- Institute of Materials Research and Engineering (IMRE), Agency for Science, Technology and Research (A*STAR), Innovis, Singapore, Singapore
In recent years, two-dimensional (2D) graphitic carbon nitride (g-C3N4) has elicited interdisciplinary research fascination among the scientific communities due to its attractive properties such as appropriate band structures, visible-light absorption, and high chemical and thermal stability. At present, research aiming at engineering 2D g-C3N4 photocatalysts at an atomic and molecular level in conquering the global energy demand and environmental pollution has been thriving. In this review, the cutting-edge research progress on the 2D/2D g-C3N4-based hybrid nanoarchitectures will be systematically highlighted with a specific emphasis on a multitude of photocatalytic applications, not only in waste degradation for pollution alleviation, but also in renewable energy production [e.g., water splitting and carbon dioxide (CO2) reduction]. By reviewing the substantial developments on this hot research platform, it is envisioned that the review will shed light and pave a new prospect for constructing high photocatalytic performance of 2D/2D g-C3N4-based system, which could also be extended to other related energy fields, namely solar cells, supercapacitors, and electrocatalysis.
Introduction
Photocatalysis is emerged as one of the Holy Grails of sustainable and green technologies for solar energy conversion, energy storage, and environmental remediation, which has been intensively examined over the past few decades worldwide to search for novel photocatalysts (Inoue et al., 1979; Linsebigler et al., 1995; Ma et al., 2014; Ong et al., 2014a, 2016b; He and Que, 2016; Li et al., 2016a; Wenderich and Mul, 2016; Zhang et al., 2016a; Eftekhari, 2017; Liu et al., 2017; Osterloh, 2017; Roger et al., 2017). By harvesting solar energy as the source of renewable energy, photocatalysis will make significant impacts in the areas of (1) light-driven water splitting to hydrogen (H2) and oxygen (O2) (Chen et al., 2010; Bai et al., 2016; Wei et al., 2016; Putri et al., 2017; Yubin et al., 2017), (2) conversion of carbon dioxide (CO2) to energy bearing fuels (Ong et al., 2013, 2014c; Tan et al., 2014, 2016, 2017; Gui et al., 2015; Guo et al., 2016a; Zhang et al., 2016c), (3) mineralization of waste and pollutants (Ong et al., 2014d,e; Fang et al., 2016; Liu et al., 2016c; Topcu et al., 2016; Zhao et al., 2016b), (4) selective organic transformations (Liu et al., 2014; Zhao et al., 2016a), and (5) disinfection of bacteria (Keane et al., 2014; Bing et al., 2015) (Figure 1). Very recently, two-dimensional (2D) semiconductor photocatalysts have triggered a renaissance of interest in the field of energy, and environmental-related applications thank to the high ratio of surface-to-volume and unprecedented electronic and optical characteristics (Ong et al., 2014b; Bai et al., 2015; Liang et al., 2015d; Fang et al., 2016; Kalantar-zadeh et al., 2016; She et al., 2017; Xueting et al., 2017). Among a large array of photocatalysts, research targeting at graphitic carbon nitride (g-C3N4) has been flourishing in recent years. Since the first exploratory study on the use of g-C3N4 in photocatalytic H2 evolution in 2009 (Wang et al., 2009), there has been an exponential increase in the scientific research on the subject of g-C3N4-based materials with more than 800 publications in 2016 based on Web of Science.
By and large, g-C3N4 can be facilely prepared by nitrogen-rich precursors, namely urea, thiourea, melamine, and dicyandiamide (Han et al., 2015; Guo et al., 2016b; Zhou et al., 2016; Tong et al., 2017). Therefore, the development of g-C3N4-based photocatalysts is anticipated to surmount the issues of increasing concerns on fossil fuel depletion and environmental threats due to combustion of exhaustible fossil fuels. The metal-free g-C3N4 demonstrates distinctive attributes such as visible-light responsiveness with moderate band gap of ca. 2.7 eV, appealing band structures and electronic characteristic, its earth-abundant nature, non-toxicity, relative ease of synthesis, and excellent chemical stability (Lu et al., 2016; Ong et al., 2016b; Zhang et al., 2016b; Lee et al., 2017). Additionally, it has been proven that 2D semiconductor possessed improved mobility of charge carriers and reduced charge recombination as compared to the 0D and 1D nanomaterials (Meng et al., 2012; Ida and Ishihara, 2014). In spite of the fascinating properties possessed by 2D g-C3N4, pristine g-C3N4 demonstrated several shortfalls such as sluggish separation of electron–hole pairs, limited visible-light absorption beyond 460 nm, small specific surface area, and low electrical conductivity (Liang et al., 2015b; Hou et al., 2016; Shi et al., 2016; Zhang et al., 2016g; Li et al., 2017; Xia et al., 2017). To overcome these bottlenecks, modification of bare g-C3N4 such as nanostructure design (Niu et al., 2012; Liang et al., 2015c; Zheng et al., 2015), intercalation with Li+ and Cl− (Liang et al., 2015a), elemental doping (Hu et al., 2015; Huang et al., 2015; She et al., 2016), copolymerization (Fan et al., 2016; Rahman et al., 2016), coupling with metals or noble metals (Tonda et al., 2014; Ong et al., 2015b), incorporation with other semiconductors (Ong et al., 2016a; Putri et al., 2016a; Zhang et al., 2016g; Ye et al., 2017), hybridization with metal phosphides (Pan et al., 2017; Wen et al., 2017; Yi et al., 2017; Zhao et al., 2017a,b), and many more has been widely investigated to enhance the photocatalytic efficiency for practical benefits. To date, there are a number of excellent review articles highlighting on g-C3N4-based photocatalysts ranging from materials synthesis, functionalization, and hybridization to diversified applications (Cao et al., 2015; Dong and Cheng, 2015; Yin et al., 2015; Zhang et al., 2015a; Zhao et al., 2015; Liu et al., 2016a; Mamba and Mishra, 2016). This undoubtedly connotes the significance of this research field hitherto in the scientific community.
Recently, the incorporation of 2D g-C3N4 photocatalyst with other 2D nanomaterials forming 2D/2D heterojunction hybrid nanocomposites has conceivably drawn increasing attention with practical importance (Hou et al., 2014; Xing et al., 2017). As a matter of fact, the layered heterojunction comprised dissimilar 2D nanomaterials is projected to give rise to positive impacts on charge transfer and separation as a result of built-in electric field at the atomically well-defined ultrathin interface (Hou et al., 2013b; Lu et al., 2016). Thus, the research on 2D/2D heterojunction with intimate face-to-face interface is of timely significance, which will elucidate us to deeply comprehend the photocatalytic reaction mechanism at the molecular level. Therefore, in this review, this leads to my immense interest to summarize the state-of-the-art research on 2D/2D g-C3N4 heterojunction nanohybrids to throw light on the future research horizon of g-C3N4 in artificial photosynthesis and environmental remediation.
Development of 2D/2D g-C3N4-Based Heterojunction
It is well documented that 2D/2D nanocomposites bestow greater electron–hole mobility across the heterojunction interface, which will in turn reduce the distance and time of charge transport to impede the electron–hole recombination rate (Hou et al., 2013a; Cheng et al., 2015; Ong et al., 2015c). This is attributed to the larger 2D/2D face-to-face contact area compared with line-to-face contact in 1D/2D heterojunction and point-to-face contact in 0D/2D heterojunction as depicted in Figure 2.
Hybridization with 2D Transition Metal Chalcogenides
In recent years, the incorporation of 2D metal sulfides has underpinned enormous interests in photocatalysis (Liu et al., 2016b; Lu et al., 2016; Yu and Sivula, 2016). In a work by Dong’s research group, they reported a hierarchical sheet-on-sheet ZnIn2S4/g-C3N4 heterostructure by growing ultrathin ZnIn2S4 onto g-C3N4 nanosheets (Zhang et al., 2016e). As a result of intimate heterojunction interface formed between ZnIn2S4 and g-C3N4, the hybrid nanocomposites demonstrated walloping 17.6- and 3.9-folds enhancement of H2 evolution compared to the single component g-C3N4 and ZnIn2S4, respectively. From the perspective of lifetime of charge carriers evidenced from time-resolved photoluminescence analysis, the average lifetime of the ZnIn2S4/g-C3N4 nanohybrids was reduced from 10.45 to 8.97 ns relative to that of pristine g-C3N4 nanosheets, which was attributed to rapid charge transfer and separation to hinder the electron–hole recombination. In addition to ZnIn2S4, MoS2-decorated S-doped g-C3N4 heterojunction films were successfully developed by Chen and coworkers (Figure 3A) for enhanced photoelectrocatalysis (Ye et al., 2016). It is noted that the generation of anodic current by the MoS2/S-doped g-C3N4 photoanode was markedly twice than that by the S-doped g-C3N4, highlighting the rational importance of a robust heterointerface with intact p–n junctions for effective charge migration (Figure 3B).
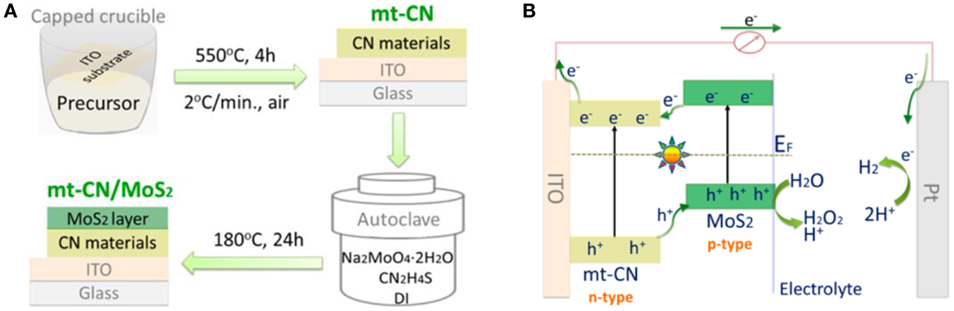
Figure 3. (A) Synthesis route of MoS2/S-doped g-C3N4 heterojunction film on ITO glass. (B) Charge transfer and separation of MoS2/S-doped g-C3N4 photoanode via p–n heterojunctions for the improved H2 generation. Reproduced from Ye et al. (2016) with permission from the American Chemical Society.
Till now, the theoretical understanding on the coupling interaction and transfer of charge carriers between 2D g-C3N4 and 2D MoS2 has not been exhaustively investigated. Wang et al. (2014) elucidated the fundamental mechanism of photocatalytic improvements by systematically exploring the interface region between MoS2 and g-C3N4. Based on the density functional theory (DFT) calculations, it was confirmed the presence of charge redistribution at the 2D/2D heterojunction interface of MoS2/g-C3N4. It is worth mentioning that a type II heterojunction structure was successfully developed due to the well-matched band alignment as attested by the density of states results. As a result of efficient migration of charge carriers, a polarized field was formed at the contact heterointerface, prohibiting the electron–hole recombination. Therefore, this DFT finding provides new inroads into the importance of constructing 2D/2D nanocomposites for face-to-face interaction, which could certainly be extended to other binary or even ternary layered heterojunction for enhanced photochemistry applications.
Hybridization with 2D Metal Oxides
Apart from 2D transition metal chalcogenides, coupling g-C3N4 nanosheets with 2D metal oxides, such as TiO2 (Gu et al., 2014), WO3 (Li et al., 2016b), and SnSb2O6 (Zhang et al., 2016d), has become the recent research focus to improve the photocatalytic performance. For instance, Li et al. (2016b) employed a hydrothermal and deposition-heating technique to fabricate WO3/g-C3N4 nanosheet arrays on the FTO substrates. The photoelectrochemical splitting of natural seawater using the WO3/g-C3N4 nanostructures presented two times greater in the photocurrent density in reference to the pristine WO3 nanosheet arrays under the simulated sunlight source. This was accredited to the well-matched band energy of WO3 and g-C3N4 forming synergistic interfacial contacts for remarkably boosting the charge migration ability. In another work, Xing’s group hybridized AgIO3 with anisotropic g-C3N4 nanosheets to form a 2D/2D layered heterointerface toward increased photodegradation of Rhodamine B and methyl orange pollutants (Li et al., 2015). It is anticipated that these works will lay a pioneer groundwork and shed light for future directions in the interface engineering of 2D metal oxides and g-C3N4 for the advancement in solar energy conversion and environmental remediation toward practical applications.
Most recently, perovskite-type nitrogen-doped La2Ti2O7 (NLTO), comprising a 2D architecture with a thickness of 7 nm, was for the first time hybridized with 2 nm thick g-C3N4 nanosheets by means of a facile two-step hydrothermal method and a thermal treatment process (Figure 4A) (Cai et al., 2017). The hybrid layered nanomaterials showed excellent photocatalytic H2 evolution with a high apparent quantum efficiency of 2.1% at 400 nm. The enhanced photoactivity was ascribed to the successful development of the large 2D/2D interface between NLTO and g-C3N4, resulting in long lifetime and favorable transfer of charge carriers via type II band alignment. Upon visible-light illumination, the photoexcited electrons were facilely migrated from g-C3N4 to NLTO, whereas the photogenerated holes were transported from NLTO to g-C3N4, hampering the charge recombination process (Figure 4B). Notably, this was arisen from the band bending formed at the heterointerface, which induced a built-in electric field for the flow of charge carriers.
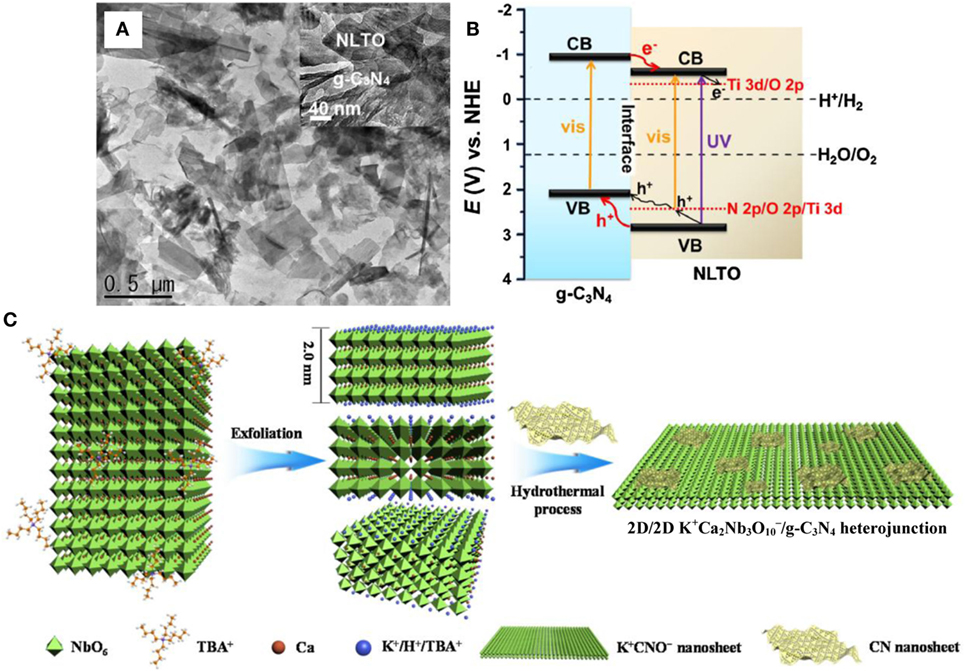
Figure 4. (A) Transmission electron microscopy image of NLTO/g-C3N4 hybrid photocatalysts. (B) Schematic of effective transfer of photogenerated electrons and holes across the heterojunction interface of NLTO/g-C3N4 through a type II band alignment. Reproduced from Cai et al. (2017) with permission from Elsevier. (C) Synthesis procedure of 2D/2D -layered nanohybrids. Reproduced from Jiang et al. (2017) with permission from Elsevier.
Furthermore, 2D niobium phase-layered perovskite Dion–Jacobson compounds have become a hot focal field in materials science and engineering for clean energy production and environmental cleaning in the past few years (Maeda et al., 2014; Oshima et al., 2016). It is well known that the layered perovskite nanosheets, emerging from NbO6 octahedra building blocks, are promising owing to their good chemical stability, high surface area, and relatively cost-effective (Sabio et al., 2010). Therefore, it is expected that by integrating the idea of nanosheets into 2D g-C3N4 photocatalysts from the viewpoint of materials design, the photocatalytic efficiency of the composite nanomaterials will be exhilaratingly elevated. In a very recent work published in 2017, Chen’s group designed a visible-light-responsive 2D/2D nanosheet heterojunction, which was fabricated by a hydrothermal process (Figure 4C), for photodegradation of tetracycline hydrochloride (Jiang et al., 2017). The hybrid photocatalyst manifested a strikingly high activity, which was 6.6 and 1.8 times greater compared with that of pure and g-C3N4, respectively, due to the well-contacted heterointerfaces with strong interfacial coupling. Similarly, another group of researchers has synthesized the nanosheet composites by combining Ca2Nb2TaO10 and g-C3N4 nanosheets through a simple solution exfoliation-reassembly technique for solar H2 production (Thaweesak et al., 2017). As such, it is believed that this research will cast new opportunities for engineering 2D/2D perovskite-based nanosheets coupled with g-C3N4 heterojunction interface for multitudinous light-driven applications.
Hybridization with 2D Graphene
At present, π-conjugated carbonaceous nanomaterials, including carbon nanotubes, grapheme, and carbon nanodots has emerged as one of the most fascinating and exciting research directions in the past 10 years especially in the arena of environmental remediation, energy conversion, and energy storage (Tan et al., 2013; Cazorla-Amorós, 2014; Li et al., 2014; Cao and Wei, 2015; Fan, 2015; Ali Tahir et al., 2016; Carmona et al., 2016; Kotal et al., 2016; Xu et al., 2016). These carbon nanostructures have been commonly utilized as excellent reduction cocatalysts by incorporating with semiconductor photocatalysts to prolong the lifetime of charge carriers to diminish the recombination of electron–hole pairs (Xu et al., 2013; Himaja et al., 2015; Liu et al., 2015; Tan et al., 2015a; Hu, 2016; Zhang et al., 2016f; Ong et al., 2017). It is discernable that the 2D graphene has directed a worldwide trend in the materials research stemming from its large surface area, remarkable electronic, optical and mechanical features, and high chemical stability (Putri et al., 2015, 2016b; Voon et al., 2016; Xiang et al., 2016). Up to now, a plethora of literature reports has been devoted to fabricate 2D/2D graphene/g-C3N4 nanohybrids for photoredox catalysis in H2 evolution, pollutant degradation, and CO2 reduction (Xiang et al., 2011; Li et al., 2013; Xu et al., 2015; Wan et al., 2016). In a work by Ong et al. (2015a), sandwich-like graphene/g-C3N4 nanocomposites were prepared via a one-step impregnation-thermal reduction process by employing graphene oxide and urea as the precursors (Figure 5A). Interestingly, the absorption band edge of the nanohybrids was slightly red shifted toward a longer wavelength, resulting in a reduction in the band gap energy. This phenomenon was contributed by the covalent cross linker (C–O–C) formed between g-C3N4 and graphene as a result of thermal heating at the high temperature. For the first time, the metal-free graphene/g-C3N4 photocatalyst played a prominent role in the reduction of CO2 to CH4 under visible light, which was 2.3 times higher than pristine g-C3N4. In this sense, this study incontrovertibly focuses the spotlight on the innovative design of metal-free layered photocatalysts as a new class of light-active materials for a cornucopia of catalytic applications.
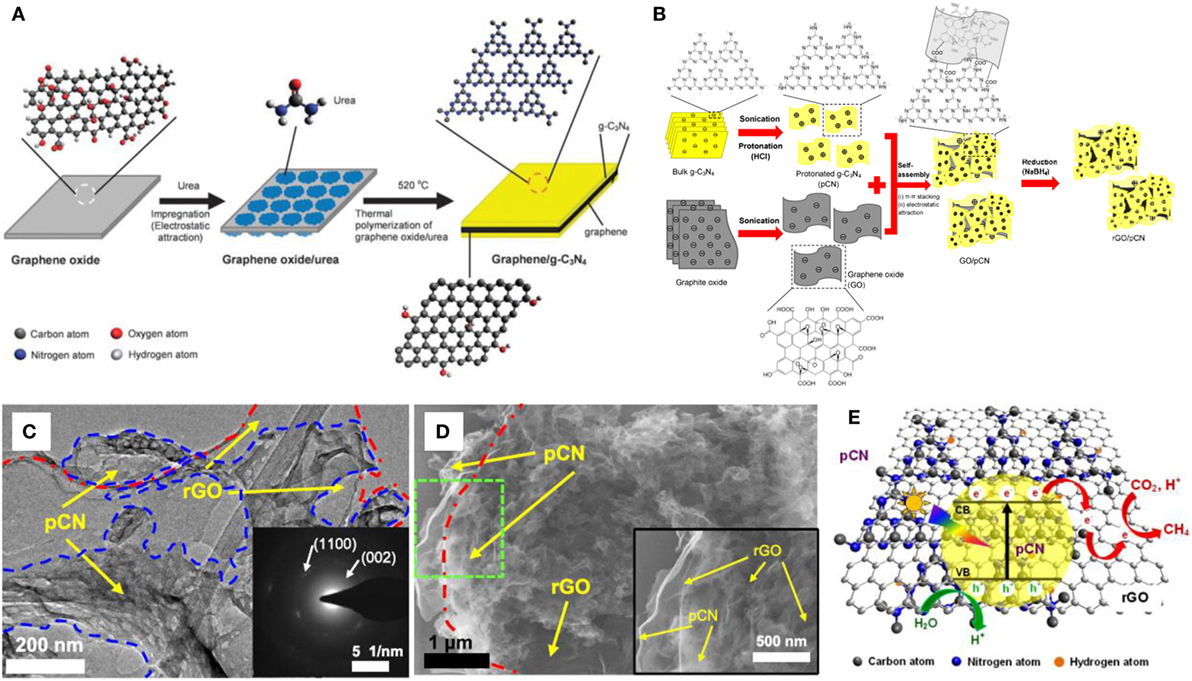
Figure 5. (A) Fabrication of graphene/g-C3N4 photocatalysts by means of a facile impregnation-thermal reduction process. Reproduced from Ong et al. (2015a) with permission from Royal Society of Chemistry. (B) Schematic of reduced graphene oxide (rGO)/pCN samples developed by a sonication-aided electrostatic attraction and π–π interaction process. (C) Transmission electron microscopy and (D) scanning electron microscopy images of rGO/pCN samples. Inset of panel (C) is the selected area electron diffraction of rGO/pCN. (E) Reaction mechanism of photoreduction of CO2 with H2O over rGO/pCN samples. Reproduced from Ong et al. (2015c) with permission from Elsevier.
In another closely related work by the similar research group, the novel 2D/2D-reduced graphene oxide (rGO)-hybridized protonated g-C3N4 (rGO/pCN) was rationally constructed by π–π stacking and electrostatic self-assembly between the positively charged pCN and the negatively charged rGO (Figure 5B) (Ong et al., 2015c). In the rGO/pCN hybrid nanoarchitectures, a well-dispersed sheet-on-sheet structure of rGO and pCN confirmed the well-intact interfacial contact (Figures 5C,D) as compared to the rGO/g-C3N4, which employed the unmodified g-C3N4 with a negatively charged surface. Essentially, the rGO/pCN heterojunction nanosheets endowed pronounced 5.4 and 1.7 times enhancement in the photoconversion of CO2 to CH4 with respect to the pCN and rGO/g-C3N4, respectively. Thus, this distinctly underlines the fundamental and technological importance of surface charge modification between two dissimilar 2D nanomaterials for robust interfacial interactions in unraveling the charge dynamics for enhanced photocatalysis. Benefiting from the predominant role of graphene as the electron reservoir (Zhang et al., 2012, 2015b; Tan et al., 2015b; Mateo et al., 2016; Varadwaj and Nyamori, 2016), the photoinduced electrons were transferred from pCN to rGO across the interface to overwhelmingly suppress the charge recombination rate (Figure 5E). All in all, the smart 2D/2D interface engineering design of graphene/g-C3N4 developed thus far is considered as an auspicious means, which could be eminently extended to heteroatom-doping graphene-hybridized g-C3N4 for targeting superior photochemistry applications for real-life applications.
Conclusion and Outlook
In short, the burgeoning developments of nanoscale architecturing of g-C3N4-based hybrid structures over the past 8 years have witnessed a wealth of knowledge and information for the intelligent design and myriads of applications in sustainable energy conversion and environmental purification. The applications, which encompass water splitting, H2 generation, O2 evolution, CO2 fixation, and pollutant degradation, have readily made full use of the intriguing features of g-C3N4, namely metal-free 2D nanomaterials, earth-abundant nature of the elements, visible-light optical absorption, high redox power, and excellent chemical stability. Since the advent of g-C3N4 photocatalysts by Wang et al. (2009) for H2 generation, there is a rocket rise of research works on the modification of bare g-C3N4 to conspicuously increase the specific surface area, introduce porosity by textural modifications, extend visible-light absorption to longer wavelengths (even up to near infra-red) for the utilization of whole solar spectrum, decrease the band gap energy and bolster the charge migration and separation.
In this mini-review, a systematic discussion on the most updated advancements of engineering 2D/2D g-C3N4 heterojunction layered nanoarchitectures with boosted photoactivity has been reviewed. It is worth mentioning that albeit there is a large library of recent research discoveries on the 2D/2D g-C3N4-based photocatalysts, there exist numerous open issues, limitations, questions, and complexities of materials science, chemistry, physics, and environmental science, which require extensive research now and future. Among all, the actual mechanisms of enhanced catalytic efficiency toward the water splitting and CO2 reduction followed by their respective reaction pathways are still up in the air yet until now. It is envisaged that the pertinent mechanism underlying the photocatalytic performance should be deeply explored by joining the experimental findings and theoretical computational simulations for the future research. In this manner, the rationale behind the profound photocatalytic enhancement especially on the rate determining steps of the reaction in the 2D/2D nanohybrids can be entirely comprehended. Apart from that, the charge carrier dynamics and transfer pathway for the Z-scheme system, p–n heterojunction, n–n heterojunction, Schottky junction, homojunction, and facet junction in the 2D/2D g-C3N4-based system will be facilely understood. Benefiting from both experimental results and first-principles DFT calculations, this will in turn provide us a rational outline to advance the state of the research on photocatalysis for the next breakthrough in the field of energy conversion.
Moreover, the coupling interaction between 2D g-C3N4 nanosheet and another 2D semiconductor is of utmost importance for developing intact heterojunction interfaces for efficient electron–hole shuttling to prolong the lifetime of charge carriers to accelerate the photocatalytic efficiency. In-depth studies in engineering, the intimate heterointerfaces of the 2D/2D nanohybrids at a molecular level will give rise to captivating results for tuning the existing molecular structure of bare g-C3N4, thereby enhancing mobility of electron–hole pairs and subsequently improving the photocatalytic redox ability. Additionally, it is crucial to attain a facile and low-cost metal-free 2D/2D g-C3N4-based photocatalyst system without comprising metal-containing semiconductors for practical benefits. Thus, continuous efforts in exploring non-metal semiconductors to couple with g-C3N4 will be advantageous for industrialization and commercialization in the long run to combat the cost concern for the large-scale processes.
To cut a long story short, it is apparent that the research progress has been tremendously impressive at this juncture by viewing at the relatively short period of time and history of g-C3N4-based photocatalysis. Undeniably, the incessant research efforts on the 2D/2D-layered nanocomposites will open new vistas and lay a strong foundation for advanced light-driven catalysis and electrocatalysis, which undeniably warrant continuous research along this direction. Without any doubts, this will act as a new paradigm for the next generation smart artificial photocatalytic systems for practical and commercial benefits in order to bridge the gap between lab-scale research and large-scale industrial applications. All in all, with the ceaseless cooperative work from all segments and disciplines in the world, the targets of building a cleaner, greener, sustainable, and zero-energy environment will be systematically accomplished in years to come. Last but not least, it is genuinely hoped that this mini-review will paint a much clearer image to direct us for the upcoming research horizons in 2D/2D photocatalysis for momentous breakthroughs in attaining highly effective, efficient, and economical g-C3N4-based system in future.
Author Contributions
W-JO carefully outlined the contents of the review and wrote the entire manuscript.
Conflict of Interest Statement
The author declares that the research was conducted in the absence of any commercial or financial relationships that could be construed as a potential conflict of interest.
Acknowledgments
This work was financially supported by the Institute of Materials Research and Engineering (IMRE), Agency for Science, Technology and Research (A*STAR) in Singapore.
References
Ali Tahir, A., Ullah, H., Sudhagar, P., Asri Mat Teridi, M., Devadoss, A., and Sundaram, S. (2016). The application of graphene and its derivatives to energy conversion, storage, and environmental and biosensing devices. Chem. Rec. 16, 1591–1634. doi: 10.1002/tcr.201500279
Bai, S., Wang, L., Chen, X., Du, J., and Xiong, Y. (2015). Chemically exfoliated metallic MoS2 nanosheets: a promising supporting co-catalyst for enhancing the photocatalytic performance of TiO2 nanocrystals. Nano Res. 8, 175–183. doi:10.1007/s12274-014-0606-9
Bai, S., Xie, M., Kong, Q., Jiang, W., Qiao, R., Li, Z., et al. (2016). Incorporation of Pd into Pt co-catalysts toward enhanced photocatalytic water splitting. Part. Part. Syst. Charact. 33, 506–511. doi:10.1002/ppsc.201500239
Bing, W., Chen, Z., Sun, H., Shi, P., Gao, N., Ren, J., et al. (2015). Visible-light-driven enhanced antibacterial and biofilm elimination activity of graphitic carbon nitride by embedded Ag nanoparticles. Nano Res. 8, 1648–1658. doi:10.1007/s12274-014-0654-1
Cai, X., Zhang, J., Fujitsuka, M., and Majima, T. (2017). Graphitic-C3N4 hybridized N-doped La2Ti2O7 two-dimensional layered composites as efficient visible-light-driven photocatalyst. Appl. Catal. B 202, 191–198. doi:10.1016/j.apcatb.2016.09.021
Cao, S., Low, J., Yu, J., and Jaroniec, M. (2015). Polymeric photocatalysts based on graphitic carbon nitride. Adv. Mater. 27, 2150–2176. doi:10.1002/adma.201500033
Cao, Z., and Wei, B. (2015). A facile route to metal oxides/single-walled carbon nanotube macrofilm nanocomposites for energy storage. Front. Mater. 2:40. doi:10.3389/fmats.2015.00040
Carmona, R. J., Velasco, L. F., Laurenti, E., Maurino, V., and Ania, C. O. (2016). Carbon materials as additives to WO3 for an enhanced conversion of simulated solar light. Front. Mater. 3:9. doi:10.3389/fmats.2016.00009
Cazorla-Amorós, D. (2014). Grand challenges in carbon-based materials research. Front. Mater. 1:6. doi:10.3389/fmats.2014.00006
Chen, X., Shen, S., Guo, L., and Mao, S. S. (2010). Semiconductor-based photocatalytic hydrogen generation. Chem. Rev. 110, 6503–6570. doi:10.1021/cr1001645
Cheng, H., Hou, J., Takeda, O., Guo, X.-M., and Zhu, H. (2015). A unique z-scheme 2D/2D nanosheet heterojunction design to harness charge transfer for photocatalysis. J. Mater. Chem. A 3, 11006–11013. doi:10.1039/c5ta01864a
Dong, X., and Cheng, F. (2015). Recent development in exfoliated two-dimensional g-C3N4 nanosheets for photocatalytic applications. J. Mater. Chem. A 3, 23642–23652. doi:10.1039/c5ta07374j
Eftekhari, A. (2017). Molybdenum diselenide (MoSe2) for energy storage, catalysis, and optoelectronics. Appl. Mater. Today 8, 1–17. doi:10.1016/j.apmt.2017.01.006
Fan, X. (2015). Graphene: a promising two-dimensional support for heterogeneous catalysts. Front. Mater. 1:39. doi:10.3389/fmats.2014.00039
Fan, X., Zhang, L., Wang, M., Huang, W., Zhou, Y., Li, M., et al. (2016). Constructing carbon-nitride-based copolymers via Schiff base chemistry for visible-light photocatalytic hydrogen evolution. Appl. Catal. B 182, 68–73. doi:10.1016/j.apcatb.2015.09.006
Fang, D., Xiaoying, L., Fei, Z., Xule, P., Xubiao, L., Shenglian, L., et al. (2016). Fabrication of 2D sheet-like BiOCl/carbon quantum dot hybrids via a template-free coprecipitation method and their tunable visible-light photocatalytic activities derived from different size distributions of carbon quantum dots. Nanotechnology 27, 065701. doi:10.1088/0957-4484/27/6/065701
Gu, L., Wang, J., Zou, Z., and Han, X. (2014). Graphitic-C3N4-hybridized TiO2 nanosheets with reactive {001} facets to enhance the UV- and visible-light photocatalytic activity. J. Hazard. Mater. 268, 216–223. doi:10.1016/j.jhazmat.2014.01.021
Gui, M. M., Tan, L.-L., Ong, W.-J., Chai, S.-P., and Mohamed, A. R. (2015). “CO2 photocatalytic reduction: photocatalyst choice and product selectivity,” in CO2 Sequestration, Biofuels and Depollution, eds E. Lichtfouse, J. Schwarzbauer, and D. Robert (Switzerland: Springer International Publishing), 71–104.
Guo, L.-j., Wang, Y.-j., and He, T. (2016a). Photocatalytic reduction of CO2 over heterostructure semiconductors into value-added chemicals. Chem. Rec. 16, 1918–1933. doi:10.1002/tcr.201600008
Guo, Y., Song, S., Zheng, Y., Li, R., and Peng, T. (2016b). Synthesis and characterization of an A2BC type phthalocyanine and its visible-light-responsive photocatalytic H2 production performance on graphitic carbon nitride. Dalton Trans. 45, 14071–14079. doi:10.1039/c6dt01248e
Han, C., Wang, Y., Lei, Y., Wang, B., Wu, N., Shi, Q., et al. (2015). In situ synthesis of graphitic-C3N4 nanosheet hybridized N-doped TiO2 nanofibers for efficient photocatalytic H2 production and degradation. Nano Res. 8, 1199–1209. doi:10.1007/s12274-014-0600-2
He, Z., and Que, W. (2016). Molybdenum disulfide nanomaterials: structures, properties, synthesis and recent progress on hydrogen evolution reaction. Appl. Mater. Today 3, 23–56. doi:10.1016/j.apmt.2016.02.001
Himaja, A. L., Karthik, P. S., and Singh, S. P. (2015). Carbon dots: the newest member of the carbon nanomaterials family. Chem. Rec. 15, 595–615. doi:10.1002/tcr.201402090
Hou, Y., Laursen, A. B., Zhang, J., Zhang, G., Zhu, Y., Wang, X., et al. (2013a). Layered nanojunctions for hydrogen-evolution catalysis. Angew. Chem. Int. Ed. 52, 3621–3625. doi:10.1002/anie.201210294
Hou, Y., Wen, Z., Cui, S., Guo, X., and Chen, J. (2013b). Constructing 2D porous graphitic C3N4 nanosheets/nitrogen-doped graphene/layered MoS2 ternary nanojunction with enhanced photoelectrochemical activity. Adv. Mater. 25, 6291–6297. doi:10.1002/adma.201303116
Hou, Y., Wen, Z., Cui, S., Feng, X., and Chen, J. (2016). Strongly coupled ternary hybrid aerogels of N-deficient porous graphitic-C3N4 nanosheets/N-doped graphene/NiFe-layered double hydroxide for solar-driven photoelectrochemical water oxidation. Nano Lett. 16, 2268–2277. doi:10.1021/acs.nanolett.5b04496
Hou, Y., Zhu, Y., Xu, Y., and Wang, X. (2014). Photocatalytic hydrogen production over carbon nitride loaded with WS2 as cocatalyst under visible light. Appl. Catal. B 15, 122–127. doi:10.1016/j.apcatb.2014.03.002
Hu, S. (2016). Tuning optical properties and photocatalytic activities of carbon-based “quantum dots” through their surface groups. Chem. Rec. 16, 219–230. doi:10.1111/tcr.201500225
Hu, S., Ma, L., Xie, Y., Li, F., Fan, Z., Wang, F., et al. (2015). Hydrothermal synthesis of oxygen functionalized S-P codoped g-C3N4 nanorods with outstanding visible light activity under anoxic conditions. Dalton Trans. 44, 20889–20897. doi:10.1039/c5dt04035c
Huang, Z.-F., Song, J., Pan, L., Wang, Z., Zhang, X., Zou, J.-J., et al. (2015). Carbon nitride with simultaneous porous network and O-doping for efficient solar-energy-driven hydrogen evolution. Nano Energy 12, 646–656. doi:10.1016/j.nanoen.2015.01.043
Ida, S., and Ishihara, T. (2014). Recent progress in two-dimensional oxide photocatalysts for water splitting. J. Phys. Chem. Lett. 5, 2533–2542. doi:10.1021/jz5010957
Inoue, T., Fujishima, A., Konishi, S., and Honda, K. (1979). Photoelectrocatalytic reduction of carbon dioxide in aqueous suspensions of semiconductor powders. Nature 277, 637–638. doi:10.1038/277637a0
Jiang, D., Wang, T., Xu, Q., Li, D., Meng, S., and Chen, M. (2017). Perovskite oxide ultrathin nanosheets/g-C3N4 2D-2D heterojunction photocatalysts with significantly enhanced photocatalytic activity towards the photodegradation of tetracycline. Appl. Catal. B 201, 617–628. doi:10.1016/j.apcatb.2016.09.001
Kalantar-zadeh, K., Ou, J. Z., Daeneke, T., Mitchell, A., Sasaki, T., and Fuhrer, M. S. (2016). Two dimensional and layered transition metal oxides. Appl. Mater. Today 5, 73–89. doi:10.1016/j.apmt.2016.09.012
Keane, D. A., McGuigan, K. G., Ibanez, P. F., Polo-Lopez, M. I., Byrne, J. A., Dunlop, P. S. M., et al. (2014). Solar photocatalysis for water disinfection: materials and reactor design. Catal. Sci. Technol. 4, 1211–1226. doi:10.1039/c4cy00006d
Kotal, M., Kim, J., Oh, J., and Oh, I.-K. (2016). Recent progress in multifunctional graphene aerogels. Front. Mater. 3:29. doi:10.3389/fmats.2016.00029
Lee, H. L., Sofer, Z., Mazánek, V., Luxa, J., Chua, C. K., and Pumera, M. (2017). Graphitic carbon nitride: effects of various precursors on the structural, morphological and electrochemical sensing properties. Appl. Mater. Today. doi:10.1016/j.apmt.2016.09.019
Li, C., Du, Y., Wang, D., Yin, S., Tu, W., Chen, Z., et al. (2017). Unique P-Co-N surface bonding states constructed on g-C3N4 nanosheets for drastically enhanced photocatalytic activity of H2 evolution. Adv. Funct. Mater. 27, 1604328. doi:10.1002/adfm.201604328
Li, P., Chen, C., Zhang, J., Li, S., Sun, B., and Bao, Q. (2014). Graphene-based transparent electrodes for hybrid solar cells. Front. Mater. 1:26. doi:10.3389/fmats.2014.00026
Li, X., Yu, J., and Jaroniec, M. (2016a). Hierarchical photocatalysts. Chem. Soc. Rev. 45, 2603–2636. doi:10.1039/c5cs00838g
Li, Y., Wei, X., Yan, X., Cai, J., Zhou, A., Yang, M., et al. (2016b). Construction of inorganic-organic 2D/2D WO3/g-C3N4 nanosheet arrays toward efficient photoelectrochemical splitting of natural seawater. Phys. Chem. Chem. Phys. 18, 10255–10261. doi:10.1039/c6cp00353b
Li, Y., Li, K., Yang, Y., Li, L., Xing, Y., Song, S., et al. (2015). Ultrathin g-C3N4 nanosheets coupled with AgIO3 as highly efficient heterostructured photocatalysts for enhanced visible-light photocatalytic activity. Chem. Eur. J. 21, 17739–17747. doi:10.1002/chem.201502945
Li, Y., Zhang, H., Liu, P., Wang, D., Li, Y., and Zhao, H. (2013). Cross-linked g-C3N4/rGO nanocomposites with tunable band structure and enhanced visible light photocatalytic activity. Small 9, 3336–3344. doi:10.1002/smll.201203135
Liang, Q., Huang, Z.-H., Kang, F., and Yang, Q.-H. (2015a). Facile synthesis of crystalline polymeric carbon nitrides with an enhanced photocatalytic performance under visible light. ChemCatChem 7, 2897–2902. doi:10.1002/cctc.201500076
Liang, Q., Li, Z., Huang, Z.-H., Kang, F., and Yang, Q.-H. (2015b). Holey graphitic carbon nitride nanosheets with carbon vacancies for highly improved photocatalytic hydrogen production. Adv. Funct. Mater. 25, 6885–6892. doi:10.1002/adfm.201503221
Liang, Q., Li, Z., Yu, X., Huang, Z.-H., Kang, F., and Yang, Q.-H. (2015c). Macroscopic 3D porous graphitic carbon nitride monolith for enhanced photocatalytic hydrogen evolution. Adv. Mater. 27, 4634–4639. doi:10.1002/adma.201502057
Liang, Q., Ye, L., Xu, Q., Huang, Z.-H., Kang, F., and Yang, Q.-H. (2015d). Graphitic carbon nitride nanosheet-assisted preparation of N-enriched mesoporous carbon nanofibers with improved capacitive performance. Carbon N. Y. 94, 342–348. doi:10.1016/j.carbon.2015.07.001
Linsebigler, A. L., Lu, G., and Yates, J. T. (1995). Photocatalysis on TiO2 surfaces: principles, mechanisms, and selected results. Chem. Rev. 95, 735–758. doi:10.1021/cr00035a013
Liu, J., Liu, Y., Liu, N., Han, Y., Zhang, X., Huang, H., et al. (2015). Metal-free efficient photocatalyst for stable visible water splitting via a two-electron pathway. Science 347, 970–974. doi:10.1126/science.aaa3145
Liu, J., Wang, H., and Antonietti, M. (2016a). Graphitic carbon nitride “reloaded”: emerging applications beyond (photo)catalysis. Chem. Soc. Rev. 45, 2308–2326. doi:10.1039/c5cs00767d
Liu, Q., Cao, F., Wu, F., Chen, S., Xiong, J., and Li, L. (2016b). Partial ion exchange derived 2D Cu–Zn–In–S nanosheets as sensitizers of 1D TiO2 nanorods for boosting solar water splitting. ACS Appl. Mater. Interfaces 8, 26235–26243. doi:10.1021/acsami.6b08648
Liu, P., Liu, Y., Ye, W., Ma, J., and Gao, D. (2016c). Flower-like N-doped MoS2 for photocatalytic degradation of RhB by visible light irradiation. Nanotechnology 27, 225403. doi:10.1088/0957-4484/27/22/225403
Liu, S., Zhang, N., and Xu, Y.-J. (2014). Core–shell structured nanocomposites for photocatalytic selective organic transformations. Part. Part. Syst. Charact. 31, 540–556. doi:10.1002/ppsc.201300235
Liu, X., Iocozzia, J., Wang, Y., Cui, X., Chen, Y., Zhao, S., et al. (2017). Noble metal-metal oxide nanohybrids with tailored nanostructures for efficient solar energy conversion, photocatalysis and environmental remediation. Energy Environ. Sci. 10, 402–434. doi:10.1039/c6ee02265k
Lu, X., Jin, Y., Zhang, X., Xu, G., Wang, D., Lv, J., et al. (2016). Controllable synthesis of graphitic C3N4/ultrathin MoS2 nanosheet hybrid nanostructures with enhanced photocatalytic performance. Dalton Trans. 45, 15406–15414. doi:10.1039/c6dt02247b
Ma, Y., Wang, X., Jia, Y., Chen, X., Han, H., and Li, C. (2014). Titanium dioxide-based nanomaterials for photocatalytic fuel generations. Chem. Rev. 114, 9987–10043. doi:10.1021/cr500008u
Maeda, K., Eguchi, M., and Oshima, T. (2014). Perovskite oxide nanosheets with tunable band-edge potentials and high photocatalytic hydrogen-evolution activity. Angew. Chem. Int. Ed. 53, 13164–13168. doi:10.1002/anie.201408441
Mamba, G., and Mishra, A. K. (2016). Graphitic carbon nitride (g-C3N4) nanocomposites: a new and exciting generation of visible light driven photocatalysts for environmental pollution remediation. Appl. Catal. B 198, 347–377. doi:10.1016/j.apcatb.2016.05.052
Mateo, D., Esteve-Adell, I., Albero, J., Royo, J. F. S., Primo, A., and Garcia, H. (2016). 111 oriented gold nanoplatelets on multilayer graphene as visible light photocatalyst for overall water splitting. Nat. Commun. 7, 11819. doi:10.1038/ncomms11819
Meng, F., Hong, Z., Arndt, J., Li, M., Zhi, M., Yang, F., et al. (2012). Visible light photocatalytic activity of nitrogen-doped La2Ti2O7 nanosheets originating from band gap narrowing. Nano Res. 5, 213–221. doi:10.1007/s12274-012-0201-x
Niu, P., Zhang, L., Liu, G., and Cheng, H.-M. (2012). Graphene-like carbon nitride nanosheets for improved photocatalytic activities. Adv. Funct. Mater. 22, 4763–4770. doi:10.1002/adfm.201200922
Ong, W.-J., Gui, M. M., Chai, S.-P., and Mohamed, A. R. (2013). Direct growth of carbon nanotubes on Ni/TiO2 as next generation catalysts for photoreduction of CO2 to methane by water under visible light irradiation. RSC Adv. 3, 4505–4509. doi:10.1039/C3RA00030C
Ong, W.-J., Putri, L. K., Tan, L.-L., Chai, S.-P., and Yong, S.-T. (2016a). Heterostructured AgX/g-C3N4 (X = Cl and Br) nanocomposites via a sonication-assisted deposition-precipitation approach: emerging role of halide ions in the synergistic photocatalytic reduction of carbon dioxide. Appl. Catal. B 180, 530–543. doi:10.1016/j.apcatb.2015.06.053
Ong, W.-J., Tan, L.-L., Ng, Y. H., Yong, S.-T., and Chai, S.-P. (2016b). Graphitic carbon nitride (g-C3N4)-based photocatalysts for artificial photosynthesis and environmental remediation: are we a step closer to achieving sustainability? Chem. Rev. 116, 7159–7329. doi:10.1021/acs.chemrev.6b00075
Ong, W.-J., Putri, L. K., Tan, Y.-C., Tan, L.-L., Li, N., Ng, Y. H., et al. (2017). Unravelling charge carrier dynamics in protonated g-C3N4 interfaced with carbon nanodots as co-catalysts toward enhanced photocatalytic CO2 reduction: a combined experimental and first-principles DFT study. Nano Res. doi:10.1007/s12274-016-1391-4
Ong, W.-J., Tan, L.-L., Chai, S.-P., and Yong, S.-T. (2015a). Graphene oxide as a structure-directing agent for the two-dimensional interface engineering of sandwich-like graphene-g-C3N4 hybrid nanostructures with enhanced visible-light photoreduction of CO2 to methane. Chem. Commun. 51, 858–861. doi:10.1039/c4cc08996k
Ong, W.-J., Tan, L.-L., Chai, S.-P., and Yong, S.-T. (2015b). Heterojunction engineering of graphitic carbon nitride (g-C3N4) via Pt loading with improved daylight-induced photocatalytic reduction of carbon dioxide to methane. Dalton Trans. 44, 1249–1257. doi:10.1039/c4dt02940b
Ong, W.-J., Tan, L.-L., Chai, S.-P., Yong, S.-T., and Mohamed, A. R. (2015c). Surface charge modification via protonation of graphitic carbon nitride (g-C3N4) for electrostatic self-assembly construction of 2D/2D reduced graphene oxide (rGO)/g-C3N4 nanostructures toward enhanced photocatalytic reduction of carbon dioxide to methane. Nano Energy 13, 757–770. doi:10.1016/j.nanoen.2015.03.014
Ong, W.-J., Tan, L.-L., Chai, S.-P., Yong, S.-T., and Mohamed, A. R. (2014a). Facet-dependent photocatalytic properties of TiO2-based composites for energy conversion and environmental remediation. ChemSusChem 7, 690–719. doi:10.1002/cssc.201300924
Ong, W.-J., Tan, L.-L., Chai, S.-P., Yong, S.-T., and Mohamed, A. R. (2014b). Highly reactive {001} facets of TiO2-based composites: synthesis, formation mechanism and characterizations. Nanoscale 6, 1946–2008. doi:10.1039/c3nr04655a
Ong, W.-J., Tan, L.-L., Chai, S.-P., Yong, S.-T., and Mohamed, A. R. (2014c). Self-assembly of nitrogen-doped TiO2 with exposed {001} facets on a graphene scaffold as photo-active hybrid nanostructures for reduction of carbon dioxide to methane. Nano Res. 7, 1528–1547. doi:10.1007/s12274-014-0514-z
Ong, W.-J., Voon, S.-Y., Tan, L.-L., Goh, B. T., Yong, S.-T., and Chai, S.-P. (2014d). Enhanced daylight-induced photocatalytic activity of solvent exfoliated graphene (SEG)/ZnO hybrid nanocomposites towards degradation of reactive black 5. Ind. Eng. Chem. Res. 53, 17333–17344. doi:10.1021/ie5027088
Ong, W.-J., Yeong, J.-J., Tan, L.-L., Goh, B. T., Yong, S.-T., and Chai, S.-P. (2014e). Synergistic effect of graphene as a co-catalyst for enhanced daylight-induced photocatalytic activity of Zn0.5Cd0.5S synthesized via an improved one-pot co-precipitation-hydrothermal strategy. RSC Adv. 4, 59676–59685. doi:10.1039/c4ra10467f
Oshima, T., Eguchi, M., and Maeda, K. (2016). Photocatalytic water oxidation over metal oxide nanosheets having a three-layer perovskite structure. ChemSusChem 9, 396–402. doi:10.1002/cssc.201501237
Osterloh, F. E. (2017). Photocatalysis versus photosynthesis – a sensitivity analysis of devices for solar energy conversion and chemical transformations. ACS Energy Lett. 2, 445–453. doi:10.1021/acsenergylett.6b00665
Pan, Z., Zheng, Y., Guo, F., Niu, P., and Wang, X. (2017). Decorating CoP and Pt nanoparticles on graphitic carbon nitride nanosheets to promote overall water splitting by conjugated polymers. ChemSusChem 10, 87–90. doi:10.1002/cssc.201600850
Putri, L. K., Ng, B.-J., Ong, W.-J., Lee, H. W., Chang, W. S., and Chai, S.-P. (2017). Heteroatom nitrogen- and boron-doping as a facile strategy to improve photocatalytic activity of standalone reduced graphene oxide in hydrogen evolution. ACS Appl. Mater. Interfaces 9, 4558–4569. doi:10.1021/acsami.6b12060
Putri, L. K., Ong, W.-J., Chang, W. S., and Chai, S.-P. (2015). Heteroatom doped graphene in photocatalysis: a review. Appl. Surf. Sci. 358(Part A), 2–14. doi:10.1016/j.apsusc.2015.08.177
Putri, L. K., Ong, W.-J., Chang, W. S., and Chai, S.-P. (2016a). Enhancement in the photocatalytic activity of carbon nitride through hybridization with light-sensitive AgCl for carbon dioxide reduction to methane. Catal. Sci. Technol. 6, 744–754. doi:10.1039/c5cy00767d
Putri, L. K., Tan, L.-L., Ong, W.-J., Chang, W. S., and Chai, S.-P. (2016b). Graphene oxide: exploiting its unique properties toward visible-light-driven photocatalysis. Appl. Mater. Today 4, 9–16. doi:10.1016/j.apmt.2016.04.001
Rahman, M. Z., Ran, R. J., Tang, Y., Jaroniec, M., and Qiao, S. (2016). Surface activated carbon nitride nanosheets with optimized electro-optical properties for highly efficient photocatalytic hydrogen production. J. Mater. Chem. A 4, 2445–2452. doi:10.1039/c5ta10194h
Roger, I., Shipman, M. A., and Symes, M. D. (2017). Earth-abundant catalysts for electrochemical and photoelectrochemical water splitting. Nat. Rev. Chem. 1, 0003. doi:10.1038/s41570-016-0003
Sabio, E. M., Chi, M., Browning, N. D., and Osterloh, F. E. (2010). Charge separation in a niobate nanosheet photocatalyst studied with photochemical labeling. Langmuir 26, 7254–7261. doi:10.1021/la904377f
She, X., Wu, J., Xu, H., Mo, Z., Lian, J., Song, Y., et al. (2017). Enhancing charge density and steering charge unidirectional flow in 2D non-metallic semiconductor-CNTs-metal coupled photocatalyst for solar energy conversion. Appl. Catal. B 202, 112–117. doi:10.1016/j.apcatb.2016.09.013
She, X., Wu, J., Zhong, J., Xu, H., Yang, Y., Vajtai, R., et al. (2016). Oxygenated monolayer carbon nitride for excellent photocatalytic hydrogen evolution and external quantum efficiency. Nano Energy 27, 138–146. doi:10.1016/j.nanoen.2016.06.042
Shi, L., Chang, K., Zhang, H., Hai, X., Yang, L., Wang, T., et al. (2016). Drastic enhancement of photocatalytic activities over phosphoric acid protonated porous g-C3N4 nanosheets under visible light. Small 12, 4431–4439. doi:10.1002/smll.201601668
Tan, L.-L., Ong, W.-J., Chai, S.-P., Goh, B. T., and Mohamed, A. R. (2015a). Visible-light-active oxygen-rich TiO2 decorated 2D graphene oxide with enhanced photocatalytic activity towards carbon dioxide reduction. Appl. Catal. B 179, 160–170. doi:10.1016/j.apcatb.2015.05.024
Tan, L.-L., Ong, W.-J., Chai, S.-P., and Mohamed, A. R. (2015b). Noble metal modified reduced graphene oxide/TiO2 ternary nanostructures for efficient visible-light-driven photoreduction of carbon dioxide into methane. Appl. Catal. B 16, 251–259. doi:10.1016/j.apcatb.2014.11.035
Tan, L.-L., Ong, W.-J., Chai, S.-P., and Mohamed, A. R. (2013). Growth of carbon nanotubes over non-metallic based catalysts: a review on the recent developments. Catal. Today 217, 1–12. doi:10.1016/j.cattod.2012.10.023
Tan, L.-L., Ong, W.-J., Chai, S.-P., and Mohamed, A. R. (2014). Band gap engineered, oxygen-rich TiO2 for visible light induced photocatalytic reduction of CO2. Chem. Commun. 50, 6923–6926. doi:10.1039/c4cc01304b
Tan, L.-L., Ong, W.-J., Chai, S.-P., and Mohamed, A. R. (2016). Visible-light-activated oxygen-rich TiO2 as next generation photocatalyst: importance of annealing temperature on the photoactivity toward reduction of carbon dioxide. Chem. Eng. J. 283, 1254–1263. doi:10.1016/j.cej.2015.07.093
Tan, L.-L., Ong, W.-J., Chai, S.-P., and Mohamed, A. R. (2017). Photocatalytic reduction of CO2 with H2O over graphene oxide-supported oxygen-rich TiO2 hybrid photocatalyst under visible light irradiation: process and kinetic studies. Chem. Eng. J. 308, 248–255. doi:10.1016/j.cej.2016.09.050
Thaweesak, S., Lyu, M., Peerakiatkhajohn, P., Butburee, T., Luo, B., Chen, H., et al. (2017). Two-dimensional g-C3N4/Ca2Nb2TaO10 nanosheet composites for efficient visible light photocatalytic hydrogen evolution. Appl. Catal. B 202, 184–190. doi:10.1016/j.apcatb.2016.09.022
Tonda, S., Kumar, S., Kandula, S., and Shanker, V. (2014). Fe-doped and -mediated graphitic carbon nitride nanosheets for enhanced photocatalytic performance under natural sunlight. J. Mater. Chem. A 2, 6772–6780. doi:10.1039/c3ta15358d
Tong, Z., Yang, D., Li, Z., Nan, Y., Ding, F., Shen, Y., et al. (2017). Thylakoid-inspired multishell g-C3N4 nanocapsules with enhanced visible-light harvesting and electron transfer properties for high-efficiency photocatalysis. ACS Nano 11, 1103–1112. doi:10.1021/acsnano.6b08251
Topcu, S., Jodhani, G., and Gouma, P. (2016). Optimized nanostructured TiO2 photocatalysts. Front. Mater. 3:35. doi:10.3389/fmats.2016.00035
Varadwaj, G. B. B., and Nyamori, V. O. (2016). Layered double hydroxide- and graphene-based hierarchical nanocomposites: synthetic strategies and promising applications in energy conversion and conservation. Nano Res. 9, 3598–3621. doi:10.1007/s12274-016-1250-3
Voon, S.-Y., Ong, W.-J., Tan, L.-L., Yong, S.-T., and Chai, S.-P. (2016). “Graphene-based semiconductor materials for photocatalytic applications,” in Graphene Science Handbook: Size-Dependent Properties, eds M. Aliofkhazraei, N. Ali, W. I. Milne, C. S. Ozkan, S. Mitura and J. L. Gervasoni (Florida, USA: CRC Press), 331–352.
Wan, W., Yu, S., Dong, F., Zhang, Q., and Zhou, Y. (2016). Efficient C3N4/graphene oxide macroscopic aerogel visible-light photocatalyst. J. Mater. Chem. A 4, 7823–7829. doi:10.1039/c6ta01804a
Wang, J., Guan, Z., Huang, J., Li, Q., and Yang, J. (2014). Enhanced photocatalytic mechanism for the hybrid g-C3N4/MoS2 nanocomposite. J. Mater. Chem. A 2, 7960–7966. doi:10.1039/c4ta00275j
Wang, X., Maeda, K., Thomas, A., Takanabe, K., Xin, G., Carlsson, J. M., et al. (2009). A metal-free polymeric photocatalyst for hydrogen production from water under visible light. Nat. Mater. 8, 76–80. doi:10.1038/nmat2317
Wei, Y., Su, J., Wan, X., Guo, L., and Vayssieres, L. (2016). Spontaneous photoelectric field-enhancement effect prompts the low cost hierarchical growth of highly ordered heteronanostructures for solar water splitting. Nano Res. 9, 1561–1569. doi:10.1007/s12274-016-1050-9
Wen, J., Xie, J., Shen, R., Li, X., Luo, X., Zhang, H., et al. (2017). Markedly enhanced visible-light photocatalytic H2 generation over g-C3N4 nanosheets decorated by robust nickel phosphide (Ni12P5) cocatalysts. Dalton Trans. 46, 1794–1802. doi:10.1039/c6dt04575h
Wenderich, K., and Mul, G. (2016). Methods, mechanism, and applications of photodeposition in photocatalysis: a review. Chem. Rev. 116, 14587–14619. doi:10.1021/acs.chemrev.6b00327
Xia, P., Zhu, B., Yu, J., Cao, S., and Jaroniec, M. (2017). Ultra-thin nanosheet assemblies of graphitic carbon nitride for enhanced photocatalytic CO2 reduction. J. Mater. Chem. A 5, 3230–3238. doi:10.1039/c6ta08310b
Xiang, Q., Cheng, F., and Lang, D. (2016). Hierarchical layered WS2/graphene-modified CdS nanorods for efficient photocatalytic hydrogen evolution. ChemSusChem 9, 996–1002. doi:10.1002/cssc.201501702
Xiang, Q., Yu, J., and Jaroniec, M. (2011). Preparation and enhanced visible-light photocatalytic H2-production activity of graphene/C3N4 composites. J. Phys. Chem. C 115, 7355–7363. doi:10.1021/jp200953k
Xing, W., Li, C., Wang, Y., Han, Z., Hu, Y., Chen, D., et al. (2017). A novel 2D/2D carbonized poly-(furfural alcohol)/g-C3N4 nanocomposites with enhanced charge carrier separation for photocatalytic H2 evolution. Carbon N. Y. 115, 486–492. doi:10.1016/j.carbon.2017.01.045
Xu, J., Gu, P., Zhang, J., Xue, H., and Pang, H. (2016). Copper-based nanomaterials for high-performance lithium-ion batteries. Part. Part. Syst. Charact. 33, 784–810. doi:10.1002/ppsc.201600150
Xu, L., Huang, W.-Q., Wang, L.-L., Tian, Z.-A., Hu, W., Ma, Y., et al. (2015). Insights into enhanced visible-light photocatalytic hydrogen evolution of g-C3N4 and highly reduced graphene oxide composite: the role of oxygen. Chem. Mater. 27, 1612–1621. doi:10.1021/cm504265w
Xu, Y., Xu, H., Wang, L., Yan, J., Li, H., Song, Y., et al. (2013). The CNT modified white C3N4 composite photocatalyst with enhanced visible-light response photoactivity. Dalton Trans. 42, 7604–7613. doi:10.1039/c3dt32871f
Xueting, C., Xinxin, Z., Shibin, S., Danxia, G., Lihua, D., Yansheng, Y., et al. (2017). MnO2/g-C3N4 nanocomposite with highly enhanced supercapacitor performance. Nanotechnology 28, 135705. doi:10.1088/1361-6528/aa6107
Ye, L., Wang, D., and Chen, S. (2016). Fabrication and enhanced photoelectrochemical performance of MoS2/S-doped g-C3N4 heterojunction film. ACS Appl. Mater. Interfaces 8, 5280–5289. doi:10.1021/acsami.5b11326
Ye, M.-Y., Zhao, Z.-H., Hu, Z.-F., Liu, L.-Q., Ji, H.-M., Shen, Z.-R., et al. (2017). 0D/2D heterojunctions of vanadate quantum dots/graphitic carbon nitride nanosheets for enhanced visible-light-driven photocatalysis. Angew. Chem. Int. Ed. doi:10.1002/anie.201611127
Yi, S.-S., Yan, J.-M., Wulan, B.-R., Li, S.-J., Liu, K.-H., and Jiang, Q. (2017). Noble-metal-free cobalt phosphide modified carbon nitride: an efficient photocatalyst for hydrogen generation. Appl. Catal. B 200, 477–483. doi:10.1016/j.apcatb.2016.07.046
Yin, S., Han, J., Zhou, T., and Xu, R. (2015). Recent progress in g-C3N4 based low cost photocatalytic system: activity enhancement and emerging applications. Catal. Sci. Technol. 5, 5048–5061. doi:10.1039/c5cy00938c
Yu, X., and Sivula, K. (2016). Toward large-area solar energy conversion with semiconducting 2D transition metal dichalcogenides. ACS Energy Lett. 1, 315–322. doi:10.1021/acsenergylett.6b00114
Yubin, C., Chi-Hung, C., Zhixiao, Q., Shaohua, S., Tennyson, D., and Clemens, B. (2017). Electron-transfer dependent photocatalytic hydrogen generation over cross-linked CdSe/TiO2 type-II heterostructure. Nanotechnology 28, 084002. doi:10.1088/1361-6528/aa5642
Zhang, H., Liu, G., Shi, L., Liu, H., Wang, T., and Ye, J. (2016a). Engineering coordination polymers for photocatalysis. Nano Energy 22, 149–168. doi:10.1016/j.nanoen.2016.01.029
Zhang, Q., Hu, S., Fan, Z., Liu, D., Zhao, Y., Ma, H., et al. (2016b). Preparation of g-C3N4/ZnMoCdS hybrid heterojunction catalyst with outstanding nitrogen photofixation performance under visible light via hydrothermal post-treatment. Dalton Trans. 45, 3497–3505. doi:10.1039/c5dt04901f
Zhang, Y., Zhou, Y., Tang, L., Wang, M., Li, P., Tu, W., et al. (2016c). Fabrication of oxygen-doped double-shelled GaN hollow spheres toward efficient photoreduction of CO2. Part. Part. Syst. Charact. 33, 583–588. doi:10.1002/ppsc.201500235
Zhang, Z., Jiang, D., Li, D., He, M., and Chen, M. (2016d). Construction of SnNb2O6 nanosheet/g-C3N4 nanosheet two-dimensional heterostructures with improved photocatalytic activity: synergistic effect and mechanism insight. Appl. Catal. B 183, 113–123. doi:10.1016/j.apcatb.2015.10.022
Zhang, Z., Liu, K., Feng, Z., Bao, Y., and Dong, B. (2016e). Hierarchical sheet-on-sheet ZnIn2S4/g-C3N4 heterostructure with highly efficient photocatalytic H2 production based on photoinduced interfacial charge transfer. Sci. Rep. 6, 19221. doi:10.1038/srep19221
Zhang, Z., Zheng, T., Li, X., Xu, J., and Zeng, H. (2016f). Progress of carbon quantum dots in photocatalysis applications. Part. Part. Syst. Charact. 33, 457–472. doi:10.1002/ppsc.201500243
Zhang, C., Lu, Y., Jiang, Q., and Hu, J. (2016g). Synthesis of CdS hollow spheres coupled with g-C3N4 as efficient visible-light-driven photocatalysts. Nanotechnology 27, 355402. doi:10.1088/0957-4484/27/35/355402
Zhang, J., Chen, Y., and Wang, X. (2015a). Two-dimensional covalent carbon nitride nanosheets: synthesis, functionalization, and applications. Energy Environ. Sci. 8, 3092–3108. doi:10.1039/c5ee01895a
Zhang, N., Yang, M.-Q., Liu, S., Sun, Y., and Xu, Y.-J. (2015b). Waltzing with the versatile platform of graphene to synthesize composite photocatalysts. Chem. Rev. 115, 10307–10377. doi:10.1021/acs.chemrev.5b00267
Zhang, N., Zhang, Y., and Xu, Y.-J. (2012). Recent progress on graphene-based photocatalysts: current status and future perspectives. Nanoscale 4, 5792–5813. doi:10.1039/c2nr31480k
Zhao, H., Jiang, P., and Cai, W. (2017a). Graphitic C3N4 decorated with CoP co-catalyst: enhanced and stable photocatalytic H2 evolution activity from water under visible-light irradiation. Chem. Asian J. 12, 361–365. doi:10.1002/asia.201601543
Zhao, H., Sun, S., Jiang, P., and Xu, Z. J. (2017b). Graphitic C3N4 modified by Ni2P cocatalyst: an efficient, robust and low cost photocatalyst for visible-light-driven H2 evolution from water. Chem. Eng. J. 315, 296–303. doi:10.1016/j.cej.2017.01.034
Zhao, J., Ke, X., Liu, H., Huang, Y., Chen, C., Bo, A., et al. (2016a). Comparing the contribution of visible-light irradiation, gold nanoparticles, and titania supports in photocatalytic nitroaromatic coupling and aromatic alcohol oxidation. Part. Part. Syst. Charact. 33, 628–634. doi:10.1002/ppsc.201600049
Zhao, L., Xu, H., Jiang, B., and Huang, Y. (2016b). Synergetic photocatalytic nanostructures based on Au/TiO2/reduced graphene oxide for efficient degradation of organic pollutants. Part. Part. Syst. Charact. doi:10.1002/ppsc.201600323
Zhao, Z., Sun, Y., and Dong, F. (2015). Graphitic carbon nitride based nanocomposites: a review. Nanoscale 7, 15–37. doi:10.1039/c4nr03008g
Zheng, D., Huang, C., and Wang, X. (2015). Post-annealing reinforced hollow carbon nitride nanospheres for hydrogen photosynthesis. Nanoscale 7, 465–470. doi:10.1039/c4nr06011c
Keywords: graphitic carbon nitride (g-C3N4), photocatalysis, energy conversion, environmental remediation, 2D/2D heterojunction, face-to-face interface
Citation: Ong W-J (2017) 2D/2D Graphitic Carbon Nitride (g-C3N4) Heterojunction Nanocomposites for Photocatalysis: Why Does Face-to-Face Interface Matter? Front. Mater. 4:11. doi: 10.3389/fmats.2017.00011
Received: 26 January 2017; Accepted: 17 March 2017;
Published: 12 April 2017
Edited by:
Wei Lv, Tsinghua University, ChinaReviewed by:
Chen Zhang, Tianjin University, ChinaYi Wen Phuan, Monash University Malaysia, Malaysia
Copyright: © 2017 Ong. This is an open-access article distributed under the terms of the Creative Commons Attribution License (CC BY). The use, distribution or reproduction in other forums is permitted, provided the original author(s) or licensor are credited and that the original publication in this journal is cited, in accordance with accepted academic practice. No use, distribution or reproduction is permitted which does not comply with these terms.
*Correspondence: Wee-Jun Ong, b25nd2omI3gwMDA0MDtpbXJlLmEtc3Rhci5lZHUuc2c=, b25nd2VlanVuJiN4MDAwNDA7Z21haWwuY29t, https://sites.google.com/site/wjongresearch/