- 1State Key Laboratory of Electronic Thin Films and Integrated Devices, School of Optoelectronic Science and Engineering, University of Electronic Science and Technology of China, Chengdu, China
- 2International Collaborative Laboratory of 2D Materials for Optoelectronic Science and Technology, Engineering Technology Research Center for 2D Material Information Function Devices and Systems of Guangdong Province, College of Optoelectronic Engineering, Shenzhen University, Shenzhen, China
The functional groups of organic gas-sensing materials play a crucial role in adsorbing specific gas molecules, which is significant to the sensing performances of gas sensor. In this work, amido-graphene oxide (GO-NH2) loaded poly(ethyleneimine) (PEI) composite thin film (PEI/GO-NH2) with abundant amino functional groups -NH2 was successfully prepared on quartz crystal microbalance (QCM) by a combined spraying and drop coating method for acetone detection at room temperature (25°C). The morphological, spectrographic and acetone-sensing properties of composite film were investigated. The results demonstrated that a wrinkled surface morphology was formed and the ratio of nucleophilic -NH2 was increased for PEI/GO-NH2 composite film. Meanwhile, the composite film sensor possessed excellent acetone-sensing performances, and its sensitivity was about 4.2 times higher than that of pure PEI one owing to the increased -NH2 groups. This study reveals the important role of absorbing favorable functional groups and provides a novel method for the rational design and construction of acetone-sensing materials.
Introduction
Acetone is a volatile, flammable and deleterious industrial product that widely used in our daily life (Jia et al., 2014). In addition, acetone detection can be applied to painless diabetes diagnosis because the concentration of acetone exhaled by diabetics is much higher than that by healthy people (Righettoni and Tricoli, 2011; Shin et al., 2013; Zhang et al., 2017). Therefore, it is urgent to develop acetone gas sensors with excellent performances through optimizing the sensing materials (Wang et al., 2017). So far, many types of acetone gas sensors have been developed (Xu et al., 2009; Epifani et al., 2015; Kim et al., 2016; Wang et al., 2016), in which most of them were chemiresistive sensor operated at high temperature with metal-oxide sensing-materials. On the other hand, quartz crystal microbalance (QCM, for short) is an excellent and reliable mass gas sensing device that can detect mass change at sub-nanogram level and has attracted attention for its room working temperature, high sensitivity, good stability, and small physical size (Matsuguchi and Uno, 2006; Lal and Tiwari, 2018). The gas sensing properties of QCM gas sensor are mainly determined by the coated sensing material to the gas molecules (Wang et al., 2017). Conductive polymer materials were widely used as sensing films in QCM gas sensors because they possess specific functional groups for adsorbing target gas molecules. In order to develop high performance gas sensor based on QCM, one of important strategies is to construct gas sensing materials with special functional groups. As a typical organic polymer, poly(ethyleneimine) (PEI) can be used in QCM-based acetone sensors due to the amino functional groups (-NH2, for short) that can adsorb acetone molecules through nucleophilic addition reaction (Smith and March, 2007). However, the acetone-sensing properties of pure PEI based QCM gas sensors are restricted and still need to be further improved. Inspired by the large specific surface area of graphene oxide (GO, for short) and the importance of functional groups in gas sensing materials, compositing with GO to improve the acetone performance of PEI is a good strategy. In view of the amide reaction between—NH2 of PEI and hydroxyl groups (-OH, for short) of GO (Tai et al., 2016), the amido-graphene oxide (GO-NH2, for short) could be chose to prepare composite film, and the acetone-sensing properties would be enhanced by loading more -NH2 onto the PEI film. In this work, PEI film loaded GO-NH2 (PEI/GO-NH2, for short) with abundant -NH2 was prepared by a combined spraying and drop coating method, and characterized by scanning electron microscopy (SEM), X-ray photoelectron spectroscopy (XPS), ultraviolet-visible spectroscopy (UV-vis). The QCM acetone sensors based on PEI and PEI/GO-NH2 films were fabricated and their acetone gas sensing properties including dynamic response, repeatability, selectivity and stability were investigated. Moreover, the mechanisms for the enhanced acetone sensing properties of the PEI/GO-NH2 gas sensors were discussed.
Experimental
All the chemicals used in the experiment were analytical reagents. PEI aqueous solution [50% (w/v)] was obtained from Sigma-Aldrich and diluted to 1% (w/v) by deionized water. GO-NH2 aqueous solution (0.5 mg/ml) was prepared by Shenzhen University (-NH2 was carried by 3-aminopropyltriethoxysilane (APTES), which was modified on GO by a one-pot hydrothermal method described in Liu et al. (2013). QCM devices (Wuhan Hitrusty Electronics Co., Ltd., China) consist of AT-cut quartz crystal with a fundamental resonance frequency of 10 MHz and the 5 mm diameter sliver electrodes were used on both sides of devices.
PEI/GO-NH2 composite thin film was deposited on QCM devices by spraying 0.9 ml PEI solution and dropping 20 μl GO-NH2 solution in sequence. After the deposition, the composite thin film sensors were dried in vacuum drying oven at 80°C for 48 h. For comparison, pure PEI film QCM gas sensor was also fabricated by the same spray method. The SEM, XPS and UV-vis of PEI and PEI/GO-NH2 composite films were characterized by S4800 (HITACHI, Japan), UV-1700 (Shimadzu, Japan), and ESCALAB 250Xi (Thermo Fisher, American), respectively.
The details for measurement of QCM gas sensors can refer to our previous work (Yuan et al., 2016). The acetone gas was generated by MF-3C (Beijing Ruiyisi Technology Co., Ltd.). QCM-5 Oscillator (ShenyangVacuum Technology Institute, China) drived the QCM gas sensors, and an SS7200 intelligent frequency counter (The Fourth Radio Factory, China) was used to measure the resonance-frequency of QCM gas sensors. All the sensing measurements were executed at room temperature 25°C. The response (Δf) of the sensor is calculated as following equation: Δf = fair-fgas, where fair and fgas is the frequency measured in air and target gases, respectively. The slope coefficient of response vs. gas concentrations curve is defined as sensitivity of the gas sensor:
where ΔConc. is the gas range of linear concentration. The response/recovery times (τres/τrec) are defined as the time spans to the sensor achieved 90% of total frequency change exposure to gas and air.
Results and Discussion
The SEM and XPS of pure PEI and PEI/GO-NH2 composite films are shown in Figure 1. As can be seen, the SEM image in Figure 1A indicates a smooth and uniform deposition of PEI film. However, Figure 1B displays a rough and wrinkled surface morphology caused by GO-NH2, which should be beneficial to enlarging the specific surface area of sensing film and improving the adsorption capacity of gas molecules (Kuila et al., 2011). As shown in Figure 1C, the binding energy of GO-NH2 at O1s can be assigned to individual peaks at 283.7, 284.5, 285.5, 286.2, and 287.6 eV, corresponding to C-Si,C-C/C-H,C-OH/ C-N/C-O-Si, C-O, and C = O, respectively, which are conformed to the structure diagram (inset of Figure 1C) of GO-NH2 (Liu et al., 2013). N1s spectra of pure PEI and PEI/GO-NH2 are curve fitted and shown in Figure 1D. The N1s peaks are assigned to -NH2 and protonated amine groups (-NH2H+), respectively. The N1s peaks of PEI/GO-NH2 shifted about 1.3 eV compared with that of pure PEI, implying the existence of hydrogen bonds between PEI and GO-NH2 (Finšgar et al., 2009). On the other hand, -NH2 are nucleophilic while -NH2H+ are not nucleophilic, so the increased ratio of nucleophilic -NH2 in PEI/GO-NH2 (from 30.6 to 64.6%) can increase the acetone gas molecules adsorption and enhance the acetone-sensing properties of composite thin film (Smith and March, 2007).
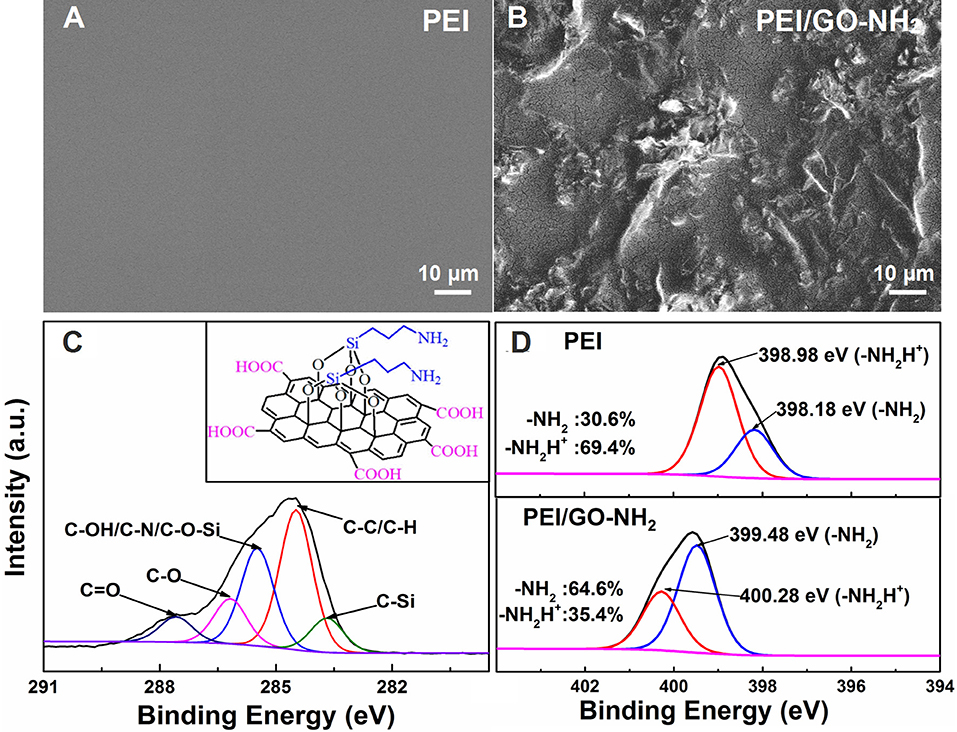
Figure 1. SEM images of (A) PEI and (B) PEI/GO-NH2 film. High-resolution XPS of (C) C1s spectra of PEI/GO-NH2 and (D) N1s spectra of pure PEI and PEI/GO-NH2, the inset of (C) is GO-NH2 material structure.
Typical dynamic responses of pure PEI and PEI/GO-NH2 film sensors vs. acetone concentration (2–10 ppm) were measured at room temperature (25°C), as shown in Figure 2A. It can be observed that the responses of two gas sensors increase gradually with the increasing acetone concentrations and reach saturation, and the frequency come back to the initial state with insignificant baseline drift after the acetone is replaced by dry air. The response of pure PEI and PEI/GO-NH2 sensors to 10 ppm acetone is about 21.1 and 55.4 Hz, respectively. Figure 2B demonstrates the linear fitting curves of two gas sensors, indicating both pure PEI and PEI/GO-NH2 film sensors are of good linearity to 2–10 ppm acetone. The sensitivity of pure PEI and PEI/GO-NH2 QCM gas sensor is 0.86 and 3.62 Hz/ppm, respectively, indicating that the sensitivity of PEI/GO-NH2 composite thin film sensor shows about 4.2 times higher than that of pure PEI one. It is worth noting that the PEI/GO-NH2 film sensor could detect 1 ppm acetone with 6.4 Hz response as shown in the inset of Figure 2A, whereas PEI film sensor shows no obvious response. Figure 2C shows good repeatability for two film sensors on successive exposure to 10 ppm acetone. It can be observed that the responses of two sensors exhibit very little fluctuation in adsorption and desorption process, indicating excellent repeatability and baseline stability. The response and recovery times of the PEI/GO-NH2 film sensor are 81 s and 148 s. As shown in Figure 2D, the selectivity of PEI/GO-NH2 acetone sensor was investigated in comparison to different kinds of interference gases (10 ppm HCHO, CH4, NH3, SO2, H2S, and 1,000 ppm CO2), which demonstrate that the composite film has higher response to acetone gas than other gases. Except for formaldehyde and acetone, other gases have no carbon-heteroatom double bond (C = O) which plays an important role in nucleophilic addition reaction with –NH2. In addition, the molecular mass of formaldehyde is less than that of acetone at the same concentration. Therefore, PEI/GO-NH2 composite film shows a comparatively good selectivity to acetone. The insert in Figure 2D shows the long-term stability of PEI/GO-NH2 acetone sensor during a period of 6 weeks. The result shows the response of composite sensor to 10 ppm acetone decreases about 18% in the first 14 days and tends to be stable afterwards.
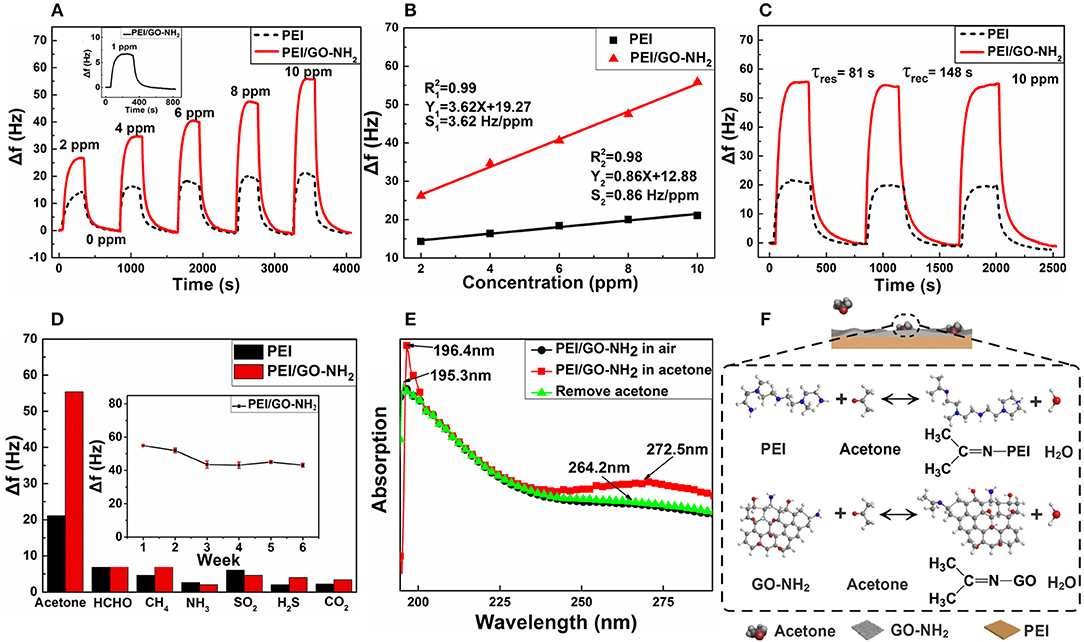
Figure 2. (A) Dynamic response curves. (B) Linear fitting curves. (C) Repeatability curves and (D) selectivity of PEI and PEI/GO-NH2 QCM sensors to acetone, the inset of (A) is the response curve of PEI/GO-NH2 sensor for 1 ppm acetone, the inset of (D) is long-term stability curve. (E) UV-Vis spectrum of PEI/GO-NH2 films. (F) Schematic of acetone-sensing mechanism.
UV-Vis spectra were carried out to further confirm the recoverability of PEI/GO-NH2 film. As shown in Figure 2E, when exposed to acetone atmosphere, enhancement and redshift of absorption peaks appeared in PEI/GO-NH2 film, representing the adsorption of acetone molecules. When above films were replaced back to the air atmosphere, the absorption peaks also restored to their positions, indicating the good recovery characteristic of the films. The acetone-sensing mechanism is further proposed. Figure 2F indicates the chemical reaction during adsorption/desorption process. Carbon atom of polar carbon-heteroatom double bond (C = O) on acetone molecule carries a partial positive charge, making the carbon atom as electrophilic center. When exposed to acetone gas, negatively charged nucleophilic -NH2 on PEI/GO-NH2 film will attack and bond with the electrophilic carbon atom to form an imine and produce a molecule of water. The above adsorption will lead to mass increase of PEI/GO-NH2 film and the frequency shift of QCM sensor based on Sauerbrey's equation (Sauerbrey, 1959). According to the pattern followed by analogous nucleophiles, the produced compounds are generally unstable. In the process of acetone molecular desorption, carbon atom of polar carbon-nitrogen double bond (C = N) for produced compounds carries a partial positive charge, produced water molecule will bond with the electrophilic carbon atom to form reversible reaction, thus the PEI/GO-NH2 film coated QCM acetone sensors possess good response and recoverability (Madura and Jorgensen, 1986; Smith and March, 2007).
Conclusion
In summary, PEI/GO-NH2 composite film was fabricated on QCM via a combined spraying and drop coating method. Moreover, the acetone-sensing properties were investigated at room temperature (25°C). Compared with the pure PEI film based QCM acetone sensor, PEI/GO-NH2 composite film sensor exhibited an improved acetone-sensing performance, and its sensitivity was about 4.2 times higher than that of pure PEI film. The gas sensor based on PEI/GO-NH2 composite film showed nearly linear response at acetone gas concentrations ranges of 2–10 ppm, and its sensitivity was about 3.62 Hz/ppm. In addition, the PEI/GO-NH2 composite film sensor possessed good repeatability and selectivity. The enhanced acetone-sensing performances could be attributed to the rich -NH2 and enlarged surface area introduced by GO-NH2. This work demonstrated a promising PEI/GO-NH2 composite film for high-performance acetone-sensing materials construction.
Author Contributions
QZ and ZH co-wrote the submitted perspective and performed the experiments. HW and CS prepared GO-NH2 aqueous solution. YJ, ZY, and HT designed and guided the entire experimental process.
Conflict of Interest Statement
The authors declare that the research was conducted in the absence of any commercial or financial relationships that could be construed as a potential conflict of interest.
Acknowledgments
This work is supported by the National Science Funds for Excellent Young Scholars of China (Grant No. 61822106), National Science Funds for Creative Research Groups of China (Grant No. 61421002), and Natural Science Foundation of China (Grant No. 61671115).
References
Epifani, M., Comini, E., Siciliano, P., Faglia, G., and Morante, J. R. (2015). Evidence of catalytic activation of anatase nanocrystals by vanadium oxide surface layer: acetone and ethanol sensing properties. Sensors Actuat. B Chem. 217, 193–197. doi: 10.1016/j.snb.2014.07.118
Finšgar, M., Fassbender, S., Hirth, S., and Milošev, I. (2009). Electrochemical and XPS study of polyethyleneimines of different molecular sizes as corrosion inhibitors for AISI 430 stainless steel in near-neutral chloride media. Mater. Chem. Phys. 116, 198–206. doi: 10.1016/j.matchemphys.2009.03.010
Jia, Q., Ji, H., Zhang, Y., Chen, Y., Sun, X., and Jin, Z. (2014). Rapid and selective detection of acetone using hierarchical ZnO gas sensor for hazardous odor markers application. J. Hazard. Mater. 276, 262–270. doi: 10.1016/j.jhazmat.2014.05.044
Kim, N. H., Choi, S. J., Kim, S. J., Cho, H. J., Jang, J. S., Koo, W. T., et al. (2016). Highly sensitive and selective acetone sensing performance of WO3 nanofibers functionalized by Rh2O3 nanoparticles. Sensors Actuators B Chem. 224, 185–192. doi: 10.1016/j.snb.2015.10.021
Kuila, T., Bose, S., Khanra, P., Mishra, A. K., Kim, N. H., and Lee, J. H. (2011). Recent advances in graphene-based biosensors. Biosens. Bioelectron. 26, 4637–4648. doi: 10.1016/j.bios.2011.05.039
Lal, G., and Tiwari, D. C. (2018). Investigation of nanoclay doped polymeric composites on piezoelectric Quartz Crystal Microbalance (QCM) sensor. Sensors Actuat. B Chem. 262, 64–69. doi: 10.1016/j.snb.2018.01.200
Liu, Z., Duan, X., Qian, G., Zhou, X., and Yuan, W. (2013). Eco-friendly one-pot synthesis of highly dispersible functionalized graphene nanosheets with free amino groups. Nanotechnology 24:045609. doi: 10.1088/0957-4484/24/4/045609
Madura, J. D., and Jorgensen, W. L. (1986). Ab initio and Monte Carlo calculations for a nucleophilic addition reaction in the gas phase and in aqueous solution. J. Am. Chem. Soc. 108, 2517–2527. doi: 10.1021/ja00270a005
Matsuguchi, M., and Uno, T. (2006). Molecular imprinting strategy for solvent molecules and its application for QCM-based VOC vapor sensing. Sensors Actuat. B Chem. 113, 94–99. doi: 10.1016/j.snb.2005.02.028
Righettoni, M., and Tricoli, A. (2011). Toward portable breath acetone analysis for diabetes detection. J. Breath Res. 5:037109. doi: 10.1088/1752-7155/5/3/037109
Sauerbrey, G. (1959). Verwendung von schwingquarzen zur wägung dünner schichten und zur mikrowägung. Zeitschrift Für Phys. 155, 206–222. doi: 10.1007/BF01337937
Shin, J., Choi, S. J., Lee, I., Youn, D. Y., Park, C. O., Lee, J. H., et al. (2013). Thin-wall assembled SnO2 fibers functionalized by catalytic Pt nanoparticles and their superior exhaled-breath-sensing properties for the diagnosis of diabetes. Adv. Funct. Mater. 23, 2357–2367. doi: 10.1002/adfm.201202729
Smith, M. B., and March, J. (2007). March's Advanced Organic Chemistry: Reactions, Mechanisms, and Structure, Sixth Edition. Hoboken, NJ: John Wiley and Sons.
Tai, H., Yuan, Z., Liu, C., Ye, Z., Xie, G., Du, X., et al. (2016). Facile development of high performance QCM humidity sensor based on protonated polyethylenimine-graphene oxide nanocomposite thin film. Sensors Actuat. B Chem. 230, 501–509. doi: 10.1016/j.snb.2016.01.105
Wang, L., Wang, Z., Xiang, Q., Chen, Y., Duan, Z., and Xu, J. (2017). High performance formaldehyde detection based on a novel copper (II) complex functionalized QCM gas sensor. Sensors Actuat. B Chem. 248, 820–828. doi: 10.1016/j.snb.2016.12.015
Wang, P., Wang, D., Zhang, M., Zhu, Y., Xu, Y., Ma, X., et al. (2016). ZnO nanosheets/graphene oxide nanocomposites for highly effective acetone vapor detection. Sensors Actuat. B Chem. 230, 477–484. doi: 10.1016/j.snb.2016.02.056
Xu, X., Cang, H., Li, C., Zhao, Z. K., and Li, H. (2009). Quartz crystal microbalance sensor array for the detection of volatile organic compounds. Talanta 78, 711–716. doi: 10.1016/j.talanta.2008.12.031
Yuan, Z., Tai, H., Ye, Z., Liu, C., Xie, G., Du, X., et al. (2016). Novel highly sensitive QCM humidity sensor with low hysteresis based on graphene oxide (GO)/poly (ethyleneimine) layered film. Sensors Actuat. B Chem. 234, 145–154. doi: 10.1016/j.snb.2016.04.070
Keywords: amino functional groups, composite materials, poly(ethyleneimine), amido-graphene oxide, acetone sensing performances
Citation: Zhao Q, He Z, Jiang Y, Yuan Z, Wu H, Su C and Tai H (2019) Enhanced Acetone-Sensing Properties of PEI Thin Film by GO-NH2 Functional Groups Modification at Room Temperature. Front. Mater. 5:82. doi: 10.3389/fmats.2018.00082
Received: 17 November 2018; Accepted: 20 December 2018;
Published: 15 January 2019.
Edited by:
Xiaogan Li, Dalian University of Technology (DUT), ChinaCopyright © 2019 Zhao, He, Jiang, Yuan, Wu, Su and Tai. This is an open-access article distributed under the terms of the Creative Commons Attribution License (CC BY). The use, distribution or reproduction in other forums is permitted, provided the original author(s) and the copyright owner(s) are credited and that the original publication in this journal is cited, in accordance with accepted academic practice. No use, distribution or reproduction is permitted which does not comply with these terms.
*Correspondence: Huiling Tai, taitai1980@uestc.edu.cn