- 1College of Engineering and Technology, Southwest University, Chongqing, China
- 2Chongqing Electric Power Research Institute, Chongqing, China
- 3College of Materials Science and Engineering, Chongqing University, Chongqing, China
Hollow structured nanofibers have attracted much attention in diverse domains owing to their unique physicochemical properties and characteristics. Gas sensing is one of the most promising applications. In electrical engineering, the detection of SF6 decomposing gas products is significant to monitor the insulation status online of gas insulated switchgear (GIS). This mini-review presents the developments of hollow structured nanofibers in synthesis strategies and gas sensing application, especially in the detection of SF6 decomposing gas products, including hydrogen disulfide (H2S), sulfur dioxide (SO2), thionyl fluoride (SOF2), and sulfuryl fluoride (SO2F2). In addition, the gas sensing mechanism of metal oxide hollow nanofibers based gas sensor are discussed.
Introduction
Sulfur hexafluoride (SF6) as an insulating gas is commonly utilized in gas insulated switchgear (GIS) (Zhang et al., 2018a). Though SF6 has high stability at normal operating temperatures, parts of the SF6 molecules would react with little water and solid insulated material under electrical stress conditions caused by arc, spark, and partial discharge, and then decompose to a variety of typical decomposition byproducts including SO2, H2S, SOF2, SO2F2, SOF4, S2F10, HF gases, and solid byproducts (Li et al., 2018; Zhou et al., 2018a; Chen et al., 2019c). This can lead to insulating property reduction of GIS. Furthermore, the research results showed that the type, concentration, and formation regularity of the SF6 decomposition byproducts and the severity of the insulation fault have the close correlation (Tang et al., 2018; Zhang et al., 2018b). Therefore, the detection of the typical decomposition components of SF6 gas is significant for identifying and diagnosing the insulation faults of the GIS. Over the past few years, photoacoustic (PA) spectroscopy (Luo et al., 2015), gas chromatography (Jong et al., 2015), and infrared absorption spectrometry (Dong et al., 2017) have been applied for the detection of the decomposition components of SF6. Nevertheless, the preceding three methods only monitor the insulation status offline. With the development of gas sensitive materials, gas sensors for detecting SF6 decomposing products have developed further in recent years. Gas sensors detection methods have grown to analyzing the insulation status of GIS online (Liu et al., 2017; Lu et al., 2018; Cui et al., 2019).
There are a variety of gas-sensitive materials that can be made into SF6 decomposition component gas sensor, including semiconducting oxides, carbon nanotubes, molybdenum disulfide, etc. (Chen et al., 2018, 2019a,b). Nanostructure materials received extensive attention recent years due to the outstanding physical and chemical properties (Xu et al., 2017b,c; Zhou et al., 2018b). Hollow structured nanofibers as potential gas sensing nanomaterials have attracted increasing research due to their extensive specific surface areas, larger accessible active area, and higher aspect ratios (Zhang et al., 2009; Li et al., 2017; Diltemiz and Ecevit, 2019). Although the fabrication and application of hollow nanofibers have been well reviewed (Kenry and Lim, 2017; Thenmozhi et al., 2017). Few studies focused on the application of detecting SF6 decomposing gas products in the field of electrical engineering, which developed in recent years. In this mini review, synthesis strategies of novel hollow nanofibers and their gas sensing application, especially detecting SF6 decomposing gas products, are introduced.
Synthesis Strategies of Hollow Nanofibers
Hollow Nanofibers Based on Template Synthesis
Up to now, template synthesis, self-assembly, and electrospinning technique have been employed for producing the hollow structured nanofibers (Khajavi and Abbasipour, 2012; Wang and Lu, 2012; Kim et al., 2019). Among them, the template synthesis method is more adaptable, because it is easy to control the width of the middle hollow (Homaeigohar et al., 2017). As shown in Figure 1A, the template is mostly prepared by electrospining. Then the target precursor is coated or deposited on the prepared template. The employed deposition technique includes atomic layer, electrochemistry, chemical vapor deposition, and so on. The last process is calcination in order to remove the core template fibers. Donmez et al. fabricated hafnia hollow nanofibers by atomic layer deposition using electrospun nylon 6,6 nanofibers as templates (Donmez et al., 2013). Hollow TiO2 nanofibers were fabricated by using oxidized electrospun polyacrylonitrile fibers as templates (Ouyang et al., 2018). Han et al. used controllable sacrifice template route to synthesize polyaniline hollow nanofibers with adjustable hollow structure (Han et al., 2019b). Kim et al. successfully synthesized ultra-thin SiO2 hollow nanofibers with surface-replicated morphologies using herringbone-, platelet-, and tubular-type carbon nanofibers templates (Kim et al., 2019). The template synthesis methods can create hollow nanofibers of different diameters and hollow structure but cannot prepare them continuously.
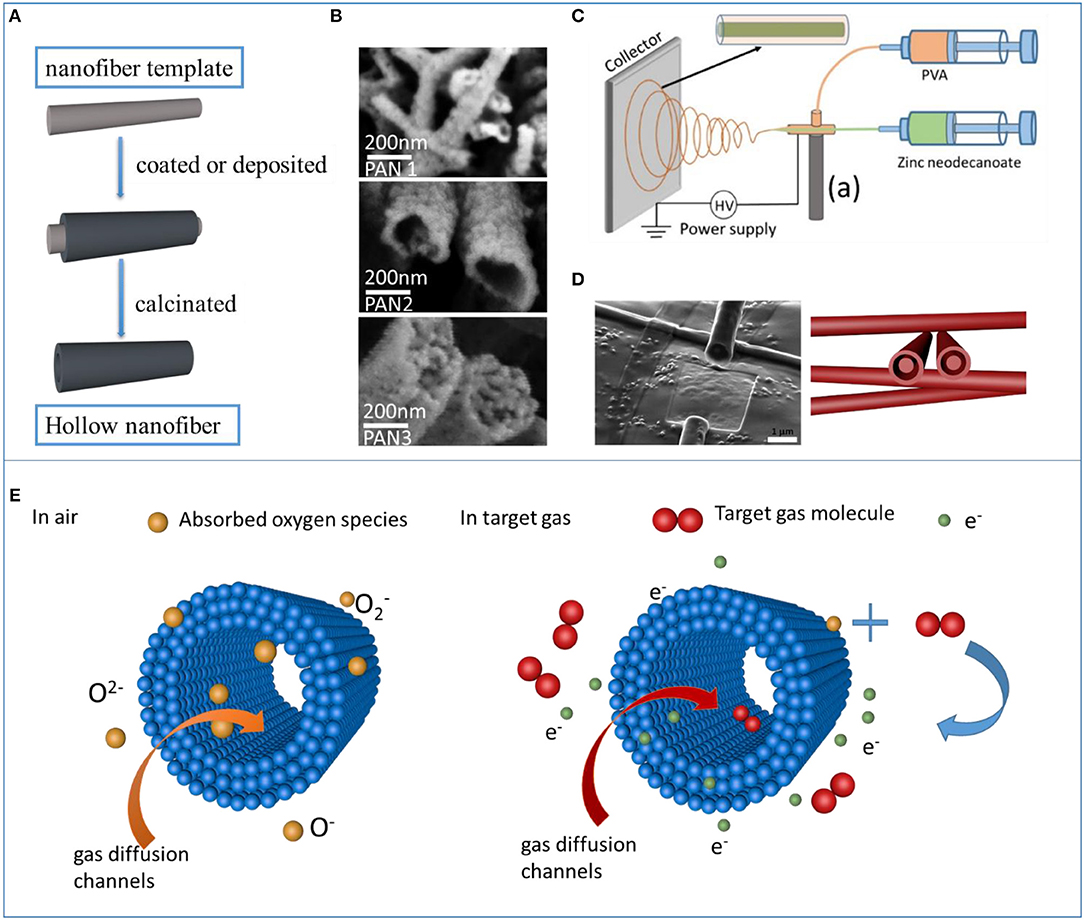
Figure 1. (A) A schematic of template synthesis method. (B) SEM images of the SnO2 nanofibers electrospun. Reprinted with permission from Ab Kadir et al. (2014). (C) A schematic of the coaxial electrospinning. Reprinted with permission from Aziz et al. (2018). (D) SEM and 3D structure of coaxial core–sheath structured hollow nanofibers. Reprinted with permission from Liu et al. (2013). (E) Schematic illustration of hollow nanofibers gas sensing.
Hollow Nanofibers Based on Self-Assembly Methods
The self-assembly technique has attracted wide interest and employed to form polymers owing to the omitting preparation of template and post-treatment of removing template. There have been many studies on preparing nanotubes. However, there is very little research on hollow nanofiber structured polymers fabricated by self-assembly because of the special continuous hollow structure. Wang et al. successfully prepared polyaniline nanomaterials with different morphologies including 1D-nanotubes, 3D-nanofiber by simply changing the concentration of aniline and the molar ratio of dichloroacetic acid to aniline. They found that strong hydrogen bonding effect between dichloroacetic and aniline may result in the formation of self-assembly nanofibers networks, and thus producing phenazine-like units to further self-assembly (Wang and Lu, 2012).
Hollow Nanofibers Based on Electrospinning Methods
Compared with template synthesis and self-assembly methods which need complex manipulations of molecules to form the nanofibers structure, electrospinning is the most straightforward, and efficient method to prepare continuous hollow nanofibers (Xia et al., 2012). Firstly, the high voltage is applied between a positively-charged syringe needle and a grounded collector. Then, the charged solution is stretch under the electronic forces to produce nanofibers. Usually, in order to obtain hollow nanofibers by electrospinning, the nanofibers with core-shell structure should be prepared firstly. Then, the inner layer material is removed by heat treatment or solvent extraction to obtain the hollow structured nanofibers (Di et al., 2008; Zhang and Hsieh, 2009). Many kinds of electrospinning apparatus and methods including single spinneret, coaxial, triaxial, microfluidic, and emulsion electrospinning have been applied to prepare hollow nanofibers (Wei et al., 2011; Zhang et al., 2019). The mechanism of single spinneret electrospinning to produce hollow nanofibers is the phase separation (Wu et al., 2017). Tang et al. fabricated multichannel hollow TiO2 nanofibers by single-nozzle electrospinning. The hollow structure was formed because the polyvinyl pyrrolidone (PVP) and polyacrylonitrile (PAN) are immiscible caused phase separation (Tang et al., 2013). Ab Kadir et al. prepared hollow SnO2 nanofibers and demonstrated that the diameter of nanofibers depends on the polymer concentration in the electrospinning solution (Ab Kadir et al., 2014). The SEM is shown in Figure 1B. The PAN concentration of three kinds of samples is different from PAN 1 to PAN 3 (the ratio of them is 1:2:3). The results showed that with the increase of solution concentration from PAN 1 to PAN 2 and PAN 3, thicker nanofibers will be formed. As shown in SEM of PAN 3, the nanostructure is filled nanofibers. That is because the stirring speed is different from PAN 1 and PAN2 to PAN 3 which is faster than the others. As the stirring speed increases, the droplet diameter of PAN decreases, and the PAN which are supposed to form the core of the nanofibers will disperse in the PVP solution more randomly. Coaxial electrospinning technology is more flexible to form hollow nanofiber. The experimental setup has been shown in Figure 1C (Aziz et al., 2018). The coaxial electrospinning technology follows the traditional electrospinning principal, just replaces the traditional single spinneret with a spinneret composed of two coaxial capillaries. Compared with single spinneret electrospinning which can only produce hollow nanofibers of a limited kind of material, the advantages of coaxial electrospinning is that a diverse range of materials can be made into hollow nanofibers (Yoon et al., 2018). Hyun et al. utilized the coaxial electrospinning technology to prepare solid, hollow, multi-channel, and hollow multi-channel nanofibers by adjusting the shell-core spinning solution and combining the post-heat treatment process (Hyun et al., 2016). To format nanofibers with higher surface areas, the triaxial electrospinning was developed in recent years. The triaxial electrospinning has been widely used in the field of biomedical applications. Liu et al. designed a triaxial electrospinning system and successfully developed a biodegradable multilayer nanofiber (Liu et al., 2013). The structure of triaxial electrospinning can produce coaxial core–sheath structured hollow nanofibers as shown in Figure 1D. The spinneret of microfluidic electrospinning apparatus consists of several inner capillaries. This method is mainly used in biomedical and textile field (Chae et al., 2013). Emulsion electrospinning spinning can be used to produce hollow nanofibers without changing the spinneret, but the continuity of the prepared nanofibers is low (Wei et al., 2015).
Up to now, diverse electrospun hollow nanofibers which are made of a range of materials such as polymers, ceramics, semiconductors, and composites have been made for different application including batteries, hydrogen storage and generation, biological sensing, and gas sensing. In the next part of this mini review, we focus on the gas sensing application, especially the SF6 decomposing gas products.
Application of Hollow Nanofibers in Gas Sensing
Gas sensing is one of important applications of hollow nanofibers. Among gas sensing materials, semiconducting metal oxides such as SnO2, ZnO, TiO2, and CuO are applied the most widely in gas sensors due to the excellent gas sensing properties in terms of response, response and recovery times, and stability (Xu et al., 2017a; Zhou et al., 2018c; Wang et al., 2019a). The higher specific surface area of sensing materials can provide the higher sensitivity. The surface area of hollow structured nanofibers is around two times than that of solid nanofibers (Wali et al., 2014). Therefore, the gas sensing applications such as the detection of volatile organic compounds, inflammable gases, and toxic gases of hollow nanofibers, have been activity studied in recent years. Katoch et al. reported that the ZnO hollow fibers with smaller hole diameters show higher gas sensitivity to both oxidizing and reducing gases than those with larger hole diameters. The improved gas sensing properties is attributed to the increased surface area of the nanomaterials (Katoch et al., 2016). Besides increasing the active surface area, by doping metallic nanoparticles or constructing nano-heterojunction can also improve the sensing capability especially enhance the selectivity of gas sensor. Gas sensor produced by electrospining of pure ZnO hollow nanofibers and Ce doped ZnO hollow nanofibers have been successfully synthesized and their gas sensing properties were measured. The result showed that Ce doping can improve the response performance toward acetone, ethanol, acetic acid, DMF and ammonia (Li et al., 2015). In addition, 0.6 wt% Pr-doped SnO2 hollow nanofibers exhibit the higher response of ethanol (Li et al., 2014). ZnO/CoNiO2 composite hollow nanofibers offered a good choice of ammonia gas sensing materials (Alali et al., 2017). Besides, the metallic nanoparticles doping concentration obviously affects specific gas sensitivity. Choi et al. reported 0.08 and 0.4 wt% Pd doped SnO2 hollow nanofibers increase and decrease the sensitive of C2H5OH, respectively (Choi et al., 2010).
Gas Sensing Mechanism
The gas sensing mechanism of the hollow nanofibers materials is relevant to the reactions between the target gas molecule and the oxygen species pre-adsorbed (, O−, O2−) on the surface as shown in Figure 1E. When the sensor is exposed in air, oxygen molecules on the surface of nanomaterials convert into surface adsorbed oxygen species including, O−, and O2− by capturing electrons from conduction band of the nanomaterials. Therefore, the electron depletion region extends and it can lead to the increase of the gas sensor resistance. The reaction can be described as follow (Li et al., 2014):
When the sensor is exposed to the target gases, these gas molecules react with adsorbed oxygen and then release the electrons back to the conduction band of nanomaterials. Thus, the resistance of the sensor decreases at target gas atmosphere. The following equations take H2S and SO2 gas as examples (Han et al., 2019a; Queraltó et al., 2019):
The high specific surface area offered by inner and outer walls of hollow nanofibers provides more active centers of gas molecules adsorption. In addition, the sufficient diffusion channels makes it easier for gas molecules to diffuse into the inner surface.
Hollow Nanofibers Sensor for the SF6 Decomposing Products
For the SF6 decomposition component, current research efforts are focused on the detection of SO2 and H2S gases. Combined with the research, some of the typical gas sensing performance of SO2 and H2S sensors are listed in Table 1.
S, the gas sensing sensitivity of the gas sensor. The S was determined by the formula in the Table 1. Ra is the resistance of sensor in air. Rg is the resistance of sensor in SO2 or H2S gas.
As shown in the table above, compared with filled nanofibers or other nanostructure, hollow structured nanofibers sensors show excellent gas sensitivity, and lower working temperature to H2S and SO2. Albert Queralto et al. prepared single phase LaFeO3 (LFO) perovskite nanofibers for high detection of sulfur-containing gases. The detection limitation of H2S and SO2 was as low as 0.5 ppm at 250°C (Queraltó et al., 2019). Nanomaterials decorated CuO such as CuO-ZnO and CuO-SnO are potential H2S gas sensing materials. The 0.04 CuO-ZnO composite hollow spheres prepared by Kim et al. exhibited high response to 5 ppm H2S (Ra/Rg = 32.4) at 336°C (Kim et al., 2012). 0.3 CuO-ZnO composite hollow nanofibers showed high performance to H2S gas detection at 250°C (Han et al., 2019a). The response value of CuO modified hollow SnO2 nanofibers gas sensor to 10 ppm H2S gas reached 410 at 125°C. And this sensor is almost no response to other gases when the temperature is lower than 200°C indicating the excellent selectivity toward H2S gas (Yang et al., 2017). Liang et al. synthesized CuO-loaded In2O3 hollow nanofibers for H2S gas sensing application. It is observed that the sensor shows high gas response (9.17 × 103 toward 5 ppm H2S) at room temperature (Liang et al., 2015). The high response of hollow nanofibers decorated CuO can be attributed to the specific interaction between H2S molecular and CuO nanoparticles. The reaction between CuO and H2S is as follow:
At present, there are limited researches on the detection of other SF6 decomposing products such as SOF2 and SO2F2. Present research mainly focus on the sensor with nanotube structure. Zhang et al. were devoted to the application of TiO2 nanotubes and carbon nanotubes sensors to monitor SF6 decomposition byproducts online of GIS equipment. The team researched the adsorption process between gas sensing material (including functional group-modified or metal/non-metal-doped carbon nanotubes) and SF6 decomposing products based on density functional theory. For example, they investigated the adsorption process between four types (H2S, SO2, SOF2, and SO2F2) of SF6 decomposing products and Co-doped single-walled carbon nanotubes (SWCNT) using density functional theory calculations. The result showed that the adsorption leads to the obvious bond breakings of SOF2 and SO2F2. The conductance of Co-SWCNT changed significantly when the SOF2 or SO2F2 gases absorbed on the sensing materials, which indicated that the Co-SWCNT is a promising SOF2 or SO2F2 gas sensor (Zhang et al., 2017). Gui et al. studied the adsorption mechanism between N-doped SWCNT and SOF2, SO2F2 gases. The N doping reduces the adsorption capacity of N-doped SWCNT to SOF2, SO2F2 gases. Thus, the problem of poisoning sensor can be fixed (Gui et al., 2018). The team also discussed TiO2 nanotube arrays and the adsorption of SO2, SOF2, and SO2F2 on different surface of TiO2. The gas sensing responses of Pt, Au- modified TiO2 are better than pure TiO2 (Zhang et al., 2016). Although the most of present studies focus on the TiO2 and carbon nanotubes, the hollow structure and density functional theory calculations can inspire further research on the detection of SF6 decomposing products. Meanwhile, the adsorption process between traditional metal oxide semiconductor and SF6 decomposing products has been researched based on density functional theory. Wang et al. reported that Ni dopant can improve the adsorption capacity and selectivity of ZnO materials to SO2, SOF2, SO2F2 gases (Wang et al., 2019b). The authors believe that the hollow nanofibers for SF6 decomposing products detection application is feasible.
Conclusion
In this mini review, the synthesis strategies and gas sensing application of hollow structured nanofibers have been summarized, especially the detection of SF6 decomposing products. Various gas sensors based on hollow nanofibers materials with ultrahigh response value and low detection limit have been applied to gas detection. The hollow structure provides more diffusion channels and active surface area between target gas and gas sensing nanomaterials. It can be foreseen that more hollow nanofibers gas sensing materials will be synthesized and applied in the near future. Although achieving good performance, there is still a lot of room for development. Further enlarging the surface area of nanofibers is an effective way to enhance gas sensing properties. For example, the multichannel hollow nanofibers with more diffusion channels for gas spreading can be applied in gas sensing tests. In addition, the further study on high performance gas sensor and its application in the detection of SF6 decomposing products should be investigated, aiming to the actual industrial application in SF6 insulated equipment.
Author Contributions
All authors listed have made a substantial, direct and intellectual contribution to the work, and approved it for publication.
Funding
This work has been supported in part by the National Natural Science Foundation of China (No. 51507144), Fundamental Research Funds for the Central Universities (No. XDJK2019B021), the artificial intelligence key project of Chongqing (No. cstc2017rgzn-zdyfX0030), the Chongqing Science and Technology Commission (CSTC) (No. cstc2016jcyjA0400), and the project of China Scholarship Council (CSC).
Conflict of Interest Statement
The authors declare that the research was conducted in the absence of any commercial or financial relationships that could be construed as a potential conflict of interest.
References
Ab Kadir, R., Li, Z., Sadek, A. Z., Abdul Rani, R., Zoolfakar, A. S., Field, M. R., et al. (2014). Electrospun granular hollow SnO2 nanofibers hydrogen gas sensors operating at low temperatures. J. Phys. Chem. C 118, 3129–3139. doi: 10.1021/jp411552z
Alali, K. T., Liu, T., Liu, J., Liu, Q., Fertassi, M. A., Li, Z., et al. (2017). Preparation and characterization of ZnO/CoNiO2 hollow nanofibers by electrospinning method with enhanced gas sensing properties. J. Alloy. Compd. 702, 20–30. doi: 10.1016/j.jallcom.2017.01.167
Aziz, A., Tiwale, N., Hodge, S. A., Attwood, S. J., Divitini, G., and Welland, M. E. (2018). Core-shell electrospun polycrystalline ZnO nanofibers for ultra-sensitive NO2 gas sensing. ACS Appl. Mater. Inter. 10, 43817–43823. doi: 10.1021/acsami.8b17149
Chae, S. K., Kang, E., Khademhosseini, A., and Lee, S. H. (2013). Micro/Nanometer-scale fiber with highly ordered structures by mimicking the spinning process of silkworm. Adv. Mater. 25, 3071–3078. doi: 10.1002/adma.201300837
Chen, D., Tang, J., Zhang, X., Li, Y., and Liu, H. (2019a). Detecting decompositions of sulfur hexafluoride using MoS2 monolayer as gas sensor. IEEE Sens. J. 19, 39–46. doi: 10.1109/JSEN.2018.2876637
Chen, D., Zhang, X., Tang, J., Cui, H., and Li, Y. (2018). Noble metal (Pt or Au)-doped monolayer MoS2 as a promising adsorbent and gas-sensing material to SO2, SOF2, and SO2F2: a DFT study. Appl. Phys. A-Mater. 124:194. doi: 10.1007/s00339-018-1629-y
Chen, D., Zhang, X., Tang, J., Cui, H., Li, Y., Zhang, G., et al. (2019b). Density functional theory study of small Ag cluster adsorbed on graphyne. Appl. Surf. Sci. 465, 93–102. doi: 10.1016/j.apsusc.2018.09.096
Chen, D., Zhang, X., Tang, J., Cui, Z., and Cui, H. (2019c). Pristine and Cu decorated hexagonal InN monolayer, a promising candidate to detect and scavenge SF6 decompositions based on first-principle study. J. Hazard. Mater. 363, 346–357. doi: 10.1016/j.jhazmat.2018.10.006
Choi, J.-K., Hwang, I.-S., Kim, S.-J., Park, J.-S., Park, S.-S., Jeong, U., et al. (2010). Design of selective gas sensors using electrospun Pd-doped SnO2 hollow nanofibers. Sens. Actuators B Chem. 150, 191–199. doi: 10.1016/j.snb.2010.07.013
Cui, H., Zhang, X., Zhang, G., and Tang, J. (2019). Pd-doped MoS2 monolayer: a promising candidate for DGA in transformer oil based on DFT method. Appl. Surf. Sci. 470, 1035–1042. doi: 10.1016/j.apsusc.2018.11.230
Di, J., Chen, H., Wang, X., Zhao, Y., Jiang, L., Yu, J., et al. (2008). Fabrication of zeolite hollow fibers by coaxial electrospinning. Chem. Mater. 20, 3543–3545. doi: 10.1021/cm8006809
Diltemiz, S. E. R., and Ecevit, K. (2019). High-performance formaldehyde adsorption on CuO/ZnO composite nanofiber coated QCM sensors. J. Alloy. Compd. 783, 608–616. doi: 10.1016/j.jallcom.2018.12.237
Dong, M., Zhang, C., Ren, M., Albarracin, R., and Ye, R. (2017). Electrochemical and infrared absorption spectroscopy detection of SF6 decomposition products. Sensors 17:2627. doi: 10.3390/s17112627
Donmez, I., Kayaci, F., Ozgit-Akgun, C., Uyar, T., and Biyikli, N. (2013). Fabrication of hafnia hollow nanofibers by atomic layer deposition using electrospun nanofiber templates. J. Alloy. Compd. 559, 146–151. doi: 10.1016/j.jallcom.2013.01.064
Gui, Y. G., Tang, C., Zhou, Q., Xu, L. N., Zhao, Z. Y., and Zhang, X. X. (2018). The sensing mechanism of N-doped SWCNTs toward SF6 decomposition products: a first-principle study. Appl. Surf. Sci. 440, 846–852. doi: 10.1016/j.apsusc.2018.01.247
Han, C., Li, X., Shao, C., Li, X., Ma, J., Zhang, X., et al. (2019a). Composition-controllable p-CuO/n-ZnO hollow nanofibers for high-performance H2S detection. Sens. Actuators B Chem. 285, 495–503. doi: 10.1016/j.snb.2019.01.077
Han, H., Lu, H., Jiang, X., Zhong, F., Ai, X., Yang, H., et al. (2019b). Polyaniline hollow nanofibers prepared by controllable sacrifice-template route as high-performance cathode materials for sodium-ion batteries. Electrochim. Acta 301, 352–358. doi: 10.1016/j.electacta.2019.02.002
Homaeigohar, S., Davoudpour, Y., Habibi, Y., and Elbahri, M. (2017). The electrospun ceramic hollow nanofibers. Nanomaterials 7:383. doi: 10.3390/nano7110383
Hyun, B. G., Son, H. J., Ji, S., Jang, J., Hur, S.-H., and Park, J.-U. (2016). Multi-dimensional carbon nanofibers for supercapacitor electrodes. J. Electroceram 38, 43–50. doi: 10.1007/s10832-016-0055-9
Jong, E. C., Macek, P. V., Perera, I. E., Luxbacher, K. D., and Mcnair, H. M. (2015). Ultra-trace analysis technique for SF6 using gas chromatography with negative ion chemical ionization mass spectrometry. J. Chromatogr. Sci. 53, 854–859. doi: 10.1093/chromsci/bmu154
Katoch, A., Abideen, Z. U., Kim, J.-H., and Kim, S. S. (2016). Influence of hollowness variation on the gas-sensing properties of ZnO hollow nanofibers. Sens. Actuators B Chem. 232, 698–704. doi: 10.1016/j.snb.2016.04.013
Kenry, and Lim, C. T. (2017). Nanofiber technology: current status and emerging developments. Prog. Polym. Sci. 70, 1–17. doi: 10.1016/j.progpolymsci.2017.03.002
Khajavi, R., and Abbasipour, M. (2012). Electrospinning as a versatile method for fabricating coreshell, hollow and porous nanofibers. Sci. Iran. 19, 2029–2034. doi: 10.1016/j.scient.2012.10.037
Kim, S.-J., Na, C. W., Hwang, I.-S., and Lee, J.-H. (2012). One-pot hydrothermal synthesis of CuO–ZnO composite hollow spheres for selective H2S detection. Sens. Actuators B Chem. 168, 83–89. doi: 10.1016/j.snb.2012.01.045
Kim, T., Naoki, W., Miyawaki, J., Park, J.-I., Lee, C., Jung, H.-K., et al. (2019). Synthesis of surface-replicated ultra-thin silica hollow nanofibers using structurally different carbon nanofibers as templates. J. Solid State Chem. 272, 21–26. doi: 10.1016/j.jssc.2019.01.025
Li, L., Peng, S., Lee, J. K. Y., Ji, D., Srinivasan, M., and Ramakrishna, S. (2017). Electrospun hollow nanofibers for advanced secondary batteries. Nano. Energy. 39, 111–139. doi: 10.1016/j.nanoen.2017.06.050
Li, W., Man, S., Yang, G., Mao, Y., Luo, J., Cheng, L., et al. (2015). Preparation, characterization and gas sensing properties of pure and Ce doped ZnO hollow nanofibers. Mater. Lett. 138, 188–191. doi: 10.1016/j.matlet.2014.09.130
Li, W. Q., Ma, S. Y., Li, Y. F., Li, X. B., Wang, C. Y., Yang, X. H., et al. (2014). Preparation of Pr-doped SnO2 hollow nanofibers by electrospinning method and their gas sensing properties. J. Alloy. Compd. 605, 80–88. doi: 10.1016/j.jallcom.2014.03.182
Li, Y., Zhang, X., Xiao, S., Chen, Q., Tang, J., Chen, D., et al. (2018). Decomposition properties of C4F7N/N2 gas mixture: an environmentally friendly gas to replace SF6. Ind. Eng. Chem. Res. 57, 5173–5182. doi: 10.1021/acs.iecr.8b00010
Liang, X., Kim, T. H., Yoon, J. W., Kwak, C. H., and Lee, J. H. (2015). Ultrasensitive and ultraselective detection of H2S using electrospun CuO-loaded In2O3 nanofiber sensors assisted by pulse heating. Sens. Actuator B Chem. 209, 934–942. doi: 10.1016/j.snb.2014.11.130
Liu, H. C., Zhou, Q., Zhang, Q. Y., Hong, C. X., Xu, L. N., Jin, L. F., et al. (2017). Synthesis, characterization and enhanced sensing properties of a NiO/ZnO p–n junctions sensor for the SF6 decomposition byproducts SO2, SO2F2, and SOF2. Sensors 17:913. doi: 10.3390/s17040913
Liu, W., Ni, C., Chase, D. B., and Rabolt, J. F. (2013). Preparation of multilayer biodegradable nanofibers by triaxial electrospinning. ACS Macro Lett. 2, 466–468. doi: 10.1021/mz4000688
Lu, Z., Zhou, Q., Wang, C., Wei, Z., Xu, L., and Gui, Y. (2018). Electrospun ZnO-SnO2 composite nanofibers and enhanced sensing properties to SF6 decomposition byproduct H2S. Front. Chem. 6:540. doi: 10.3389/fchem.2018.00540
Luo, J., Fang, Y. H., Su, Z. X., Li, D. C., Zhao, Y. D., Wang, A. J., et al. (2015). The research of temperature properties of photoacoustic spectroscopy detection for SF6 decomposition products in gas insulated switchgear. Anal. Methods-UK 7, 3806–3813. doi: 10.1039/C5AY00585J
Ouyang, W., Liu, S., Yao, K., Zhao, L., Cao, L., Jiang, S., et al. (2018). Ultrafine hollow TiO2 nanofibers from core-shell composite fibers and their photocatalytic properties. Compos. Commun. 9, 76–80. doi: 10.1016/j.coco.2018.06.006
Queraltó, A., Graf, D., Frohnhoven, R., Fischer, T., Vanrompay, H., Bals, S., et al. (2019). LaFeO3 Nanofibers for high detection of sulfur-containing gases. ACS Sust. Chem. Eng. 7, 6023–6032. doi: 10.1021/acssuschemeng.8b06132
Tang, J., Yang, X., Yao, Q., Miao, Y., She, X., and Zeng, F. (2018). Correlation analysis between SF6 decomposed components and negative DC partial discharge strength initiated by needle-plate defect. IEEJ T. Electr. Electr. 13, 382–389. doi: 10.1002/tee.22579
Tang, K., Yu, Y., Mu, X., Van Aken, P. A., and Maier, J. (2013). Multichannel hollow TiO2 nanofibers fabricated by single-nozzle electrospinning and their application for fast lithium storage. Electrochem. Commun. 28, 54–57. doi: 10.1016/j.elecom.2012.12.005
Thenmozhi, S., Dharmaraj, N., Kadirvelu, K., and Kim, H. Y. (2017). Electrospun nanofibers: new generation materials for advanced applications. Mat. Sci. Eng. B 217, 36–48. doi: 10.1016/j.mseb.2017.01.001
Wali, Q., Fakharuddin, A., Ahmed, I., Ab Rahim, M. H., Ismail, J., and Jose, R. (2014). Multiporous nanofibers of SnO2 by electrospinning for high efficiency dye-sensitized solar cells. J. Mater. Chem. A 2, 17427–17434. doi: 10.1039/C4TA03056G
Wang, H., and Lu, Y. (2012). Morphological control of self-assembly polyaniline micro/nano-structures using dichloroacetic acid. Synthetic Met. 162, 1369–1374. doi: 10.1016/j.synthmet.2012.05.024
Wang, J. X., Zhou, Q., Lu, Z., Wei, Z., and Zeng, W. (2019a). Gas sensing performances and mechanism at atomic level of Au-MoS2 microspheres. Appl. Surf. Sci. 490, 124–136. doi: 10.1016/j.apsusc.2019.06.075
Wang, J. X., Zhou, Q., and Zeng, W. (2019b). Competitive adsorption of SF6 decompositions on Ni-doped ZnO (100) surface: computational and experimental study. Appl. Surf. Sci. 479,185–197. doi: 10.1016/j.apsusc.2019.01.255
Wei, J., Shi, H., Zhou, M., Song, D., Zhang, Y., Pan, X., et al. (2015). Effect of oil on the morphology and photocatalysis of emulsion electrospun titanium dioxide nanomaterials. Appl. Catal. A-Gen. 499, 101–108. doi: 10.1016/j.apcata.2015.03.006
Wei, S., Zhou, M., and Du, W. (2011). Improved acetone sensing properties of ZnO hollow nanofibers by single capillary electrospinning. Sens. Actuators B Chem. 160, 753–759. doi: 10.1016/j.snb.2011.08.059
Wu, Y., Jiang, Y., Shi, J., Gu, L., and Yu, Y. (2017). Multichannel porous TiO2 hollow nanofibers with rich oxygen vacancies and high grain boundary density enabling superior sodium storage performance. Small 13:1700129. doi: 10.1002/smll.201700129
Xia, X., Dong, X. J., Wei, Q. F., Cai, Y. B., and Lu, K. Y. (2012). Formation mechanism of porous hollow SnO2 nanofibers prepared by one-step electrospinning. Express Polym. Lett. 6, 169–176. doi: 10.3144/expresspolymlett.2012.18
Xu, K., Yang, L., Yang, Y., and Yuan, C. (2017a). Improved ethanol gas sensing performances of a ZnO/Co3O4 composite induced by its flytrap-like structure. Phys. Chem. Chem. Phys. 19, 29601–29607. doi: 10.1039/C7CP05228F
Xu, K., Yang, L., Zou, J., Yang, Y., Li, Q., Qu, Y., et al. (2017b). Fabrication of novel flower-like Co3O4 structures assembled by single-crystalline porous nanosheets for enhanced xylene sensing properties. J. Alloy. Compd. 706, 116–125. doi: 10.1016/j.jallcom.2017.02.217
Xu, K., Zou, J., Tian, S., Yang, Y., Zeng, F., Yu, T., et al. (2017c). Single-crystalline porous nanosheets assembled hierarchical Co3O4 microspheres for enhanced gas-sensing properties to trace xylene. Sens. Actuators B Chem.246, 68–77. doi: 10.1016/j.snb.2017.02.071
Yang, J., Gao, C., Yang, H., Wang, X., and Jia, J. (2017). High selectivity of a CuO modified hollow SnO2 nanofiber gas sensor to H2S at low temperature. Eur. Phys. J. Appl. Phys. 79:30101. doi: 10.1051/epjap/2017170123
Yoon, J., Yang, H. S., Lee, B. S., and Yu, W. R. (2018). Recent progress in coaxial electrospinning: new parameters, various structures, and wide applications. Adv. Mater. 30:e1704765. doi: 10.1002/adma.201704765
Zhang, L., and Hsieh, Y.-L. (2009). Carbon nanofibers with nanoporosity and hollow channels from binary polyacrylonitrile systems. Eur. Polym. J. 45, 47–56. doi: 10.1016/j.eurpolymj.2008.09.035
Zhang, M., Huang, X., Xin, H., Li, D., Zhao, Y., Shi, L., et al. (2019). Coaxial electrospinning synthesis hollow Mo2C@C core-shell nanofibers for high-performance and long-term lithium-ion batteries. Appl. Surf. Sci. 473, 352–358. doi: 10.1016/j.apsusc.2018.12.098
Zhang, X., Chen, D., Cui, H., Dong, X., Xiao, S., and Tang, J. (2017). Understanding of SF6 decompositions adsorbed on cobalt-doped SWCNT: a DFT study. Appl. Surf. Sci. 420, 371–382. doi: 10.1016/j.apsusc.2017.05.163
Zhang, X., Gui, Y., and Dong, X. (2016). Preparation and application of TiO2 nanotube array gas sensor for SF6-insulated equipment detection: a review. Nanoscale Res. Lett. 11:302. doi: 10.1186/s11671-016-1516-4
Zhang, X., Li, Y., Chen, D., Xiao, S., Tian, S., Tang, J., et al. (2018a). Dissociative adsorption of environment-friendly insulating medium C3F7CN on Cu (111) and Al (111) surface: a theoretical evaluation. Appl. Surf. Sci. 434, 549–560. doi: 10.1016/j.apsusc.2017.10.201
Zhang, X., Li, Y., Tian, S., Xiao, S., Chen, D., Tang, J., et al. (2018b). Decomposition mechanism of the C5-PFK/CO2 gas mixture as an alternative gas for SF6. Chem. Eng. J. 336, 38–46. doi: 10.1016/j.cej.2017.11.051
Zhang, Z., Li, X., Wang, C., Wei, L., Liu, Y., and Shao, C. (2009). ZnO Hollow nanofibers: fabrication from facile single capillary electrospinning and applications in gas sensors. J. Phys. Chem. C 113, 19397–19403. doi: 10.1021/jp9070373
Zhou, Q., Chen, W., Li, J., Peng, S., Lu, Z., Yang, Z., et al. (2018a). Highly sensitive hydrogen sulfide sensor based on titanium dioxide nanomaterials. J. Nanoelectron. Optoe. 13, 1784–1788. doi: 10.1166/jno.2018.2417
Zhou, Q., Umar, A., Sodki, E. M., Amine, A., Xu, L., Gui, Y., et al. (2018b). Fabrication and characterization of highly sensitive and selective sensors based on porous NiO nanodisks. Sens. Actuators B Chem. 259, 604–615. doi: 10.1016/j.snb.2017.12.050
Keywords: hollow nanofibers, sensing application, sensing mechanism, SF6 decomposing products, gas insulated switchgear
Citation: Lu Z, Zhou Q, Wei Z, Xu L, Peng S and Zeng W (2019) Synthesis of Hollow Nanofibers and Application on Detecting SF6 Decomposing Products. Front. Mater. 6:183. doi: 10.3389/fmats.2019.00183
Received: 04 June 2019; Accepted: 12 July 2019;
Published: 26 July 2019.
Edited by:
Zhenyu Li, Southwest Petroleum University, ChinaCopyright © 2019 Lu, Zhou, Wei, Xu, Peng and Zeng. This is an open-access article distributed under the terms of the Creative Commons Attribution License (CC BY). The use, distribution or reproduction in other forums is permitted, provided the original author(s) and the copyright owner(s) are credited and that the original publication in this journal is cited, in accordance with accepted academic practice. No use, distribution or reproduction is permitted which does not comply with these terms.
*Correspondence: Qu Zhou, zhouqu@swu.edu.cn; Wen Zeng, wenzeng@cqu.edu.cn