- 1Dipartimento di Meccanica, Matematica e Management, Politecnico di Bari, Bari, Italy
- 2Dipartimento di Medicina Sperimentale e Clinica, Università di Foggia, Foggia, Italy
Invisible aligners are medical devices, which allow repositioning of teeth through a treatment designed by the orthodontists. During this orthodontic treatment, patients use several aligners each for a couple of weeks. The aligner will apply a system of forces on the teeth to shift them to desired position. Since aligners exert forces thanks to their particular shape, it is important that during lifetime’s service they do not undergo significant deformations. This research aims to study the mechanical behavior of invisible aligners made by polyethylene terephthalate-glycol (PET-G), which is one of most used the plastic materials to produce such devices. In this study, cyclic compression tests in atmospheric environment (∼25°C) as well as in the presence of saliva (to simulate intraoral environment) were performed. The mechanical behavior of aligners with two different thicknesses (0.75 and 0.88 mm) was studied. In particular, each aligner was subjected to 22500 load cycles from 0 to 50 N. The chosen number of load cycles simulates the average load history to which an aligner is subjected during its lifetime. The tests were performed on a testing machine, using a hard resin dental cast properly fixed to the machine. Analysis of the results shows that the stiffness of the aligners increases during the cyclic test. In particular, a gradual reduction of the crosshead displacement was observed during the test, highlighting the occurrence of cyclic hardening phenomena. It was also found that the aligners show a residual strain recovery after removing the applied load. Moreover, in the analyzed range of load rate, the aligners show a low tendency to accumulate residual strains as loading cycles progress.
Introduction
Polyethylene terephthalate-glycol (PET-G) is one of the most famous and used thermoplastic materials in the world. In particular, it is widely used in many applications such as displays, vending machine windows, food packaging, cosmetics and electronics. This material is an amorphous copolymer of PET but, unlike this one, it is not able to undergo strain-induced crystallization independently of the stretching rates and temperatures used during processing (Dupaixa and Boyce, 2005; Elkholy et al., 2019). Due to the favorable combination of excellent esthetic characteristics, formability, high mechanical strength, excellent flexibility and high impact resistance (Sheridan et al., 1994; Sheridan and Armbruster, 1999; Oh and Wang, 2007; Lloyd et al., 2001), this material has gained great interest in the last decade in the medical device industry. In fact, it is adopted for manufacturing orthodontic retainers, temporomandibular joint splints, periodontal splints, and mouth guards (Ryokawa et al., 2006; Fang et al., 2013; Ma et al., 2016). At the same time, its excellent transparency makes PET-G copolymer promising in manufacturing removable tooth aligners for orthodontics (Ponitz, 1971; McNamara et al., 1985; Lindauer and Shoff, 1998). Orthodontic aligners allow repositioning of teeth through a treatment designed by the orthodontists. During this orthodontic treatment, the patients wear several aligners, each of them for about 2 weeks. Aligners are typically removed only during eating and teeth brushing (Jeremiah et al., 2010). The aligner will apply a system of forces on the teeth to shift them to the desired position. As the teeth move, the forces applied by the aligner decrease as a consequence of the shifting of the teeth. Since aligners exert forces thanks to their particular shape, it is important therefore that during lifetime’s service they do not undergo significant deformations (Kesling, 1945; Nahoum, 1964; Ponitz, 1971; Pohl and Yoshii, 1994; Boyd et al., 2006). Generally, a number of factors may contribute to affect the deformation of the thermoplastic materials under loading. These may include environmental conditions (i.e., temperature and humidity), thickness of the aligner, time after elastic deformation and forming procedures features (Landel and Nielsen, 1993; Ryokawa et al., 2006).
Several studies have looked at the effect of different environmental conditions on the mechanical behavior of thermoplastic materials used for invisible aligners. These studies have shown that the mechanical properties differ depending on the environmental factors and that they are influenced by molecular structure and orientation (Ryokawa et al., 2006). In particular, under wet conditions, the water molecules are able to penetrate into the polymer chain or to form hydrogen bonding with the hydrogen end group thereby altering the free volume and mobility of neighboring polymer chains. In addition, water in the polymer matrix may attack the hard and soft-segment domains (Scaffaro et al., 2008; Rajeesh et al., 2010; Xu and Muldowney, 2015; Kim et al., 2016). Moreover, since PET-G is a thermoplastic material with highly viscoelastic properties, the stress induced by initial deformation affects severely the co-polymer’s mechanical properties (Tricca and Li, 2006). This phenomenon is known as stress relaxation. In (Fang et al., 2013) the dynamic stress relaxation of a variety of commercial orthodontic thermoplastic materials was studied including PET-G and PET. It was observed that hygrothermal environment can significantly accelerate the stress relaxation of these materials. Moreover, the residual stress within all materials decreases with time. In (Lombardo et al., 2017) the relaxation behavior during a 24-h loading time was evaluated during a three-point-bending test. It was observed that PET-G specimens show stress relaxation value of 44% after the first 8 h, reaching a value of 62% after a 24-h loading period.
Other experimental studies report results from tensile tests or uniaxial and plane strain compression tests conducted over a wide range of temperatures and strain rates (Eliades and Bourauel, 2005; Kwon et al., 2008; Pascual et al., 2010; Zhang et al., 2011; Elkholy et al., 2019).
Nowadays the literature is very poor of experimental data on the cyclic deformation behavior of thermoplastic materials, despite of this it is well known that the cyclic stress-strain behavior of polymers is not the same as that of static loading. The investigation of the cyclic deformation behavior is a very important topic for a correct design of aligners made by these materials. The cyclic deformation behavior was investigated experimentally in Kizypow and Rimnac (2000) and in Ariyama (1993, 1996), respectively, for the ultra-high-molecular-weight polyethylene (UHMWPE) and for PET. In particular, regarding PET polymer, also the effects of strain rate, number of cycles, mean strain, and strain amplitude on the constitutive curves were investigated.
Unfortunately, to date, the response of PET-G co-polymer under cyclic loading has not been investigated.
Therefore, this study presents the experimental results of compression cyclic tests performed on PET-G invisible aligners. Each aligner was subjected to 22500 loading cycles ranging from 0 to 50 N. The number of load cycles was chosen to simulate the average load history to which an aligner is subjected normally during its lifetime. In particular, aligners with two different thicknesses (0.75 and 0.88 mm) were produced and analyzed. The tests have been conducted in atmospheric environment (∼25°C) and in presence of artificial saliva to simulate intraoral environment. The effects of thicknesses and environmental conditions on the mechanical behavior of the co-polymer have been analyzed. A better comprehension of the mechanical behavior of the aligners at room temperature and under simulated intraoral conditions will be helpful for a better product design.
Materials and Methods
Specimen Preparation
An intraoral scan (TRIOS-3Shape) was performed on a patient with a moderate crowding of upper and lower incisors. This scan has been used to generate a STL file, using the 3Shape OrthoAnalyzer® software (TRIOS-3Shape). Subsequently the STL file was used to 3D print a resin cast (Daylight Hard Resin, Photocentric Ltd.) by means of a Liquid Crystal HR2 3D printer (Photocentric Ltd.).
The aligners specimens were produced from PET-G discs with two different nominal thicknesses: 0.75 and 0.88 mm. In particular the PET-G was a Ace® Plastic produced by GAC International. Its composition was as it follows: 95% copolyester and 5% trade secret. Starting from these transparent disks the aligners were thermoformed using the Essix® SelectVac vacuum machine. The Essix® SelectVac vacuum machine used to fabricate the invisible aligners of different thicknesses is shown in Figure 1. Before testing, the aligners were stored at room temperature (approximately 25°C) and indoor humidity (ambient conditions) for 24 h while saliva was added just a few minutes before starting the test.
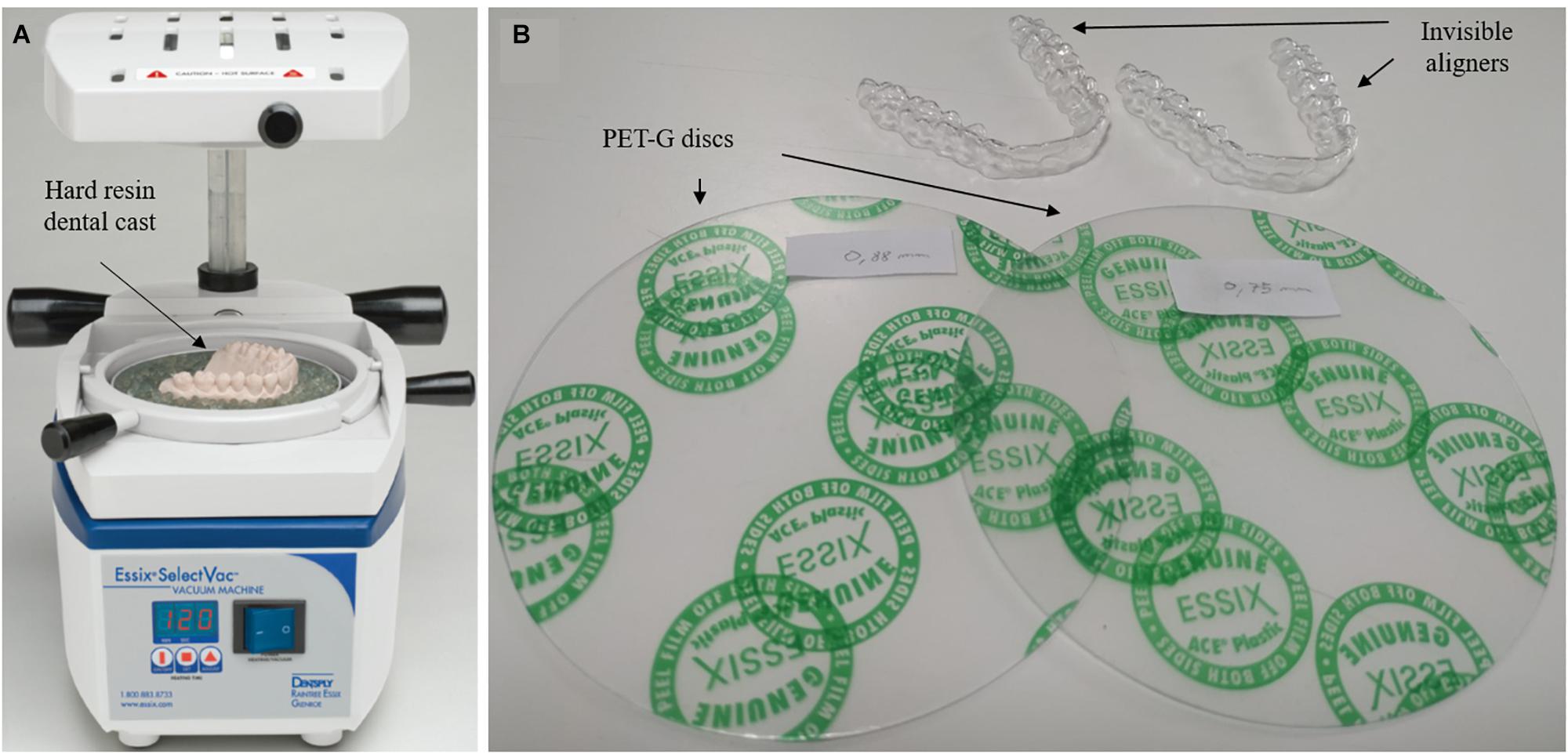
Figure 1. (A) Essix® SelectVac vacuum machine used for fabricated the invisible aligners and (B) PET-G discs used and specimen produced.
Compression Cyclic Testing
The compression cyclic tests were performed by using a model 3344 single column universal testing machine produced by Instron (Norwood, MA, United States) equipped with a 1 kN loading cell. The loading machine is directly controlled by a PC through the Instron Bluehill software which was programmed in order to define the desired test procedure (e.g., features of the cycle and number of the cycles). The hard resin dental casts were gripped by flat face hydraulic jaws at a pressure of 10 MPa. During assembling of the dental casts, particular attention was paid to ensure that they were perfectly lined up one above the other and perpendicular to the direction of application of the load. The invisible aligner was placed on the upper dental cast and then casts were put in occlusion obtaining the contact between the upper and lower dental cast. For the case of tests in saliva they were performed by impregnating a thin sponge with artificial saliva. Sponge was preliminary cut in order to follow the shape of the dental cast. In such a way it was possible to insert the cast and the over imposed aligners inside the sponge. A thin transparent film of cellulose hydrate was used to envelope the entire system in order to preserve humidity of the system and avoid fast evaporation.
Figure 2 illustrates the PET-G specimen undergoing to the cyclic compression test. The tests were performed under load control mode at constant cyclic load frequency f = 0.25 cycles/s. The applied load history is schematized in Figure 3. Figure 3 shows that each loading cycle is constituted by four stages each one lasting 1 s. In the first stage the load increases from 0 to 50 N. That maximum load is maintained for 1 s (stage 2) and then the descending ramp starts down to 0 N (stage 3). Finally, the sample is kept at 0 N for 1 s (stage four) and then a new cycle starts. Compression cycles were repeated 22500 times, distributed over 4 days in order to simulate also the non-loading time corresponding to the phases when the aligner is not used. The chosen number of load cycles simulates the average load history to which an aligner is subjected normally during its lifetime. The number of cycles and ramp duration was calculated on the basis of the average number of daily dental contacts during the swallowing act (1500 swallowing act) and the time of swallowing act (1 s).
Samples with two different thicknesses (0.75 and 0.88 mm) were tested in atmospheric environment (∼25°C). Furthermore, tests in presence of artificial saliva and at room temperature were performed to understand better the mechanical behavior of PET-G aligner within the oral environment. To this scope, commercially available artificial saliva was used whose composition is reported in Table 1.
Three samples for each test condition and for each thickness were tested. Average values and standard deviations were calculated for each analyzed point. The total energy and stiffness were calculated to compare their behaviors. In particular, total energy was determined by integrating the load-displacement hysteresis loops while the stiffness was determined as the slope of a linear regression fitting the loops.
Results and Discussion
The evolution of the load–displacement hysteresis loops (ratcheting displacement) for each specimen tested at both atmospheric environment (∼25°C) and at presence of artificial saliva is shown in Figure 4. The data reported in the figure are referred to the first day of cyclic loading. Similar ratchetting behavior can be found on other days.
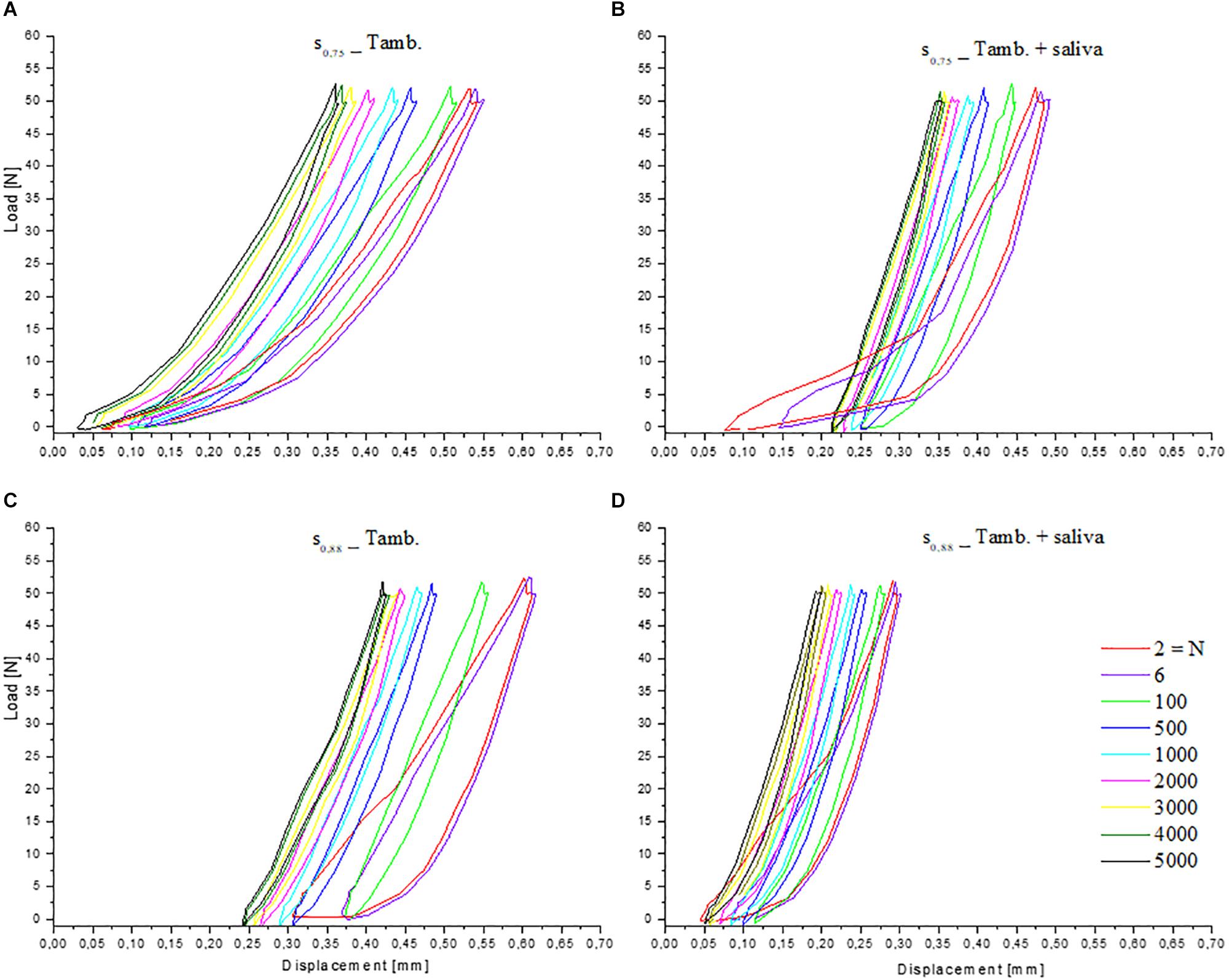
Figure 4. Evolution of the load–displacement loops for each sample tested under different environmental conditions. 0.75 mm sample tested (A) in atmospheric environment (∼25°C) and (B) in presence of artificial saliva. 0.88 mm sample tested (C) in atmospheric environment (∼25°C) and (D) in presence of artificial saliva. The serial number of the compression cycle (N) increases from right to left.
In Figures 4A–D it is possible to observe that the two samples tested at both atmospheric environment and with artificial saliva follow analog trends. As it can be observed, the hysteresis loops of two samples are shifted to the left (lower displacements) during the cyclic compression testing. Furthermore, it is noted that, for the sample of 0.75 mm thick (s0.75) tested at atmospheric environment (Figure 4A), the hysteresis loops are almost non-linear in shape during the entire cycling process. This behavior is similar that observed in Shen et al. (2004) for the case of the hardened epoxy resin Epon 826/Epi-Cure Curing Agent 9551. While, for the s0.75 sample tested in presence of artificial saliva the hysteresis loops exhibit, initially, some non-linear response, but with the increase number of cycles (N) the hysteresis loops become slimmer and more linear (Figure 4B). The 0.88 mm thick samples (s0.88) tested with and without saliva display similar behavior (Figures 4C,D). In fact, it can be observed that the hysteresis loops in the high cycle region become slightly stiffer while the corresponding irreversible work decreases.
Moreover, it was observed that the ratcheting displacement reduction was greater in the first cycles than in the final ones. In general, above the 1000 cycles the displacement reduction tends to stabilize (Figure 4). The s0.75 sample both at atmospheric environment and with artificial saliva displays in the low cycle region (between 2 and 1000 cycles), a decrease in the ratcheting displacement of about 20%. The same level of displacement reduction was also observed for the s0.88 sample both with or without artificial saliva.
In order to assess the viscoelastic nature and explain the mechanical behavior of aligners tested at environmental ambient and with artificial saliva, the stiffness and the viscoelastic energy loss per cycle were determined. Figures 5 and 6 show, respectively, the relative stiffness defined as the stiffness per unit length multiplied by the resistant area, and the energy as a function of the number of cycles (N). In particular, in Figure 5 it can be noted an abrupt increase in stiffness in the aligners in the low cycle region (during the first 1000 cycles), after that the stiffness values remained almost constant. The maximum stiffness value obtained was 400 N/mm. This trend was observed for both the thicknesses, for both the testing conditions and for all days of cyclic loading (Figure 5). A possible interpretation of this stiffening effect could be connected with molecular orientation and/or polymeric chain entanglement (network) which takes place as the compression test progresses (Schrauwen, 2003; Dupaixa and Boyce, 2005). In addition, another effect that contributes to increase the stiffness of the aligners can be connected with the stress generated during cyclic mechanical compression. In fact, stresses in aligners tend to accumulate during fatigue. As a consequence, stress level rises throughout the test, making the aligners at the same time stiffer and less viscoelastic. Finally, also the absorption of water molecules through humidity in the air or immersion in water may alter the behavior of the aligner during compression loading (Ryokawa et al., 2006).
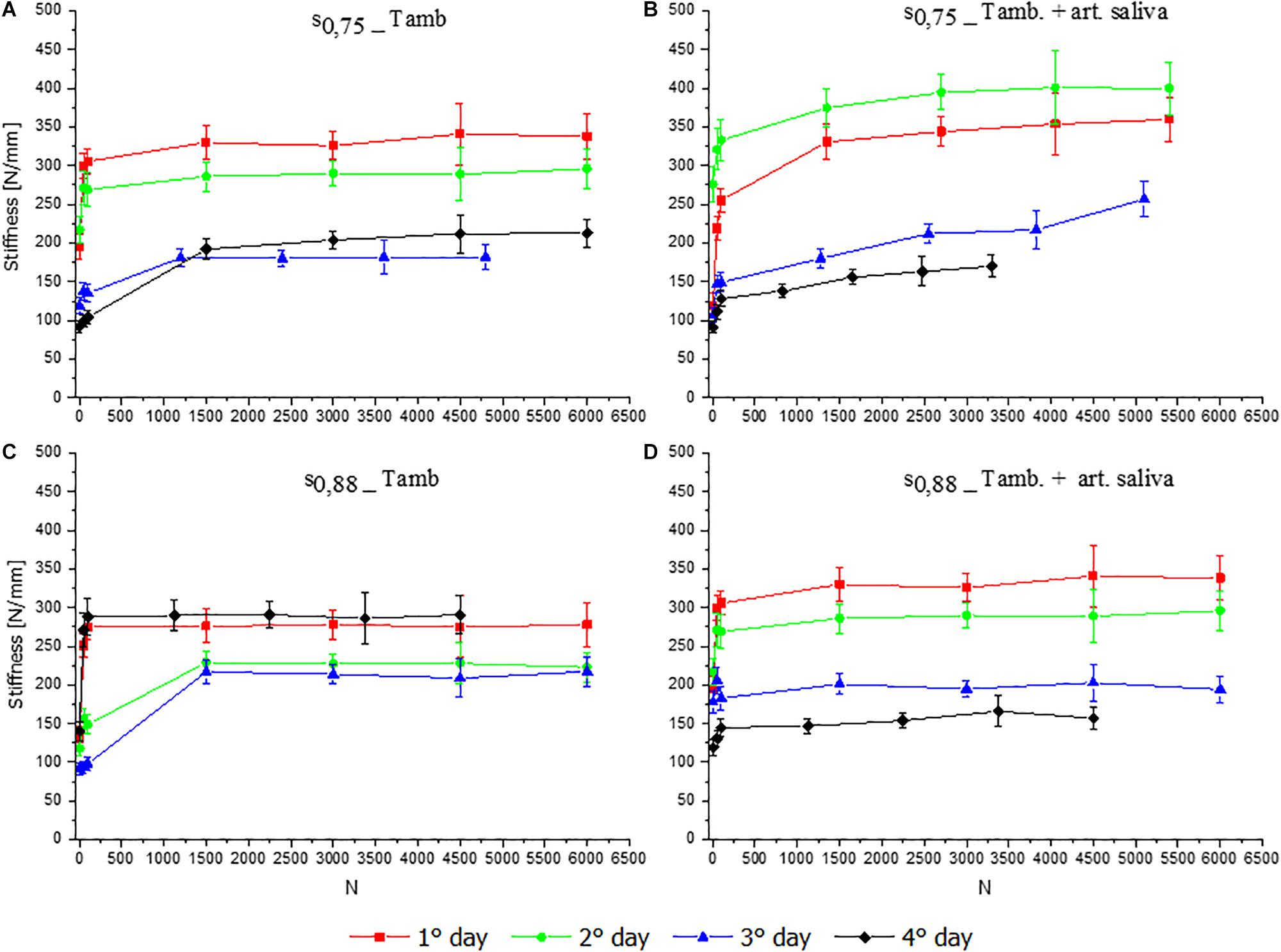
Figure 5. Relative stiffness vs. number of cycles for the two copolymer thicknesses: (A) 0.75 mm sample tested in atmospheric environment (∼25°C), (B) 0.75 mm sample tested in presence of artificial saliva and at room temperature, (C) 0.88 mm sample tested in atmospheric environment (∼25°C), and (D) 0.88 mm sample tested in presence of artificial saliva and at room temperature.
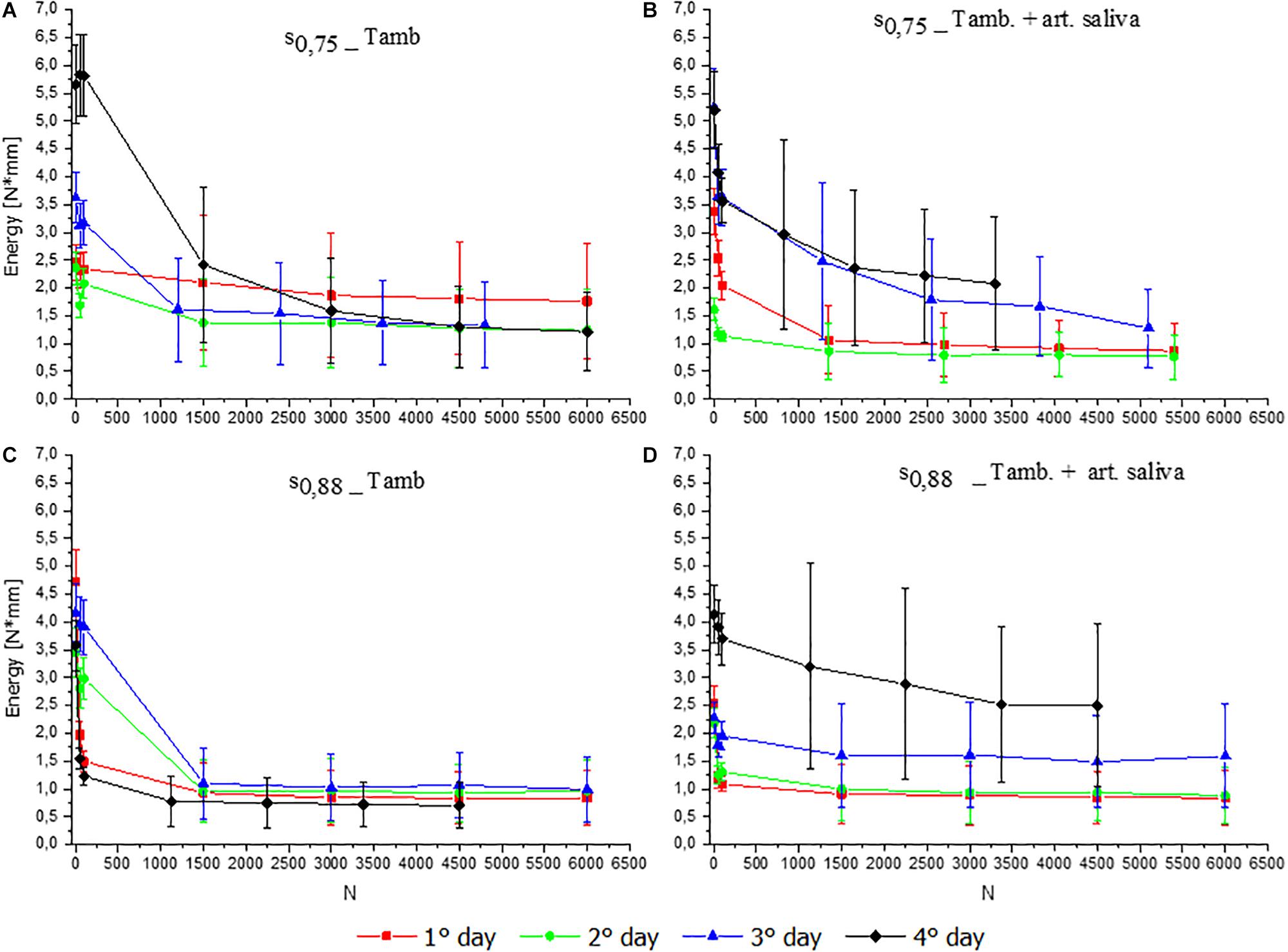
Figure 6. Variation of hysteresis loop energy vs. number of cycles for the two copolymers thicknesses. 0.75 mm sample tested (A) in atmospheric environment (∼25°C) and (B) in presence of artificial saliva and at room temperature. 0.88 sample mm tested (C) in atmospheric environment (∼25°C) and (D) 0.88 mm sample tested in presence of artificial saliva and at room temperature.
By comparing the behavior of s0.75 and s0.88 samples tested in presence of artificial saliva with those tested at atmospheric environment, two observations are possible. The first is that during the compression loading the increase of stiffening of samples tested in presence of saliva was higher with respect to the same samples tested at atmospheric environment. The increase of stiffness observed is, in fact, also due to the absorption of the saliva (compare Figures 5A,B and Figures 5C,D). Such water absorption can produce not only an expansion of the aligner but it can contributes to a change in the molecular orientation (Ryokawa et al., 2006). At the same time, the authors believed that subsequent water molecules absorption or binding into material makes the overall structure more rigid due to modification (reduction) of the free volume. This phenomenon is particularly evident at the beginning of the test in the first day of loading, when the level of saliva absorbed is poor, and tends to increase along the test contributing to increase the stiffness.
A second observation can be done for the case of samples tested in presence of saliva. In this case, in fact, a more marked difference, in average, between the stiffening experienced at the end of the previous test and that shown at the beginning of the next one (Figures 5B,D) can be deduced if these data are compared with those obtained in absence of saliva. This is due to a stress relaxation effect during unloading time (time between two subsequent days of testing). This indicates that a hygrothermal environment, such as that connected with the presence of saliva, can accelerate stress relaxation of aligners (Fang et al., 2013). In other words, the aligners tested in presence of saliva display higher capability to recover the local stress, after load removal, with respect to samples tested at environmental ambient.
Moreover, as already evidenced by the hysteresis response (Figure 4) of the aligners, the energy (irreversible work) decreases as the cycles increase (Figure 6). This trend was observed for the two thicknesses both in presence and without saliva even if, for the case of s0.88 in presence of saliva higher data deviation is observed that would require more consistent statistic to be confirmed. The initial higher value of energy can be attributed to the progressive accumulation of stress inside the material as observed before. This effect is high in the initial parts of the test and tends to stabilize while the loading cycles increase. As a consequence, the accumulated energy tends to reduce. In the non-exercise time between two subsequent days of testing, stress recovery was observed and, as consequence, the amount of energy dissipation is observed to increase again in the first cycles of a new session of cyclic compression test.
To understand better the relative ratcheting displacement of the aligners when the tests are conducted both at atmospheric environment (∼25°C) and with artificial saliva the maximum displacement was calculated. Figure 7 shows the relationship between the maximum displacement and the number of cycles (N) for the two thicknesses tested under the same conditions, throughout the 4 days. In close accordance with the above presented results (Figure 5), the maximum displacement decreases with increasing N (Figure 7). This trend was observed for both the thicknesses, for both the testing conditions, and throughout the 4 days. The displacement reduction is directly related to the stiffening of aligners. In addition, it can be observed that the displacement at the first cycle of a new test session is always greater than that at the last cycle of the previous day (Figures 7A–D) moreover, the displacement values (for the same N) recorded during a test day are always lower than those of the previous day (Figures 7A,B) if tests in absence of saliva are considered. Hence, the data indicate that after load removal (unloading time: time between 1 day and the next day) the aligners recover a part of the displacement because of their viscoelasticity. So, when the aligner is completely unloaded it can recover from the loading cycle.
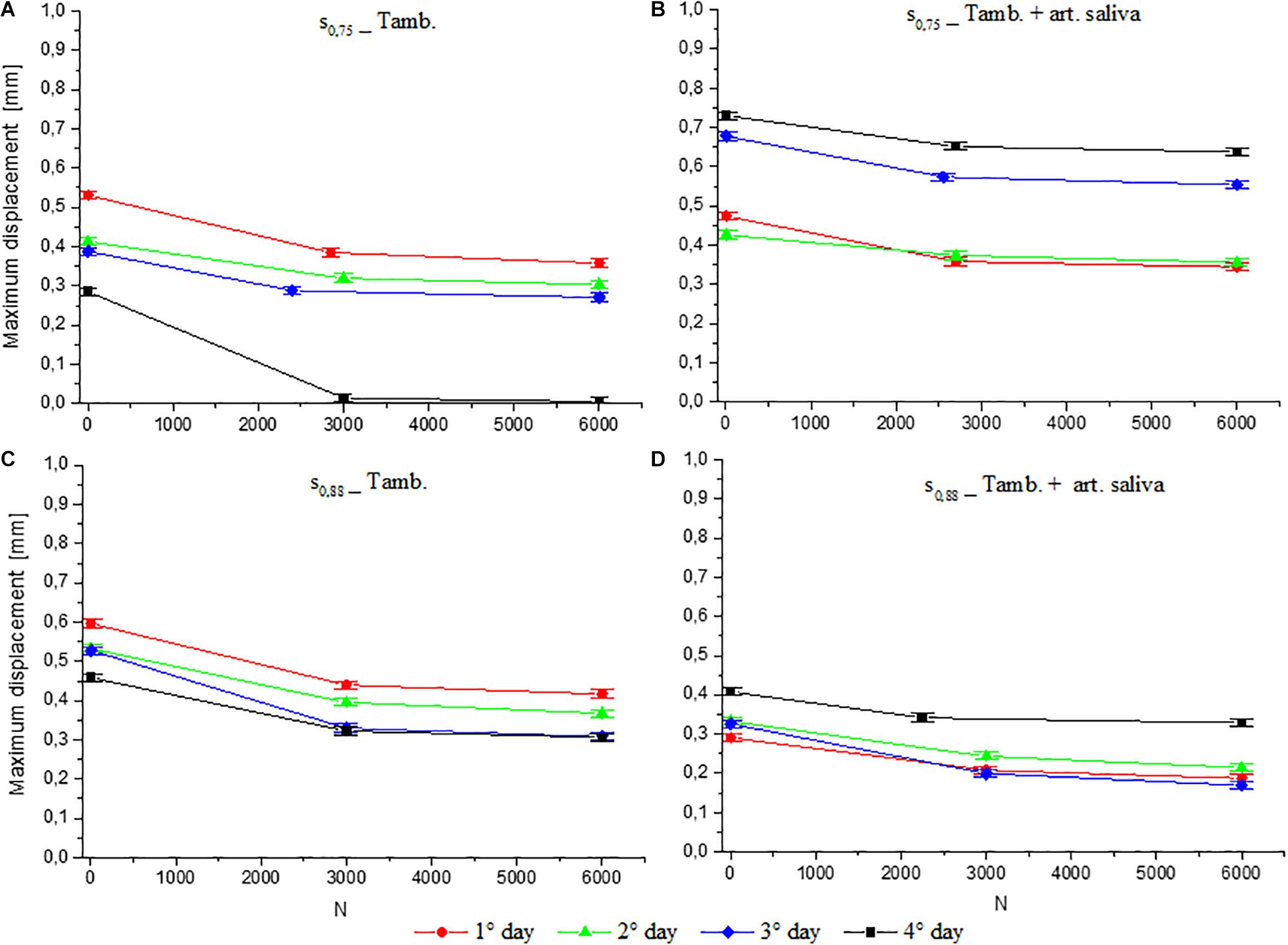
Figure 7. Relationship between maximum displacement and number of cycles of two sample: (A) 0.75 mm sample tested in atmospheric environment (∼25°C), (B) 0.75 sample tested in presence of artificial saliva and at room temperature, (C) 0.88 mm sample tested in atmospheric environment (∼25°C), and (D) 0.88 mm sample tested in presence of artificial saliva and at room temperature.
During cyclic compression loading in presence of artificial saliva, aligners exhibit extensive recovery (Figures 7B,D). Indeed, it may be observed that increased the displacement recovery occurs, between 1 day and the next one, due to the stress relaxation of aligners. At the same time, the hygrothermal environment contributes to stress relaxation even during the loading cycles within of the same day. As a result, only a small amount of displacement reduction was observed with increase of N for each day. Our results, therefore, indicate that samples tested in presence of saliva undergo major deformations in the last days of loading unlike of same tested at environmental ambient. Of course, the residual stress win aligners tested with saliva decrease with time (Figure 7B) and this confirms higher displacement during the last days (Ryokawa et al., 2006).
Figure 8 depicts the displacement variation as a function of fraction of compression test life (N/Nfinal) for the two copolymer thicknesses tested under the same conditions. In particular, the displacement variation along the different days was calculated as the difference between the displacement value of the first cycle of a new test session and that recorded at the end of the previous test session. An increase of displacement variation indicates an increase of deformation recovery. Therefore, it was found that displacement recovery of s0.75 sample tested in ambient environmental was greater in halfway of compression test life, after which the polymer is not able to recover the displacement due to the high level of accumulated stress (Figure 8A). While, for s0.88 tested under the same conditions (Figure 8B), a constant deformation recovery for each interval in which the load is removed was observed, indicating that a greater thickness allows a more uniform stress distribution, and consequently a slower loss of viscoelastic nature. Finally as it was previously observed the presence of saliva increases displacement recovery, expressing a stress relaxation effect (Figures 8A,B) which is not reduced during the test.
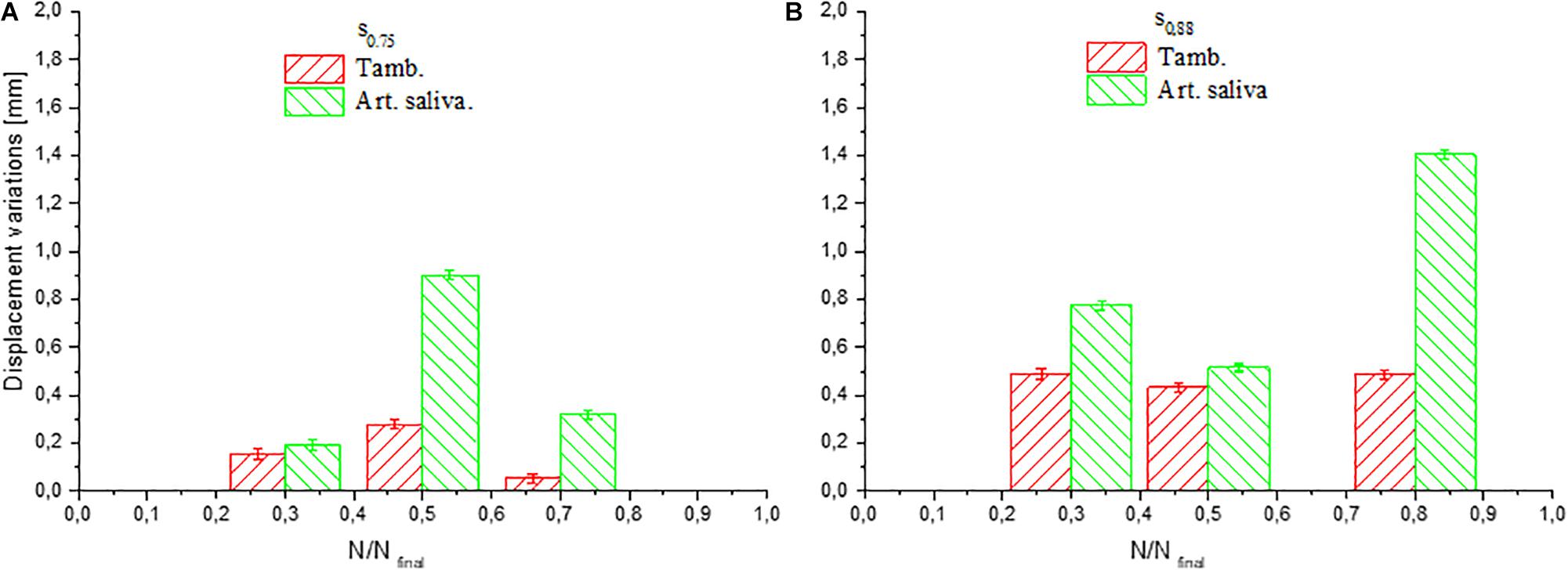
Figure 8. Displacement variation among the different days for the two PET_G copolymer thickness: (A) sample of 0.75 mm thick tested in both atmospheric environment (∼25°C) and in presence of artificial saliva and (B) sample of 0.88 mm thick tested in both atmospheric environment (∼25°C) and in presence of artificial saliva. (N/Nfinal = fraction of compression test life).
Conclusion
The compression behavior under cyclic loading of PET_G invisible aligners was studied for two different thickness and two different testing conditions: atmospheric environment (∼25°C) and in presence of artificial saliva. It was observed for the first day of testing of the aligners in the four different configurations that stiffening effects occur while cyclic loading progresses. It was also observed that stiffening decreases during the non-loading time between two successive sessions of test while stiffening effects are observed again when a new set of cycles is applied to the aligners. Moreover, a work dissipative effect is also observed during cycling loading, this is more pronounced at the beginning of the test while it tends to reduce and stabilize while the test progresses. Also in this case, the time elapsed during two subsequent sessions is observed to contribute to increase again the work dissipative effect in the first cycles of the new session of loadings. With reference to the comparison between the test performed at atmospheric environment and those performed in presence of saliva, it was observed that, in this last case, an higher stress recovery is observed between two subsequent loading sessions; moreover, the hygrothermal environment showed a contribution to reduce the stress accumulation effect during the test. In addition, with reference to the comparison between the two thicknesses, it was observed a reduction in the capability to recover accumulated stresses with the increasing of the total number of applied cycles for the case of 0.75 mm. This effect was not observed for the thicker aligner (0.88 mm) this being an indication that larger thickness allows more even distribution of accumulated stress and minor degradation of viscoelastic properties. Tests have shown that aligners with 0.75 mm thickness have a greater deformation than those with 0.88 mm thickness, in presence of artificial saliva. This result is important from a clinical point of view by taking into account that, in the management of orthodontic treatment, the replacement of the aligner occurs every 15 days. This research suggests that the 0.75 mm thickness aligners should therefore be replaced more quickly due to the modification of their initial rigidity and therefore of the fitting on dental elements, while the 0.88 mm thickness aligners can be used for a longer time.
Data Availability Statement
The datasets generated for this study are available on request to the corresponding author.
Ethics Statement
Ethical review and approval was not required for the study on human participants in accordance with the local legislation and institutional requirements. The patient/participant provided their written informed consent to participate in this study.
Author Contributions
CCi contributed to design the experiment, to perform the mechanical tests, to elaborate and analyze data, to prepare and review the manuscript, and to elaborate state of the art. GP and GR contributed to elaborate and analyze the data, to prepare and review the manuscript, and to elaborate state of the art. CCa contributed to design the experiment. ML and GB specialized medical doctor and contributed to the experimental campaign. CP and DC contributed to ideate the research, to design the experiment, and to analyze the data.
Funding
The specimens were designed and manufactured in the laboratory of the Department of Clinical and Experimental Medicine, University of Foggia. The tests were done in the laboratory of Experimental Mechanics of the Dept. of Mechanical, Mathematics and Management of Politecnico di Bari.
Conflict of Interest
The authors declare that the research was conducted in the absence of any commercial or financial relationships that could be construed as a potential conflict of interest.
Acknowledgments
The authors acknowledge Fabio Salcuni for his contribution to the manufacturing of casts and aligners used in this work.
References
Ariyama, T. (1993). Stress relaxation behavior after cyclic preloading in polypropylene. Polym. Eng. Sci. 33, 1494–1501. doi: 10.1002/pen.760332209
Ariyama, T. (1996). Viscoelastic-plastic behaviour with mean strain changes in polypropylene. J. Mater. Sci. 31, 4127–4131. doi: 10.1007/bf00352678
Boyd, R. L., Oh, H., Fallah, M., and Vlaskalic, V. (2006). An update on present and future considerations of aligners. J. Calif. Dent. Assoc. 34, 793–805.
Dupaixa, R. B., and Boyce, M. C. (2005). Finite strain behavior of poly (ethylene terephthalate) (PET) and poly (ethylene terephthalate)-glycol (PETG). Polymer 46, 4827–4838. doi: 10.1016/j.polymer.2005.03.083
Eliades, T., and Bourauel, C. (2005). Intraoral aging of orthodontic materials: the picture we miss and its clinical relevance. Am. J. Orthod. Dentofac. 127, 403–412. doi: 10.1016/j.ajodo.2004.09.015
Elkholy, F., Schmidt, S., Amirkhani, M., Schmidt, F., and Lapatki, B. G. (2019). Mechanical characterization of thermoplastic aligner materials: recommendations for test parameter standardization. J. Healtc. Eng. 2019:8074827. doi: 10.1155/2019/8074827
Fang, D., Zhang, N., Chen, H., and Bai, Y. (2013). Dynamic stress relaxation of orthodontic thermoplastic materials in a simulated oral environment. Dent. Mater. 32, 946–951. doi: 10.4012/dmj.2013-131
Jeremiah, H. G., Bister, D., and Newton, J. T. (2010). Social perceptions of adults wearing orthodontic appliances: a cross-sectional study. Eur. J. Orthod. 33, 476–482. doi: 10.1093/ejo/cjq069
Kesling, H. D. (1945). The philosophy of the tooth positioning appliance. Am. J. Orthod. Oral Surg. 31, 297–304. doi: 10.1016/0096-6347(45)90101-3
Kim, D. H., Yoo, S. S., Kim, H. J., and Kim, D. E. (2016). Surface damage behavior of polyurethane O-rings in automated material handling system for glass panels. Int. J. Precis. Eng. Man. 17, 43–50. doi: 10.1007/s12541-016-0006-z
Kizypow, D. J., and Rimnac, C. M. (2000). Cyclic steady state stress-strain behavior of UHMW polyethylene. Biomaterials 21, 2081–2087. doi: 10.1016/s0142-9612(00)00138-1
Kwon, J. S., Lee, Y. K., Lim, B. S., and Lim, Y. K. (2008). Force delivery properties of thermoplastic orthodontic materials. Am. J. Orthod. Dentofac. 133, 228–234. doi: 10.1016/j.ajodo.2006.03.034
Landel, R. F., and Nielsen, L. E. (1993). Mechanical Properties of Polymers and Composites. Boca Raton, FL: CRC Press.
Lindauer, S. J., and Shoff, R. C. (1998). Comparison of Essix and Hawley retainers. J. Clin. Orthod. 32, 95–97.
Lloyd, T., Nightingale, C., and Edler, R. (2001). The use of vacuum formed splints for temporary intermaxillary fixation in the management of unilateral condylar fractures. Br. J. Oral Maxillofac. Surg. 39, 301–303. doi: 10.1054/bjom.2001.0649
Lombardo, L., Martines, E., Mazzanti, V., Arreghini, A., Mollica, F., and Siciliani, G. (2017). Stress relaxation properties of four orthodontic aligner materials: a 24-hour in vitro study. Angle Orthodontist. 87, 11–18. doi: 10.2319/113015-813.1
Ma, Y. S., Yu Fang, D. N., Zhang, X. J., Ding, K., Zhang, Y., and Bai, Y. X. (2016). Mechanical properties of orthodontic thermoplastic PETG/PC2858 after blending. Chinese J. Dent. Res. 19, 43–48. doi: 10.3290/j.cjdr.a35696
McNamara, J. A., Kramer, L., and Juenker, J. P. (1985). Invisible retainers. J. Clin. Orthod. 19, 570–578.
Oh, W. S., and Wang, C. (2007). Use of thermoplastic vacuum-formed matrix for emergency management of crown-root fracture. J. Prosthet. Dent. 98, 335–336. doi: 10.1016/s0022-3913(07)60109-7
Pascual, A. L., Beeman, C. S., Hicks, E. P., Bush, H. M., and Mitchell, R. J. (2010). The essential work of fracture of thermoplastic orthodontic retainer materials. Angle Orthodontist. 80, 554–561. doi: 10.2319/042809-232.1
Pohl, M., and Yoshii, O. (1994). Der Osamu-Retainer und sein Indikationsbereich. Kieferorthop, Prakt. Kieferothop 8:89.
Ponitz, R. J. (1971). Invisible retainers. Am. J. Orthod. 59, 266–272. doi: 10.1016/0002-9416(71)90099-6
Rajeesh, K. R., Gnanamoorthy, R., and Velmurugan, R. (2010). Effect of humidity on the indentation hardness and flexural fatigue behavior of polyamide 6 nanocomposite. Mater. Sci. Eng. A 527, 2826–2830.
Ryokawa, H., Miyazaki, Y., Fujishima, A., Miyazaki, T., and Maki, K. (2006). The mechanical properties of dental thermoplastic materials in a simulated intraoral environment. Orthod. Waves 65, 64–72. doi: 10.1016/j.odw.2006.03.003
Scaffaro, R., Dintcheva, N. T., and La Mantia, F. P. (2008). A new equipment to measure the combined effects of humidity, temperature, mechanical stress and UV exposure on the creep behaviour of polymers. Polym. Test. 27, 49–54. doi: 10.1016/j.polymertesting.2007.08.005
Schrauwen, B. A. G. (2003). Deformation and Failure of Semicrystallie Polymer Systems: Influence of Micro and Molecular Structure. Eindhoven: Technische Universiteit Eindhoven.
Shen, X., Xia, Z., and Ellyin, F. (2004). Cyclic deformation behavior of an epoxy polymer. part I: experimental investigation. Polym. Eng. Sci. 44, 2240–2246. doi: 10.1002/pen.20251
Sheridan, J. J., and Armbruster, P. (1999). Bleaching teeth during supervised retention. J. Clin. Orthod. 33, 339–344.
Sheridan, J. J., Ledoux, W., McMinn, R., and Essix, R. (1994). Technology for the fabrication of temporary anterior bridges. J. Clin. Orthod. 28, 482–486.
Tricca, R., and Li, C. (2006). “Properties of aligner materia Ex30,” in The Invisalign System, ed. O. C. Tuncay, (Philadelphia: Quintessence Publishing), 177–186.
Xu, W., and Muldowney, G. (2015). Quantification of water diffusion in CMP pad polymers and impact on mechanical properties. ECS J. Solid State Sci. 4, 5078–5087.
Keywords: compression cyclic loading, mechanical characterization, PET-G, invisible aligners, residual stress
Citation: Cianci C, Pappalettera G, Renna G, Casavola C, Laurenziello M, Battista G, Pappalettere C and Ciavarella D (2020) Mechanical Behavior of PET-G Tooth Aligners Under Cyclic Loading. Front. Mater. 7:104. doi: 10.3389/fmats.2020.00104
Received: 05 December 2019; Accepted: 06 April 2020;
Published: 08 May 2020.
Edited by:
Admir Masic, Massachusetts Institute of Technology, United StatesReviewed by:
Joanna Mystkowska, Bialystok University of Technology, PolandHon Fai Chan, The Chinese University of Hong Kong, China
Copyright © 2020 Cianci, Pappalettera, Renna, Casavola, Laurenziello, Battista, Pappalettere and Ciavarella. This is an open-access article distributed under the terms of the Creative Commons Attribution License (CC BY). The use, distribution or reproduction in other forums is permitted, provided the original author(s) and the copyright owner(s) are credited and that the original publication in this journal is cited, in accordance with accepted academic practice. No use, distribution or reproduction is permitted which does not comply with these terms.
*Correspondence: Claudia Cianci, Y2xhdWRpYS5jaWFuY2lAcG9saWJhLml0