- Magnel Laboratory for Concrete Research, Department of Structural Engineering, Faculty of Engineering and Architecture, Ghent University, Ghent, Belgium
To obtain a more sustainable concrete, occurring cracks should be repaired. However, as manual repair is time-consuming and expensive, self-healing may provide a proper solution. Autogenous healing is an already a present feature in cementitious materials, but it is an inferior mechanism as it can only completely heal cracks up to 30 μm in the presence of water. Therefore, a cementitious material with synthetic microfibers to limit the crack widths and superabsorbent polymers (SAPs) to provide the necessary water was proposed. However, due to ongoing hydration, autogenous healing based on further hydration of unhydrated cement may be less efficient in time. In this study, the ability of autogenous healing in time (on specimens with an age of 7 days, 28 days, 3 months, 1 year, 3 years, and 8 years) is investigated by comparing the mechanical characteristics after performing four-point-bending tests. The specimens were first loaded to 1% strain at their respective age, stored in a specific healing condition (a relative humidity of 60%, more than 90% and wet/dry cycles) for 28 days and were subsequently reloaded. The results show that, with increasing age, the mean crack width decreases. All specimens can partially heal and regain some of the mechanical properties after being preloaded and re-cracked under four-point-bending. If SAPs are added, there is partial healing in an environment without liquid water (more than 90% RH). At an early age, the healing is governed by further hydration and calcium carbonate crystallization. From 3 months onwards, the main autogenous healing mechanism is calcium carbonate crystallization. Overall, mixtures with SAPs generally showed more self-healing and are thus a promising material to be used in future building applications.
Introduction
Concrete is one of the most-used construction materials in the world and has left an undeniable mark on society. Dating back to the classical and Roman era, concrete was used to construct domes—such as on the Pantheon –, aqueducts and bridges, amongst others. Almost 200 years ago, the modern cementitious material was re-designed by Joseph Aspdin. Now, structures such as the Hoover Dam and the Burj Khalifa are shaping the view of the world. The gray material is all around us, but it has one major downside. Even at low tensile stress, (micro-)cracks can easily originate resulting in ingress of potentially harmful substances which deteriorate reinforced concrete from the inside. Costly maintenance is required which is not always successful, therefore, opportunities are studied to tackle this problem. A possible durable and sustainable solution is the use of a concrete with self-healing capacity mimicking the human body.
Concrete possesses a passive healing of its own, called autogenous healing (Snoeck and De Belie, 2015a). Other healing mechanisms include the use of protected bacteria (Wang et al., 2014, 2015), encapsulated polyurethanes (Van Tittelboom et al., 2011, 2012b), microcapsules containing healing agents (Kanellopoulos et al., 2016; Van Tittelboom et al., 2016), other minerals (Sisomphon et al., 2012; Roig-Flores et al., 2015), and a porous vascular system to introduce healing agents (Sangadji and Schlangen, 2012), amongst others (Van Tittelboom and De Belie, 2013; Snoeck et al., 2018b). Generally, autogenous healing is considered to be the combination of further hydration of unhydrated cement and pozzolanic materials, the precipitation of calcium carbonate, the expansion of calcium-silicate-hydrate phases and the closing of cracks to the blockage by loose particles and impurities (Edvardsen, 1999; ter Heide, 2005; Granger et al., 2007; Homma et al., 2009).
The autogenous healing can be improved by considering three influencing factors. These are the presence of sufficient building blocks (Ca2+, CO2, unhydrated cement, pozzolanic materials, etc.) to sustain healing, the restriction of occurring cracks to healable widths and the provision of water to trigger the healing mechanisms. Cracks in reinforced cementitious materials need to be narrow enough to be completely healable. If not, the constituents available in the cementitious mix are consumed before the crack is effectively closed. A typical boundary is 30–50 μm when water is present. From 150 μm onwards, almost no closing of the crack is expected (Yang, 2008; Yang et al., 2009; Snoeck et al., 2014b; Snoeck, 2015; Snoeck and De Belie, 2015b). A thorough material design can improve the autogenous healing capacity by influencing the three above-mentioned features. By changing the mixture design, adding synthetic microfibers and superabsorbent polymers, all criteria are met to have a proper self-healing cementitious material. This cementitious material containing microfibers and superabsorbent polymers is investigated in this study.
The inclusion of poly(vinyl alcohol) (PVA) microfibers to obtain a fiber-reinforced material with a high tensile ductility leads to a strain-hardening behavior achieved by matrix multiple cracking (Li et al., 1988; Li, 2008). Due to the use of microfibers, the crack widths are within the healable range. This healing can occur in tensile cracks (Li, 2008), bending cracks (Yang et al., 2009; Snoeck et al., 2014b) and impact cracks (Snoeck et al., 2018a), showing the same behavior. As liquid water needs to be present, the healing is limited and needs to be promoted. The material does not show any form of healing when placed in a humid environment (Yang et al., 2009).
To include water, one way is through the use of superabsorbent polymers (SAPs). These materials are able to absorb a significant amount of fluid from the surrounding environment (up to 1,000 times their own weight) and to retain the fluid without dissolving (Mignon et al., 2017). SAP particles absorb mixing water during the mixing process and shrink to their dry size during hardening of the cementitious material, forming air-filled macro pores (Yao et al., 2011; Snoeck et al., 2014a). These macro pores act as crack initiators and thus promote multiple cracking (Yao et al., 2011; Snoeck et al., 2014a). Furthermore, SAP particles absorb water during wet periods and slowly release this water during dry periods to further improve the autogenous healing (Snoeck, 2015, 2018). Upon crack formation and water ingress, SAP particles along the crack faces will swell and cause a physical blockage of the crack (Snoeck et al., 2012). In this way SAPs will initially seal the crack from intruding fluids, increasing the durability (Lee et al., 2010, 2016; Snoeck et al., 2012, 2018d). Other applications of SAPs include mitigation of autogenous shrinkage (Jensen and Hansen, 2001, 2002; Snoeck et al., 2015a, 2017) and plastic shrinkage (Snoeck et al., 2018c), increased freeze-thaw resistance (Mechtcherine et al., 2017), and change in rheology (Mechtcherine et al., 2015), amongst others (Mechtcherine and Reinhardt, 2012).
The aim of this paper is to report on how SAPs promote autogenous healing in microfiber-reinforced cementitious materials when performing wet-dry cycles, and in air with a certain relative humidity. This will be monitored as a function of time up to a total age of 8 years. As the building blocks in cementitious materials are consumed over time due to ongoing hydration, the material may behave differently upon loading and healing at different ages. In literature, a recent study showed that autogenous healing is still present in 1-year old specimens (Yıldırım et al., 2018). However, in this research, specimens with an age up to 8 years are studied.
Materials and Methods
Materials
The studied cementitious mixtures are composed of ordinary Portland cement (C) (CEM I 52.5 N, Holcim, Belgium), fly ash (FA) (Class F, OBBC, Belgium), fine quartz sand M34 (S) (D50 = 170 μm, Silbelco, Belgium), water (W), and a polycarboxylate superplasticizer (Spl) (Glenium 51, conc. 35%, BASF, Belgium). Multiple cracks needed to be created and an amount of 2 v% (volume percent) of polyvinyl alcohol fibers (PVA, 15 dtex; 8 mm cutting length; 12 cN/dtex tenacity, Kuraray, Japan) is sufficient to show a pronounced amount of multiple cracking and strain-hardening. Additionally, a varying amount of SAP (0/0.5/1 m% mass-percentage of cement weight) was added on top of the reference mixture without SAPs. Two different types of SAP were used. These include SAP A (a copolymer of acrylamide and sodium acrylate with a particle size of 100.0 ± 21.5 μm, BASF, Germany) and SAP B (a cross-linked potassium salt polyacrylate having a particle size of 477 ± 53 μm, BASF, Germany). Both SAPs are bulk-polymerized and consist of irregular crushed particles. Additional water was used to compensate for the loss in workability. This additional water is stored in the SAPs during hydration, resulting in a cement paste with the same effective water-to-binder ratio and free water content as the reference without SAPs. The reference mixture had a sand-to-binder (S/B) ratio of 0.35, a superplasticizer content (Spl/B) of 0.0097, a fly-ash-to-cement (FA/C) ratio of 1 and a water-to-binder (W/B) factor of 0.30 (Snoeck, 2015). The compositions (with superabsorbent polymers where applicable) are shown in Table 1. “REF” states a mixture without SAPs, “0.5A/0.5B/1B” a mixture with 0.5 m% SAP A and SAP B and 1 m% of SAP B, respectively.
The mixing procedure started with dry mixing of cement, fly ash and where appropriate SAPs (depending on the mixture composition) with a standard mortar mixer for 30 s until they were equally distributed. The total amount of water and superplasticizer were added to the dry constituents (mixing during 30 s at 140 rpm) and fine silica sand was added (additional mixing during 30 s at 140 rpm and at increased speed during 30 s at 285 rpm). The edges of the bowl were scraped for 30 s and the mixture was able to rest during 60 s. The synthetic microfibers were slowly added (mixing during 30 s at 140 rpm), followed by mixing for 60 s at 285 rpm. The molds (160 × 40 × 10 mm3 samples) were subsequently filled and the specimens were demoulded after 24 h. Afterwards, the specimens were stored at a relative humidity of 95 ± 5% and a temperature of 20 ± 2°C until an age of 7 days, 28 days, 3 months, 1 year, 3 years, and 8 years. Series used within this study consisted of minimally three samples per tested age.
Four-Point-Bending Test
A four-point-bending test (Walter+Bai DB 250/15) was used to study the multiple-cracking behavior of the fiber-reinforced composites at the respective age. The first-cracking-strength was measured as the first kink in the stress-strain curve prior to strain-hardening. The ductility was measured as the strain at the lower side of the sample starting at the first-cracking-strength until the point the material showed the highest stress due to strain hardening. Strain-softening was thus not considered as this would imply a previously formed crack to widen. The cracking occurred under standard laboratory conditions at a temperature of 20 ± 2°C. The displacement was increased until 1% strain at the bottom of the specimen. This strain was calculated from the vertical displacement (0.0015 mm/s to imitate a quasi-static load) during four-point-loading using trigonometry (Snoeck, 2015).
After cracking, the samples were placed for 28 days under three conditions:
i) at a relative humidity of 60 ± 5% and 20 ± 2°C in a climate-controlled room.
ii) at a relative humidity of more than 90% (95 ± 5%) and 20 ± 2°C in a climate-controlled room, and;
iii) wet/dry cycles in which samples are stored alternately for 1 h in water and 23 h in the first mentioned curing condition of 60% relative humidity;
The specimens were reloaded in four-point-bending after the healing period until complete failure and the mechanical properties (first-cracking-strength) obtained during the first (loading prior to healing) and the second (loading after healing) cycle were compared. The self-healing capacity was calculated as the regain of the first-cracking-strength. Before and after healing, the crack widths were microscopically measured and the closing percentage was calculated.
Additional reference samples and SAP samples containing 1 m% SAP B were cracked until failure at an age of 28 days and healed for a subsequent 28 days in the above-mentioned healing conditions. In this way, different crack widths could be studied as strain softening already occurred, opening previously formed cracks. This leads to complete curves to determine the average observed closure as the range of studied crack widths is expanded.
Results and Discussion
Study on the Autogenous Healing of Reference Cementitious Composites
All results of the measured crack widths, maximum crack widths, number of cracks and ductility can be found in Table 2. The crack widths were measured on specimens loaded until 1% strain, not reaching the maximal ductility. The ductility itself was determined on specimens loaded until failure. With increasing age, the crack width decreases in all mixtures. This is due to the stronger cementitious matrix with time due to hardening and thus the higher fiber-pull-out force needed, leading to the smaller pull-out length of the separate fibers and thus lower crack width. This was also found in literature (Yang et al., 2011; Kan and Shi, 2012). Generally, the crack widths are lower than in literature [16 cracks with average crack width of 39 μm and maximum of 60 μm at an age of 3 days (Yang et al., 2011) and average crack widths of 29–35, 24–31, and 15–17 μm after 3 days, 28 days, and 3 months, respectively (Yang et al., 2007; Kan and Shi, 2012)] due to a different mixture composition, the use of different raw materials and possible differences in age and curing conditions. The same conclusions can be drawn about the largest crack width.
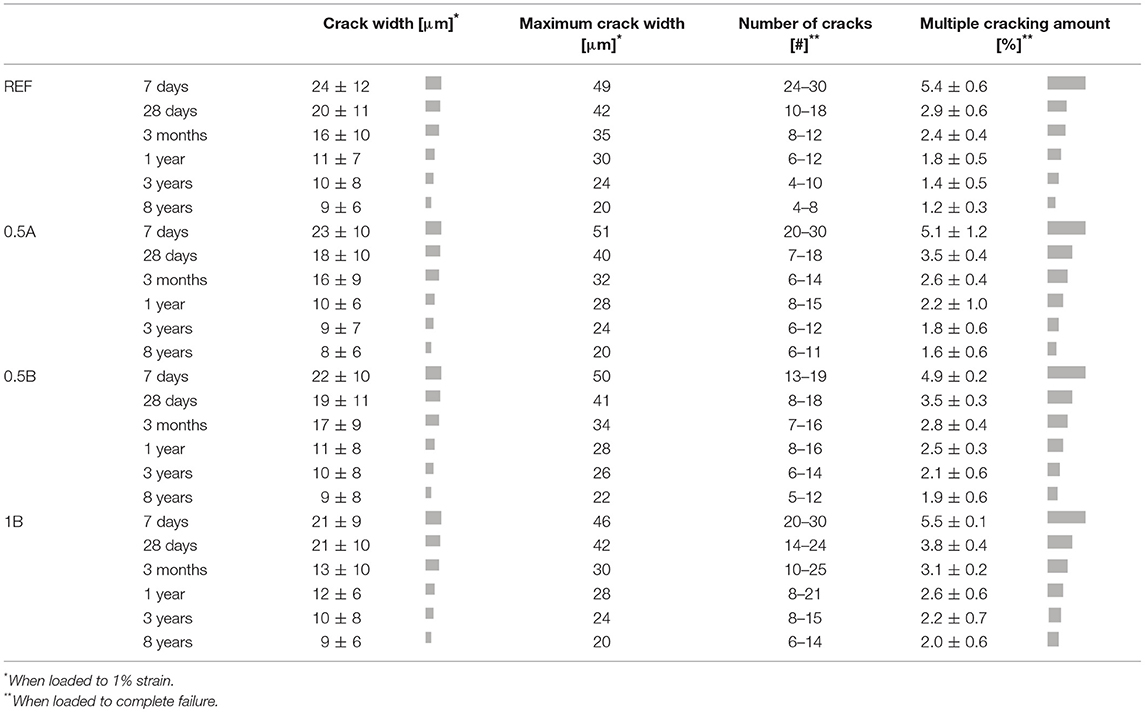
Table 2. Mean values and standard deviations for the crack width [μm] when loaded till 1% strain, the maximal crack width [μm] when loaded till 1% strain, the number of cracks [#] when loaded to complete failure, and the multiple cracking amount as measure for the ductility [%] when loaded to complete failure with their respective standard deviations on single results (n = 3).
The number of cracks and the strain in the cracked zone are closely related when specimens are loaded to complete failure. The highest ductility is found when cracking the specimens at an age of 7 days and decreases with increasing age. This is due to the hardening of the cementitious matrix in time, reducing the ductile capacity. This is mainly due to the stronger matrix, influencing the crack-tip and strength criterion to obtain strain-hardening cementitious materials (Li et al., 2002). Thus, due to the stronger bond of the microfibers with the matrix in time and the stronger cementitious matrix, the overall ductility decreases. Even though the ductility decreases, the crack widths decrease, leading to a better opportunity to show healing. This is also due to the stronger bond of the microfibers with the matrix. But, as hydration continues, the available building blocks may be less present.
In specimens loaded until total failure, sometimes more than 15–20 cracks were formed, showing the high ductility. This is an inherent feature of the pronounced strain-hardening found when using synthetic microfibers. All cracks were through-going, which is typical for steady-state cracks formed when performing four-point-bending tests on mixtures with incorporated synthetic microfibers (Li, 2008). The crack width as determined by microscopic analysis did not vary significantly over the total crack length and all microscopic properties were similar. Most important is the range of crack widths (mean value) and the largest fracture. The latter is important, as the width is mostly the limiting factor for the regain in mechanical properties. If there is one large crack compared to the others, this larger one will preferentially open even if the other cracks are perfectly sealed and healed by healing products.
The influence of the age on the strength criterion to obtain a strain-hardening cementitious material is also reflected in the value of the first-cracking-strength, as shown in Table 3. The strength increases in time during hardening, which is logical. All strength values are within the same range irrespective of the SAP addition and are typical values for strain-hardening cementitious materials (Yang et al., 2007, 2009; Li, 2008; Yang, 2008). The regain in first-cracking-strength in the three different storage conditions is also shown in Table 3. The three storage conditions are storage in wet/dry cycles (1 h in water and 23 h in standard laboratory conditions), at a relative humidity of more than 90% and at a relative humidity of 60%. The temperature was always 20 ± 2°C and the healing period was 28 days.
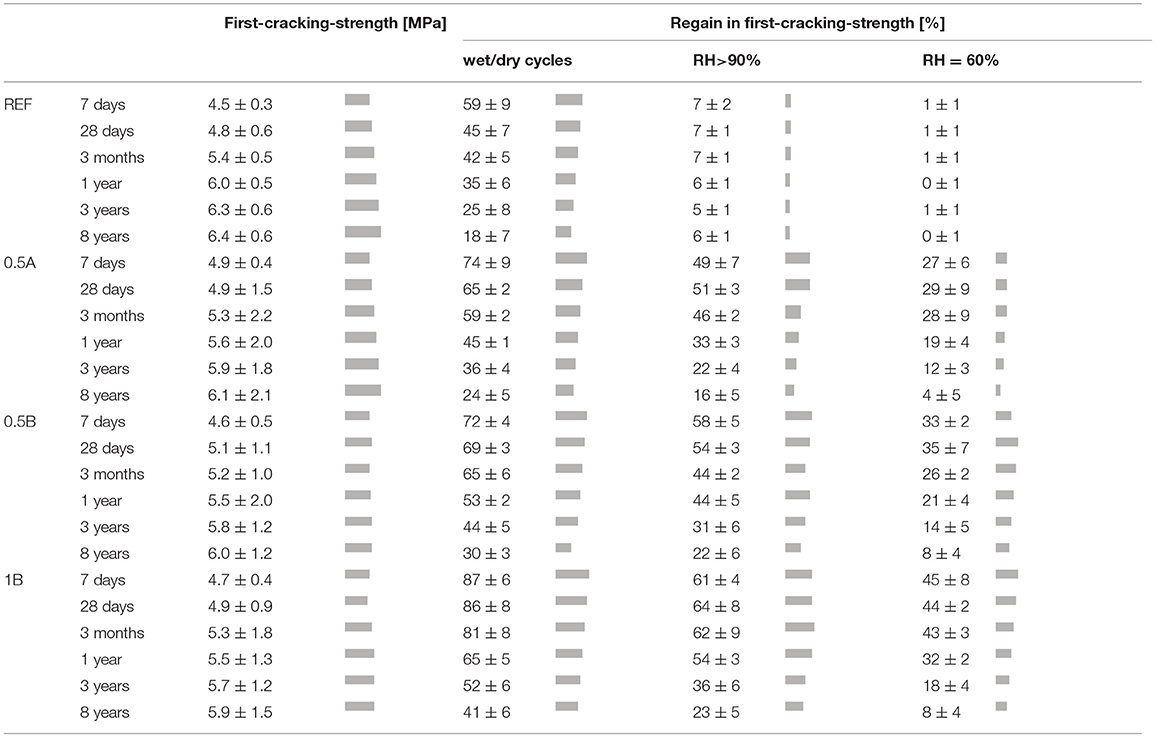
Table 3. Mean values and standard deviations for the first-cracking-strength [MPa], and the regain in first-cracking-strength [%] with their respective standard deviations on single results (n = 3).
To study the regain in mechanical properties, specimens were used with a strain at the bottom limited to 1%. The crack width was hereby always limited to 30 μm in all specimens, which is ideal for autogenous healing. Not only are cracks able to visually close, the composite is able to regain some of its mechanical properties. The regain in mechanical properties—in this case the first-cracking-strength—when storing specimens in wet/dry cycles is dependent on the age of the specimen. The older the specimen, the lower the regain in mechanical properties. This is due to the further hydration and stiffening of the cementitious matrix in time. This leads to a lower amount of available building blocks for the autogenous-healing mechanisms, even though a low water-to-binder ratio is used. The pozzolanic reaction by the fly ash [and even a latent-hydraulic reaction of blast-furnace slag as shown in previous research (Snoeck, 2015)] is promising for the autogenous healing. Fly ash continues to hydrate after 28 days, and the hydrated products may heal the cracks, increasing the self-healing ability. This was also found by (Jacobsen et al., 1995; Termkhajornkit et al., 2009; Zhou et al., 2011). In the long term, Ca(OH)2 may not exist anymore due to the well-progressed pozzolanic reaction and carbonation, explaining the lower regain in mechanical properties at later ages as the pozzolanic reaction slows down.
At an early age, there is further hydration combined with calcium-carbonate crystallization and from 3 months onwards, the main healing mechanism is the precipitation of CaCO3. This was visually monitored as the white CaCO3 could be distinguished. The results are comparable to literature (Van Tittelboom et al., 2012a). A remark can be made. The matrix is composed out of C-S-H, CH (amongst others) and alkali oxides are found in the pore solution. If more C-S-H from the fly-ash reaction is formed, the pH value is expected to decrease, especially when high amounts of fly ash are available in the composite and Ca(OH)2 is consumed. The formation of CaCO3 could hereby be limited (due to the reduced pH level and availability of Ca(OH)2). This was not the case in the studied reference mixture in this research. The pH value of the intruding fluid inside a crack is dependent on the fluid composition itself rather than the mixture composition. In time, the leaching of products from the cementitious matrix may change the pH-value of the fluid in the crack. Some examples of the (partial) healing through further hydration, calcium carbonate crystallization and their combined effect are shown in Figure 1. Even though the substantial loading was observed, the multiple small cracks were able to close completely. Instead of the need to close a wide crack—which is impossible—the smaller cracks were all closed, showing the potential of the material. Overall, CaCO3 crystallization was found as the main healing mechanism, as confirmed by means of thermogravimetric analysis (Snoeck et al., 2014b; Snoeck and De Belie, 2015b). In the interior of the crack, possible further hydration would lead to the increase in mechanical properties. Furthermore, in the interior of the crack, less healing products are present (Snoeck et al., 2016) and the material is able to regain the mechanical properties to some extent for subsequent healing periods after being re-damaged (Snoeck and De Belie, 2015b).
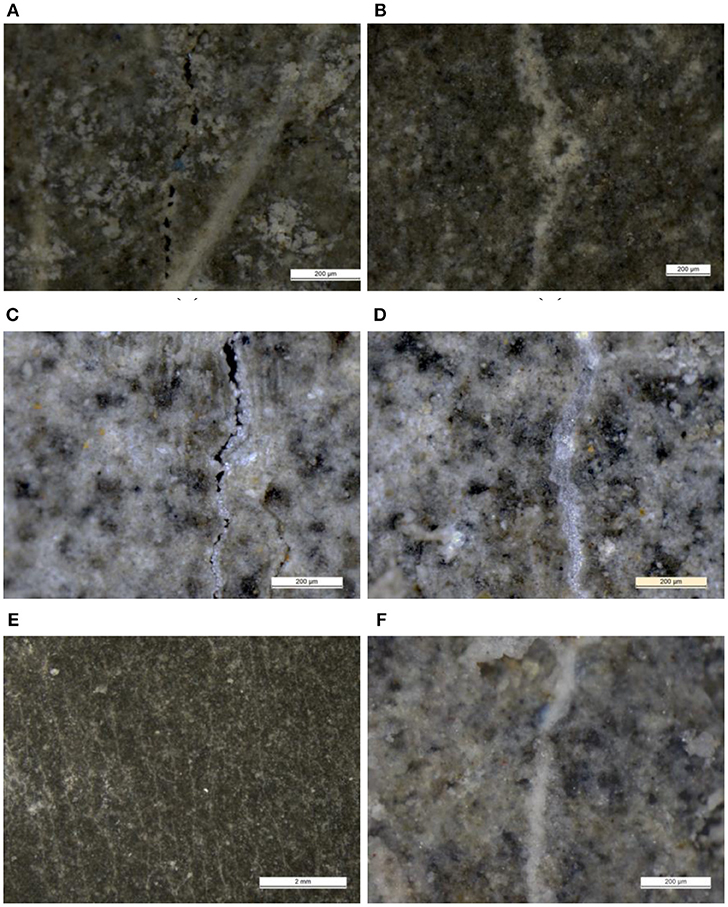
Figure 1. Autogenous healing mechanisms showing partial (A) and further hydration (B) partial (C) and full calcium carbonate crystallization (D) and the combination of both in a number of cracks (E) and close-up (F). The scale bars amount to 200 μm (A–D,F) and 2 mm (E).
Inclusion of SAPs to Further Promote Autogenous Healing
Superabsorbent polymers were also included, and their effects were studied in great detail. This information is new to literature, especially for ages up to 8 years. Important properties are the strength and the regain in strength of the cementitious materials with and without superabsorbent polymers, which will be tackled at the end of this section. First, the microscopic analysis will be discussed, mainly at the age of 28 days, as more specimens were studied at that age, giving more information about the healing over a broad crack width range in different storage conditions.
All cracks were microscopically studied and recorded. The microscopic visual crack closure of reference specimens without SAPs in wet/dry cycles, at a relative humidity of more than 90% and at a relative humidity of 60%, is shown in Figure 2 for the testing age of 28 days and different times of measuring the crack widths while healing. Figure 3 gives the results for specimens containing superabsorbent polymers (1 m% of SAP B) stored in the same healing conditions. In the left part of the figures, the crack widths of the respective cracks after a certain healing period are compared to the width of the initially unhealed crack. If the crack visually closed, the data point shifts downwards compared to the bisector. The right-hand side of the figures shows the percentage of closure of the investigated crack.
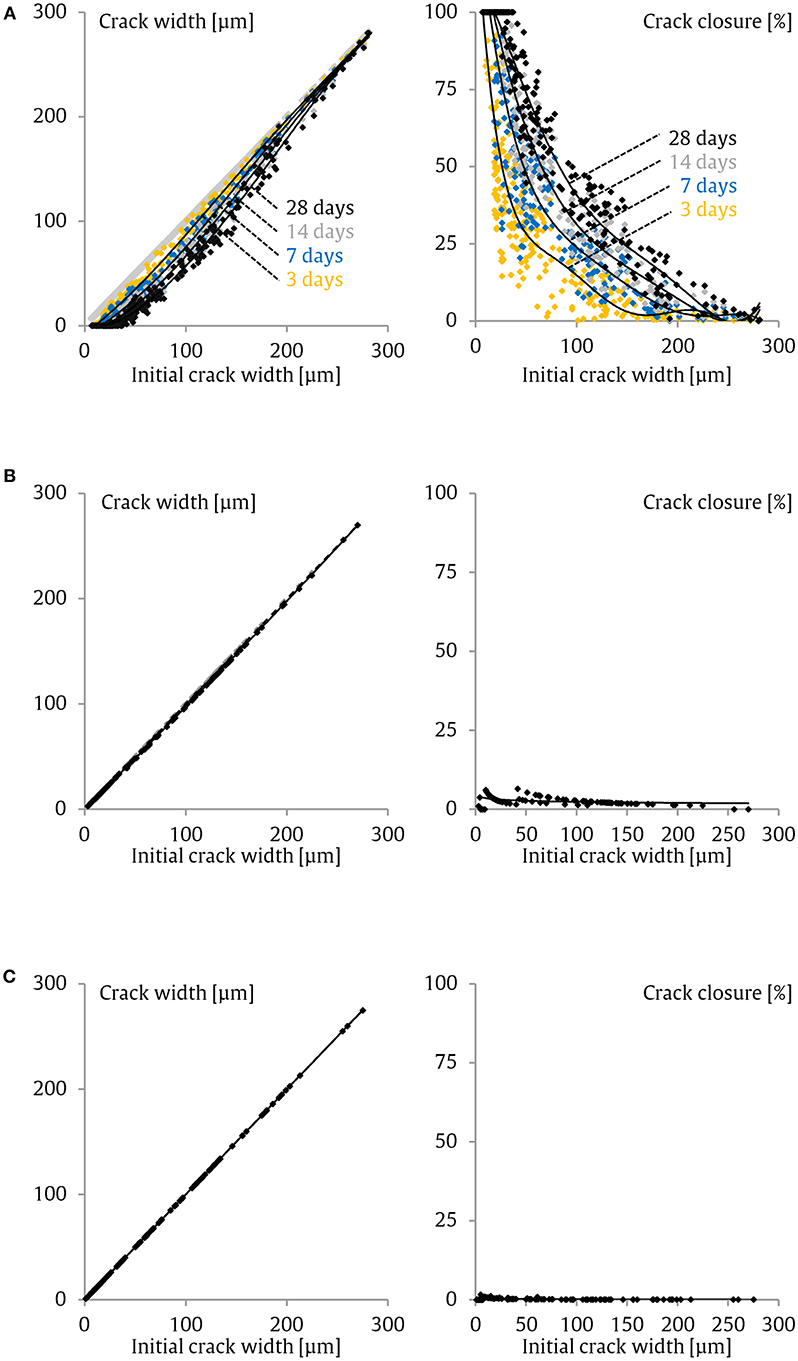
Figure 2. Specimens without SAPs healed in wet/dry cycles (after 3, 7, 14, and 28 cycles) (A), at a relative humidity of more than 90% (28 days) (B), and at 60% (28 days) (C), showing the crack closure as a function of the initial crack width.
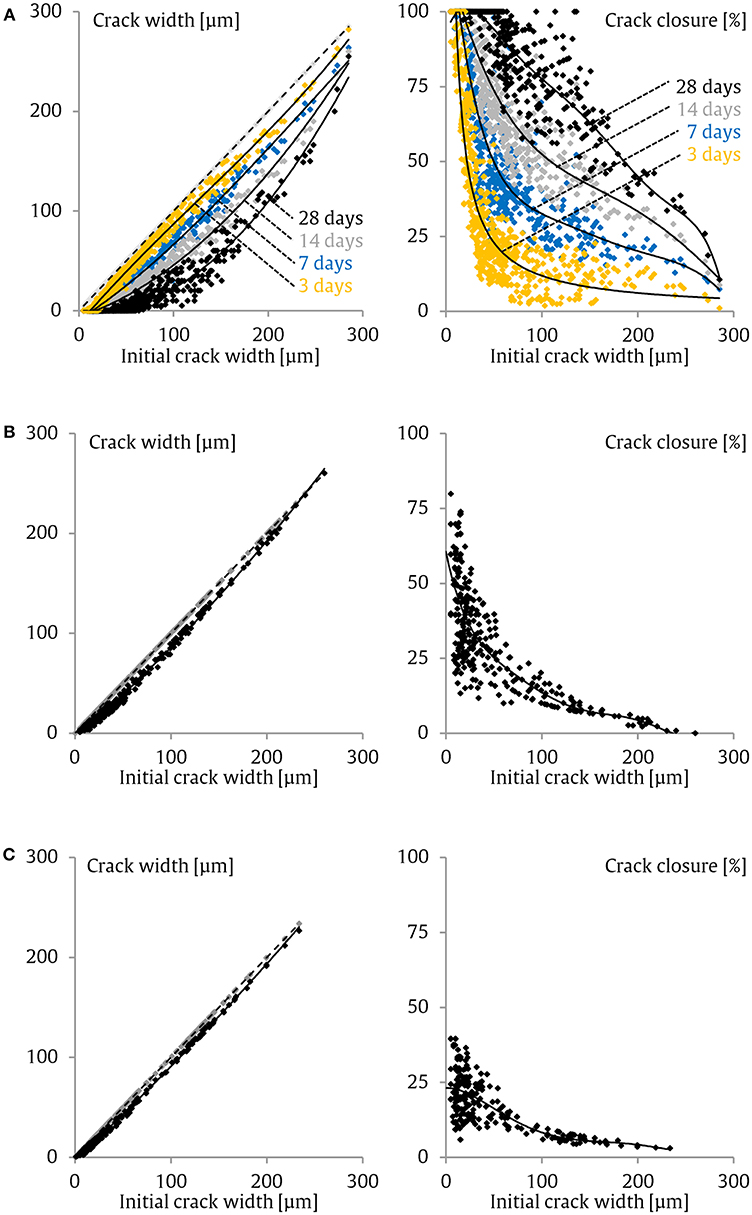
Figure 3. Specimens with 1 m% SAP B healed in wet/dry cycles (after 3, 7, 14, and 28 cycles) (A), at a relative humidity of more than 90% (28 days) (B), and at 60% (28 days) (C), showing the crack closure as a function of the initial crack width.
Cracks in specimens without SAPs heal completely up to 30 μm and heal partly up to 150 μm when subjected to 28 wet/dry cycles. The cracks steadily close in time. Also Li et al. proved this, but their limit was 50 μm instead of 30 μm (Li, 2008; Yang, 2008; şahmaran et al., 2013). They also showed that, in the presence of air (without any water available), samples cannot heal. Figure 2 shows that at a relative humidity of more than 90%, there is only partial and minor healing. At 60% RH, the formation of healing products in a crack is non-existing.
In specimens with SAPs, however, some healing occurred at 60% and at more than 90% relative humidity as crack closure was noticed visually. This means that SAPs are capable of providing liquid water toward the cementitious matrix for improved autogenous healing. SAPs are thus able to fulfill the criterion for exposure to environmental conditions and provide water as they are to adsorb four times their weight in moisture from the environment (Snoeck et al., 2015b). This adsorbed water can be given to the cementitious matrix and causes autogenous healing to occur in the form of further hydration of unhydrated cement grains (Snoeck et al., 2016). Storing pure cement in humid conditions of more than 90% RH and at a temperature of 20 ± 2°C for 1 month only partially ages the cement (Dubina and Plank, 2013). The cement paste was placed in an open cup and had a thickness of ~1 mm. When SAPs are added as well, some hard-hydrated parts in the vicinity of the SAPs are found and thus visually more hydration occurred. This led to the conclusion that SAPs are able to provide water toward the cementitious matrix due to possible small changes in relative humidity.
Cracks smaller than 30 μm healed completely both with and without SAPs after being exposed to wet/dry cycles. Cracks ranging from 50 to 150 μm only healed partly in samples without SAP but sometimes showed complete closure in specimens containing SAPs after performing 28 wet/dry cycles (Figure 3). With superabsorbent polymers, there is a higher amount of formed healing products in a crack. So, not only narrow cracks up to 30 to 50 μm are able to heal, but also cracks up to 130 μm and larger. Cracks larger than 200 μm showed a reduced visual closure. The microfibers act as a nucleation site for CaCO3 crystals and thus promote autogenous healing as well, as also seen by (Homma et al., 2009).
The crack width, maximum crack width, the number of cracks, and the amount of multiple cracking as a measure of the ductility of the specimens used to study the effect of the age are shown in Table 2. The mean crack widths are not statistically significantly different between the different mixtures and they decrease with age. This is due to the hardening of the cementitious matrix as explained before. Superabsorbent polymers do not have an influence on the properties of the cementitious matrix when additional water is used; they only facilitate multiple cracking and the cracks formed are alike. The microstructure is similar as well (Snoeck et al., 2014a, 2015b). This is a positive influence to obtain a high regain in mechanical properties and this is even further improved by using SAPs. Air voids—and thus also SAP macro pores—act as crack initiators, so the crack path likely follows the position of macro pores. The SAPs are thus available for swelling upon crack formation as the crack runs through the formed macro pore. There is no significant difference at an age of 7 days, but from 28 days onwards, the amount of multiple cracking is increased in specimens containing SAPs. This is reflected at an age of 8 years where the reference samples show 4–8 cracks and a ductility of 1.2 ± 0.3% while SAP specimens show 5–14 cracks and a ductility of 1.6–2.0%. This is mainly due to the observed facilitation due to macro pore formation. The crack widths have, as mentioned, the same order of magnitude around 10 μm.
With increasing age, the building blocks will become exhausted. This will also lead to a lower amount of autogenous healing as the healing relies on the amount of unhydrated cement particles, Ca(OH)2, unhydrated parts of the pozzolanic fly ash and the amount of leachable Ca2+ ions present in the matrix. As already found previously, the healing mechanism changes in time due to the changing availability of building blocks. At early age, a greater degree of further hydration will occur but later on, the calcium-carbonate crystallization will govern the overall healing. At an age of 7 days and 28 days of healing, the self-healing mechanism is mostly the stitching of the crack by further hydration and calcium carbonate around SAPs. In samples with an age of 28 days, there is a little bit more whitish CaCO3 in the crack. With a sample age of 3 months onwards, there is mainly the white CaCO3 on the crack faces, also for the samples which are 8 years old.
The first-cracking-strength of the different test series cracked at 7 days and stored at different healing conditions for 28 days can be compared. No significant differences exist between the stress values measured for samples with and without SAPs. SAPs have a dual effect on the strength (Hasholt et al., 2012; Snoeck et al., 2014a). On one hand, they will increase the strength due to the further hydration by the release of mixing water in time during hardening of the cementitious matrix (i.e., due to internal curing). However, on the other hand, they will decrease the strength due to the formation of macro pores. Both influences combined leads in this case to an equivalent first-cracking-strength in these strain-hardening mixtures with a low water-to-binder ratio. Overall, the strength is not significantly different between all studied samples. However, in specimens with an age of 8 years, the difference is slightly larger. The amounts of SAPs were limited to 0.5 m% and 1 m% in the case of SAP A and SAP B, respectively. These amounts did not induce a decrease in mechanical properties. Higher amounts of SAPs would result in a decrease in initial mechanical properties. The used amount of superabsorbent polymers is rather high compared to literature for other applications, as only 0.3–0.6 m% SAP (Jensen and Hansen, 2002) or 0.4 m% SAP (Brüdern and Mechtcherine, 2010) is used to reduce the shrinkage by internal curing (also see Chapter 3). In this research, the amount of SAP (up to 1 m%) was substantially higher, in order to investigate the healing efficiency. The beneficial amount for internal curing is 0.22 m% for SAP A and 0.45 m% for SAP B (Snoeck et al., 2017).
The regain in mechanical properties is certainly noticed in wet/dry cycles where abundant water is available for the autogenous healing mechanisms of further hydration and calcium-carbonate crystallization. When stored at a high relative humidity (more than 90%), partial visual closure was found in SAP samples. The adsorbed moisture by the SAPs is responsible for this closure and the subsequent observed regain in mechanical properties (Snoeck et al., 2014b). At lower relative humidity (of ~60%) only minor healing is observed in specimens containing SAPs, leading to a regain in first-cracking-strength. Again, this is due to the release of adsorbed water as if the SAPs are acting as an internal curing agent. The reference samples without superabsorbent polymers are expected to show no regain in mechanical properties in a moist environment as no water will be present to induce autogenous healing.
At an age of 28 days, reference samples in wet/dry cycles show a regain of about 45%, which is comparable to values found in literature of 40% (Yang et al., 2009) and 42% (Yang, 2008). SAPs, however, promote self-healing at a relative humidity of more than 90%. The moisture uptake by SAPs (up to four times their own weight in moisture (Snoeck et al., 2015b) seems to be sufficient to promote a certain degree of autogenous healing, especially in the interior of the crack in the form of further hydration. In the relative humidity condition of more than 90%, the material with 1 m% SAP B shows a strength regain of 60%. In those specimens, further hydration occurred, leading to the regain in mechanical properties. At the crack mouth, the crack was still clearly open and only at some distinct places, there was some bridging of a crack by healing products (Snoeck et al., 2016). At reloading, previously created cracks re-opened and no new cracks were formed until all cracks reopened. Newly formed cracks were only seen when the specimens were stored in wet/dry cycles.
A higher number of SAPs, without decreasing the strength, leads to a higher amount of promoted autogenous healing due to an increase in steadily available water in time. Using 1 m% SAP B leads to a regain of 80–90% in wet/dry cycles and sometimes, a new crack is formed instead of the reopening of a healed crack, which shows a total regain of the mechanical properties at that distinct place. In the interior, mostly further hydration occurred and more pronounced CaCO3 crystals mixed together with hydration products were formed at the crack mouth. Most regain in mechanical properties in all curing conditions is obtained by using 1 m% SAP B. An even higher amount of SAP B would be detrimental in terms of the mechanical properties.
At an age of 8 years, the healing in wet/dry cycles is reduced with a factor 3 in reference samples compared to an age of 7 days. This is approximately the same in SAP specimens, but the regain in mechanical properties is still pronounced. This is possibly due to the release during wet/dry cycling of stored water absorbed during dry periods by the SAPs. Reference samples do not show (or a limited 6%) healing in relative humidity conditions. SAP samples, however, still show around 16–23% healing in a relative humidity condition of more than 90%. In a relative humidity condition of 60%, this healing is 4–8%. Overall, the healing and regain in mechanical properties decrease over time.
An issue which may be important is the possible degradation of a SAP in time, especially in the harsh alkaline environment such as pore solution or the cementitious matrix (Vandenhaute et al., 2017). Also, the exposure to UV light may be detrimental for the use of SAPs in time, as their swelling capacity may decrease over time and may completely dissolve or carbonate (Mechtcherine et al., 2018). With the polymers used in this research, no degradation was found. The swelling was still the same after being stored in dry conditions without direct contact of UV light for a period of up to 8 years' time. Even after heating up to 75°C and freezing to −15°C, the polymer was still able to swell to full extent. In 8-year old specimens, the SAPs were still able to swell when they were exposed to water. The polymers are thus stable over time. However, when using another type of SAP which can degrade (Vandenhaute et al., 2017), one should verify the degradation phenomena in time.
Conclusions
Based on the findings on the healing capacity as a function of time for cementitious materials with and without SAPs, the following conclusions can be drawn:
- The highest number of cracks and ductility are found in younger specimens. Smaller average cracks are found in older specimens due to the further hydration and change in bond strength of the microfibers.
- SAPs improve the overall ductility due to their crack initiator capability and the subsequent formation of macro pores. This feature is more significant at later ages. At an age of 8 years, the reference samples show 4–8 cracks and a ductility of 1.2 ± 0.3%, while SAP specimens show 5–14 cracks and a ductility of 1.6–2.0% showing the same order of magnitude of crack widths around 10 μm. This higher ductility can be interesting in construction areas where high strains are expected.
- At an early age (7 days), the main healing mechanism is the stitching of a crack by further hydration. After an age of 28 days more calcium carbonate is seen, and after 3 months of age (and even up to 8 years of age), this calcium carbonate crystallization is the main healing mechanism.
- Superabsorbent polymers are beneficial as they can promote autogenous healing in wet/dry cycling and at high relative humidity (more than 90%) by the release of their adsorbed and absorbed water upon crack formation. They promote further hydration and calcium carbonate crystallization. In SAP specimens compared to reference samples, larger crack widths show complete healing.
- The healing and regain in mechanical properties decrease over time. Being cracked at an age of 8 years, the healing in reference samples is only a third of the healing when specimens are cracked at an age of 7 days. This is the same order of magnitude as for the SAP samples, but the latter specimens still show a pronounced healing, mainly due to the calcium carbonate crystallization.
- SAPs in a cementitious matrix seem to be stable up to a period of 8 years showing almost no degradation. This is important as they may be effective for sealing and healing purposes up to a minimum of 8 years of construction service life, showing an increased healing capacity compared to a reference sample without SAPs.
Data Availability
The datasets generated for this study are available on request to the corresponding author.
Author Contributions
DS was involved in work conception, experimental design, experimental work, data analysis, manuscript writing and revision, and final manuscript approval. NDB was involved in work conception, critical manuscript revision, and final manuscript approval. All authors agree for work accountability.
Conflict of Interest Statement
The authors declare that the research was conducted in the absence of any commercial or financial relationships that could be construed as a potential conflict of interest.
Acknowledgments
As a Postdoctoral Research Fellow of the Research Foundation-Flanders (FWO-Vlaanderen), DS would like to thank the foundation for the financial support (12J3617N). The authors want to thank Dr. A. Assmann and Dr. G. Herth from BASF for providing SAP A and SAP B.
References
Brüdern, A. E., and Mechtcherine, V. (2010). “Multifunctional use of SAP in strain-hardening Cement-based Composites,” in International RILEM Conference on Use of Superabsorbent Polymers and Other New Additives in Concrete. eds O. M. Jensen, M. T. Hasholt, and S. Laustsen (Lyngby: RILEM Publications, S.A.R.L), 11–22.
Dubina, E., and Plank, J. (2013). “Influence of moisture- and CO2-induced ageing of cement on the performance of chemical admixtures used in concrete and mortar,” in 8th International Symposium on Cement & Concrete. ed W. Zhang (Nanjing), 1–8.
Edvardsen, C. (1999). Water permeability and autogenous healing of cracks in concrete. ACI Mater. J. 96, 448–454.
Granger, S., Loukili, A., Pijaudier-Cabot, G., and Chanvillard, G. (2007). Experimental characterization of the self-healing of cracks in an ultra high performance cementitious material: mechanical tests and acoustic emission analysis. Cement Concr. Res. 37, 519–527. doi: 10.1016/j.cemconres.2006.12.005
Hasholt, M. T., Jensen, O. M., Kovler, K., and Zhutovsky, S. (2012). Can superabsorbent polymers mitigate autogenous shrinkage of internally cured concrete without compromising the strength? Constr. Build. Mater. 31, 226–230. doi: 10.1016/j.conbuildmat.2011.12.062
Homma, D., Mihashi, H., and Nishiwaki, T. (2009). Self-healing capability of fibre reinforced cementitious composites. Adv. Concr. Technol. 7, 217–228. doi: 10.3151/jact.7.217
Jacobsen, S., Marchand, J., and Hornain, H. (1995). SEM observations of the microstructure of frost deteriorated and self-healed concrete. Cement Concr. Res. 25, 55–62. doi: 10.1016/0008-8846(95)00174-3
Jensen, O. M., and Hansen, P. F. (2001). Water-entrained cement-based materials I. Principles and theoretical background. Cement Concr. Res. 31, 647–654. doi: 10.1016/S0008-8846(01)00463-X
Jensen, O. M., and Hansen, P. F. (2002). Water-entrained cement-based materials II. Experimental observations. Cement Concr. Res. 32, 973–978. doi: 10.1016/S0008-8846(02)00737-8
Kan, L., and Shi, H. (2012). Investigation of self-healing behavior of Engineered Cementitious Composites (ECC) materials. Constr. Buil. Mater. 29, 348–356. doi: 10.1016/j.conbuildmat.2011.10.051
Kanellopoulos, A., Giannaros, P., and Al-Tabbaa, A. (2016). The effect of varying volume fraction of microcapsules on fresh, mechanical and self-healing properties of mortars. Constr. Build. Mater. 122, 577–593. doi: 10.1016/j.conbuildmat.2016.06.119
Lee, H. X. D., Wong, H. S., and Buenfeld, N. R. (2010). Potential of superabsorbent polymer for self-sealing cracks in concrete. Adv. Appl. Ceramics 109, 296–302. doi: 10.1179/174367609X459559
Lee, H. X. D., Wong, H. S., and Buenfeld, N. R. (2016). Self-sealing of cracks in concrete using superabsorbent polymers. Cement Concr. Res. 79, 194–208. doi: 10.1016/j.cemconres.2015.09.008
Li, V. C. (2008). “Engineered Cementitious Composites (ECC) – Material, Structural, and Durability Performance,” in Concrete Construction Engineering Handbook. ed E. Nawy (Boca Raton, FL: CRC Press), 78.
Li, V. C., Lim, Y. M., and Chan, Y. W. (1988). Feasibility study of a passive smart self-healing cementitious composite. Composites Part B 29, 819–827. doi: 10.1016/S1359-8368(98)00034-1
Li, V. C., Wu, C., Wang, S., Ogawa, A., and Saito, T. (2002). Interface tailoring for strain-hardening Polyvinyl Alcohol - Engineered Cementitious Composites (PVA-ECC). ACI Mater. J. 99, 463–472. doi: 10.14359/12325
Mechtcherine, V., and Reinhardt, H. W. (eds.). (2012). Application of Super Absorbent Polymers (SAP) in Concrete Construction. Dordrecht; Heidelberg; London; New York, NY: Springer. doi: 10.1007/978-94-007-2733-5
Mechtcherine, V., Schröfl, C., Wyrzykowski, M., Gorges, M., Cusson, D., Margeson, J., et al. (2017). Effect of superabsorbent polymers (SAP) on the freeze-thaw resistance of concrete: results of a RILEM interlaboratory test. Mater. Struct. 50, 1–19. doi: 10.1617/s11527-016-0868-7
Mechtcherine, V., Secrieru, E., and Schröfl, C. (2015). Effect of superabsorbent polymers (SAPs) on rheological properties of fresh cement-based mortars – development of yield stress and plastic viscosity over time. Cement Concr. Res. 67, 52–65. doi: 10.1016/j.cemconres.2014.07.003
Mechtcherine, V., Snoeck, D., Schröfl, C., De Belie, N., Klemm, A. J., Ichimiya, K., et al. (2018). Testing superabsorbent polymer (SAP) sorption properties prior to implementation in concrete: results of a RILEM Round-Robin Test. Mater Struct. 51:28. doi: 10.1617/s11527-018-1149-4
Mignon, A., Snoeck, D., Dubruel, P., Van Vlierberghe, S., and De Belie, N. (2017). Crack mitigation in concrete: superabsorbent polymers as key to success? Materials 10, 1–25. doi: 10.3390/ma10030237
Roig-Flores, M., Moscato, S., Serna, P., and Ferrara, L. (2015). Self-healing capability of concrete with crystalline admixtures in different environments. Constr. Build. Mater. 86, 1–11. doi: 10.1016/j.conbuildmat.2015.03.091
şahmaran, M., Yildirim, G., and Erdem, T. K. (2013). Self-healing capability of cementitious composites incorporating different supplementary cementitious materials. Cement Concr. Composites 35, 89–101. doi: 10.1016/j.cemconcomp.2012.08.013
Sangadji, S., and Schlangen, E. (2012). Self healing of concrete structures - novel approach using porous network concrete. J Adv Concr Technol. 10, 185–194. doi: 10.3151/jact.10.185
Sisomphon, K., Çopuroğlu, O., and Koenders, E. A. B. (2012). Self-healing of surface cracks in mortars with expansive additive and crystalline additive. Cement Concr. Composites 34, 566–574. doi: 10.1016/j.cemconcomp.2012.01.005
Snoeck, D. (2015). Self-Healing and Microstructure of Cementitious Materials with Microfibres and Superabsorbent Polymers. Doctor in Civil Engineering: Construction Design, Ghent University.
Snoeck, D. (2018). Superabsorbent polymers to seal and heal cracks in cementitious materials. RILEM Tech. Lett. 3, 32–38. doi: 10.21809/rilemtechlett.2018.64
Snoeck, D., and De Belie, N. (2015a). From straw in bricks to modern use of microfibres in cementitious composites for improved autogenous healing – a review. Constr. Build. Mater. 95, 774–787. doi: 10.1016/j.conbuildmat.2015.07.018
Snoeck, D., and De Belie, N. (2015b). Repeated autogenous healing in strain-hardening cementitious composites by using superabsorbent polymers. J. Mater. Civil Eng. 04015086, 1–11. doi: 10.1061/(ASCE)MT.1943-5533.0001360
Snoeck, D., De Schryver, T., and De Belie, N. (2018a). Enhanced impact energy absorption in self-healing strain-hardening cementitious materials with superabsorbent polymers. Constr. Build. Mater. 191, 13–22. doi: 10.1016/j.conbuildmat.2018.10.015
Snoeck, D., Dewanckele, J., Cnudde, V., and De Belie, N. (2016). X-ray computed microtomography to study autogenous healing of cementitious materials promoted by superabsorbent polymers. Cement Concr Composites 65, 83–93. doi: 10.1016/j.cemconcomp.2015.10.016
Snoeck, D., Jensen, O. M., and De Belie, N. (2015a). The influence of superabsorbent polymers on the autogenous shrinkage properties of cement pastes with supplementary cementitious materials. Cement Concr Res. 74, 59–67. doi: 10.1016/j.cemconres.2015.03.020
Snoeck, D., Malm, F., Cnudde, V., Grosse, C. U., and Van Tittelboom, K. (2018b). Validation of self-healing properties of construction materials through non-destructive and minimal invasive testing. Adv. Mater. Int. 5:1800179. doi: 10.1002/admi.201800179
Snoeck, D., Pel, L., and De Belie, N. (2017). The water kinetics of superabsorbent polymers during cement hydration and internal curing visualized and studied by NMR. Sci. Rep. 7, 1–14. doi: 10.1038/s41598-017-10306-0
Snoeck, D., Pel, L., and De Belie, N. (2018c). Superabsorbent polymers to mitigate plastic drying shrinkage in a cement paste as studied by NMR. Cement Concr. Composites 93, 54–62. doi: 10.1016/j.cemconcomp.2018.06.019
Snoeck, D., Schaubroeck, D., Dubruel, P., and De Belie, N. (2014a). Effect of high amounts of superabsorbent polymers and additional water on the workability, microstructure and strength of mortars with a water-to-cement ratio of 0.50. Constr. Build. Mater. 72, 148–157. doi: 10.1016/j.conbuildmat.2014.09.012
Snoeck, D., Steuperaert, S., Van Tittelboom, K., Dubruel, P., and De Belie, N. (2012). Visualization of water penetration in cementitious materials with superabsorbent polymers by means of neutron radiography. Cement Concr. Res. 42, 1113–1121. doi: 10.1016/j.cemconres.2012.05.005
Snoeck, D., Van den Heede, P., Van Mullem, T., and De Belie, N. (2018d). Water penetration through cracks in self-healing cementitious materials with superabsorbent polymers studied by neutron radiography. Cement Concr. Res. 113, 86–98. doi: 10.1016/j.cemconres.2018.07.002
Snoeck, D., Van Tittelboom, K., Steuperaert, S., Dubruel, P., and De Belie, N. (2014b). Self-healing cementitious materials by the combination of microfibres and superabsorbent polymers. J. Intell. Mater. Syst. Struct. 25, 13–24. doi: 10.1177/1045389X12438623
Snoeck, D., Velasco, L. F., Mignon, A., Van Vlierberghe, S., Dubruel, P., Lodewyckx, P., et al. (2015b). The effects of superabsorbent polymers on the microstructure of cementitious materials studied by means of sorption experiments. Cement Concr. Res. 77, 26–35. doi: 10.1016/j.cemconres.2015.06.013
Termkhajornkit, P., Nawa, T., Yamashiro, Y., and Saito, T. (2009). Self-healing ability of fly ash-cement systems. Cement Concr Composites 31, 195–203. doi: 10.1016/j.cemconcomp.2008.12.009
Van Tittelboom, K., and De Belie, N. (2013). Self-healing in cementitious materials - a review. Materials 6, 2182–2217. doi: 10.3390/ma6062182
Van Tittelboom, K., De Belie, N., Van Loo, D., and Jacobs, P. (2011). Self-healing efficiency of cementitious materials containing tubular capsules filled with healing agent. Cement Concr. Composites 33, 497–505. doi: 10.1016/j.cemconcomp.2011.01.004
Van Tittelboom, K., Gruyaert, E., Rahier, H., and De Belie, N. (2012a). Influence of mix composition on the extent of autogenous crack healing by continued hydration or calcium carbonate formation. Constr. Build. Mater. 37, 349–359. doi: 10.1016/j.conbuildmat.2012.07.026
Van Tittelboom, K., Snoeck, D., Vontobel, P., Wittmann, F. H., and De Belie, N. (2012b). Use of neutron radiography and tomography to visualize the autonomous crack sealing efficiency in cementitious materials. Mater. Struct. 46, 105–121. doi: 10.1617/s11527-012-9887-1
Van Tittelboom, K., Wang, J., Gomes De Araújo, M. A., Snoeck, D., Gruyaert, E., Debbaut, B., et al. (2016). Comparison of different approaches for self-healing concrete in a large-scale lab test. Constr. Build. Mater. 108, 125–137. doi: 10.1016/j.conbuildmat.2015.12.186
Vandenhaute, M., Snoeck, D., Vanderleyden, E., De Belie, N., Van Vlierberghe, S., and Dubruel, P. (2017). Stability of Pluronic® F127 bismethacrylate hydrogels: Reality or utopia? Polymer Degrad. Stabil. 146, 201–211. doi: 10.1016/j.polymdegradstab.2017.10.003
Wang, J., Mignon, A., Snoeck, D., Wiktor, V., Boon, N., and De Belie, N. (2015). Application of modified-alginate encapsulated carbonate producing bacteria in concrete: a promising strategy for crack self-healing. Front. Microbiol. 6:1088. doi: 10.3389/fmicb.2015.01088
Wang, J., Snoeck, D., Van Vlierberghe, S., Verstraete, W., and De Belie, N. (2014). Application of hydrogel encapsulated carbonate precipitating bacteria for approaching a realistic self-healing in concrete. Constr. Build. Mater. 68, 110–119. doi: 10.1016/j.conbuildmat.2014.06.018
Yang, E.-H. (2008). Designing Added Functions in Engineered Cementitious Composites. University of Michigan.
Yang, E.-H., Yang, Y., and Li, V. C. (2007). Use of high volumes of fly ash to improve ECC mechanical properties and material greenness. ACI Mater. J. 104, 303–311. doi: 10.14359/18966
Yang, Y., Lepech, M. D., Yang, E.-H., and Li, V. C. (2009). Autogenous healing of engineered cementitious composites under wet-dry cycles. Cement Concr Res. 39, 382–390. doi: 10.1016/j.cemconres.2009.01.013
Yang, Y., Yang, E.-H., and Li, V. C. (2011). Autogenous healing of engineered cementitious composites at early age. Cement Concr Res. 41, 176–183. doi: 10.1016/j.cemconres.2010.11.002
Yao, Y., Zhu, Y., and Yang, Y. (2011). Incorporation of SAP particles as controlling pre-existing flaws to improve the performance of ECC. Constr. Build. Mater. 28, 139–145. doi: 10.1016/j.conbuildmat.2011.08.032
Yıldırım, G., Khiavi, A. H., Yeşilmen, S., and şahmaran, M. (2018). Self-healing performance of aged cementitious composites. Cement Concr. Composites 87, 172–186. doi: 10.1016/j.cemconcomp.2018.01.004
Keywords: age, self-healing, SHCC, hydrogels, fiber reinforcement
Citation: Snoeck D and De Belie N (2019) Autogenous Healing in Strain-Hardening Cementitious Materials With and Without Superabsorbent Polymers: An 8-Year Study. Front. Mater. 6:48. doi: 10.3389/fmats.2019.00048
Received: 07 November 2018; Accepted: 27 February 2019;
Published: 26 March 2019.
Edited by:
John L. Provis, University of Sheffield, United KingdomReviewed by:
Antonis Kanellopoulos, University of Hertfordshire, United KingdomAaron Richard Sakulich, Worcester Polytechnic Institute, United States
Copyright © 2019 Snoeck and De Belie. This is an open-access article distributed under the terms of the Creative Commons Attribution License (CC BY). The use, distribution or reproduction in other forums is permitted, provided the original author(s) and the copyright owner(s) are credited and that the original publication in this journal is cited, in accordance with accepted academic practice. No use, distribution or reproduction is permitted which does not comply with these terms.
*Correspondence: Didier Snoeck, didier.snoeck@UGent.be
orcid.org/0000-0001-9427-6312
†Nele De Belie orcid.org/0000-0002-0851-6242