelletized Mg-Al LDH powder and Mg-Al LDH thin membrane is shown in Figure 5. The ionic conductivity of Mg-Al LDH thin membrane is almost the same as that of the pelletized Mg-Al LDH powder. Mg-Al LDH crystals on glass paper seem to be connected each other. Thus, the moderate difference of the conductivity may be because of the decrease of the grain boundary resistance.
Figure 5. Temperature dependence of the ionic conductivity of Mg-Al LDH thin membrane and pelletized Mg-Al LDH powder.
Alkaline-type H2-O2 fuel cells using the pelletized Mg-Al LDH powder with PTFE binder and Mg-Al LDH thin membrane as electrolyte were fabricated. Figure 6 shows the cell performance for the alkaline-type H2-O2 fuel cell under 80% relative humidity. The power density (P = V × I) of the fuel cell is calculated from the measured I-V data and the active area of the electrode. The H2-O2 fuel cell using Mg-Al LDH thin membrane as electrolyte shows open circuit voltage (OCV) of more than 0.9 V, indicating that the thin membrane with well-oriented Mg-Al LDH crystals has high gas barrier property. The H2-O2 fuel cell using Mg-Al LDH thin membrane as electrolyte shows higher cell performance than the cell using pelletized Mg-Al LDH powder. The maximum power density of the H2-O2 fuel cell using Mg-Al LDH thin membrane is about 5 times higher than that using pelletized Mg-Al LDH powder. The effect of PTFE content in the pelletized electrolyte on the cell performance is much smaller at the same experiment. Therefore, the improvement of the cell performance must be because of the smaller thickness in the Mg-Al LDH thin membrane. The better performance should mainly be attributed to the lower resistance of the electrolyte.
Figure 6. Performance of H2-O2 fuel cells using Mg-Al LDH thin membrane (without PTFE) using the glass paper as support medium, and Mg-Al LDH pellet containing 5, 10 wt.% PTFE as a binder.
For detail analysis of the high cell performance using Mg-Al LDH thin membrane as electrolyte, the impedance spectra under the cell working condition were measured. Figure 7 shows the impedance plot under the cell working condition. The intercept in high frequency range is electrolyte resistance because this value is almost proportional to the thickness of the electrolytes. The circle in low frequency range is attributable to the overlap of activation overpotential and concentration overpotential (Springer et al., 1996; Yuan et al., 2007). The impedance plot of H2-O2 fuel cell using Mg-Al LDH thin membrane as electrolyte shows that electrolyte resistance is small compared to the pelletized Mg-Al LDH powder. The circle in the H2-O2 fuel cell using Mg-Al LDH thin membrane as electrolyte in low frequency range is rather small, indicating that the concentration overpotential is also lowered.
Figure 7. Nyquist plots of H2-O2 fuel cells using Mg-Al LDH thin membrane using the glass paper as support medium and Mg-Al LDH pellet containing 5, 10 wt.% PTFE as a binder at 0.6 V.
The glass paper is proved to be very effective support for making thin electrolyte membranes for fuel cells. Thin membrane with smaller thickness or larger area can be prepared by using an appropriate glass paper. This procedure can also be applied to a separator of other all solid-state electrochemical cells, such as all solid state lithium batteries, by using powder of solid electrolytes.
Self-standing Mg-Al LDH thin membranes were prepared using a glass paper as support. The H2-O2 fuel cell with using the Mg-Al LDH thin membrane as the separator showed high OCV, indicating that Mg-Al LDH thin membrane has high gas barrier property. The H2-O2 fuel cell showed higher cell performance compared with the H2-O2 fuel cell using the pelletized Mg-Al LDH powder. These results indicate that the glass paper is very effective support for making thin Mg-Al LDH electrolyte membrane.
All datasets generated for this study are included in the article/supplementary material.
DK performed experiments and wrote draft of this manuscript. KT got financial support, supervised experiments, and edited the manuscript. AH supported electrochemical experiments. MT supervised all this research.
The present work was partly supported by A-STEP program by Japan Science and Technology Agency (JST).
The authors declare that the research was conducted in the absence of any commercial or financial relationships that could be construed as a potential conflict of interest.
The authors acknowledge Nippon Sheet Glass Co. Ltd., for providing glass paper.
Adams, L. A., Poynton, S. D., Tamain, C., Slade, R. C. T., and Varcoe, J. R. (2008). A carbon dioxide tolerant aqueous-electrolyte-free anion-exchange membrane alkaline fuel cell. Chem. Sus. Chem. 1, 79–81. doi: 10.1002/cssc.200700013
Cameron, N. M., and Rapp, C. F. (2001). Fiberglass, Encyclopedia of Materials: Science and Technology. Elsevier: Pergamon. 3142–3146. https://doi.org/10.1016/B0-08-043152-6/00558-1
Cheng, J., He, G., and Zhang, F. (2015). A mini-review on anion exchange membranes for fuel cell applications: stability issue and addressing strategies. Int. Hydrogen Energy J. 40, 7348–7360. doi: 10.1016/j.ijhydene.2015.04.040
Fujiwara, N., Yao, M., Siroma, Z., Senoh, H., Ioroi, T., and Yasuda, K. (2011). Reversible air electrodes integrated with an anion-exchange membrane for secondary air batteries. Power Sources J. 196, 808–813. doi: 10.1016/j.jpowsour.2010.07.074
Furukawa, Y., Tadanaga, K., Hayashi, A., and Tatsumisago, M. (2011). Evaluation of ionic conductivity for Mg-Al layered double hydroxide intercalated with inorganic anions. Solid State Ionics 192, 185–187. doi: 10.1016/j.ssi.2010.05.032
Gultekin, S., Alsaleh, M., and Alzakri, A. (1994). Effect of CO impurity in H2 on the performance of Ni/PTFE diffusion electrodes in alkaline fuel cells. Int. Hydrogen Energy J. 19, 181–185. doi: 10.1016/0360-3199(94)90125-2
Gulzow, E., and Schulze, M. (2004). Long-term operation of AFC electrodes with CO2 containing gases. Power Sources J. 127, 243–251. doi: 10.1016/j.jpowsour.2003.09.020
Ishiyama, S., Rosero-Navarro, N. C., Miura, A., and Tadanaga, K. (2019). Mg-Al layered double hydroxide as an electrolyte membrane for aqueous ammonia fuel cell. Mater. Res. Bull. 119:110561. doi: 10.1016/j.materresbull.2019.110561
Kim, H. S., Yamazaki, Y., Kim, J.D, Kudo, T., and Honma, I. (2010). High ionic conductivity of Mg-Al layered double hydroxides at intermediate temperature (100-200 oC) under saturated humidity condition (100% RH). Solid State Ionics 181, 883–888. doi: 10.1016/j.ssi.2010.04.037
Kruusenberg, I., Matisen, L., Shah, Q., Kannan, A. M., and Tammeveski, K. (2012). Non-platinum cathode catalysts for alkaline membrane fuel cell. Int. Hydrogen Energy J. 37, 4406–4412. doi: 10.1016/j.ijhydene.2011.11.143
Kubo, D., Tadanaga, K., Hayashi, A., and Tatsumisago, M. (2012). Hydroxide ion conduction in Ni-Al layered double hydroxide. J. Electroanal. Chem. 671, 102–105. doi: 10.1016/j.jelechem.2012.02.007
Kubo, D., Tadanaga, K., Hayashi, A., and Tatsumisago, M. (2013a). Improvement of electrochemical performance in alkaline fuel cell by hydroxide ion conducting Ni-Al layered double hydroxide. Power Sources J. 222, 493–497. doi: 10.1016/j.jpowsour.2012.08.093
Kubo, D., Tadanaga, K., Hayashi, A., and Tatsumisago, M. (2013b). Multifunctional inorganic electrode materials for high-performance rechargeable metal-air batteries. J. Mater. Chem. A 1, 6804–6809. doi: 10.1039/c3ta10440k
Matsuoka, K. Y., Iriyama, A. T., Matsuoka, M., and Ogumi, Z. (2005). Alkaline direct alcohol fuel cells using an anion exchange membrane. Power Sources J. 150, 27–31. doi: 10.1016/j.jpowsour.2005.02.020
Merle, G., Wessling, M., and Nijmeijer, K. (2011). Anion exchange membranes for alkaline fuel cells: a review. J. Membr. Sci. 377, 1–35. doi: 10.1016/j.memsci.2011.04.043
Serov, A., Zenyuk, I., Arges, C., and Chatenet, M. (2018). Hot topics in alkaline exchange membrane fuel cells. Power Sources J. 375, 149–157. doi: 10.1016/j.jpowsour.2017.09.068
Spendelow, J. S., and Wieckowski, A. (2007). Electrocatalysis od oxygen reduction and small alcohol oxidation in alkaline media. Phys. Chem. Chem. Phys. 9, 2654–2675. doi: 10.1039/b703315j
Springer, T. E., Zawodzinski, T. A., Wilson, M. S., and Gottesfeld, S. (1996). Characterization of polymer electrolyte fuel cells using AC impedance spectroscopy. J. Electrochem. Soc. 143, 587–599. doi: 10.1149/1.1836485
Strasser, K. (1990). The design of alkaline fuel cells. Power Sources J. 29, 149–166. doi: 10.1016/0378-7753(90)80016-7
Sun, Z., Ma, R. Z., Bai, X. Y., Wang, K. L., Zhu, H. W., and Sasaki, T. (2017). Single-layer nanosheets with exceptionally high and anisotropic hydroxyl ion conductivity. Sci. Adv. 3:e1602626. doi: 10.1126/sciadv.1602629
Tadanaga, K., Furukawa, Y., Hayashi, A., and Tatsumisago, M. (2010). Direct ethanol fuel cell using hydrotalcite clay as a hydroxide ion conductive electrolyte. Adv. Mater. 22, 4401–4403. doi: 10.1002/adma.201001766
Tezuka, T., Tadanaga, K., Matsuda, A., Hayashi, A., and Tatsumisago, M. (2005a). Utilization of glass papers as a support for proton conducting inorganic–organic hybrid membranes from 3-glycidoxypropyltrimethoxysilane, tetraalkoxysilane and orthophosphoric acid. Solid State Ionics 176, 3001–3004. doi: 10.1016/j.ssi.2005.09.039
Tezuka, T., Tadanaga, K., Matsuda, A., Hayashi, A., and Tatsumisago, M. (2005b). Utilization of glass paper as a support of proton conductive inorganic–organic hybrid membranes based on 3-glycidoxypropyltrimethoxysilane. Electrochem. Commun. 7, 245–248. doi: 10.1016/j.elecom.2005.01.009
Keywords: glass paper, layered double hydroxide, alkaline fuel cells, hydroxide ion conductivity, electrolyte membrane
Citation: Kubo D, Tadanaga K, Hayashi A and Tatsumisago M (2020) Fabrication of Mg-Al Layered Double Hydroxide Thin Membrane for All-Solid-State Alkaline Fuel Cell Using Glass Paper as a Support. Front. Mater. 7:117. doi: 10.3389/fmats.2020.00117
Received: 21 August 2019; Accepted: 15 April 2020;
Published: 30 April 2020.
Edited by:
Maria Jesus Pascual, Consejo Superior de Investigaciones Científicas (CSIC), SpainReviewed by:
Stefan Karlsson, RISE Research Institutes of Sweden, SwedenCopyright © 2020 Kubo, Tadanaga, Hayashi and Tatsumisago. This is an open-access article distributed under the terms of the Creative Commons Attribution License (CC BY). The use, distribution or reproduction in other forums is permitted, provided the original author(s) and the copyright owner(s) are credited and that the original publication in this journal is cited, in accordance with accepted academic practice. No use, distribution or reproduction is permitted which does not comply with these terms.
*Correspondence: Kiyoharu Tadanaga, dGFkYW5hZ2FAZW5nLmhva3VkYWkuYWMuanA=
Disclaimer: All claims expressed in this article are solely those of the authors and do not necessarily represent those of their affiliated organizations, or those of the publisher, the editors and the reviewers. Any product that may be evaluated in this article or claim that may be made by its manufacturer is not guaranteed or endorsed by the publisher.
Research integrity at Frontiers
Learn more about the work of our research integrity team to safeguard the quality of each article we publish.