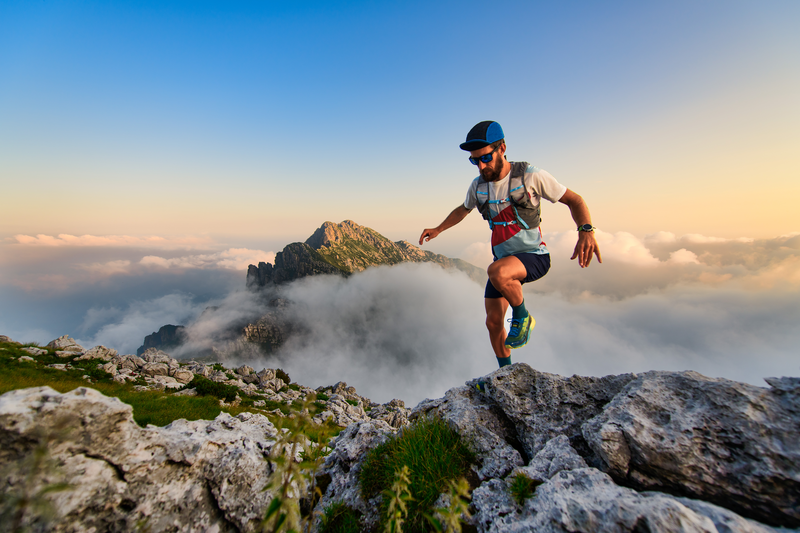
94% of researchers rate our articles as excellent or good
Learn more about the work of our research integrity team to safeguard the quality of each article we publish.
Find out more
ORIGINAL RESEARCH article
Front. Mater. , 14 December 2018
Sec. Energy Materials
Volume 5 - 2018 | https://doi.org/10.3389/fmats.2018.00074
Herein, the free-standing porous carbonized-membranes (CMs) derived from a series of commercial fiber products including airlaid papers, cellulose papers and cleanroom wipers by one-step carbonization at 160°C have for the first time explored as independent solar absorbers to realize highly efficient solar steam generation. These newly-developed CMs not only exhibit the strong absorption (low reflectance) and rapid transport of vapor/liquid, but also possess the restricted thermal diffusion. All these merits render CMs with excellent evaporation performance for solar steam generation. Particularly, the CMs derived from carbonized cellulose papers (CCPs) exhibits the best performance, which affords the water evaporation rate of 0.959 kg·m−2·h−1 and the energy conversion efficiency of 65.8% under 1 kW·m−2 solar illumination, due to the higher light absorption (92.20%) and lower thermal conductivity (0.031 W·m-1·K-1) competing favorable with those of the Au nanoparticles-loaded airlaid papers (Au-APs, 0.856 kg·m−2·h−1, 58.7%). Due to the low-cost, recyclability and highly efficient evaporation performance, the CMs, especially the CCPs, show great potential as solar absorbers for large-scale application of solar steam generation.
The solar steam generation as one of the most promising technology for the water scarcity (Shannon et al., 2008; Elimelech and Phillip, 2011; Ni et al., 2016), which focuses on the effective conversion from solar energy to thermal energy, has attracted a lot of research attention (Elimelech and Phillip, 2011; Zhou et al., 2016c). Such hopeful and efficient technology of light-to-heat conversion can be widely used in the field of purification of drinking water, domestic water heating (Ghasemi et al., 2014; Ni et al., 2016), solar desalination (Zhu et al., 2016; Liu et al., 2017), absorption chillers (Schiermeier, 2008; Philibert, 2011; Lewis, 2016), and sewage treatment (Liu et al., 2015b; Lou et al., 2016), etc. Nevertheless, the traditional techniques of the solar steam generation generally rely on complicated and costly optical concentrators to enhance the water evaporation, leading to a large number of energy loss and the maintenance expense of the facilities in the long term (Karagiannis and Soldatos, 2008; Lenert and Wang, 2012; El-Agouz et al., 2014; Liu et al., 2015c). Therefore, it is necessary for solar steam generation to exploit more cost-effective and efficient evaporators.
Generally, the highly efficient solar steam generation strongly depends on the efficient light-absorbers in the evaporators. In the previous studies, the plasmonic (Wang et al., 2014; Bae et al., 2015; Liu et al., 2015c; Zhou et al., 2016b, 2017), and carbon-based materials (Ito et al., 2015; Zhou et al., 2016a; Hu et al., 2017), have been extensively studied among the reported absorbers due to their remarkable solar-thermal conversion efficiency. Nevertheless, in order to lower the cost and increase the evaporation efficiency, most previous reports usually added low-cost/insulated supports [e.g., airlaid paper Liu et al., 2015c, 2017; Lou et al., 2016; Wang et al., 2017a,b] with effective light-absorbing materials [e.g., carbon black (Liu et al., 2015a, 2017) and gold nanoparticles (Liu et al., 2015c; Wang et al., 2017b)] to construct the multilayer-composite absorbers. However, the composite structures often suffer from the costly preparation (complex process) and interfacial barriers to heat/mass transfer, which would be adverse to the large-area industrial manufacture (Lin et al., 2018). Therefore, ideal solar absorbers should not only afford the high light-to-heat conversion efficiency for the efficient solar steam generation, but also render cost-effective and facile preparation for the large scale industrial manufacture.
Due to the low-cost and high-abundance, the commercial fiber products, such as the airlaid papers (APs), cellulose papers (CPs) and cleanroom wipers (CWs), have been used in cleaning of the optical/medical devices, batteries separators, and solar steam generation (Nyholm et al., 2011; Xu et al., 2015; Wang et al., 2017a,b). They could not only be a great support layer for absorbing materials, but also have great potential to be an independent solar absorber without any additive of absorbing layer, owing to the advantages of lightweight, mechanical stability, and low thermal conductivity (Liu et al., 2017).
In this work, we demonstrate that the free-standing porous carbonized-membranes (CMs) derived from the commercial fiber products (e.g., APs, CPs, CWs) via the one-step carbonization at 160°C, for the first time, can be directly used as independent solar absorbers to realize highly efficient solar steam generation. Our proposed CMs could show multiple advantages to promote the evaporation efficiency including strong absorption (low reflectance) due to the carbonization, rapid transport of vapor/liquid because of the porous structure, and restricted thermal diffusion ascribed to low thermal conductivity. As expected, the carbonized cellulose papers (CCPs) with higher light absorption (92.20%) and lower thermal conductivity (0.031 W·m−1·K−1) as our optimal CMs yields the highest water evaporation rates (0.959 kg·m−2·h−1) and energy conversion efficiency (65.8%) under 1 kW·m−2 solar illumination, competing favorable with those of the Au nanoparticles-loaded airlaid papers (Au-APs, 0.856 kg·m−2·h−1, 58.7%).
The commercial cellulose papers were purchased from Kimberly-Clark Corporation (USA, 34155 KIMWIPES) with a size of 11 cm × 21 cm. The commercial airlaid papers were purchased from CLEANROOM (China, WIP-0609) with a size of 22.86 cm × 22.86 cm. The commercial cleanroom wipers (polyester fiber) were purchased from CLEANROOM (China, WIP-2009) with a size of 22.86 cm × 22.86 cm.
Au-APs were firstly produced based on the literature reported method (Liu et al., 2015c). Typically, 50 mL of 1 mM HAuCl4 aqueous solution (prepared by Chloroauric acid tetrahytrate, Sinopharm Chemical Reagent Co., Ltd., 99.99%) was added into a round-bottom flask (100 mL) with a condenser and boiled with vigorous stirring. Subsequently, 5 mL of 38.8 mM sodium citrate solution (Guangzhou Chemical Reagent Factory) was added into the HAuCl4 aqueous solution. We note that the color of mixture solution turned from pale yellow to kermesinus. Afterwards, the mixture solution was boiled for 15 min and kept stirring (stop heating) for 1 h until the solution cooled down. Finally, the aqueous solution of colloidal Au nanoparticles was obtained.
The preparation of Au-APs were described as follows: a piece of clipped AP was placed at bottom of the 100-mL beaker. Afterwards, the beaker was filled with 30 mL of colloidal Au nanoparticles aqueous solution. Simultaneously, the beaker together with a petri dish filling with 10 mL of formic acid (Guangzhou Chemical Reagent Factory) was placed inside a sealed desiccator at room temperature. After 48 h, the Au nanoparticles film could be formed at the air-water interface. Followed by extracting the solution between the bottomed AP and the Au nanoparticles film, the film would be coated onto the AP. Finally, the Au-APs were achieved by drying at 50°C in the oven.
In a typical experiment, a piece of fabric (including APs, CPs, CWs) was dipped into the H2SO4:water:ethanol mixture solution (1:25:60, volume ratio) and was heated in an oven for 4 h under the normal atmosphere at 160°C. Finally, after washing by the deionized water, various CMs were achieved via drying.
The UV-Vis diffuse reflectance spectra and transmittance spectra of the CMs were measured using a UV/VIS/NIR spectrophotometer (UV3600, Shimadzu) combined with an integrating sphere (ISR-3100). The absorptance was calculated by A = 1-R-T, where R and T were the reflectance and transmittance, respectively. The surface compositions of the CMs were measured with a photo-electron spectroscopy (XPS, ESCALAB 250, Thermofisher Scientific) equipped with a monochromatic X-ray source (Al Kα, 1486.6 eV). The contents of the C, H, S, O of the CMs were tested by the Elemental Analyzer (EA, Vario EL, Elementar). The field emission scanning electron microscopy (FE-SEM, Hitachi S-4800) was used to obtain the SEM images. The thermal conductivity of the CMs were measured by a thermal conductivity measuring instrument (TPS 550 S, Hot disk). The concentrations of Fe3+, Al3+, Cr3+, Cu2+, Mn2+, and Ni2+ of the CMs were measured using plasma atomic emission spectrometry (ICP-AES, IRIS(HR), TJA).
The light source of the test system was generated by a 300 W xenon lamp (simulated solar irradiation, Microsolar 300, Perfectlight), which was calibrated by a photoradiometer (PL-MW2000, Perfectlight). The change of the mass was accurately recorded by an electronic balance (AR224CN, OHAUS) coupled with a computer for the calculation of the water evaporation rate. Simultaneously, an infrared radiation (IR) camera (T420, FLIR) monitored the real-time temperature for the final calculation of energy conversion efficiency.
In the previous reports, the APs was often used as a substrate to support the plasmonic absorbers or the carbon-based absorbers for notable water evaporation efficiency, respectively. The increased surface roughness of the APs substrate could cause the multiple scattering of incident solar light, leading to the high absorption of the sunlight. Simultaneously, the porous structure of the APs could potentially utilize the capillarity to facilitate the water forward the hot zone, resulting in the rapid supplement for the loss of the surface water evaporation. Moreover, airlaid paper also could be as a good thermal insulator to prevent the thermal loss because of the low thermal conductivity (Liu et al., 2015c, 2017). However, the composite structures could suffer from the complicated preparation and interfacial barriers to heat/mass transfer (Lin et al., 2018). Therefore, the commercial fiber products such as APs might be directly applied as a light-to-heat conversion absorber for solar steam generation.
For this purpose, the carbonized airlaid paper (CAPs), which were based on the precursor of commercial APs (composed of 55% cellulose and 45% polyester), have been chosen. In order to inherit the porous structure and lightweight (ca. 80 g·m−2, see in Supplementary Table S1), the CAPs were directly obtained from APs through a simple carbonized process using H2SO4:water:ethanol mixture solution (Figure 1) in an oven under the normal atmosphere. The CAPs could sufficiently retain the porous structures as evidenced by the scanning electron microscopy (SEM) images in Supplementary Figure S1. The samples were of low densities and could safely float on the surface of the water. Such open-pore structure would provide transportation channels for the rapid transport of vapor and liquid (Hu et al., 2017; Lin et al., 2018). Moreover, due to the specific microstructures of APs, the local convection would be suppressed during the water evaporation (Liu et al., 2015c; Lin et al., 2018).
Figure 1. (A) The photographs of APs, Au-APs and CAPs. (B) The fabrication process of the carbonized airlaid paper.
As shown in the schematic diagram in Figure 2 and the photograph in Supplementary Figure S2, the test system of the solar steam generation ability for the CMs consists of a simulated solar irradiation, a weighing module and a temperature monitoring system. The CMs with a diameter of ca. 4 cm was floated on the surface of the water in a weighing bottle, which was located on the electronic balance under the light source. When subjected to the illumination, the mass change of water during the solar steam generation was detected by the weighing module, which was equipped with the electronic balance and the computer. Simultaneously, the real-time temperature on the surface of the CMs was measured by an infrared radiation (IR) camera (ambient temperature:25°C and humidity: 50%).
Figure 2. The schematic diagram of the test system for solar steam generation ability. Bottom left corner: the schematic of the free-floating CMs on the water contained in a weighing bottle; Top right corner: the IR image of the CMs under illumination.
The typical curves of the mass change vs. time under 1 sun illumination (Copt = 1, solar illumination with a power density of 1 kW·m−2) for different samples are exhibited in Figure 3A, while the water evaporation rates (ṁ) are calculated from the slope of the curves (Supplementary Table S1). Under the solar illumination with a power density of 1 kW·m−2, the average water evaporation rates of CAPs were measured to be 0.924 kg·m−2·h−1, ca. 2.1 times those of pure water (0.431 kg·m−2·h−1) and APs (0.440 kg·m−2·h−1). Moreover, another reference sample of the Au-APs (shown in Figure 1A) was fabricated to obtain an evaporation rate of 0.856 kg·m−2·h−1, which was lower than that of CAPs (0.924 kg·m−2·h−1). Compared with previous reports (Liu et al., 2015c, 2017; Lou et al., 2016; Wang et al., 2017a,b). the optimal evaporation rate of CAPs (0.924 kg·m−2·h−1) representsone of the best AP-based solar absorbers' results for solar steam generation under similar temperature and humidity (Copt = 1, 25°C, 50%).
Figure 3. (A) The typical curves of the mass change vs. time for pure water, APs, Au-Aps, and CAPs under 1 sun illumination. (B) The photographs of APs, CAPs, CPs, CCPs, CWs, and CCWs. (C) The typical curves of the mass change vs. time for pure water, APs, CAPs, CPs, CCPs, CWs, and CCWs under 1 sun illumination. (D) The reflectance spectra of Au-APs, APs, CAPs, CPs, CCPs, CWs, and CCWs.
However, it is well-known that the characteristics of the cellulose, such as the thermal conductivity and absorbency, are obviously different from that of the polyester (Idicula et al., 2006), while the composition of the APs is 55% cellulose and 45% polyester. Therefore, it indicates that optimizing the content of the commercial fiber products' cellulose and polyester could effectively improve their thermal conductivity and absorbency, in order to help reduce heat loss and increase the utilization of solar energy that the potentially improve the solar steam generation ability of the CMs (Hu et al., 2017). The commercial CPs (100% cellulose, cellulose papers) and CWs (100% polyester, cleanroom wipers) would be chosen as the sources to prepare various CMs in the same way, which was labeled as CCPs (carbonized cellulose papers) and CCWs (carbonized cleanroom wipers), respectively (Figure 3B).
As shown in Figure 3C, the average evaporation rates of the various CMs under the solar illumination of 1 sun were measured to be 0.924 (CAPs), 0.959 (CCPs), and 0.722 (CCWs) kg·m−2·h−1, respectively, which were about 1.7~2.2 times compared with those of the pure water (0.431 kg·m−2·h−1), APs (0.440 kg·m−2·h−1), CPs (0.438 kg·m−2·h−1), and CWs (0.440 kg·m−2·h−1) (Table 1, Supplementary Table S1). In particular, the evaporation rates of the CCPs were higher than all other CMs. Such high evaporation rates of CCPs could be attributed to multiple advantages such as strong absorption (low reflectance) due to the carbonization, rapid transport of vapor/liquid because of the porous structure, and restricted thermal diffusion ascribed to low thermal conductivity (Liu et al., 2015c; Hu et al., 2017; Lin et al., 2018).
Table 1. The water evaporation rates (ṁ) of various samples and the calculation results of the energy conversion efficiency (ηECE).
The strong light absorption is one of the most important conditions for the light-to-heat conversion which could be measured by the UV-Vis diffuse reflectance spectra (DRS). As illustrated in Figure 3D and Supplementary Table S2, the reflectance of the APs, CPs and CWs reached up to 65.44, 53.03, and 70.33%, respectively, consistent with the low surface temperature under irradiation. After the carbonization, the reflectance of CAPs, CCPs, and CCWs dramatically decreased to 8.38, 7.34, and 27.57%, respectively. In addition, the transmittance of all the samples were < ca. 2.5% (see Supplementary Figure S3), which confirmed the strong absorption (70.86~92.20%, Supplementary Table S2) of the CMs. In particular, the CCPs has obtained the highest absorption of 92.20% compared with other CMs and Au-APs(84.06%), corresponding to the highest evaporation rates of CCPs among all the samples.
Moreover, the highly efficient solar steam generation of CMs depended on the temperature of the interface between liquid and vapor, which was considered as one of the most important factors for the water evaporation (Sartori, 2000; Al-Shammiri, 2002). In order to further explore the temperature of the interface affected by the CMs, an IR camera was used to monitor the interfacial temperature vs. time during the solar steam generation (Figure 4). After 1 h illumination of 1 sun, the CMs reached the temperature of 40.3°C (CAPs), 41.2°C (CCPs), and 37.5°C (CCWs), respectively, which showed an increase than those of pure water (29.3°C) and the fiber products (29.7~30.0°C). Especially, the temperature of the CCPs and CAPs was the higher than the Au-APs, supporting their better performance in the water evaporation tests than that of the Au-APs. Meanwhile, the real-temperature changes vs. time for the CCPs under 1 sun showed in Supplementary Figure S4A, which certificated a sharper increase than those of pure water and the CPs.
Figure 4. The IR images of temperature changes for the pure water, Au-APs, APs, CAPs, CPs, CCPs, CWs, and CCWs (with water immersion) under 1 sun illumination within 1 h.
After carbonization, the component of the CMs would be changed, which could be confirmed by the elemental analysis (EA, see Supplementary Table S3). Very few contents of the trace metals in the CMs were also tested by the inductively coupled plasma atomic emission spectrometry (ICP-AES, Supplementary Table S4). With the variation of the carbonized degree and the oxidation degree, the decrease of the thermal conductivity of the CMs should probably be attributed to the increase of the C-C (the specra of the C 1s, Supplementary Figure S6), the increase of the O-C and the decrease of the -OH (the specra of the O 1s, Supplementary Figure S7) after carbonization, which could be verified by and X-ray photoelectron spectroscopy (XPS, see Supplementary Figures S5–S7). The thermal conductivities (Supplementary Table S1) of the CMs (0.031~0.058 W·m−1·K−1) were lower than the fiber products (0.119~0.150 W·m−1·K−1), Au-APs (0.49 W·m−1·K−1) (Liu et al., 2015c), and pure water (0.56 W·m−1·K−1) (Liu et al., 2015c). Compared with other CMs (see Supplementary Table S1), the CCPs have the lower thermal conductivity (0.031 W·m−1·K−1), which would be beneficial to restrict the thermal diffusion, leading to the remarkable performance boost of CCPs for solar steam generation.
Based on the aforementioned discussion, the CCPs have shown optimal water evaporation rates among the CMs ascribed to their lower thermal conductivity and better light absorption. In order to evaluate the solar steam generation performance more accurately, the energy conversion efficiency (ηECE) was calculated via the following equation:
where ṁ is the mass flux, hLV is the total enthalpy of the liquid–vapor phase change, P0 is the normal solar illumination of one sun (1 kW·m−2), and CoptP0 refers to the illumination intensity on the absorbers surface (Zhou et al., 2016c). In particular, the calculation of hLV was based on the sensible heat and the temperature-dependent enthalpy of vaporization (see in Supplementary Table S1, Liu et al., 2017). The calculated ηECE of solar steam generations under corresponding solar illumination was shown in Figure 5A with error bars. The ηECE of CCPs (red circular points) was calculated to be 65.8% under 1 sun illumination, as the best efficiency when compared with other samples (Table 1, Supplementary Table S1).
Figure 5. (A) The average evaporation rates (left-hand side axis) with pure water, Au-APs, CAPs, CCPs, and CCWs (black square points) under the solar illumination of 1 sun, together with the corresponding ηECE of pure water, Au-APs, CAPs, CCPs, and CCWs (red circular points, right-hand side axis). (B) The recyclability of CCPs under 1 sun illumination by recycling 20 times. Each cycle was tested for 1 h.
Due to the importance of the solar intensity fluctuates as a feature for solar steam generation, as shown in Supplementary Figure S4B, the average evaporation rates of the CCPs under the various solar illumination of 1, 2, 3, 4, and 6 sun have been additionally investigated, corresponding to 0.959, 1.877, 2.843, 3.801, and 5.712 kg·m−2·h−1, respectively. They were ca. 2.1 times in contrast with those of the pure water (corresponded to 0.431, 0.883, 1.267, 1.740, and 2.684 kg·m−2·h−1, respectively). In order to obtain better performance of the CCPs, the influence of the CMs' thickness (CCPs with different layers) has been explored (Supplementary Figure S4C), which showed that the two-layers CCPs (CCPs-2 layers, 1.057 kg·m−2·h−1) has achieved the best water evaporation rates compared with the single-layers and three-layers CCPs (CCPs-1 layers, 0.954 kg·m−2·h−1and CCPs-3 layers 0.988 kg·m−2·h−1). With the increase of the CCPs' thickness, the water evaporation rates of the CCPs first raised and then decreased a bit, but the results still indicated an enhancement via adding the CCPs' layers. In addition, the recyclability of the solar absorbers was important aspect for efficient solar steam generation. As shown in Figure 5B, the recyclability of the CCPs was investigated (Copt = 1, at 25°C and humidity of 50%) that no significant attenuation in evaporation rates was observed for 20 cycles.
In conclusion, we have demonstrated the use of free-standing porous carbonized-membranes (CMs) derived from the commercial fiber products by one-step carbonization as independent solar absorbers for highly efficient solar steam generation. Our proposed CMs featuring strong absorption, porous structure and low thermal conductivity have shown better evaporation performance compared with the pure water and corresponding original fiber products. As a result, the optimized CMs derived from carbonized cellulose papers (CCPs), achieve the best water evaporation rate of 0.959 kg·m−2·h−1 and the energy conversion efficiency of 65.8% under 1 kW·m−2 solar illumination, outperforming another CMs and plasmonic airlaid paper (Au-APs). Therefore, the CMs, especially the CCPs, hold great promise as independent solar absorbers for the large-scale application of solar steam generation.
XL wrote the paper. XL and MY carried out the experiments. DY, WH, and XC not only designed the experiments, but also reviewed and revised the paper. All the authors joined discussion about the paper.
The authors declare that the research was conducted in the absence of any commercial or financial relationships that could be construed as a potential conflict of interest.
This work was supported by the National Natural Science Foundation of China (Grant No. 51573214; 51833011), Guangdong YangFan Innovative & Entepreneurial Research Team Program (Project No. 201633002) and the Youth 1000 Talent Program of China.
The Supplementary Material for this article can be found online at: https://www.frontiersin.org/articles/10.3389/fmats.2018.00074/full#supplementary-material
Al-Shammiri, M. (2002). Evaporation rate as a function of water salinity. Desalination 150, 189–203. doi: 10.1016/S0011-9164(02)00943-8
Bae, K., Kang, G., Cho, S. K., Park, W., Kim, K., and Padilla, W. J. (2015). Flexible thin-film black gold membranes with ultrabroadband plasmonic nanofocusing for efficient solar vapour generation. Nat. Commun. 6:10103. doi: 10.1038/ncomms10103
El-Agouz, S. A., El-Aziz, G. A., and Awad, A. M. (2014). Solar desalination system using spray evaporation. Energy 76, 276–283. doi: 10.1016/j.energy.2014.08.009
Elimelech, M., and Phillip, W. A. (2011). the future of seawater desalination: energy, technology, and the environment. Science 333, 712–717. doi: 10.1126/science.1200488
Ghasemi, H., Ni, G., Marconnet, A. M., Loomis, J., Yerci, S., Miljkovic, N., et al. (2014). Solar steam generation by heat localization. Nat. Commun. 5:4449. doi: 10.1038/ncomms5449
Hu, X., Xu, W., Zhou, L., Tan, Y., Wang, Y., Zhu, S., et al. (2017). Tailoring graphene oxide based aerogels for efficient solar steam generation under one sun. Adv. Mater. 29:1604031. doi: 10.1002/adma.201604031
Idicula, M., Boudenne, A., Umadevi, L., Ibos, L., Candau, Y., and Thomas, S. (2006). Thermophysical properties of natural fibre reinforced polyester composites. Compos. Sci. Technol. 66, 2719–2725. doi: 10.1016/j.compscitech.2006.03.007
Ito, Y., Tanabe, Y., Han, J., Fujita, T., Tanigaki, K., and Chen, M. (2015). Multifunctional porous graphene for high-efficiency steam generation by heat localization. Adv. Mater. 27, 4302–4307. doi: 10.1002/adma.201501832
Karagiannis, I. C., and Soldatos, P. G. (2008). Water desalination cost literature: review and assessment. Desalination 223, 448–456. doi: 10.1016/j.desal.2007.02.071
Lenert, A., and Wang, E. N. (2012). Optimization of nanofluid volumetric receivers for solar thermal energy conversion. Sol. Energy 86, 253–265. doi: 10.1016/j.solener.2011.09.029
Lewis, N. S. (2016). Research opportunities to advance solar energy utilization. Science 351:aad1920. doi: 10.1126/science.aad1920
Lin, X., Chen, J., Yuan, Z., Yang, M., Chen, G., Yu, D., et al. (2018). Integrative solar absorbers for highly efficient solar steam generation. J. Mater. Chem. A 6, 4642–4648. doi: 10.1039/C7TA08256H
Liu, Y., Chen, J., Guo, D., Cao, M., and Jiang, L. (2015a). Floatable, self-cleaning, and carbon-black-based superhydrophobic gauze for the solar evaporation enhancement at the air-water interface. ACS Appl. Mater. Interfaces 7, 13645–13652. doi: 10.1021/acsami.5b03435
Liu, Y., Lou, J., Ni, M., Song, C., Wu, J., Dasgupta, N. P., et al. (2015b). Bioinspired bifunctional membrane for efficient clean water generation. ACS Appl. Mater. Interfaces 8, 772–779. doi: 10.1021/acsami.5b09996
Liu, Y., Yu, S., Feng, R., Bernard, A., Liu, Y., Zhang, Y., et al. (2015c). A bioinspired, reusable, paper-based system for high-performance large-scale evaporation. Adv. Mater. 27, 2768–2774. doi: 10.1002/adma.201500135
Liu, Z., Song, H., Ji, D., Li, C., Cheney, A., Liu, Y., et al. (2017). Extremely cost effective and efficient solar vapor generation under nonconcentrated illumination using thermally isolated black paper. Glob. Challenge 1:1600003. doi: 10.1002/gch2.201600003
Lou, J., Liu, Y., Wang, Z., Zhao, D., Song, C., Wu, J., et al. (2016). Bioinspired multifunctional paper-based rGO composites for solar-driven clean water generation. ACS Appl. Mater. Interfaces 8, 14628–14636. doi: 10.1021/acsami.6b04606
Ni, G., Li, G., Boriskina, S. V., Li, H., Yang, W., Zhang, T., et al. (2016). Steam generation under one sun enabled by a floating structure with thermal concentration. Nat. Energy 1:16126. doi: 10.1038/nenergy.2016.126
Nyholm, L., Nyström, G., Mihranyan, A., and Strømme, M. (2011). Toward flexible polymer and paper-based energy storage devices. Adv. Mater. 23, 3751–3769. doi: 10.1002/adma.201004134
Sartori, E. (2000). A critical review on equations employed for the calculation of the evaporation rate from free water surfaces. Sol. Energy 68, 77–89. doi: 10.1016/S0038-092X(99)00054-7
Schiermeier, Q. (2008). Water: purification with a pinch of salt. Nature 452, 260–261. doi: 10.1038/452260a
Shannon, M. A., Bohn, P. W., Elimelech, M., Georgiadis, J. G., Mariñas, B. J., and Mayes, A. M. (2008). Science and technology for water purification in the coming decades. Nature 452, 301–310. doi: 10.1038/nature06599
Wang, G., Fu, Y., Ma, X., Pi, W., Liu, D., and Wang, X. (2017a). Reusable reduced graphene oxide based double-layer system modified by polyethylenimine for solar steam generation. Carbon 114, 117–124. doi: 10.1016/j.carbon.2016.11.071
Wang, X., He, Y., Liu, X., Cheng, G., and Zhu, J. (2017b). Solar steam generation through bio-inspired interface heating of broadband-absorbing plasmonic membranes. Appl. Energy 195, 414–425. doi: 10.1016/j.apenergy.2017.03.080
Wang, Z., Liu, Y., Tao, P., Shen, Q., Yi, N., Zhang, F., et al. (2014). Bio-inspired evaporation through plasmonic film of nanoparticles at the air–water interface. Small 10, 3234–3239. doi: 10.1002/smll.201401071
Xu, Y., Zhu, Y., Han, F., Luo, C., and Wang, C. (2015). 3D Si/C fiber paper electrodes fabricated using a combined electrospray/electrospinning technique for Li-ion batteries. Adv. Energy Mater. 5:1400753. doi: 10.1002/aenm.201400753
Zhou, J., Sun, Z., Chen, M., Wang, J., Qiao, W., Long, D., et al. (2016a). Macroscopic and mechanically robust hollow carbon spheres with superior oil adsorption and light-to-heat evaporation properties. Adv. Funct. Mater. 26, 5368–5375. doi: 10.1002/adfm.201600564
Zhou, L., Tan, Y., Ji, D., Zhu, B., Zhang, P., Xu, J., et al. (2016b). Self-assembly of highly efficient, broadband plasmonic absorbers for solar steam generation. Sci. Adv. 2:e1501227. doi: 10.1126/sciadv.1501227
Zhou, L., Tan, Y., Wang, J., Xu, W., Yuan, Y., Cai, W., et al. (2016c). 3D self-assembly of aluminium nanoparticles for plasmon-enhanced solar desalination. Nat. Photonics 10, 393–398. doi: 10.1038/nphoton.2016.75
Zhou, L., Zhuang, S., He, C., Tan, Y., Wang, Z., and Zhu, J. (2017). Self-assembled spectrum selective plasmonic absorbers with tunable bandwidth for solar energy conversion. Nano Energy 32, 195–200. doi: 10.1016/j.nanoen.2016.12.031
Keywords: solar absorber, solar steam generation, porous membrane, fiber products, photothermal conversion
Citation: Lin X, Yang M, Hong W, Yu D and Chen X (2018) Commercial Fiber Products Derived Free-Standing Porous Carbonized-Membranes for Highly Efficient Solar Steam Generation. Front. Mater. 5:74. doi: 10.3389/fmats.2018.00074
Received: 04 October 2018; Accepted: 27 November 2018;
Published: 14 December 2018.
Edited by:
Dong Wook Chang, Pukyong National University, South KoreaReviewed by:
Dong Ryeol Whang, Johannes Kepler University of Linz, AustriaCopyright © 2018 Lin, Yang, Hong, Yu and Chen. This is an open-access article distributed under the terms of the Creative Commons Attribution License (CC BY). The use, distribution or reproduction in other forums is permitted, provided the original author(s) and the copyright owner(s) are credited and that the original publication in this journal is cited, in accordance with accepted academic practice. No use, distribution or reproduction is permitted which does not comply with these terms.
*Correspondence: Dingshan Yu, eXVkaW5nc0BtYWlsLnN5c3UuZWR1LmNu
Wei Hong, aG9uZ3dlaTlAbWFpbC5zeXN1LmVkdS5jbg==
Xudong Chen, Y2VzY3hkQG1haWwuc3lzdS5lZHUuY24=
Disclaimer: All claims expressed in this article are solely those of the authors and do not necessarily represent those of their affiliated organizations, or those of the publisher, the editors and the reviewers. Any product that may be evaluated in this article or claim that may be made by its manufacturer is not guaranteed or endorsed by the publisher.
Research integrity at Frontiers
Learn more about the work of our research integrity team to safeguard the quality of each article we publish.