- Department of Chemistry, University of Delhi, New Delhi, India
A high surface area and the possibility of high charge separation and transportability make the two-dimensional (2D) nanomaterials very special in many applications, particularly in the field of catalysis. The usefulness of 2D nanomaterial is positively demonstrated in this study by employing it as an efficient heterogeneous catalyst in Sonogashira cross-coupling reaction, where the later one is well known for the formation of carbon–carbon bonds. 2D nanosheets of CuCo2S4 are synthesized using a hydrothermal synthesis and the average thickness of the sheets is found to be in the range of 10–15 nm. The transparent nanosheets are interconnected to form thick sheets and then aggregated to form a highly open three-dimensional hierarchical structure. This study explores the Sonogashira reaction very efficiently in water/ethanol at room temperature using hydrothermally synthesized CuCo2S4 nanosheets as catalyst for the C-C coupling reaction for the first time. The developed method is found to be economic, green, high yields, low catalyst loading and reusability of the catalysts. This present CuCo2S4 nanosheet heterogeneous catalyst afford the Sonogashira coupling of various aryl halides with terminal alkynes, giving a variety of aryl acetylenes in excellent yields and can be recovered by a simple filtration and reused for at least 10 consecutive reactions with almost constant yield and activity.
Introduction
Over the past decades, two-dimensional nanosheets possess a specific perspective in nanoscience and nanotechnology. The sheet nanostructures are very important as they provide more surface area in comparison to other morphologies obtained from nanomaterials like nanoplates, nanowires, nanodiscs etc. (Liu et al., 2011; Min et al., 2012; Gunjakar et al., 2013; Chauhan et al., 2016). Therefore, remarkable development has been seen in 2D materials due to their potential applications in gas storage, sensing, catalysis, electronic applications like supercapacitors, batteries, and electrolyzer cell and hydrogen storage applications (Tan and Zhang, 2015; Chauhan et al., 2017a; Antil et al., 2019). The 2D materials which have individual properties like pure crystalline nature, tunable thickness, and extraordinary mechanical-thermal stability with high charge conductivity endowed them vital for various catalytic reactions. Moreover, the planar nature of nanosheets facilitates easy adsorption and removal of reactant species which also enhance their reactivity. Because of unique features and outstanding performances, 2D nanomaterials are very popular and extensive research efforts have been dedicated for their development and exploration of their properties like lateral size dependent transport, crystal phase, defects, layer thickness, and surface properties (Mika et al., 2018). But still synthetic procedures for the 2D materials with desirable topographies is somewhat challenging.
Two-dimensional transition-metal chalcogenides have tremendously impacted the areas of heterogeneous catalysis in many organic transformation reactions, such as in oxidation, reduction, C-C coupling, C-S coupling, C-N coupling, etc. Cross-coupling (C-C) reactions play the main role in organic synthesis to set up the carbon skeleton of organic molecules (Maaliki et al., 2017). Transition-metal based catalysts (such as Pd, Cu, Ni etc.) displayed remarkable efficiency for achieving these reactions. Mainly palladium (Oppolzer et al., 1991), or nickel (Beletskaya et al., 2003) complexes have been used for “cross-coupling reactions.” Sonogashira reaction is the most general, reliable, and effective method for the building of C(sp2)–C(sp) bonds by cross-coupling of terminal alkyne and aryl halide (Tykwinski, 2003; Nicolaou et al., 2005; Chinchilla and Nájera, 2007). This reaction has been successfully used in the synthesis of natural products (Toyota et al., 2000), biologically active compounds (Cosford et al., 2003), materials showing photophysical properties (Mongin et al., 2002); polymeric materials (Li et al., 1999), and polyalkynylated molecules (Mongin et al., 1998). Sonogashira reactions occurs in the presence of Pd(OAc)2 (Oppolzer et al., 1991), Palladium complexes such as Pd(PPh3)4 (Nicolaou et al., 2005), [PdCl2(PPh3)2] (Nicolaou et al., 2001) and copper (I) iodide as a co-catalytic salts. As the Palladium is expensive, hence nowadays researchers are exploring the methods which can replace the costly metals with the cheaply available metals such as Nickel (Beletskaya et al., 2003), Copper etc. (Thathagar et al., 2004). Because antecedently copper salts were used in the catalytic reactions with the palladium complexes (Nicolaou et al., 2005), recent attention has been focused on employing copper-only or copper based catalytic systems in Sonogashira coupling. Ma et al. reported first time the coupling reaction of aryl halides and terminal alkynes in the presence of Copper iodide and N, N-dimethylglycine hydrochloride salt (Ma and Liu, 2004). Rothenberg et al. also reported Sonogashira coupling using copper nanoclusters (Thathagar et al., 2004). The literature studies on the various organic reactions catalyzed over transition metal oxide/chalcogenide nanosheets have clearly demonstrated that these materials reduce the reaction barrier of many organic reactions (coupling reactions, oxidation-reduction, polymerization, cycloaddition, and condensation processes) and control of the reaction products (Raza et al., 2016). These studies also unrevealed why 2D nanosheet morphology of transition metal chalcogenides are very popular in heterogeneous catalysis. The synergy effect of reactant molecules with catalyst surface and activity allow some reactions to catalyzed with different pathways and amended their product selectivity (Ng and Raitses, 2015; Zhou et al., 2017). Very recently, various homo-coupling and cross-coupling reactions were widely studied over 2D nanosheets of WS2 (Raza et al., 2016) and Pd-doped WS2 (Raza et al., 2017) with remarkable reactivity and permanence.
Herein, we report an effective approach of hydrothermally synthesized CuCo2S4 two-dimensional nanosheets for cross-coupling reactions of terminal alkynes and aryl halides. The CuCo2S4 nanosheets work as a heterogeneous catalyst with exceptional performance (yield up to 95%). 2D CuCo2S4 nanosheets were applied without any exfoliation, surface modification or without the introduction of any foreign expensive metal particles (Pt, Pd, Ru) or without the addition of any co-catalysts under very mild conditions (water /ethanol, 25°C). In addition, all reaction condition like the variation in the solvent mixture, temperature, catalyst loading, and reaction mechanism was investigated thoroughly. Furthermore, durability of the catalyst was examined by running continuous 10 cycles of used catalyst, where the catalyst showed retentivity of its properties suggesting the great strength and high reactivity toward cross-coupling reactions. It was found that the introduction of electron-donating and electron-withdrawing groups in aryl halide didn't affect the efficiency of catalyst and product was obtained with 100% selectivity. However, yield of the reactions decreases in the order of I > Br > Cl. Apart from CuCo2S4, two more thiospinel nanosheet samples by varying the Cu amount were synthesized following the similar synthesis method, viz. Cu0.5Co2.5S4 and Co3S4 to examine the effect of Cu incorporation into the original Co3S4 lattice, where CuCo2S4 was found to be the best catalyst among them.
Materials and Methods
Materials
Copper (II) sulfate pentahydrate (CuSO4.5H2O), cobalt (II) nitrate hexahydrate (Co(NO3)2·6H2O, 99.5%), copper(II) nitrate trihydrate(Cu(NO3)2.3H2O, 99.0%) were purchased from Sigma Aldrich USA. Thiourea (CH4N2S, 99%), ethylene glycol (C2H6O2) and ethylenediamine (C2H8N2) were purchased from Thomas Baker, India. Double distilled water was used in all experiments. 4-Bromoanisole, benzyl bromide, 4-bromotoluene, 4-tertbutyl bromo benzene, bromo benzene, 4-nitro bromo benzene, iodo benzene, chloro benzene, phenyl acetylene, 4-bromo aniline, sodium sulfate and sodium chloride were purchased from Spectrochem. Absolute ethanol and isopropanol (99%) were procured from Fischer India, KOH from Rankem, India. All chemicals were used as received without any further purification.
Synthesis of CuCo2S4 Nanosheets
2D nanosheets of CuCo2S4 (mixed metal thiospinel) was synthesized by using a simple hydrothermal technique as reported by our group earlier (Chauhan et al., 2017b, 2019). In a typical procedure, metal nitrate precursor of cobalt and copper, i.e., Co(NO3)2.6H2O and Cu(NO3)2.3H2O were dissolved in 30 ml of distilled water in a molar ratio 2:1. After stirring the solution for 10 min, 4 mmol of thiourea was added to this solution and solution further stirred for 15 min. Now ethylenediamine (2 ml) was added into this solution and the solution color changed to brown. The whole solution was transferred to a 50 ml teflon lined autoclave. The autoclave was sealed and kept at 200°C for 12 h. The reaction vessel was allowed to cool up to room temperature. The obtained black powder product was washed with distilled water and ethanol for 3 times. The product was dried at 50°C for 6 h and stored for further studies. Nanosheets of Co3S4 and Cu0.5Co2.5S4 were also synthesized following the method similar to that mentioned for CuCo2S4 nanosheets. No Cu salt was added during the synthesis of Co3S4. In the case of Cu0.5Co2.5S4, 2.5 mmol of Co(NO3)2.6H2O and 0.5 mmol of Cu(NO3)2.3H2O were used during the reaction.
Characterization
X-Ray Diffraction (XRD) patterns of thiospinel species were obtained at 298K using a Bruker D8 Advance diffractometer system using monochromatized Cu Kα radiation source. Scanning electron microscopy (SEM) and Energy Dispersive Spectroscopy (EDS) measurements have been performed by using a JEOL JSM 6610 operating at 20 kV, EDAX was accomplished at a resolution of 135.2 eV. Transmission electron microscopy (TEM), high-resolution TEM (HRTEM), and selected area electron diffraction (SAED) study have done by using a Philips Technai G230 transmission electron microscope with an accelerating voltage of 200 kV equipped with an energy dispersive X-ray spectroscopy (EDAX) attachment. To analyze the thickness of nanosheets AFM images were obtained by using a Bruker multimode 8 scanning probe microscope through tapping mode analysis. 1H (proton) NMR spectra was recorded on Bruker Avance 400 MHz NMR spectrometer and CDCl3 was used as a solvent. Chemical shifts are reported in parts per million shift (δ-value) from (CDCl3) (δ7.24 ppm for 1H) as an internal standard. Signal patterns are indicated as s, singlet; d, doublet; dd, double doublet; t, triplet; m, multiplet; bs, broad singlet; bm, broad multiplet. Mass of samples was detected using Agilent high resolution liquid chromatography and mass spectrometer (G6530AA) with mass accuracy >2 ppm.
Catalytic Reactions
All the catalytic reactions were carried out in round bottom flask. The catalytic activity of CuCo2S4 nanosheets was investigated in the synthesis of various diphenylacetylenes. Primarily, the catalytic reaction of nanomaterial was investigated in a model reaction of phenylacetylene and aryl halides to obtain diphenylacetylene using 10 mg of catalyst in water/ethanol mixture at 25°C (Scheme 1). The reaction did not proceed in the absence of the catalyst.
In order to study the efficiency of the nanocatalyst, a variety of aryl halides substrates and phenylacetylene were used to obtain various diphenylacetylenes. It is noteworthy that all the reactions proceeded smoothly and generated diphenylacetylenes in good yields (70–95%) within 150–300 min of reaction time. Furthermore, present nanosheet is found to be the best catalytic system in terms of activity, selectivity, and greenness of the protocol, with negligible waste or by-product generation from the reaction mixture.
General Procedure for the Synthesis of Diphenylacetylenes. A mixture of aryl halide (1 mmol), phenylacetylene (1 mmol), catalyst (10 mg), K2CO3 (2.0 mmol.), ethanol (1.0 mL) and water (1.0 mL) were taken in a 10 mL round-bottomed flask and stirred at room temperature for 3–5 h. After completion of the reaction (monitored by TLC), the product was extracted in Et2O (2 × 5 mL). The ethereal layer was dried over Na2SO4 and the solvent removed under reduced pressure. The crude product, thus isolated, was purified by recrystallization. All compounds were characterized by NMR and mass (see Supplementary Material).
NMR and mass data of the synthesized compounds in Sonogahira cross-coupling reaction.
Diphenylacetylene, white solid; reported m.p. 60–62°C; observed m.p. 60°C; 1H NMR: (CDCl3 400 MHz) δ 7.33–7.35 (m, 6H), 7.55–7.53 (m, 4H). 13C NMR (100 MHz, CDCl3) δ (ppm) 131.73, 128.48, 128.39 123.37, 89.50 LCMS (ES)–calculated 178.08 found 179.06 Isolated yield: 95%.
(4-Methoxyphenyl)phenylacetylene, light yellow solid; reported m.p. 57–61°C; observed m.p. 59°C; 1H NMR: (CDCl3 400 MHz) δ 3.77 (s, 3H), 6.76-6.78 (m, 2H), 7.35–7.36 (m, 3H), 7.38–7.39 (m, 4H).13C NMR (100 MHz, CDCl3) δ (ppm) 159.71, 133.16, 131.55, 128.42, 128.05, 123.68, 115.46, 114.10, 89.48, 88.17, 55.40. LCMS (ES)- calculated 208.09 found 209.09 Isolated yield: 92%.
(4-Nitrophenyl)phenylacetylene, light yellow solid; reported m.p. 120–122°C; observed m.p. 121°C; 1H NMR (400 MHz, CDCl3) δ (ppm) 8.22-8.20(d, 1H), 8.10–8.08(d, 2H), 7.70–7.65(m, 3H), 7.56–7.55 (m, 1H), 7.39–7.38 (m, 2H). 13C NMR (100 MHz, CDCl3) δ (ppm) 147.10, 132.72, 132.37, 131.94, 130.10, 129.38, 128.64, 125.11, 123.75, 94.80, 87.63. LCMS (ES)- calculated 223.06 found 223.09. Isolated yield: 85%.
1-methyl-4-(phenylethynyl)benzene, white solid; reported m.p. 118–122°C; observed m.p. 120°C; 1H NMR (400 MHz, CDCl3) δ (ppm) 7.53–7.50 (m, 4 H), 7.36–7.32 (m, 5 H), 2.56 (s, 3 H). 13C NMR (100 MHz, CDCl3) δ (ppm) 141.40, 136.45, 131.65, 129.25, 128.87, 128.75, 128.48, 128.32, 126.44, 123.55, 91.89, 89.04, 21.64. LCMS (ES)- calculated 192.09 found 193.1005 Isolated yield: 82%.
Results and Discussion
A novel hydrothermal synthesis protocol was used as per our earlier report for the synthesis of mixed-metal thiospinel CuCo2S4 nanosheets. The crystalline phase purity of the as-synthesized material was characterized by using powder X-ray measurements. Figure 1a depicts the powder XRD pattern of the as-prepared sample which matches well with the standard diffraction pattern obtained for CuCo2S4. The peak intensity at 2θ = 31.6 for (311) plane is maximum which suggested more growth of nanosheets toward the corresponding particular hkl values. The diffraction pattern also suggested spinel structure of CuCo2S4 NSs, in which all divalent metal ions (Cu2+) occupy the tetrahedral voids and all trivalent metal ions (Co3+) occupy the octahedral voids. It was reported earlier that, the octahedral coordinated site of thiospinel system are more responsible for certain catalytic activities, therefore cobalt ions plays a major role in the superior reactivity of CuCo2S4 (Liu et al., 2015; Chauhan et al., 2017b). The morphology of the as-synthesized material was analyzed by using transmission electron microscopy (Figure 1b). It reveals general sheet structure of as-prepared thiospinel sample. All nanosheets are interconnected to other adjacent sheets because of agglomeration. A few sheets lying parallel to the surface were near to transparency which specifies ultrathin nature of nanosheets. Inset of Figure 1b is the HRTEM image which clearly shows the lattice fringes of the nanosheets with d = 0.54 nm indicating the presence of largely (311) planes. FESEM imaging shows that nanosheets were not free or in isolated form. The high-magnification FESEM image in the inset (Figure 1d) shows that these two dimensional nanosheets are assembled and give rise to a bulkier 3D hierarchical flower like open structure. Furthermore, the ratio among the elements present in the thiospinel system was examined by using energy dispersive studies (EDS, Figure 1c). The ratio of Cu, Co and S is found to be close to that of 1:2:4, which supported the formation of stoichiometric thiospinel CuCo2S4. Surface area, pore volume and pore size distribution used to be important parameters of a catalyst material. A high surface area as well as sufficient pore volume with narrow pore size distribution are highly essential to be an efficient catalyst. Hence we have carried out specific BET measurement to analyze the surface area and pore volume/pore distribution (Figure 1e). The isotherm is found to be adsorption on mesoporous solids proceeds via multilayer adsorption of a combination of Type IV and V with H3 hysteresis loop. The present nanosheets exhibited 31 m2/g of specific BET surface area and 0.09 cm3/g of pore volume. A sharp mesoporous size distribution is found at 2.5 nm as seen in the inset of Figure 1e. Thus, these significant surface properties of the as-synthesized CuCo2S4 nanosheets demonstrate it's possibility to be a good catalyst.
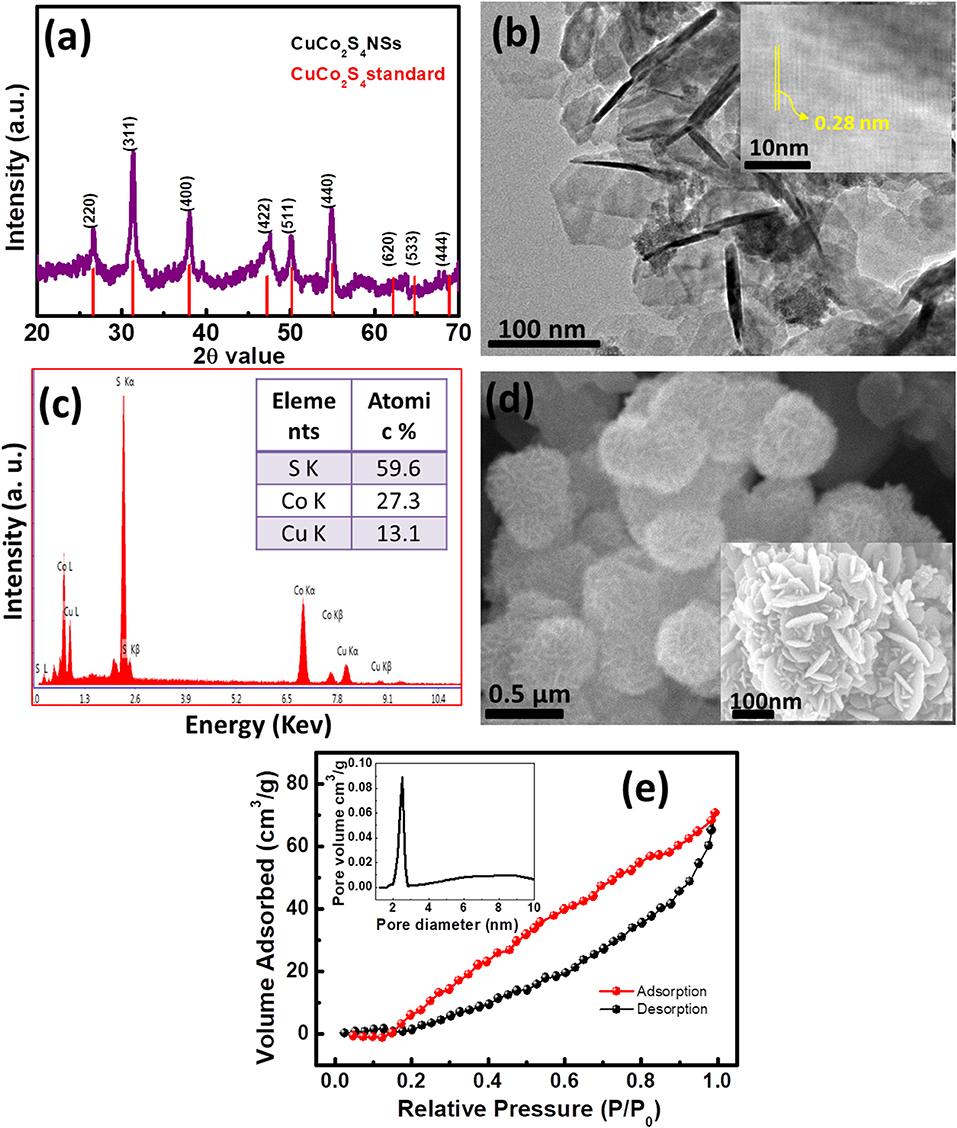
Figure 1. (a) Powder XRD pattern of as-synthesized CuCo2S4 nanosheets compared with the standard pattern, (b) Low-magnification TEM image, (Inset: HRTEM image), (c,d) SEM-EDS and FESEM imaging, respectively. (e) Nitrogen adsorption–desorption isotherms at 77 K of the as-synthesized nanosheet sample. Inset: pore volume vs. pore size distribution of CuCo2S4 nanosheets.
As prepared CuCo2S4 thiospinel nanosheets were futher analyzed by using Raman spectroscopy (Figure 2a). The vibrational modes in the Raman spectrum of thiospinel were obtained because of lattice vibration of tetrahedral and octahedral cations. The higher frequency vibrational modes (A1g) were affected directly via metal cations present in the octahedral voids while other lower frequency modes (F2g, Eg) generated due to metal ion in tetrahedral voids. CuCo2S4 have five allowed transitions for normal spinel system. All the peaks present below 653 cm−1 are corresponds to the stretching frequency of bond between octahedral coordinated metal cation and sulfur (Co-S). The vibrational mode present at 653.7 cm−1 resembles to stretching frequency related to the bonding between the anions (S-S). There are no evidence of bonding between tetrahedral coordinated copper ions and sulfur (Nie et al., 2016). The surface topology and average thickness of the CuCo2S4 nanosheets were examined by atomic force microscopy (AFM) measurements. The AFM study of nanosheets defines the uniformity of the sheets, which also confirmed from the TEM images of nanosheets. The AFM topographic image of CuCo2S4 nanosheets shown in Figures 2b,c together with the sheets thickness profiles (Figure 2d) taken from the much diluted sample. The AFM analysis effectively ensure the aggregated nature of nanosheets however when we have thoroughly analyzed very diluted sample of nanosheets it discloses that the average thickness of single nanosheets was approximately 10–12 nm (Chauhan et al., 2017b).
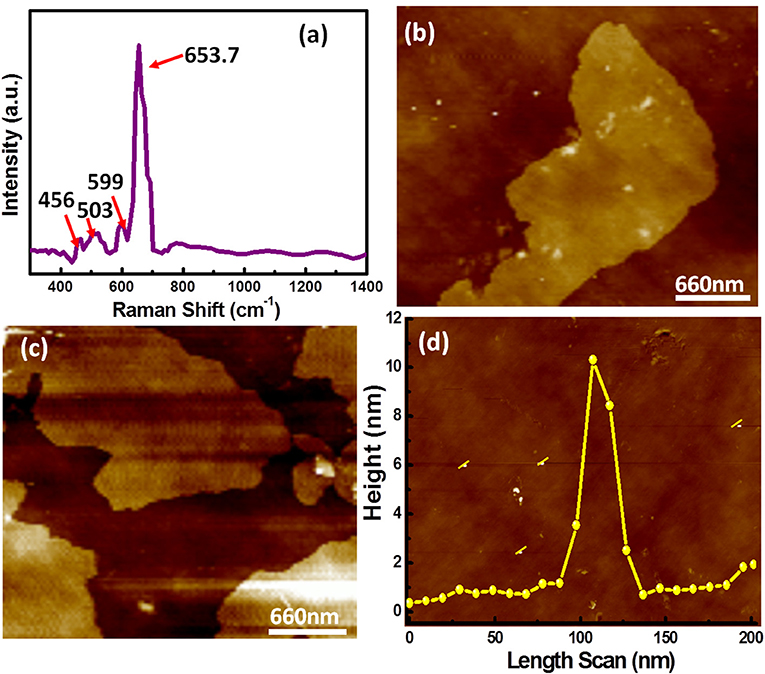
Figure 2. (a) Raman spectrum of as-synthesized CuCo2S4 nanosheets, (b,c) AFM images of nanosheets with (d) cross section height profile graph showing the average height of CuCo2S4 nanosheets ~10–12 nm.
Catalytic Application of CuCo2S4 Nanosheets
In order to explore C-C coupling reaction such as Sonogashira reaction, we focused our attention toward the CuCo2S4 catalyst due to its easy separation, inexpensiveness and recyclability for various reactions. Its catalytic activity in the Sonogashira reaction was investigated under mild conditions. The obtained results are summarized in Table 1.
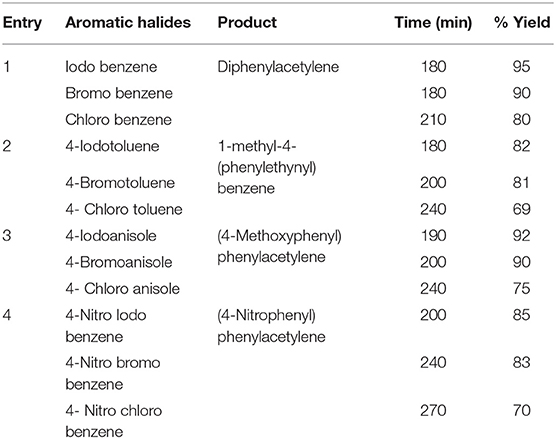
Table 1. CuCo2S4 catalyzed synthesis of diphenylaceteylenes and its derivatives in one pot reaction.
The coupling of iodobenzene with phenylacetylene was first chosen as the model reaction for optimization of the reaction conditions, and the effects of the concentration of catalyst, temperature, time, type of base and solvent on the reaction were examined. The results are shown in Table 2. Firstly, different solvents were employed in this reaction using K2CO3 as a base and in the presence of 10 mol% of catalyst. We can see that the water/ethanol system is best of among all tried solvents and it is greener and environmentally friendly. Reaction temperature is another parameter that considerably could affect the reaction. Therefore, its effect was also examined by performing the model reaction at room temperature (25°C), 60 and 90°C. According to the results shown in Table 2, the reaction can be carried out optimally at 25°C resulting in higher conversion into desirable product. On increasing the temperature there is a degradation of catalyst (color change from black to green). Same way, the types of base used in these reactions also affect their yields. The substrate conversions and product yields (%) are calculated as reported earlier (Deori et al., 2015). So, in the next step, various types of organic and inorganic bases were investigated. K2CO3 conduct the reaction effectively. Thus, considering more availability of K2CO3 and its lower price, it was chosen as the optimum base for the reaction. As it is mentioned in the experimental section, two more nanosheet samples, viz. Co3S4 and Cu0.5Co2.5S4 were synthesized and now these two are too loaded as catalyst for the Sonogashira reaction as shown in Figure 3A. We found that the activity of the catalyst is increasing with the increase of copper amount in the catalyst. We also varied the loading amount of catalyst (5, 10, 15, and 20 mg) as shown in Figure 3B. Low yield is observed for 5 mg of catalyst loading, and the yield became maximum for 10 mg of catalyst loading. After that there is saturation in the reaction and no further increase in yield was observed on increasing the catalyst loading. Hence 10 mg of Cu0.5Co2.5S4 nanosheet catalyst, 2 mmol K2CO3 base, 5 ml water/ethanol solvent, 180 min of reaction time and 25°C reaction temperature is found to be the best condition for the synthesis of diphenylaceteylenes and its derivatives in one pot reaction with maximum yield.
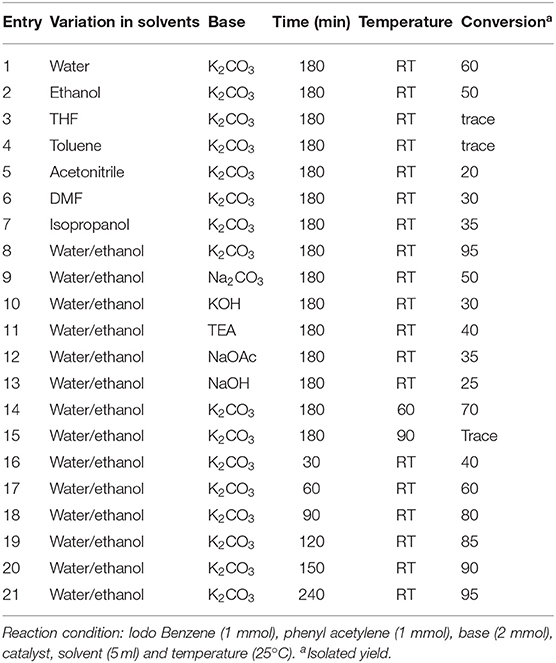
Table 2. Optimization study for variation of various solvents, base, time and temperature in the synthesis of diphenylaceteylenes.
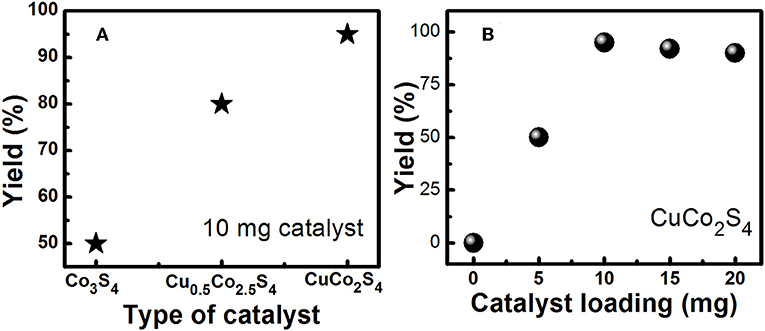
Figure 3. (A) Optimization study for variation of catalyst (10 mg catalyst, 2 mmol K2CO3, 5 ml solvent, 180 min, 25°C). (B) Optimization study for variation of catalyst loading (CuCo2S4 catalyst, 2 mmol K2CO3, 5 ml solvent, 180 min, 25°C).
From the optimized conditions, we have explored the Sonogashira reaction. The results are summarized in Table 1 (Entry 1). We have introduced different types of aryl halides containing both electron-donating and electron-withdrawing groups under the defined conditions. In general, the reactivity of aryl chlorides in the coupling reactions is low and same has been observed in the present case too where yield of the reaction decreases in the order of I > Br > Cl. However, employment of these presently delivered conditions are found to be best suitable to carry out the reactions between various types of aryl chlorides as well as aryl iodides and bromides. Observing the excellent activity of the present nanosheets catalytic system under the defined reaction conditions, in the next step, the reactions of some activated alkyl halides were investigated (Table 1, Entry 2–4). In these cases too, efficient catalytic activities are seen for CuCo2S4 nanosheet catalyst and all the reactions were completed with 100% selectivity and very good yields as noted.
We have compared our results with those of the Pd, Ni and Cu based catalysts for the Sonogashira coupling reaction, taking the example of the reaction between iodobenzene and phenylacetylene as shown in Table 3. For example Moghaddam et al. and Beletskaya et al. prepared diphenylacetylenes in 94 and 93% using nickel based catalyst (Beletskaya et al., 2003; Moghaddam et al., 2015). Stein et al. and Zhao et al. used the palladium based catalyst for the synthesis of diphenylacetylenes in the range of 82% to 94% (Stein et al., 2015; Zhao and Song, 2015). In 2004 copper salt is used to prepare diphenylacetylene in the isolated yield of 82–98% (Ma and Liu, 2004). Till date lot of catalyst had been synthesized for Sonogashira coupling and some of them give high yields of diphenylacetylenes, however, our new CuCo2S4 nanosheet catalyst is found to be excellent as compared to earlier these reports (Table 3).
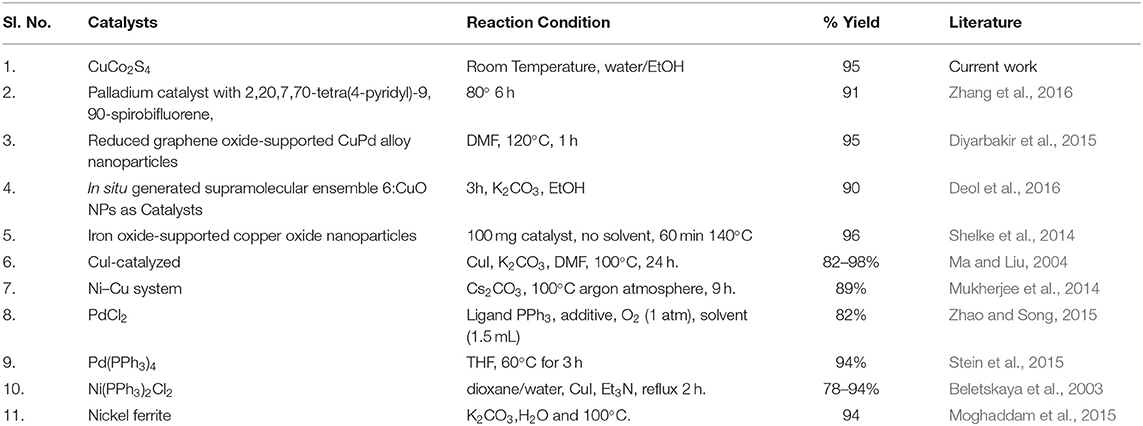
Table 3. Comparison of the catalytic properties (synthesis of diphenylaceteylenes) between present CuCo2S4 nanosheets and literature reports.
We propose a possible reaction mechanism of the Sonogashira reaction in Scheme 2. Firstly, K2CO3 base abstract the proton from the phenylacetylene and CuCo2S4 forms the Cu-acetylide intermediate. In the second step, there is a formation of intermediate of Cu-acetylide complex and aryl moiety with the elimination of halide ion. So, this step can be the oxidative addition step. Finally the formation of the dipheylacetylene as the desired product (reductive elimination) and regeneration of CuCo2S4 catalyst again. The activity of catalyst depends on defects on the surface and high surface area as corroborated from the nanosheet's surface characterization in Figure 1e. Somewhat intrinsic properties also affect the efficiency of catalyst for the organic reaction.
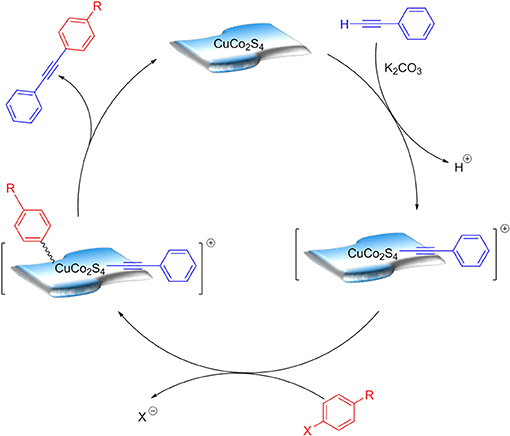
Scheme 2. A plausible mechanism of CuCo2S4 nanosheet catalyzed one-pot synthesis of diphenylacetylene.
To check the reusability and stability of the catalyst, the reaction of iodobenzene with phenyl acetylene was performed under the optimized reaction conditions. CuCo2S4 nanosheets recovered after washing with water and drying in air was reemployed in the same reaction for the second run. This process was repeated for 10 times and each time isolated yield was monitored. The conversion of reactants to product for each repeated cycle is shown in Figure 4a. These results concluded efficient recyclability of thiospinel nanosheet with the minor decrease in product yield after 10 successive cycles and the catalyst was not deactivated during the catalysis of the reactions. This negligible decrease in efficiency may be attributed to catalyst lost during successive cycles. After each test, the catalyst was recovered by centrifugation followed by washing with water and dried. For a heterogeneous catalyst it is important to retain original properties, such as crystal structure, phase, morphology, etc. Therefore, to check the structural changes in crystal structure, XRD measurement has been performed on the dried used catalysts and the obtained XRD pattern is depicted in Figure 4b. It can be seen from XRD pattern of reused CuCo2S4 that peak positions remain unchanged and matching well with the standard XRD pattern, which presents the structural stability of present nanosheet sample. The retention of nanosheet morphology or shape stability of the spent catalyst can be seen by examining the TEM images in Figures 4c,d.
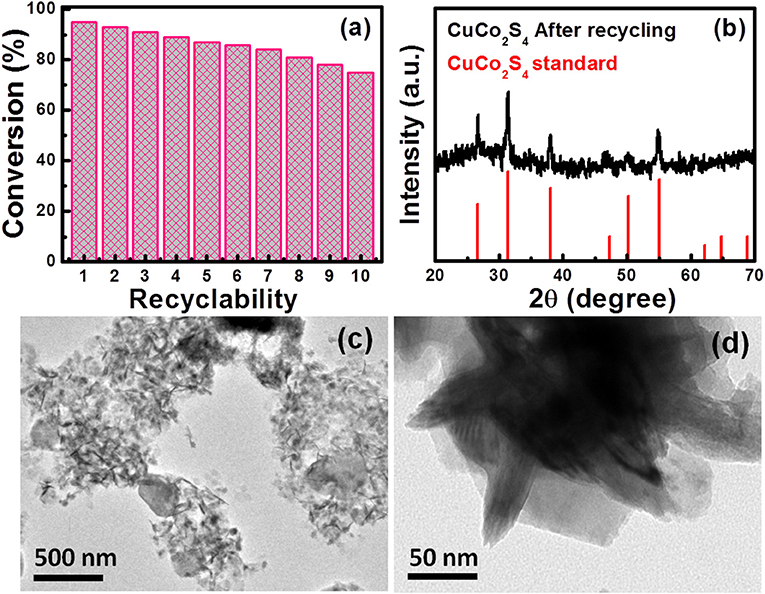
Figure 4. (a) Recycling study of CuCo2S4 catalyst for the synthesis of diphenylacetylene. (b) powder XRD pattern of CuCo2S4 nanosheet sample after performing 10 catalytic cycles. (c,d) Low and high magnification images of spent catalysts.
Conclusions
Two dimensional CuCo2S4 nanosheets have been synthesized following a new hydrothermal approach and this material is found to be the excellent heterogeneous catalyst in the Sonogashira coupling reaction for the first time. All the reactions proceeded smoothly and diphenylacetylenes were synthesized in good yields (70–95%) within 150–300 min of reaction time. This catalyst is economic, greener and works under milder condition. Further durability of the catalyst was also examined by reusing the catalyst for continuous 10 cycles where it shows only ~20% decline in the activity. In addition, this catalytic reaction procedure can be applied in pharmaceutical industries, because of the benefits of total removal of the thiospinel catalyst without any loss in their (product) strength and activity from the reaction media.
Data Availability Statement
All datasets generated for this study are included in the article/Supplementary Material.
Author Contributions
The experiments were conceived by SD. Synthesis and characterization of nanosheets was done by MC. KS performed all organic catalysis reactions. The results were interpreted and the paper written by KS, MC, and SD.
Conflict of Interest
The authors declare that the research was conducted in the absence of any commercial or financial relationships that could be construed as a potential conflict of interest.
Acknowledgments
KS and MC thanks to CSIR for providing research fellowship. SD gratefully acknowledges the financial support received from SERB-DST, New Delhi (EMR/2016/004833) and University of Delhi. The authors thank USIC-DU and AIIMS EM facility for sample characterizations.
Supplementary Material
The Supplementary Material for this article can be found online at: https://www.frontiersin.org/articles/10.3389/fmats.2019.00273/full#supplementary-material
NMR and Mass data for all the coupling products are given in supporting file.
References
Antil, B., Kumar, L., Reddy, K. P., Gopinath, C. S., and Deka, S. (2019). Direct thermal polymerization approach to N-Rich holey carbon nitride nanosheets and their promising photocatalytic H2 evolution and charge-storage activities. ACS Sustainable Chem. Eng. 7, 9428–9438 doi: 10.1021/acssuschemeng.9b00626
Beletskaya, I. P., Latyshev, G. V., Tsvetkov, A. V., and Lukashev, N. V. (2003). The nickel-catalyzed Sonogashira–Hagihara reaction. Tetrahedron Lett. 44, 5011–5013. doi: 10.1016/S0040-4039(03)01174-2
Chauhan, H., Singh, M. K., Kumar, P., Hashmi, S. A., and Deka, S. (2017a). Development of SnS2/RGO nanosheet composite for cost-effective aqueous hybrid supercapacitors. Nanotechnology 28:025401. doi: 10.1088/1361-6528/28/2/025401
Chauhan, H., Soni, K., Kumar, M., and Deka, S. (2016). Tandem photocatalysis of graphene-stacked SnS2 nanodiscs and nanosheets with efficient carrier separation. ACS Omega 1, 127–137. doi: 10.1021/acsomega.6b00042
Chauhan, M., Reddy, K. P., Gopinath, C. S., and Deka, S. (2017b). Copper cobalt sulfide nanosheets realizing a promising electrocatalytic oxygen evolution reaction. ACS Catal. 7, 5871–5879. doi: 10.1021/acscatal.7b01831
Chauhan, M., Soni, K., Karthik, P. E., Reddy, K. P., Gopinath, C. S., and Deka, S. (2019). Promising visible-light driven hydrogen production from water on a highly efficient CuCo2S4 nanosheet photocatalyst. J. Mater. Chem A. 7, 6985–6994. doi: 10.1039/C9TA00391F
Chinchilla, R., and Nájera, C. (2007). The Sonogashira reaction: a booming methodology in synthetic organic chemistry. Chem Rev. 107, 874–922. doi: 10.1021/cr050992x
Cosford, N. D., Tehrani, L., Roppe, J., Schweiger, E., Smith, N. D., Anderson, J., et al. (2003). 3-[(2-Methyl-1, 3-thiazol-4-yl) ethynyl]-pyridine: a potent and highly selective metabotropic glutamate subtype 5 receptor antagonist with anxiolytic activity. J. Med. Chem. 46, 204–206. doi: 10.1021/jm025570j
Deol, H., Pramanik, S., Kumar, M., Khan, I. A., and Bhalla, V. (2016). Supramolecular ensemble of a TICT-AIEE active pyrazine derivative and CuO NPs: a potential photocatalytic system for sonogashira couplings. ACS Catal. 6, 3771–3783. doi: 10.1021/acscatal.6b00393
Deori, K., Kalita, C., and Deka, S. (2015). (100) surface-exposed CeO2 nanocubes as an efficient heterogeneous catalyst in the tandem oxidation of benzyl alcohol, para-chlorobenzyl alcohol and toluene to the corresponding aldehydes selectively. J. Mater. Chem. A, 3, 6909–6920. doi: 10.1039/C4TA06547F
Diyarbakir, S., Can, H., and Metin, Ö. (2015). Reduced graphene oxide-supported CuPd alloy nanoparticles as efficient catalysts for the Sonogashira cross-coupling reactions. ACS Appl. Mater. Interfaces. 7, 3199–3206. doi: 10.1021/am507764u
Gunjakar, J. L., Kim, I. Y., Lee, J. M., Lee, N. S., and Hwang, S. J. (2013). Self-assembly of layered double hydroxide 2D nanoplates with graphene nanosheets: an effective way to improve the photocatalytic activity of 2D nanostructured materials for visible light-induced O2 generation. Energy Environ. Sci. 6, 1008–1017. doi: 10.1039/c3ee23989f
Li, J., Ambroise, A., Yang, S. I., Diers, J. R., Seth, J., Wack, C. R., et al. (1999). Template-directed synthesis, excited-state photodynamics, and electronic communication in a hexameric wheel of porphyrins. J. Am. Chem. Soc. 121, 8927–8940. doi: 10.1021/ja991730d
Liu, J., Jiang, J., Cheng, C., Li, H., Zhang, J., Gong, H., et al. (2011). Co3O4 nanowire@ MnO2 ultrathin nanosheet core/shell arrays: a new class of high-performance pseudocapacitive materials. Adv. Mater. 23, 2076–2081. doi: 10.1002/adma.201100058
Liu, Y., Xiao, C., Lyu, M., Lin, Y., Cai, W., Huang, P., et al. (2015). Ultrathin Co3S4 Nanosheets that synergistically engineer spin states and exposed polyhedra that promote water oxidation under neutral conditions. Angew. Chem. 127, 11383–11387. doi: 10.1002/ange.201505320
Ma, D., and Liu, F. (2004). CuI-catalyzed coupling reaction of aryl halides with terminal alkynes in the absence of palladium and phosphine. Chem. Commun. 17, 1934–1935. doi: 10.1039/b407090a
Maaliki, C., Thiery, E., and Thibonnet, J. (2017). Emergence of copper-mediated formation of C–C bonds. Eur. J. Org. Chem. 2017, 209–228. doi: 10.1002/ejoc.201600540
Mika, L. T., Cséfalvay, E., and Németh, A. (2018). Catalytic conversion of carbohydrates to initial platform chemicals: chemistry and sustainability. Chem Rev. 118, 505–613. doi: 10.1021/acs.chemrev.7b00395
Min, Y., Moon, G. D., Kim, B. S., Lim, B., Kim, J. S., Kang, C. Y., et al. (2012). Quick, controlled synthesis of ultrathin Bi2Se3 nanodiscs and nanosheets. J. Am. Chem. Soc. 134, 2872–2875. doi: 10.1021/ja209991z
Moghaddam, F. M., Tavakoli, G., and Rezvani, R. (2015). A copper-free Sonogashira reaction using nickel ferrite as catalyst in water. Catal. Commun. 60. 82–87. doi: 10.1016/j.catcom.2014.11.018
Mongin, O., Papamicaël, C., Hoyler, N., and Gossauer, A. (1998). Modular synthesis of benzene-centered porphyrin trimers and a dendritic porphyrin hexamer. J. Org. Chem. 63, 5568–5580. doi: 10.1021/jo980846+
Mongin, O., Porrès, L., Moreaux, L., Mertz, J., and Blanchard-Desce, M. (2002). Synthesis and photophysical properties of new conjugated fluorophores designed for two-photon-excited fluorescence. Org. Lett. 4, 719–722. doi: 10.1021/ol017150e
Mukherjee, N., Kundu, D., and Ranu, B. C. (2014). A co-operative Ni–Cu system for C sp–C sp and C sp–C sp2 cross-coupling providing a direct access to unsymmetrical 1, 3-diynes and en-ynes. Chem. Commun. 50,15784–15787. doi: 10.1039/C4CC07413K
Ng, J., and Raitses, Y. (2015). Self-organisation processes in the carbon arc for nanosynthesis. J. Appl. Phys. 117:063303. doi: 10.1063/1.4906784
Nicolaou, K., Bulger, P. G., and Sarlah, D. (2005). Palladium-catalyzed cross-coupling reactions in total synthesis. Angew. Chem. Int. Ed. 44, 4442–4489. doi: 10.1002/anie.200500368
Nicolaou, K., Vassilikogiannakis, G., Mägerlein, W., and Kranich, R. (2001). Total synthesis of colombiasin A and determination of its absolute configuration. Chem. Eur. J. 7, 5359–53713. doi: 10.1002/1521-3765(20011217)7:24<5359::AID-CHEM5359>3.0.CO;2-Z
Nie, L., Wang, H., Chai, Y., Liu, S., and Yuan, R. (2016). In situ formation of flower-like CuCo2S4 nanosheets/graphene composites with enhanced lithium storage properties. RSC Adv. 6, 38321–38327. doi: 10.1039/C5RA28022B
Oppolzer, W., Bienayme, H., and Genevois-Borella, A. (1991). Enantioselective synthesis of (+)-3-isorauniticine via a catalytic tandem palladium-ene/carbonylation reaction. J. Am. Chem. Soc. 113, 9660–9661. doi: 10.1021/ja00025a035
Raza, F., Park, J. H., Lee, H. R., Kim, H. I., Jeon, S. J., and Kim, J. H. (2016). Visible-light-driven oxidative coupling reactions of amines by photoactive WS2 Nanosheets. ACS Catal. 6, 2754–2759. doi: 10.1021/acscatal.5b02798
Raza, F., Yim, D., Park, J. H., Kim, H. I., Jeon, S. J., and Kim, J. H. (2017). Structuring Pd nanoparticles on 2H-WS2 nanosheets induces excellent photocatalytic activity for cross-coupling reactions under visible light. J. Am. Chem. Soc. 139, 14767–14774. doi: 10.1021/jacs.7b08619
Shelke, S. N., Bankar, S. R., Mhaske, G. R., Kadam, S. S., Murade, D. K., Bhorkade, S. B., et al. (2014). Iron oxide-supported copper oxide nanoparticles (Nanocat-Fe-CuO): magnetically recyclable catalysts for the synthesis of pyrazole derivatives, 4-methoxyaniline, and Ullmann-type condensation reactions. ACS Sustainable Chem. Eng. 2, 1699–1706. doi: 10.1021/sc500160f
Stein, A. L., Bilheri, F. N., and Zeni, G. (2015). Application of organoselenides in the Suzuki, Negishi, Sonogashira and Kumada cross-coupling reactions. Chem. Commun. 51, 15522–15525. doi: 10.1039/C5CC06347G
Tan, C., and Zhang, H. (2015). Epitaxial growth of hetero-nanostructures based on ultrathin two-dimensional nanosheets. J. Am. Chem. Soc. 137, 12162–12174. doi: 10.1021/jacs.5b03590
Thathagar, M. B., Beckers, J., and Rothenberg, G. (2004). Palladium-free and ligand-free Sonogashira cross-coupling. Green Chem. 6, 215–218. doi: 10.1039/b401586j
Toyota, M., Komori, C., and Ihara, M. (2000). A concise formal total synthesis of mappicine and nothapodytine B via an intramolecular hetero diels–alder reaction, J. Org. Chem. 65, 7110–7113. doi: 10.1021/jo000816i
Tykwinski, R. R. (2003). Evolution in the palladium-catalyzed cross-coupling of sp-and sp2-hybridized carbon atoms. Angew. Chem. Int. Ed. 42, 1566–1568. doi: 10.1002/anie.200201617
Zhang, J., Li, T., Zhao, X., Zhao, Y., Li, F., and Li, X. (2016). High catalytic active palladium nanoparticles gradually discharged from multilayer films to promote Suzuki, Heck and Sonogashira cross coupling reactions. J. Colloid Interf. Sci. 463, 13–21. doi: 10.1016/j.jcis.2015.10.035
Zhao, Y., and Song, Q. (2015). Palladium-catalyzed aerobic oxidative cross-coupling of arylhydrazines with terminal alkynes. Chem. Commun. 51, 13272–13274. doi: 10.1039/C5CC04111B
Keywords: CuCo2S4, nanosheet, thiospinel two-dimensional (2D), sonogashira coupling, cross-coupling reaction
Citation: Soni K, Chauhan M and Deka S (2019) Hydrothermally Synthesized CuCo2S4 Nanosheets as an Easily Accessible and Convenient Heterogeneous Catalyst for the Sonogashira Cross-Coupling Reactions. Front. Mater. 6:273. doi: 10.3389/fmats.2019.00273
Received: 01 August 2019; Accepted: 16 October 2019;
Published: 01 November 2019.
Edited by:
Jiatao Zhang, Beijing Institute of Technology, ChinaReviewed by:
Kebin Zhou, University of Chinese Academy of Sciences, ChinaZhongbin Zhuang, Beijing University of Chemical Technology, China
Copyright © 2019 Soni, Chauhan and Deka. This is an open-access article distributed under the terms of the Creative Commons Attribution License (CC BY). The use, distribution or reproduction in other forums is permitted, provided the original author(s) and the copyright owner(s) are credited and that the original publication in this journal is cited, in accordance with accepted academic practice. No use, distribution or reproduction is permitted which does not comply with these terms.
*Correspondence: Sasanka Deka, c2Rla2EmI3gwMDA0MDtjaGVtaXN0cnkuZHUuYWMuaW4=; c3NkZWthJiN4MDAwNDA7Z21haWwuY29t
†ORCID: Sasanka Deka orcid.org/0000-0003-4794-0406