- Department of Chemistry, Mahatma Phule Arts, Science and Commerce College, Panvel, India
ZnS thin films have been deposited on a glass substrate by aerosol assisted chemical vapor deposition (AACVD) from the Zinc thiosemicarbazone precursors is reported. This approach exploits the use of Zn(II) thiosemicarbazone complexes as single source precursors. Thiosemicarbazone complexes such as Zn(cinnamtscz)2, Zn(4-Clbenztscz)2, ZnCl2(cinnamtsczH)2, ZnCl2(4-ClbenztsczH)2 and ZnCl2(thioptsczH)2 (Where, cinnamtsczH = cinnamaldehyde, 4-ClbenztsczH = 4-chlorobenzaldehyde, and thioptsczH = thiophene 2-carboxaldehyde thiosemicarbazones) were used as single source precursors for the deposition of thin films. They were obtained by treatment of zinc chloride with various thiosemicarbazone as sulfur-containing ligands. The deposition was carried out in the temperature range between 400 and 500° C on a glass substrate. The X-ray diffraction pattern reveals the hexagonal phase of ZnS thin films. Scanning electron microscopy and atomic force microscopy analysis shows the granular shaped type of surface texture. The energy dispersive X-ray analysis indicates 1:1 stoichiometry of zinc and sulfur. UV-Visible spectroscopy is used to find the optical band gaps of thin films using Tauc's plots.
Introduction
Transition metals with group 16 elements, commonly known as metal chalcogenide, have been the topic of basic and applied research in the last couple of decades. Metal chalcogenides are wide bandgap semiconducting materials and useful in optoelectronics, solar cells, photoluminescence, optical sensor devices, etc. Among all metal chalcogenide, ZnS is an important II-VI direct wide bandgap semiconductor material with bandgap 3.68 eV and has two basic types: cubic phase (sphalerite) and hexagonal phase (wurtzite). ZnS is extensively used in optoelectronics (Xiong et al., 2004; Daniels et al., 2006), lasers (Biswas et al., 2008) ultraviolet light emitting diodes (Luo et al., 2008), as a phosphor host (Wang and Hong, 2000), optical sensors (Biswas et al., 2005), luminescence (Zhu et al., 2003; Goswami and Sen, 2007), because of high refractive index (2.35) it is used in antireflection and coating (Liu et al., 2009) etc. The various methods like chemical vapor deposition (Lee et al., 2005), metal organic chemical vapor deposition (MOCVD) (Seo et al., 2005), chemical spray Pyrolysis (Hernandez-Fenollosa et al., 2008), liquid-liquid interface method (Fan et al., 2007), chemical bath deposition method (Roy et al., 2006) successive atomic layer adsorption and reaction (Pathan and Lokhande, 2004) etc. were reported for growth of ZnS thin films.
The use of independent sources of zinc and sulfur was reported in the literature (Cockayne and Wright, 1984; Hsu, 2000; Zhenyi et al., 2002) for the deposition of ZnS thin films. Cockayne and Wright used dimethyl zinc (CH3)2Zn, diethyl zinc (C2H5)Zn and dimethyl cadmium (CH3)2Cd as a metal source and H2S and H2Se as chalcogen source (Cockayne and Wright, 1984). In such type of multiple source precursors starting materials are toxic (like H2S gas) and contamination was observed in the deposition of the thin films. Later this problem was solved by using single source precursors. The II-VI semiconductor thin films were accomplished using single source precursors such as dithiophosphinates (Takahashi et al., 1980; Byrom et al., 2000) and dithiocarbamate (Lee et al., 2005; Seo et al., 2005). The main advantage of single-source precursors over multiple source precursors is that it contains both elements which we required in desired the product. In addition to this, it is one step synthesis, improved air/moisture stability, stoichiometric control, limited pre reactions and no need to use harmful gases such as H2S.
Thiosemicarbazone complexes are widely studied for their biological application (Casas et al., 2000; Castineiras and West, 2002; Sharma et al., 2006; Bernhardt et al., 2009; Lobana et al., 2009), analytical application (Campbell, 1975) and structural versatility (Padhye and Kauffman, 1985; Singh and Ishii, 1991). Then after more attention has been given on improved biological activity of the metal complexes than ligands (Kurup and Joseph, 2003). But less attention was paid in use of these compounds in the synthesis of nanomaterial and thin films. These single-source precursors are air stable and contain direct metal to chalcogen bond. In our previous study thiosemicarbazone complexes were successfully used in the preparation of ZnS nanocrystallites using Zn(II)thiosemicarbazones complexes by pyrolysis (Palve and Garje, 2011a) and solvothermal method (Palve and Garje, 2011b). These single-source precursors gave very optimistic results in the deposition of semiconductor thin films.
Here in we report the use of thiosemicarbazone complexes as single source precursors for deposition of ZnS thin films via aerosol assisted chemical vapor deposition technique. Zinc(II) thiosemicarbazone complexes of the type Zn(L)2 and ZnCl2(LH)2 (Where, LH = thiosemicarbazone ligands of cinnamtsczH = cinnamaldehyde, 4-ClbenztsczH = 4-chloro benzaldehyde and thioptsczH = thiophene 2-carboxaldehyde) were used for the deposition of the ZnS thin films. The deposition of the thin films was carried out using aerosol-assisted chemical vapor deposition (AACVD) on a glass substrate. The methanol was used as solvent and deposition was carried out in temperature arrange 400 to 500° C.
Experimental
Synthesis of Single Source Precursors (SSPs)
Zinc(II) thiosemicarbazone complexes of the type Zn(L)2 and ZnCl2(LH)2 (Where, LH = thiosemicarbazone ligands of cinnamtsczH = cinnamaldehyde, 4-ClbenztsczH = 4-chlorobenzaldehyde and thioptsczH = thiophene 2-carboxaldehyde) were prepared according to our previously reported procedures (Palve and Garje, 2011b). The representative synthesis of the single source precursors has been given below.
Preparation of Zn(cinnamtscz)2
0.0971 g (2.02 mmol) of sodium hydride in 20 cm3 of isopropanol was taken in a two necked round bottom flask and the mixture was stirred for half an hour to get a clear solution of sodium isopropoxide. In another flask 0.8311 g (2.02 mmol) of cinnamaldehydethiosemicrbazone was dissolved in THF. This ligand solution was added to the above sodium isopropoxide solution with continuous stirring. The reaction mixture was refluxed for 2 h and then cooled. To this sodium salt of ligand, 0.2760 g (2.02 mmol) of ZnCl2 dissolved in THF was added and the reaction mixture was refluxed for 24 h with constant stirring. The byproduct obtained was filtered off through G-4 filtration assembly. From the filtrate, the solvent was removed under vacuum to get a yellow product. It was repeatedly washed with cyclohexane (5 × 5 cm3) to remove any impurities present and then dried under vacuum (yield, 0.7201 g, 75.15%) MP: 215°C.
Preparation of ZnCl2(cinnamtsczH)2
To a round bottom flask containing 0.7520 g (1.83 mmol) of cinnamaldehydethiosemicarbazone dissolved in ~20 ml THF, 0.2500 g (1.83 mmol) of ZnCl2 in about 10 ml THF was added with continuous stirring and stirring was continued for 36 h. Then the solvent was evaporated in vacuo, when a yellow solid was obtained. It was repeatedly washed with cyclohexane (5 × 5 cm3) to remove any impurities present and then dried under vacuum to get free solid (yield, 0.8921 g, 89.03 %) M.P.: 103°C.
Deposition of Thin Films
Zinc sulfide thin films were deposited using aerosol-assisted chemical vapor deposition (AACVD) method. The films were grown on microscopic a glass substrate in a horizontal AACVD reactor under a flowing nitrogen atmosphere. For deposition of ZnS, 0.150 g of each precursor dissolved in methanol (20 cm3) and taken in a two-necked round bottom flask. The glass substrates were kept in nitric acid for 24 h to get a clean surface, then wash with water and acetone. Then 6–7 substrates of ~1 × 2 cm dimensions were placed inside a quartz tube in an AACVD reactor. Nitrogen gas was kept flowing throughout the experiment for maintaining the inert atmosphere and also used as carrier gas. The furnace was then heated at a rate of 25°C/min to the desired temperature under nitrogen atmosphere. The precursor solution in the two-neck round flask was placed on an ultrasonic humidifier in order to create aerosol droplets. The aerosol was carried into the hot wall zones of the AACVD reactor with the help of nitrogen as a carrier gas with a flow rate 1.5 l/h. The precursors 0.150 g in 20 cm3 methanol were used for both precursors, over a period of 1 h at substrate temperature 400, 450, and 500°C. After deposition, the reactor was cooled to room temperature under nitrogen atmosphere to avoid in situ oxidation. The deposited films were blackish reflected in nature.
Characterization
XRD studies were carried out using Cu Kα radiation on X'pert PRO PANalytical X-ray diffractometer (Philips). The morphologies of the films were analyzed by using JEOL JSM- 840 Scanning Electron Microscope (SEM) with an operating voltage of 20 kV. The elemental composition of thin films was carried out using EDAX (Inca Energy, a model of Oxford) equipped with SEM. Atomic force microscopy (AFM) analysis was carried on di-Innova (Veeco Inc) with tapping mode in ambient conditions, silicon tip with a spring constant of 20–80 N/m was used. The absorption spectra were recorded on a UV-2401 PC Shimadzu UV-Vis Spectrophotometer.
Results and Discussion
Our previous work has explored the use of Zn(II) thiosemicarbazone complexes as a single source precursor in the synthesis of ZnS nanocrystallites (Palve and Garje, 2011b). The following representative illustrations show chemical route synthesis of 4-chlorobenzaldehyde thiosemicarbazone ligand (Equation-1) and its complexes of the type Zn(L)2 (Equation-2) and ZnCl2(LH)2 (Equation-3).
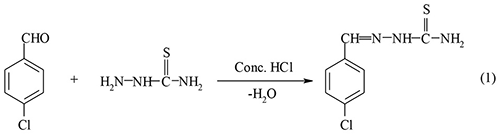
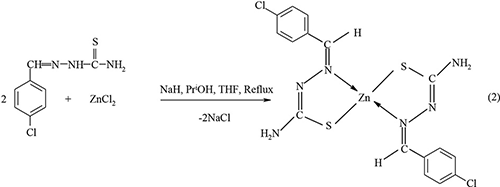
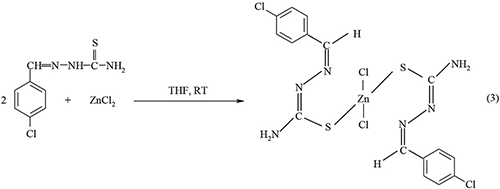
Similarly, the other complexes of the type Zn(L)2 were synthesized by elimination of sodium chloride (Equation-4) while ZnCl2(LH)2 types complexes were synthesized by direct addition reaction (Equation-5).
Where LH = thiosemicarbazones of cinnamaldehyde, 4-chlorobenzaldehyde and thiophene 2-carboxaldehyde. These precursors seem to be the promising precursor in the synthesis of metal chalcogenide films due to direct Zn-S bonding. The depositions of the thin films take place using the following route. Aerosol assisted chemical vapor deposition involves the atomization of a liquid precursor solution into submicron aerosol droplets. The aerosol droplets are generated by a piezoelectric modulator i.e., ultrasonic humidifier and transported into the hot wall zones of the reactor of chemical vapor deposition using nitrogen as a carrier gas. In the reactor the solvent undergoes rapid evaporation or decomposition, forming the vapors of precursors. This yields formation of the ZnS thin films on a glass substrate through the vapor-liquid-solid mechanism (Vallejos et al., 2016).
Figure 1 shows the thermogravimetric analysis of Zn(cinnamtscz)2 complex was carried out in order to evaluate its use as a single source precursor and understand the decomposition temperature. It was found that the weight loss obtained in TGA of Zn(cinnamtscz)2 is comparable with that of calculated for ZnS (expected 79.45%, observed 76.32) As these precursors are sparingly soluble in methanol, the warm methanol solvent is used for the deposition of thin films. AACVD of ZnS thin films is carried out in a horizontal tubular AACVD reactor. The films are deposited on a glass substrate. The deposition at 450°C was found to be reproducible with good adhesion with a glass substrate. Below 400°C the films are very poorly deposited on a glass substrate. The reflected ZnS thin films were obtained on microscopic a glass substrate in the temperature range 400–500°C.
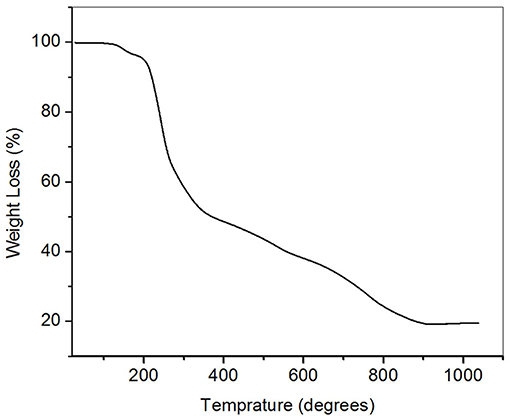
Figure 1. Thermogravimetric analysis (TGA) of Zn(cinnamtscz)2 under the nitrogen atmosphere at the heating rate of 10°C min−1.
The obtained films were characterized using X-ray diffraction study. Figures 2A,B showed XRD patterns of hexagonal ZnS thin film (JCPDS: 36-1450) as deposited from Zn(cinnamtscz)2 at 450 and 500°C. Figure 2C represents the XRD pattern of ZnS thin films deposited from Zn(4-Clbenztscz)2 at 450°C. The broadening in the XRD peaks realizes nanoscale ZnS materials were grown on a glass substrate. Figure 3 shows the XRD pattern of hexagonal ZnS thin film deposited from ZnCl2(cinnamtsczH)2 at 450°C. (JCPDS: 36-1450) using AACVD method. All the peak positions in both XRD patterns match well with the hexagonal phase of wurtzite-type ZnS. The morphology of the as- deposited thin film was studied by using SEM images. Figure 4 shows SEM images of ZnS thin film obtained by AACVD of Zn(cinnamtscz)2 at 450°C and inset image shows 1: 1 stoichiometry of zinc to sulfur. No contamination of carbon was observed in the thin films of the zinc sulfide. ZnS which was obtained from Zn(cinnamtscz)2 shows the granular type of ZnS materials. Figure 5 show SEM images of ZnS thin films obtained by deposition of ZnCl2(cinnamtsczH)2 as a SSPs at 450°C. The uniform deposition was observed with granular shape morphology using ZnCl2(cinnamtsczH)2 at 450°C. EDAX analysis of the films revealed 1:1 proportion of zinc and sulfur.
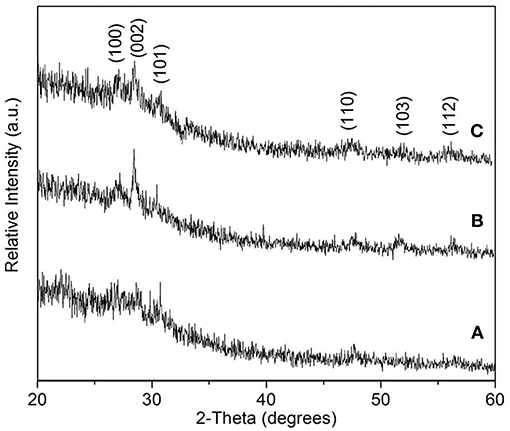
Figure 2. XRD patterns of hexagonal ZnS thin film (JCPDS: 36-1450) obtained by AACVD of Zn(cinnamtscz)2 at (A) 450°C, (B) 500°C, and (C) Zn(4-Clbenztscz)2 at 450°C.
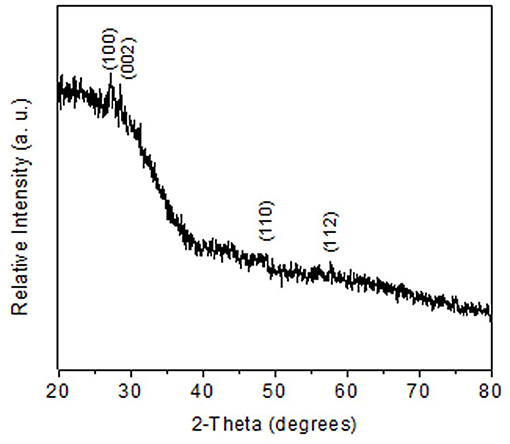
Figure 3. XRD pattern of hexagonal ZnS thin film (JCPDS: 36-1450) obtained by AACVD of ZnCl2(cinnamtsczH)2 at 450°C.
An AFM measurement of a representative film was carried out to study surface topology and roughness measurements. Figures 6A,B show AFM images of ZnS thin films deposited from ZnCl2(cinnamtsczH)2 at 450°C. AFM image is measured over 0.5 × 0.5 μm2 scanning range. The surface root- mean square roughness value was calculated from the Scanning Probe Image Processor (SPIP 5.0.1) software. It is found to be 14.33 nm.
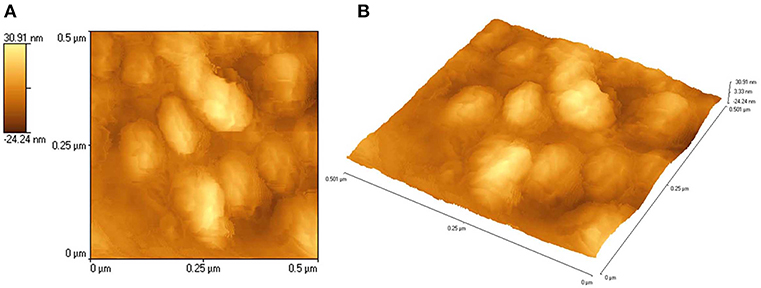
Figure 6. (A) 2D and (B) 3D AFM images of ZnS thin films deposited from ZnCl2(cinnamtsczH)2 at 450°C.
The absorption spectra of as-deposited ZnS thin films were carried out on UV-Vis spectrophotometer. Representative spectra of ZnS thin films deposited from Zn(cinnamtscz)2 and Zn(4-Clbenztscz)2 at 450°C are given in Figures 7A,B. The absorption peaks for deposited thin films from Zn(cinnamtscz)2 and Zn(4-Clbenztscz)2 are observed at 296 and 298 nm, respectively. The synthesized ZnS thin films could be used for fabricating Schottky diodes due to its suitable electrical and optical properties.
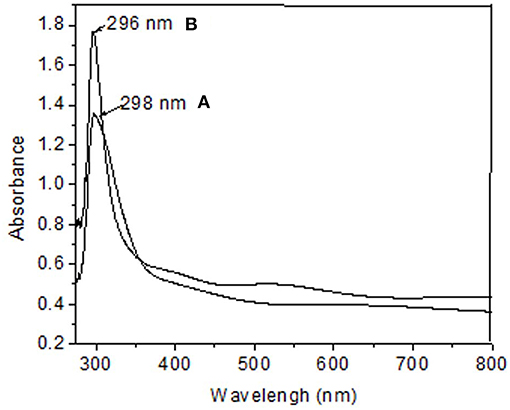
Figure 7. Absorption spectra of ZnS thin films deposited from (A) Zn(cinnamtscz)2 and (B) Zn(4-Clbenztscz)2 at 450°C.
The optical band gaps were calculated using a graphical plot of (αhν)2 vs. hν using Tauc's relation (Figures 8A,B) (Singh et al., 2008; Gupta et al., 2014). Here α is calculated using relation, α = 4πA/λ, where A is the absorbance and λ is wavelength (Koktysh et al., 2007). The extrapolation of the curves to the energy axis gave band gaps of 3.70 and 3.91 eV for ZnS thin films deposited from Zn(cinnamtscz)2 and Zn(4-Clbenztscz)2 respectively, at 450°C. The band gap values (Eg) are somewhat larger (Roy et al., 2006) are due to nano-micron scale ZnS materials.
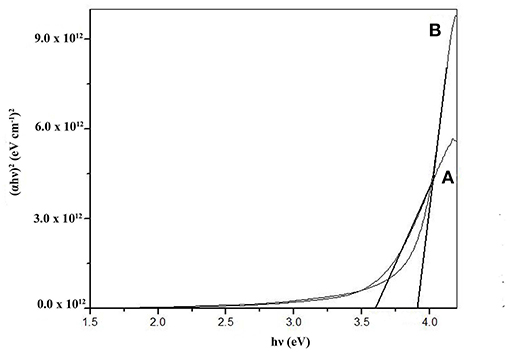
Figure 8. A plot of (αhν)2 vs. hν of ZnS thin films deposited from (A) Zn(cinnamtscz)2 and (B) Zn(4-Clbenztscz)2 at 450°C.
Conclusion
We have illustrated the facile deposition of ZnS thin films from air-stable complexes of the type Zn(L)2 and ZnCl2(LH)2 (Where LH = thiosemicarbazone ligands) as single source precursors. Pure single-phase hexagonal wurtzite-type zinc sulfide was obtained during all experiment. Morphology of the thin films was granular in shape with a controlled stoichiometry of zinc and sulfur. The use of Zn(II) thiosemicarbazone complexes shows promising results in the deposition of ZnS thin films by aerosol-assisted chemical vapor deposition. Energy dispersive X-ray analysis confirms all thin films are stoichiometrically controlled. These types of precursors may explode new area of use of such type of precursor for deposition other metal chalcogenide thin films.
Author Contributions
AP performed the experiments, carefully outlined the contents of the article and wrote the entire manuscript.
Conflict of Interest Statement
The author declares that the research was conducted in the absence of any commercial or financial relationships that could be construed as a potential conflict of interest.
Acknowledgments
Author thanks TIFR Mumbai for providing XRD, SEM, and EDAX analysis. The author also thanks Prof. Shivram Garje at the Department of Chemistry, Mumbai University for providing the UV- Visible spectral analysis.
References
Bernhardt, P. V., Sharpe, P. C., Islam, M., Lovejoy, D. B., Kalinowski, D. S., and Richardson, D. R. (2009). Iron chelators of the dipyridylketone thiosemicarbazone class: precomplexation and transmetalation effects on anticancer activity. J. Med. Chem. 52, 407–415. doi: 10.1021/jm801012z
Biswas, S., Ghoshal, T., Kar, S., Chakrabarti, S., and Chaudhuri, S. (2008). ZnS nanowire arrays: synthesis, optical and field emission properties. Cry. Growth Des. 8, 2171–2176. doi: 10.1021/cg800071g
Biswas, S., Kar, S., and Chaudhuri, S. (2005). Optical and magnetic properties of manganese-incorporated zinc sulfide nanorods synthesized by a solvothermal process. J. Phys. Chem. B 109, 17526–17530. doi: 10.1021/jp053138i
Byrom, C., Malik, M. A. O., Brien, P., White, A. J. P., and Williams, D. J. (2000). Synthesis and X-ray single crystal structures of bis(diisobutyldithiophosphinato)cadmium(II) or zinc(II): potential single-source precursors for II/VI materials. Polyhedron 19, 211–215. doi: 10.1016/S0277-5387(99)00352-6
Campbell, M. J. M. (1975). Transition metal complexes of thiosemicarbazide and thiosemicarbazones. Coord. Chem. Rev. 15, 279–319. doi: 10.1016/S0010-8545(00)80276-3
Casas, J. S., Garcia-Tasende, M. S., and Sordo, J. (2000). Main group metal complexes of semicarbazones and thiosemicarbazones. A structural review. Coord. Chem. Rev. 209, 197–261. doi: 10.1016/S0010-8545(00)00363-5
Castineiras, A., and West, D. X. (2002). Structural study of a zinc(II) complex with acetone 3-hexamethyleneiminylthiosemicarbazone. J. Mol. Struct. 604, 113–118. doi: 10.1016/S0022-2860(01)00657-3
Cockayne, B., and Wright, P. J. (1984). Metalorganic chemical vapour deposition of wide band gap II–VI compounds. J. Cryst. Growth 68, 223–230. doi: 10.1016/0022-0248(84)90420-2
Daniels, S., Christian, P., and O'Brien, P. (2006). A low temperature synthesis of ZnS nanorods. J. Exp. Nanosci. 1, 97–102. doi: 10.1080/17458080500411981
Fan, D., Thomas, P. J., and O'Brien, P. (2007). Deposition of CdS and ZnS thin films at the water/toluene interface. J. Mater. Chem. 17, 1381–1386. doi: 10.1039/B616004B
Goswami, N., and Sen, P. (2007). Photoluminescent properties of ZnS nanoparticles prepared by electro-explosion of Zn wires. J. Nanoparticle Res. 9, 513–517. doi: 10.1007/s11051-006-9137-y
Gupta, R. K., Candler, J., Kumar, D., Gupta, B. K., and Kahol, P. K. (2014). Probing on growth and characterizations of SnFe2O4 epitaxial thin films on MgAl2O4 substrate. Front Mater 1:10. doi: 10.3389/fmats.2014.00010
Hernandez-Fenollosa, M. A., Lopez, M. C., Donderis, V., Gonzalez, M. B., and Ramos-Barrado, J. R. (2008). Role of precursors on morphology and optical properties of ZnS thin films prepared by chemical spray pyrolysis. Thin Solid Films 516, 1622–1625. doi: 10.1016/j.tsf.2007.05.031
Hsu, C. T. (2000). Growth of ZnS: Tm thin films by MOCVD. J. Cryst. Growth 208, 259–263. doi: 10.1016/S0022-0248(99)00410-8
Koktysh, D. S., McBride, J. R., and Rosenthal, S. J. (2007). Synthesis of SnS nanocrystals by the solvothermal decomposition of a single source precursor. Nanoscale Res. Lett. 2, 144–148. doi: 10.1007/s11671-007-9045-9
Kurup, M. R. P., and Joseph, M. (2003). Transition metal complexes of furan-2-aldehyde thiosemicarbazone. Synth. React. Inorg. Met-Org. Chem. 33, 1275–1287. doi: 10.1081/SIM-120023500
Lee, E. Y. M., Tran, N. H., and Lamb, R. N. (2005). Growth of ZnS films by chemical vapor deposition of Zn[S2CN(CH3)2]2 precursor. Appl. Surf. Sci. 241, 493–496. doi: 10.1016/j.apsusc.2004.07.003
Liu, S., Zhang, H., and Swihart, M. T. (2009). Spray pyrolysis synthesis of ZnS nanoparticles from a single-source precursor. Nanotechnology 20:235603 doi: 10.1088/0957-4484/20/23/235603
Lobana, T. S., Sharma, R., Bawa, G., and Khanna, S. (2009). Bonding and structure trends of thiosemicarbazone derivatives of metals—an overview. Coord. Chem. Rev. 253, 977–1055. doi: 10.1016/j.ccr.2008.07.004
Luo, Y., Duan, G., Ye, M., Zhang, Y., and Li, G. (2008). Poly(ethylene glycol)-mediated synthesis of hollow ZnS microspheres. J. Phys. Chem. C 112, 2349–2352. doi: 10.1021/jp076047p
Padhye, S. B., and Kauffman, G. (1985). Transition metal complexes of semicarbazones and thiosemicarbazones. Coord. Chem. Rev. 63, 127–160. doi: 10.1016/0010-8545(85)80022-9
Palve, A. M., and Garje, S. S. (2011a). A facile synthesis of ZnS nanocrystallites by pyrolysis of single molecule precursors, Zn (cinnamtscz)2 and ZnCl2 (cinnamtsczH)2. Bull. Mat. Sci. 34, 667–671. doi: 10.1007/s12034-011-0179-0
Palve, A. M., and Garje, S. S. (2011b). Preparation of zinc sulfide nanocrystallites from single-molecule precursors. J. Cryst. Growth 326, 157–162. doi: 10.1016/j.jcrysgro.2011.01.087
Pathan, H. M., and Lokhande, C. D. (2004). Deposition of metal chalcogenide thin films by successive ionic layer adsorption and reaction (SILAR) method. Bull. Mater. Sci. 27, 85–111. doi: 10.1007/BF02708491
Roy, P., Ota, J. R., and Srivastava, S. K. (2006). Crystalline ZnS thin films by chemical bath deposition method and its characterization. Thin Solid Films 515, 1912–1917. doi: 10.1016/j.tsf.2006.07.035
Seo, K. W., Yoon, S. H., Lee, S. S., and Shim, I. I.-W. (2005). Preparation of ZnS thin film using Zn(dithiocarbamate)2 precursors by MOCVD method. Bull. Korean Chem. Soc. 26, 1582–1584. doi: 10.5012/bkcs.2005.26.10.1582
Sharma, R., Agarwal, S. K., Rawat, S., and Nagar, M. (2006). Synthesis, characterization and antibacterial activity of some transition metal cis-3,7-dimethyl-2,6-octadiensemicarbazone complexes. Transition Met. Chem. 31, 201–206. doi: 10.1007/s11243-005-6374-3
Singh, P., Kumar, A., Kaushal, A., Kaur, D., Pandey, A., and Goyal, R. N. (2008). In situ high temperature XRD studies of ZnO nanopowder prepared via cost effective ultrasonic mist chemical vapour deposition. Bull. Mater. Sci. 31, 573–577. doi: 10.1007/s12034-008-0089-y
Singh, R. B., and Ishii, H. (1991). analytical potentialities of thiosemicarbazones and semicarbazones. Crit. Rev. Anal. Chem. 22, 381–409. doi: 10.1080/10408349108051640
Takahashi, Y., Yuki, R., Sugiura, M., Motojima, S., and Sugiyama, K. (1980). Vapor deposition of thin cadmium sulfide layers using thermal decomposition of dithiolatocadmium complexes. J. Cryst. Growth 50, 491–497. doi: 10.1016/0022-0248(80)90098-6
Vallejos, S., Selina, S., Annanouch, F. E., Gracia, I., Llobet, E., and Blackman, C. (2016). Aerosol assisted chemical vapour deposition of gas sensitive SnO2 and Au-functionalised SnO2 nanorods via a non-catalysed vapour solid (VS) mechanism. Sci. Rep. 6:28464. doi: 10.1038/srep28464(2016).
Wang, L. P., and Hong, G. Y. (2000). A new preparation of zinc sulfide nanoparticles by solid-state method at low temperature. Mater. Res. Bull. 35, 695–701. doi: 10.1016/S0025-5408(00)00261-0
Xiong, Q., Chen, G., Acord, J. D., Liu, X., Zengel, J. J., Gutierrez, H. R., et al. (2004). Optical properties of rectangular cross-sectional ZnS nanowires. Nano Lett. 4, 1663–1668. doi: 10.1021/nl049169r
Zhenyi, F., Yichao, C., Yongliang, H., Yaoyuan, Y., Yanping, D., Zewu, Y., et al. (2002). CVD growth of bulk polycrystalline ZnS and its optical properties. J. Cryst. Growth 237, 1707–1710. doi: 10.1016/S0022-0248(01)02338-7
Keywords: ZnS thin films, aerosol assisted chemical vapor deposition (AACVD), semiconducting II- VI materials, zinc compounds, light emitting diodes, Schottky diodes
Citation: Palve AM (2019) Deposition of Zinc Sulfide Thin Films From Zinc(II) Thiosemicarbazones as Single Molecular Precursors Using Aerosol Assisted Chemical Vapor Deposition Technique. Front. Mater. 6:46. doi: 10.3389/fmats.2019.00046
Received: 21 January 2019; Accepted: 25 February 2019;
Published: 21 March 2019.
Edited by:
Ram Gupta, Pittsburg State University, United StatesReviewed by:
Javeed Akhtar, Mirpur University of Science and Technology, PakistanSatish K. Pardeshi, Savitribai Phule Pune University, India
Anil Vithal Ghule, Shivaji University, India
Copyright © 2019 Palve. This is an open-access article distributed under the terms of the Creative Commons Attribution License (CC BY). The use, distribution or reproduction in other forums is permitted, provided the original author(s) and the copyright owner(s) are credited and that the original publication in this journal is cited, in accordance with accepted academic practice. No use, distribution or reproduction is permitted which does not comply with these terms.
*Correspondence: Anil M. Palve, cGFsdmVfYW5pbEB5YWhvby5jb20=