- Key Laboratory for Green Chemical Process of Ministry of Education, Wuhan Institute of Technology, Wuhan, China
The phosphate ore flotation is achieved through the surface electrical behavior difference among apatite, dolomite and quartz, but it is usually affected by the dissolved ions from pulp, especially Ca2+ and Mg2+. The zeta potentials of apatite, dolomite, quartz, and phosphate ore in different solutions were measured to discuss the surface electrical behaviors of them in this study. It was found that the apatite, dolomite and phosphate ore supernatants have limited impact on the zeta potentials of the respective surfaces themselves, but the surface electrical behaviors of apatite and dolomite in the supernatant of the other is similar to the supernatant mineral source. This will increase the separation difficulty of apatite from dolomite in the phosphate ore flotation process. The negative zeta potentials of apatite, dolomite, quartz and phosphate ore increase in the presence of Ca2+ and Mg2+. Moreover, a more zeta potential increase in presence of Ca2+ than Mg2+ was investigated, suggesting a stronger influence of Ca2+ than Mg2+ on the surface electrical behaviors of ores.
Introduction
Phosphorus is an important chemical raw material, and is also a primary constituent of plants. The industrial phosphorus is extracted from the phosphate ore to produce elemental phosphorus, phosphoric acid, phosphatic fertilizer and phosphate (Santana et al., 2011; Xiao et al., 2013). Phosphate ore is a significant mineral source of phosphorus, which is general floated to recover the phosphorus in the apatite minerals (Ca5(PO4CO3)3(F, OH, Cl)) (Zhou et al., 2017). However, most of the phosphate ore industry is currently focused on recovery of apatite from low-grade ores due to the decrease of high-grade phosphate ore. Flotation as the most important method is applied in phosphate ore processing to separate the valuable mineral of apatite from the gangue minerals, such as dolomite and quartz (Zhou et al., 2015). Mineral type and electrolyte environment will result in different surface electrical behaviors of different minerals, and even the same mineral (Alroudhan et al., 2016). Therefore, the investigation of those surface electrical behaviors, which influences interfacial process such as flotation, has obvious research significance. Apatite and dolomite belong to the group of salt-type minerals. It has thus been assumed that they have similar surface electrical behaviors due to the dissolved metal ions (Mishra, 1978; Hu and Wang, 1992). Metal ions in the bulk solution which can be adsorbed on the mineral surface by physical and/or chemical interaction exist in various forms, such as bonding to mineral lattice, generating metal hydroxides, or carbonate hydroxides precipitated on mineral surface, producing new active center of beneficiation reagent, transferring mineral surface to improve or weaken the role of mineral processing reagents (Demir et al., 2003).
Dolomite can produce Ca2+ and Mg2+ ions into the pulp during the ore grinding and pulp-mixing processes (Amankonah et al., 1985; dos Santos et al., 2010; Li et al., 2017). The valuable mineral, apatite, in phosphate ore has the same lattice ion, Ca2+, as dolomite, which results in a significant effect on the selective flotation of apatite from dolomite. In the real phosphate ore flotation process, different ions in the pulp change the surface electrical behavior of minerals and hence change the selective adsorption of beneficiation reagent on the mineral surface, which enlarges the difficulties in the selective separation of apatite from dolomite and quartz (Amankonah et al., 1985).
The surface electrical behaviors of apatite, dolomite, and quartz in mineral supernatants were discussed in this study. The surface electrical behaviors of apatite, dolomite and quartz in solutions with different Ca2+ and Mg2+ concentrations have also been studied to further confirm the effects of Ca2+ and Mg2+. The comparison of surface electrical behaviors for phosphate ore in phosphate ore supernatants and in solutions containing Ca2+ and Mg2+ are conducted to explore the surface chemistry changes in phosphate ore flotation.
Materials and Methods
Materials
Potassium chloride, calcium chloride, and magnesium chloride were used in reagent grade. Reagent grade hydrochloric acid and sodium hydroxide were used as pH modifiers.
The single minerals of apatite, dolomite and quartz were purchased from Canada Wards Natural Science Establishment Company, hand-crushed by a hammer, ground, and sieved to obtain particles with diameter smaller than 20 μm for zeta potential measurement. The phosphate ore from Yichang City, China, was ground by a Mortar Grinder (Fisher Scientific) to obtain the same size fractions as the single minerals for zeta potential measurement. All mineral samples prepared were analyzed by XRD shown in Figure 1. As seen in Figure 1, the XRD peaks of the tested apatite dolomite and quartz matched closely with that of the standard samples, confirming that the samples is mainly apatite, dolomite, and quartz, respectively. The phosphate ore sample is mainly fluorapatite.
Methods
The mineral supernatants of apatite, dolomite, quartz, and phosphate ore were prepared by immersing the single mineral, respectively, in 10 mM KCl solutions for 48 h followed by suction filtration. The absence of mineral particles in the mineral supernatants was determined by an optical microscope. The ore samples were placed in the test solutions to prepare ore suspensions, which were hand-shaken for 2 min. After 10 min standing for mineral particles to reach equilibrium, the supernatant of the suspension was diluted by the test solutions, and then placed under an ultrasonic equipment HS2060A (Fisher Scientific) for 10 min. The detailed description can be found in the literature (Zhou et al., 2015). The obtained solutions were used for zeta potential measurement by Zetasize R-Malvern Nano-ZS. The measurements were repeated six times for the same suspension, and the average values as well as the standard deviations of a given ore sample were reported. All the experimental set-up was usually flushed by nitrogen gas to avoid the possible precipitation of calcium carbonate in the system and the formation of carbonate species on the ore surface (Amankonah et al., 1985). The measurement was conducted at ambient temperature of 22 ± 0.5°C.
Results and Discussion
Zeta Potentials of Apatite, Dolomite, Quartz
Results obtained for the zeta potentials of apatite, dolomite, and quartz in 10 mM KCl solutions are given in Figure 2. The Zeta potentials of apatite, dolomite, and quartz decrease with the pH increasing among the tested pH range. Some literatures report that isoelectric point (IEP) value of apatite from different deposits changes from 2.0 to 8.0, and the dolomite is around 3.0–6.0, but they can shift in the presence of electrolyte (Yu et al., 1985; Merma et al., 2013; Kasha et al., 2015). Therefore, isoelectric points of apatite and dolomite in 10 mM KCl solutions are observed, but no isoelectric points of quartz are determined around the tested pH range from 3.1 to 10.2. In general, the zeta potential of apatite is larger than that of dolomite, and that of quartz is the most electrostatically charged among the three ores.
Dissolution of Apatite and Dolomite
Apatite and dolomite are slightly soluble minerals, which can release the dissolved Ca2+ and Mg2+ to affect the surface electrical behaviors of minerals. Since the dissolution of apatite and dolomite can achieve equilibrium in 2 h according to the previous studies, the dissolved Ca2+ and Mg2+ contents as a function of pH were investigated with the two minerals soaked in different pH solutions for 2 h, respectively.
From Figure 3, the dissolved Ca2+ content in acid solutions is relatively high due to the carbonate and phosphate minerals reacting with hydrogen ions. But it decreases in alkaline environment, which is due to the precipitation of Ca(OH)2. This phenomenon is similar to that of Mg2+ in Figure 4, where the dissolved Mg2+ from dolomite decreases with the solution pH increase. Furthermore, the dissolved Ca2+ from dolomite is more than that of apatite, which is consistent with literature (Amankonah et al., 1985). Amankonah et al. (1985) found that the dissolved Ca2+ from calcite is more than that of apatite when calcite and apatite are soaked in open water, closed water and 2 mM KNO3 solution. Generally, the dissolved Ca2+ and Mg2+ will affect the surface electrical behavior of minerals in solution.
Surface Electrical Behavior of Apatite, Dolomite, and Quartz in Apatite Supernatants
Results obtained for the zeta potentials of apatite in apatite supernatants was compared to that of apatite in 10 mM KCl shown in Figure 5A. It can be seen that there was no marked difference in the zeta potential values because of the similar surfaces of apatite in the two solutions. The experimental dates suggest that the influence of dissolved ions from apatite on the degree of variation in zeta potential values of apatite can be ignored. No changes can be deduced, because the new surface formed by the dissolved ions precipitated and/or adsorbed on apatite surface is similar to the apatite surface in the absence of apatite supernatants. The dissolved ions just change the electrolyte solutions, and further the influence is tiny because of the low concentration.
For dolomite in apatite supernatants, it is interesting to find that there is no marked difference between the zeta potential-curve of dolomite in apatite supernatants and the zeta potential-curve of apatite, but both of them are distinct to the dolomite. This result is similar to the interaction of apatite and calcite reported by Somasundaran (Amankonah et al., 1985). The observations indicate that the dissolved ions from apatite change the surface of dolomite to become similar to the apatite surface due to the precipitation and adsorption of dissolved ions on the dolomite surface.
In Figure 5C, the zeta potential-curve of quartz in apatite supernatants shifts toward less negative zeta potential compared to the original values of quartz, suggesting that the zeta potentials of quartz increase at the same pH because of the dissolved positive ions from apatite. There may be two reasons. The one is that the dissolved ions change the electrolyte solutions due to the zeta potential depending on the electrolyte and the ionic concentration (Sze et al., 2003). The second is that the dissolved ions are adsorbed or precipitated on the quartz surface to form calcium phosphate or hydroxide so as to change its surface electrical behavior. However, the zeta potential for quartz in apatite supernatants is still distinct to that of apatite, indicating that the dissolved ions cannot transfer the quartz surface to the apatite surface, very different from its effects on the dolomite.
Surface Electrical Behavior of Apatite, Dolomite, and Quartz in Dolomite Supernatants
The results given in Figure 6A show the zeta potentials of apatite are increased by the dolomite supernatants, and move to the zeta potential-curve of dolomite, indicating the surface conversion of apatite to dolomite with the impact of dissolved ions from dolomite because the zeta potential increased with increase in ionic concentration of cations (Ozeki et al., 1989). This surface conversion from apatite to dolomite in dolomite supernatants is similar to the surface change from dolomite to apatite in apatite supernatants shown in Figure 5B. The zeta potentials of dolomite in dolomite supernatants are similar to that of dolomite over the entire pH range investigated shown in Figure 6B, indicating a less impact of the dissolved ions from dolomite on the zeta potential variation of dolomite. The results are similar to the effects of apatite supernatants on the surface electrical behavior of apatite in Figure 5A. The reason of the surface conversion for apatite and dolomite in the other supernatants may be the similar lattice cations of Ca2+ and Mg2+ for apatite and dolomite, which will adsorb on the surface of the minerals or form hydroxide precipitations. For quartz in dolomite supernatants shown in Figure 6C, the original negative zeta potential of quartz is increased and IEP at pH 4.7 is observed because of the increasing of dissolved ions from dolomite, which can be deduced they are divalent Ca2+ and Mg2+ ions due to the chemical component of dolomite, CaMg(CO3)2 (Pokrovsky et al., 1999).
Effects of Ca2+ on the Surface Electrical Behavior of Apatite, Dolomite, and Quartz
The effect of Ca2+ on the surface electrical behavior of apatite, dolomite and quartz is shown in Figure 7, respectively. Examination of the results shows that the zeta potentials of the three ores are more positively charged in solutions containing Ca2+ than in 10 mM KCl among the tested pH range, and the Ca2+ can alter the zeta potentials of these ores from negative to positive. The affinity between the Ca2+ and the zeta potentials of apatite, dolomite, and quartz is affected by the concentration of Ca2+. The greater the concentration of Ca2+ is, the higher the zeta potentials of these ores in calcium chloride solution is. The increase in the zeta potential values resulting from the concentration of calcium ions could be attributed to the compression of the double layer under high ionic strength conditions (Amankonah et al., 1985). Furthermore, a high concentration of CaCl2 can be expected to cause the precipitation such as calcium hydroxide on the surface of ores to produce more positive zeta-potential values.
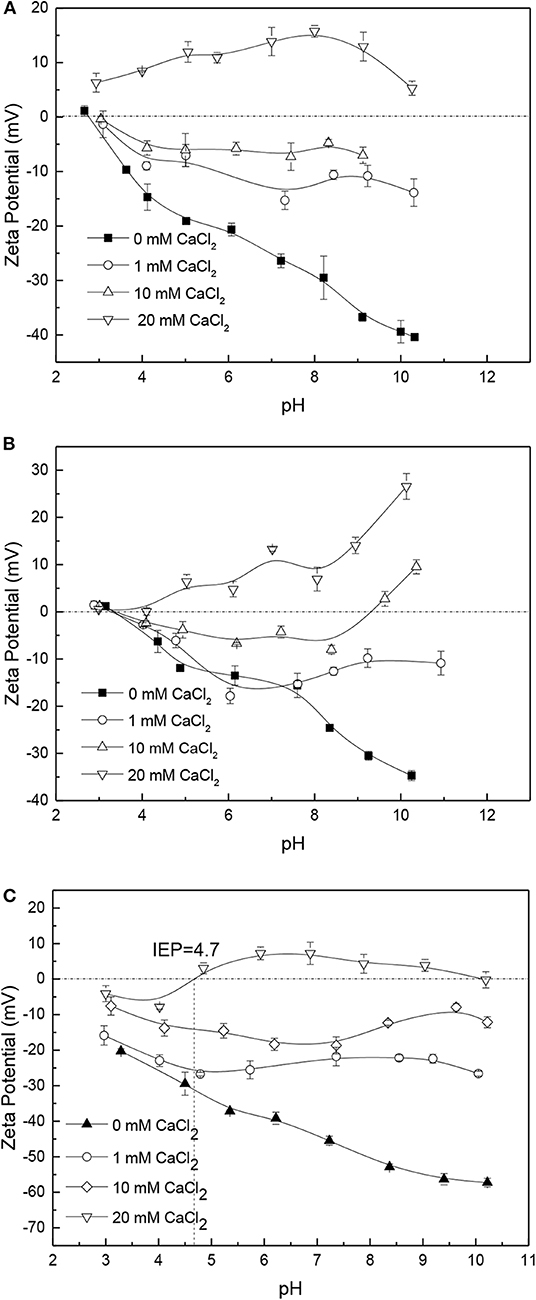
Figure 7. Zeta potential of apatite (A), dolomite (B), and quartz (C) in solutions with different concentration of CaCl2.
Effects of Mg2+ on the Surface Electrical Behavior of Apatite, Dolomite, and Quartz
The effect of Mg2+ at different concentration on the surface electrical behaviors of apatite, dolomite, and quartz is shown in Figure 8. The Mg2+ causes the zeta potentials of these ores to be increased similar to the effect of Ca2+ among the tested pH range. A higher concentration of Mg2+ has a greater impact on the surface electrical behaviors of apatite, dolomite and quartz. At a relative high concentration, 20 mM MgCl2, the original negative zeta potentials of apatite, dolomite, and quartz change to be positive. The isoelectric points of these ores shift to a relatively small values. In the acidic solution, Mg2+ is in the form of positioning ion adsorbed on the surface of apatite and dolomite in the form of characteristic adion. Under the condition of high pH value, the adsorption of Mg2+ and OH− may create precipitation on the surface of ores to produce more positive zeta potential values (Tang et al., 2010).
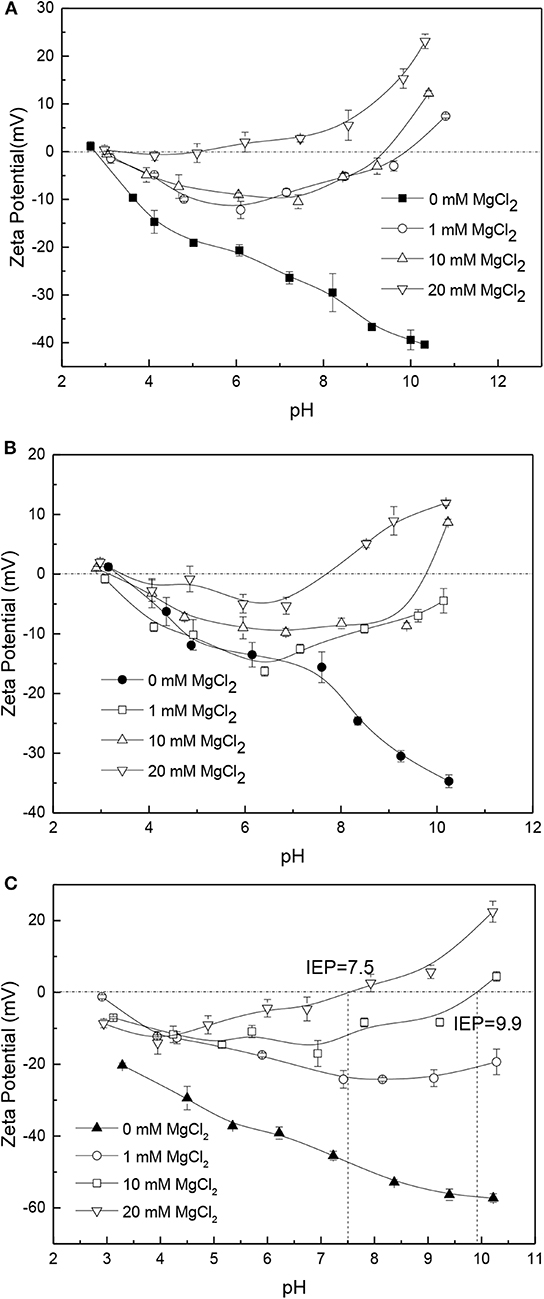
Figure 8. Zeta potential of apatite (A), dolomite (B), and quartz (C) in solutions with different concentration of MgCl2.
Electrokinetic Behavior of Phosphate Ore
Surface Electrical Behavior of Phosphate Ore in Phosphate Ore Supernatants
To understand the surface electrical behavior of phosphate ore, a phosphate rock from China was chosen to investigate the effects of its supernatants, Ca2+ and Mg2+, on its zeta potentials which can affects interfacial process such as flotation. Figure 9 shows the comparison of zeta potential-curves of phosphate ore and phosphate ore in phosphate ore supernatants. The two curves are basically coincident when pH < 6, but as pH increases, they separate gradually. In general, the zeta potential of phosphate ore in phosphate ore supernatants is greater than that of phosphate ore. The phosphate ore supernatants contain metal cations dissolved from phosphate ore. When pH > 8, these cations can attach and cause precipitation to the surface of phosphate ore to produce more positive zeta potential values.
Effects of Ca2+ on the Surface Electrical Behavior of Phosphate Ore
The effects of Ca2+ with different concentrations on the zeta potential of phosphate ore are shown in Figure 10. The results show that the zeta potentials of phosphate ore in 0 mM CaCl2, 1 mM CaCl2, and 10 mM CaCl2 are negative over the entire pH range investigated. The isoelectric point of phosphate ore in 20 mM CaCl2 is about 5.2. Data given in Figure 10 for the zeta potential of phosphate ore in calcium chloride of several concentrations show that the Ca2+ has the same impact on the zeta potential of phosphate ore to the other three ores, apatite, dolomite, and quartz. The greater the concentration of Ca2+ is, the higher the zeta potential of phosphate ore in CaCl2 solution is.
Effects of Mg2+ on the Surface Electrical Behavior of Phosphate Ore
Figure 11 shows the effects of Mg2+ on the zeta potential of phosphate ore. The original negative zeta potential of phosphate ore is increased in the presence of Mg2+. The results suggest that the Mg2+ reveals the similar influence on the zeta potential of phosphate ore as Ca2+. However, the Ca2+ shows a greater impact than the Mg2+ on the zeta potential of phosphate ore among the tested pH range according to the comparison of Figures 10, 11. The isoelectric points of phosphate ore in 20 mM MgCl2 solutions, is about 6.6, but the isoelectric points in 20 mM CaCl2 solutions is about 5.2. A relatively higher influence of CaCl2 solutions than MgCl2 solutions are also investigated for the zeta potentials of apatite, dolomite, and quartz according to the Figures 7, 8, which is consistent with the results on silica reported in literature (Datta et al., 2009). Mammen et al. (1997) used a simple model of a silica surface to present that a higher degree of association of Ca2+ to the silica surface are consistent with the experimental zeta potentials tested by Datta et al. (2009). The higher zeta potentials of Ca2+ observed for comparison to Mg2+ may result from the increase in association of Ca2+ to the mineral surface and the larger ion radius of Ca2+ leading to lower polarizability than Mg2+ as well as its smaller influence to the electrons on the outer surface of minerals.
Conclusion
No impact or limited impact of the apatite, dolomite and phosphate ore supernatants are found on the surface electrical behaviors of the respective surfaces. The zeta potentials of quartz in apatite or dolomite supernatants increase compared to the original negative zeta potential of quartz. When apatite and dolomite are conditioned in the supernatants of each other, they interchange their zeta potential-curves over the entire pH range investigated. The zeta potential-curve of apatite in dolomite supernatants moves from that of apatite to dolomite, while the zeta potential-curve of dolomite in apatite supernatants moves from that of dolomite to apatite similarly. At high Ca2+ and Mg2+ concentration, especially 20 mM, the zeta potentials of apatite, dolomite, quartz and phosphate ore increase with IEP investigated, which is distinctly different from the original negative charge. Furthermore, it is interesting to find that the effects of Ca2+ is greater than that of Mg2+ on the surface electrical behaviors of apatite, dolomite, quartz, and phosphate ore.
These results are significant for the phosphate ore flotation. In the flotation circuit, the calcium and magnesium species from dissolved ores, not only increase the consumption of flotation reagents, but also change the surface properties of apatite, dolomite and quartz. Especially for apatite and dolomite, the similar variation on surface charge will enlarge the difficulty of selective flotation of phosphate ore.
Data Availability Statement
All datasets generated for this study are included in the article/supplementary material.
Author Contributions
FZ designed experiments and wrote the manuscript. QL, XL, WL, and JF carried out experiments. RC analyzed experimental results.
Funding
Project (51404171) was supported by the National Nature Science Foundation of China. Project (T201506) was supported by the Hubei Provincial Department of Education, China.
Conflict of Interest
The authors declare that the research was conducted in the absence of any commercial or financial relationships that could be construed as a potential conflict of interest.
References
Alroudhan, A., Vinogradov, J., and Jackson, M. D. (2016). Zeta potential of intact natural limestone:impact of potential-determining ions Ca, Mg and SO4. Colloids Surf. A Physicochem. Eng. Aspects 493, 83–98. doi: 10.1016/j.colsurfa.2015.11.068
Amankonah, J. O., Somasundaran, P., and Ananthapadmabhan, K. P. (1985). Effects of dissolved mineral species on the dissolution/ precipitation characteristics of calcite and apatite, Colloids Surf. 15:295. doi: 10.1016/0166-6622(85)80080-9
Datta, S., Conlisk, A. T., Li, H. F., and Yoda, M. (2009). Effect of divalent ions on electroosmotic flow in microchannels. Mech. Res. Commun. 36, 65–74. doi: 10.1016/j.mechrescom.2008.07.006
Demir, C., Bentli, I., Gulgonul, I., and Celik, M. S. (2003). Effects of bivalent salts on the flotation separation of Na-feldspar from K-feldspar. Miner. Eng. 16, 551–554. doi: 10.1016/S0892-6875(03)00078-5
dos Santos, M. A., Santana, R. C., Capponi, F., Ataíde, C. H., and Barrozo, M. A. (2010). Effect of ionic species on the performance of apatite flotation. Sep. Purifi. Technol. 76, 15–20. doi: 10.1016/j.seppur.2010.09.014
Hu, Y. H., and Wang, D. Z. (1992). Dissolution/surface property of salt-type minerals and design of schemes of flotation separation. J. Central-South Inst. Mining Metallurgy 23, 273–279.
Kasha, A., Al-Hashim, H., Abdallah, W., Taherian, R., and Sauerer, B. (2015). Effect of Ca2+, Mg2+, and , ions on the zeta potential of calcite and dolomite particles aged with stearic acid. Colloids Surf A Physicochem. Eng. Aspects 482, 290–299. doi: 10.1016/j.colsurfa.2015.05.043
Li, D., Yin, W. Z., Xue, J. W., Yao, J., Fu, Y. F., and Liu, Q. (2017). Solution chemistry of carbonate minerals and its effects on the flotation of hematite with sodium oleate. Int. J. Miner. Metallurgy Mater. 24, 736–744. doi: 10.1007/s12613-017-1457-7
Mammen, M., Carbeck, J. D., Simanek, E. E., and Whitesides, G. M. (1997). Treating electrostatic shielding at the surface of silica as discrete siloxide·cation interactions. J. Am. Chem. Soc. 119, 3469–3476. doi: 10.1021/ja9638115
Merma, A. G., Torem, M. L., Morán, J. J., and Monte, M. B. (2013). On the fundamental aspects of apatite and quartz flotation using a Gram positive strain as a bioreagent. Miner. Eng. 48, 61–67. doi: 10.1016/j.mineng.2012.10.018
Mishra, S. K. (1978). The electrokinetics of apatite and calcite in inorganic electrolyte environment. Int. J. Miner. Process. 5, 69–83. doi: 10.1016/0301-7516(78)90006-6
Ozeki, S., Takano, I., Shimizu, M., and Kaneko, K. (1989). ζ-potential of synthetic chrysotile asbestos in aqueous simple salt solutions. J. Colloid Interface Sci. 132, 523–531. doi: 10.1016/0021-9797(89)90266-X
Pokrovsky, O. S., Schott, J., and Thomas, F. (1999). Dolomite surface speciation and reactivity in aquatic systems. Geochimica et Cosmochimica Acta. 63, 3133–3143. doi: 10.1016/S0016-7037(99)00240-9
Santana, R. C., Duarte, C. R., Ataíde, C. H., and Barrozo, M. A. S. (2011). Flotation selectivity of phosphate ore:effects of particle size and reagent concentration. Sep. Sci. Technol. 46, 1511–1518. doi: 10.1080/01496395.2011.561268
Sze, A., Erickson, D., Ren, L., and Li, D. (2003). Zeta-potential measurement using the Smoluchowski equation and the slope of the current–time relationship in electroosmotic flow. J. Colloid Interface Sci. 261, 402–410. doi: 10.1016/S0021-9797(03)00142-5
Tang, C. Q., Zhang, Q., and Mao, S. (2010). Study on differences of flotation behavior of pure minerals in collophanite and dolomite. Indust. Miner. Process. 13, 1–4 (in Chinese).
Xiao, C., Chi, R., and Fang, Y. (2013). Effects of Acidiphilium cryptum on biosolubilization of rock phosphate in the presence of Acidithiobacillus ferrooxidans. Trans. Nonferrous Met. Soc. China 23, 2153–2159. doi: 10.1016/S1003-6326(13)62711-9
Yu, J., Ge, Y., and Hou, J. (1985). Behavior and mechanism of collophane and dolomite separation using alkyl hydroxamic acid as a flotation collector. Colloids Surf. 15, 295–307.
Zhou, F., Wang, L., Xu, Z., Liu, Q., and Chi, R. (2015). Reactive oily bubble technology for flotation of apatite, dolomite and quartz. Int. J. Miner. Process. 134, 74–81. doi: 10.1016/j.minpro.2014.11.009
Keywords: apatite, dolomite, quartz, phosphate ore, zeta potential
Citation: Zhou F, Liu Q, Liu X, Li W, Feng J and Chi R (2020) Surface Electrical Behaviors of Apatite, Dolomite, Quartz, and Phosphate Ore. Front. Mater. 7:35. doi: 10.3389/fmats.2020.00035
Received: 07 September 2019; Accepted: 30 January 2020;
Published: 18 February 2020.
Edited by:
Zhiyong Gao, Central South University, ChinaReviewed by:
Hock Jin Quah, University of Science Malaysia, MalaysiaYuesheng Gao, Michigan Technological University, United States
Copyright © 2020 Zhou, Liu, Liu, Li, Feng and Chi. This is an open-access article distributed under the terms of the Creative Commons Attribution License (CC BY). The use, distribution or reproduction in other forums is permitted, provided the original author(s) and the copyright owner(s) are credited and that the original publication in this journal is cited, in accordance with accepted academic practice. No use, distribution or reproduction is permitted which does not comply with these terms.
*Correspondence: Ru-an Chi, rac513@163.com