- Department of Materials Science and Chemistry, Graduate School of Engineering, University of Hyogo, Hyogo, Japan
Pillared carbons with interlayer spacings of 1.27–1.37 nm were obtained from graphite oxide repeatedly silylated with methyltrichlorosilane, when the content of it was higher than 1.5/GO unit. The BET surface area of the resulting pillared carbons reached 723 m2/g and decreased with the increase in the content of Si because of the increase in the density of pillar. Based on the intercalation behaviors of n-alkylamine molecules into pillared carbons, it has been concluded that the entrance for organic molecules between pillars and length of pillars were almost independent of the pillar density. The molecular length of n-butylamine molecule added during the silylation of graphite oxide seems to determine the length of the pillars.
Introduction
Recently, various types of porous materials with controlled pore structures have been prepared and tested for the support of catalysts, gas storage, separation, adsorbents, electrode of electric double layer capacitor, and so on. They include template carbons (Kyotani, 2006), mesoporous silicas or non-siliceous oxides (Kresge et al., 1992; Bagshaw et al., 1995; Shimojima and Kuroda, 2008; Yamauchi et al., 2009a,b; Hoffman and Froeba, 2011; Dong and Ferdi, 2014), porous coordination polymers/metal organic frameworks (PCPs/MOFs) (Yaghi et al., 2003; Kitagawa et al., 2004; Rosseinski, 2004; Férey, 2008; Jiang and Xu, 2011), etc. In this context, we have prepared porous pillared carbons from the thermal reduction of graphite oxide silylated with various silylating reagents (Matsuo et al., 2007, 2009a,b, 2012a; Matsuo and Konishi, 2011). In these materials, the adjacent carbon layers are connected with each other by silica or silsesquioxane pillars and meso- or micropores surrounded by carbon layers and pillars are formed. Some of them have been tested for the electrode of electric double layer capacitor (Yoo et al., 2011), hydrogen storage materials (Matsuo et al., 2012b), and size-selective gas sensors (Matsuo et al., 2012c, 2014). Among them, pillared carbon obtained from graphite oxide repeatedly silyated with methyltrichlorosilane showed very unique properties. For example, organic molecules are size-selectively intercalated into it, indicating that the distance between adjacent pillars for the entrance of them is precisely controlled between 0.36 and 0.4 nm and the pillars are flexible (Matsuo and Konishi, 2011; Matsuo et al., 2012a). It also stored considerable amounts of hydrogen at room temperature under high pressure with a relatively high heat of adsorption (Matsuo et al., 2012a). Apart from the flexible PCPs, which are recently defined as soft porous crystals (Horike et al., 2009), porous materials with structural flexibility were rarely reported, except for the zeolite template porous carbons under high pressure (Ito et al., 2013). The PCPs have been widely studied and many interesting results have been provided; however, very few PCPs with high conductivity, which is inevitable for the use of them in electrical or electrochemical devices have been prepared (Yang et al., 2010; Zeng et al., 2010). On the other hand, pillared carbons show not only similar structural flexibility but also high electrical conductivity, therefore, they are promising for these applications. It would be very interesting if we can modify the pore structure of these unique pillared carbons.
In this study, pillared carbons were prepared from graphite oxide silylated with methyltrichlorosilane for various times and, their porous properties were investigated based on the nitrogen gas adsorption and the intercalation behaviors of n-alkkylamine molecules. We have already studied the pillared carbon obtained from graphite oxide silylated with methyltrichlorosilane for three times in detail (Matsuo and Konishi, 2011; Matsuo et al., 2012a); however, the effect of the Si content in the silyalted graphite oxide on the formation of pillared carbons and porous properties of them are not understood yet. It is expected that the changes of the Si contents may change the distance between pillars, the size of pores, surface area, etc.
Materials and Methods
Preparation of pillared carbon was performed in the same manner as reported in our previous studies (Matsuo and Konishi, 2011, 2012). Graphite oxide was prepared from natural graphite powder (57–74 μm) in fuming nitric acid using potassium chlorate, based on the Brodie’s method (Brodie, 1859) and oxidation procedure was repeated for five times. The composition of the resulting GO was C8H3.0O4.5. GO was silylated as follows (Matsuo et al., 2004, 2005). GO (100 mg) was mixed with n-butylamine [C4H9NH2, hereafter abbreviated as C4, 2 ml; 32 mol/GO unit (C8H3.0O4.5)] as an exfoliating reagent in a sealed glass vial under an Argon atmosphere and the resulting solution was sonicated for 15 min (VELVO-CLEAR, VS-150; 50 kHz, 150 W), then heated at 60°C for 1 h. Dry toluene (5 ml, water content <30 ppm) was added to this solution and the solution was again sonicated. Silylating reagents (methyltrichlorosilane, CH3SiCl3: 0.90 mL; this amount corresponds to 13 mol/GO unit) were added to the resulting and then allowed to stand for 2 days at 60°C. Hereafter, the silylating reagents are abbreviated as C1Si. After centrifugation at 4000 rpm for 20 min, the precipitate was washed with dry toluene, ethanol, and finally acetone. The obtained silylated GO samples were then silylated with C1Si in the same manner as described above for 1 day for 1–4 times. The composition of silylated GO was determined from the weight of residual SiO2 after thermogravimetric analysis, assuming that 0.4 n-butylamine molecules per GO were included (Matsuo et al., 2005). The resulting silylating GO was heated at 500°C under dynamic vacuum. The temperature increase rate was 1°C/min and the amount of the sample was <200 mg in order to avoid the deflagration of GO layers due to the heat generate during the removal of oxygen functionalities from GO layers. The content of silicon was also estimated from the weight of residual SiO2 after thermogravimetric measurement.
These samples were analyzed by X-ray diffraction (Rigaku, Rint-2100, CuKα), thermogravimetric (TG; Shimadzu, TGA-50), and nitrogen adsorption (Bel Japan Inc., BELSORP-max, −196°C) measurements. TG measurement was performed under air with the temperature increase rate of 5°C/min between room temperature and 800°C. Intercalation behavior of organic molecules into pillared carbons was investigated as follows. Pillared carbon samples were immersed in the solutions of n-alkylamines with various alkyl chain lengths for 24 h and the resulting samples were analyzed by X-ray diffractometry without removing excess n-alkylamine molecules.
Results
Figure 1 shows the X-ray diffraction patterns of GO repeatedly silylated with metyltrichlorosilane. The diffraction peak at 2θ = 13.9° observed for pristine GO shifted to lower angle when it was repeatedly silylated with C1Si and a new broad peak was observed at 8° for the sample silylated with C1Si for five times. Based on the thermogravimetric analysis of silylated GO samples, their compositions were determined and summarized in Table 1, together with the interlayer spacings. It is found that 0.5–0.8 C1Si molecules are attached to GO in each silylation step, except for the last one (1.13). Figure 2 shows the SEM images of GO silylated with C1Si for four and five times. While only plate like particles were observed for GO silylated with C1Si for four times, some of the plate like particles of GO silylated with C1Si for five times were covered with smaller aggregated particles as indicated with circles in Figure 2B. Considering that the larger amount of introduced Si in the last step and the new and unidentified diffraction peak observed at 2θ = 8°, these particles are ascribed to the silica based species formed as the result of polymerization of excess C1Si on the surface of silylated GO. This indicates that the interlayer space of GO was saturated with C1Si for the sample of (C1Si)3.90GO.
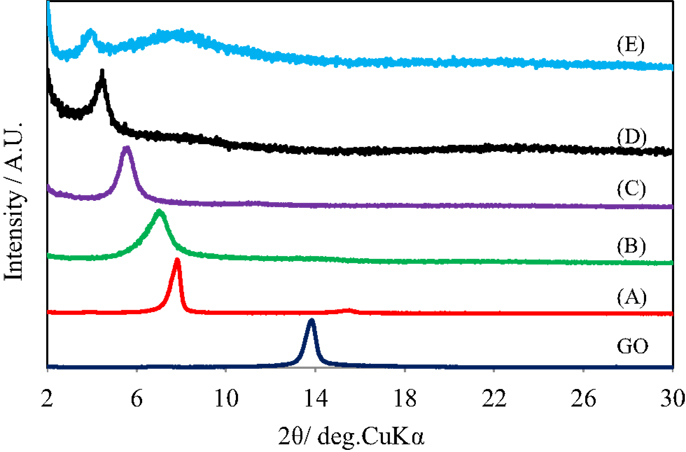
Figure 1. X-ray diffraction patterns of GO and GO silylated with metyltrichlorosilane for (A) 1, (B) 2, (C) 3, (D) 4, and (E) 5 times.
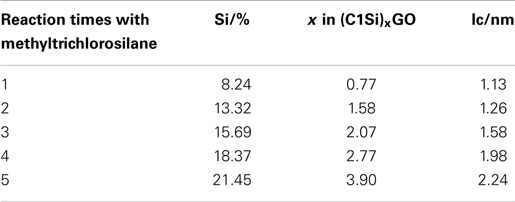
Table 1. Silicon contents, compositions, and interlayer spacings of graphite oxide silylated with methyltrichlorosilane for various times.
Figure 3 shows the X-ray diffraction patterns of GO silylated with C1Si for various times after heated under vacuum at 500°C. As reported previously, a broad diffraction peak at 2θ of around 24° due to residual carbon without pillar was observed when (C1Si)0.77GO with a low Si content was heated. The diffraction peaks at 2θ of 13–14° and 18–20° due to (002) and (003) of pillared carbon, respectively, were observed for the samples with higher Si contents and they slightly shifted to lower angle, as the increase in the Si content. Pillared carbons with interlayer spacings of 1.27–1.37 nm were obtained from GO silylated with C1Si for 2–5 times. The small difference in the interlayer spacings means that pillared carbons with a large variety of pillar density were obtained.
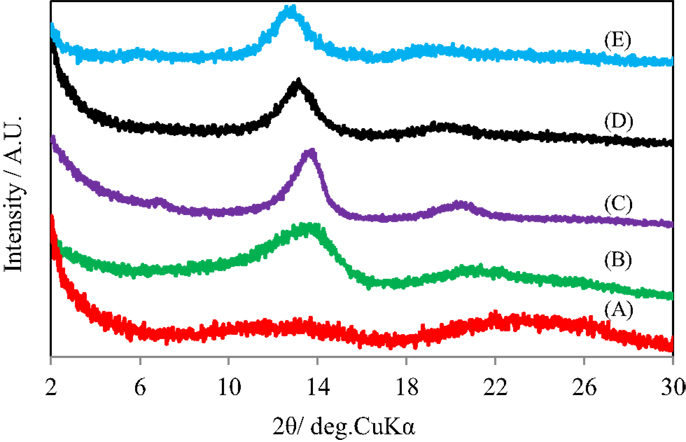
Figure 3. X-ray diffraction patterns of GO silylated with metyltrichlorosilane for (A) 1, (B) 2, (C) 3, (D) 4, and (E) 5 times after heated under vacuum at 500°C.
Figure 4 shows the N2 adsorption isotherms of the pillared carbon samples. The amount of adsorbed N2 molecules on residual carbon obtained from (C1Si)0.77GO was very low, reflecting that it does not possess pores between carbon layers as was observed previously (Matsuo et al., 2007, 2009a). On the other hand, it greatly increased for the pillared carbon obtained from (C1Si)1.55GO. The isotherm was type I and the BET surface area reached 723 m2/g. This means that many micropores were introduced between pillars and carbon layers. The adsorbed amount of N2 decreased as the increase in the Si content in the silylated GO and finally it became almost the same as that observed for residual carbon from (C1Si)0.77GO. This means that the interlayer space is not available for the adsorption of N2 molecules. Figure 5 shows the pore size distribution of pillared carbon. While a peak at 0.56 nm was observed for the sample obtained from (C1Si)1.55GO, it slightly shifted to 0.46 nm for that from (C1Si)2.11GO. The intensity of this peak decreased for the pillared carbon from (C1Si)2.77GO and finally disappeared for that from (C1Si)3.90GO.
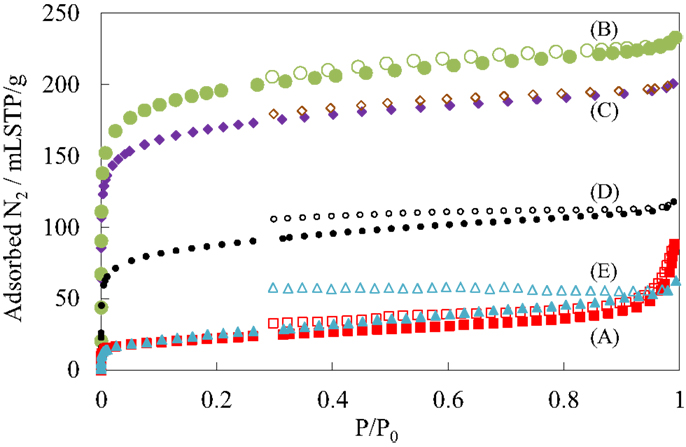
Figure 4. N2 adsorption isotherms of GO silylated with metyltrichlorosilane for (A) 1, (B) 2, (C) 3, (D) 4, and (E) 5 times after heated under vacuum at 500°C.
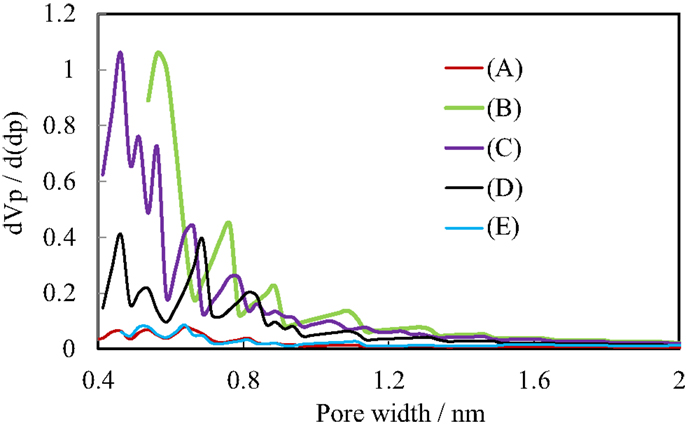
Figure 5. Pore size distribution of pillared carbons, based on the HK method. The colors for the samples are the same as those in Figure 4.
Figure 6 shows the X-ray diffraction patterns of pillared carbons after intercalation of n-octylamine molecules. The diffraction peaks observed at around 2θ = 13.5° for the pristine pillared carbons greatly shifted to lower angle of 2θ = 7.92–8.0° and the interlayer spacing increased from 1.35 to 2.2 nm. The relatively sharp peaks observed at around 2θ = 9.8 and 13.2° are ascribed to the excess n-octylamine molecules deposited on the surface of the pillared carbon samples. The increase in the interlayer spacing means that the pillars of all of these pillared carbons are flexible and they took more perpendicular orientation against the carbon layers upon the intercalation of n-octylamine molecules. In addition, the minimum molecular thickness of n-octylamine molecules was enough smaller than the distance between adjacent pillars for the entrance of the interlayer space of them. Even when the alkyl chain length of the n-alkylamine molecules increased, the diffraction peaks were observed at almost the same positions. This indicates that the pillars are fully extended and the angle of them against carbon layers reached 90°, therefore, the length of pillars are almost identical (1.9 nm) for the pillared carbons with different Si contents.
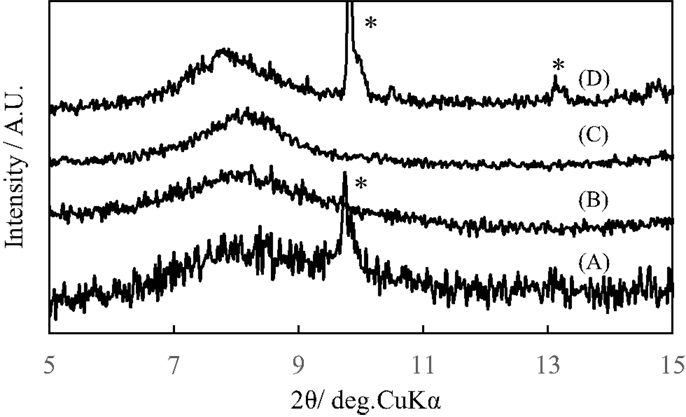
Figure 6. X-ray diffraction patterns of pillared carbons obtained from GO silylated with metyltrichlorosilane for (A) 2, (B) 3, (C) 4, and (D) 5 times after intercalation of n-octylamine molecules. The peaks marked with “*” indicate those of n-octylamine molecules deposited on the surface of pillared carbon samples.
Discussion
The above results show that microporous pillared carbons with interlayer spacings of about 1.3 nm and different pillar densities were obtained from GO repeatedly silylated with methyltrichlorosilane. Interestingly, the pillars in them are flexible and the length of them was almost constant, regardless of the pillar density. Furthermore, n-alkylamine molecules can enter the interlayer space of the pillared carbons with high pillar densities. The reason for these phenomena is discussed as follows, based on the formation process of the pillared carbons. It is reasonable to think that the distribution of the pillars on carbon layers is always the same independent of the Si contents because they are originated from the silylaing reagents attached to hydroxyl groups on GO layer at the 1st silylation process. This leads to the little change in the size of the entrance available for organic molecules as was demonstrated by the intercalation behavior of alkylamines into the above pillared carbons. On the other hand, the same length of the pillars observed for all the pillared carbons with different Si contents might be explained as follows. The length of the pillars of 1.9 nm is comparable to twice of the molecular length of n-butylamine [(0.127 × 4 + 0.28) × 2 = 1.576 nm] used as an exfoliating reagent during the silylation of GO. When the Si contents are low, the linearly grown C1Si oligomers attached to the adjacent GO layers could be connected only in the manner as shown in Figure 7A because of the steric hindrance of n-butylamine molecules. Ladder type silsesquioxane pillars, which we previously proposed for the structure of pillars (Matsuo et al., 2012a) can form when two of the neighboring pillars are attached together. However, once the length of the linearly grown oligomers of C1Si considerably exceeds that of the n-butylamine molecule, they can be connected in several ways such as shown in Figures 7B,C. In case of the latter, the pillar becomes longer; however, in the case of the former, it is almost the same as that in the pillared carbon with lower Si contents. In the presence of the pillars shown in Figure 7B, the interlayer spacing would not exceed 2.2 nm upon the intercalation of alkylamine molecules into pillared carbons, even when they contain some longer pillars such as shown in Figure 7C. The – CH3Si(OH)2 – units marked with circles in Figure 7B reduce the size and/or number of micropores arising in the interlayer space of pillared carbons and lead to the decrease in the surface area as the increase in the Si contents. The small change in the interlayer spacings of pillared carbons with different Si contents also suggests that the majority of the pillars take the structure shown in Figure 7B. It is surprising that n-alkylamine molecules can enter the interlayer space of pillared carbons and the flexibility of the pillars was still maintained even for the pillared carbons with high pillar density and low amounts of adsorbed nitrogen molecules.
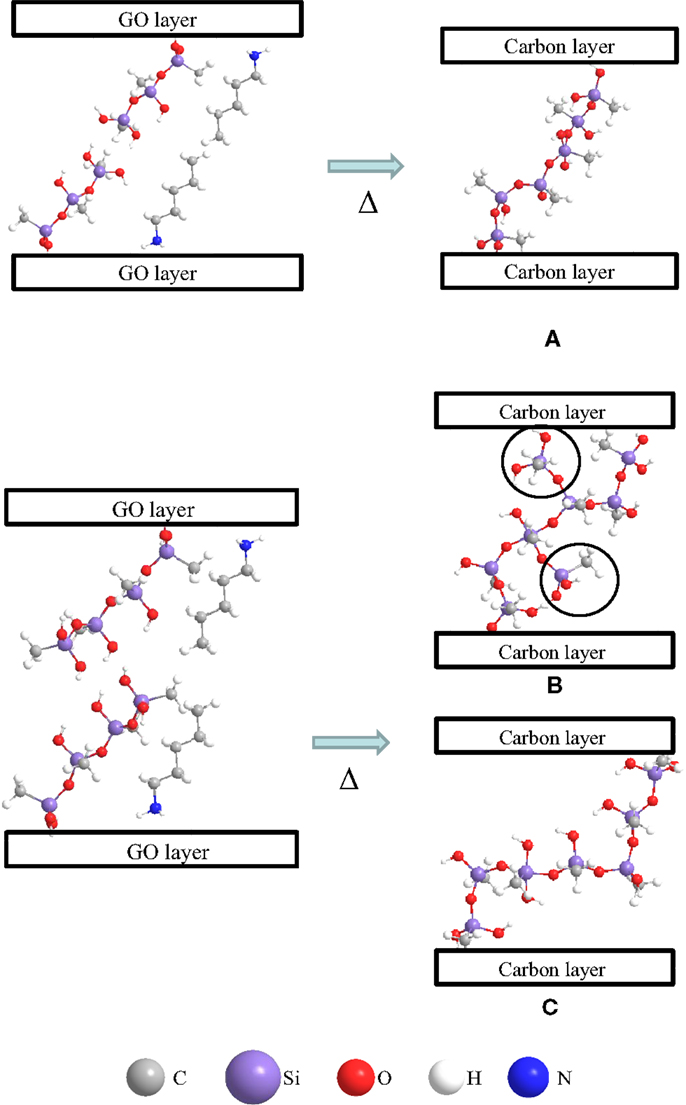
Figure 7. Possible formation processes of pillars consisting of (A), (C) linearly grown, and (B) branched C1Si oligomers.
In conclusion, pillared carbons with different pillar densities were prepared from the thermal reduction of graphite oxide repeatedly silylated with methyltrichlorosilane. The BET surface area of the resulting pillared carbons greatly varied depending on the pillar density in them between 77 and 723 m2/g without losing structural flexibility of pillars upon the intercalation of n-alkylamines. The length of the pillars determined based on the interlayer spacing of them after the intercalation of n-alkylamines was 1.9 nm, which was independent of the pillar density. The n-butylamine molecules added as an exfoliating reagent during the silylation of graphite oxide play an important role for the unchanged length of the pillars.
Conflict of Interest Statement
The authors declare that the research was conducted in the absence of any commercial or financial relationships that could be construed as a potential conflict of interest.
Acknowledgments
A part of this work was financially supported by Canon research Foundation and Japan Science and Technology Agency.
References
Bagshaw, S. A., Prouzet, E., and Pinnavaia, T. J. (1995). Templating of mesoporous molecular sieves by nonionic polyethylene oxide surfactants. Science 269, 1242–1244. doi: 10.1126/science.269.5228.1242
Pubmed Abstract | Pubmed Full Text | CrossRef Full Text | Google Scholar
Brodie, B. C. (1859). On the atomic weight of graphite. Philos. Trans. R. Soc. Lond. 149, 249–259. doi:10.1098/rstl.1859.0013
Dong, G., and Ferdi, S. (2014). Synthesis of non-siliceous mesoporous oxides. Chem. Soc. Rev. 43, 313–344. doi:10.1039/C3CS60155B
Pubmed Abstract | Pubmed Full Text | CrossRef Full Text | Google Scholar
Férey, G. (2008). Hybrid porous solids: past, present, future. Chem. Soc. Rev. 37, 191–214. doi:10.1039/b618320b
Pubmed Abstract | Pubmed Full Text | CrossRef Full Text | Google Scholar
Hoffman, F., and Froeba, M. (2011). Vitalising porous inorganic silica networks with organic functions – PMOs and related hybrid materials. Chem. Soc. Rev. 40, 608–620. doi:10.1039/c0cs00076k
Pubmed Abstract | Pubmed Full Text | CrossRef Full Text | Google Scholar
Horike, S., Shimomura, S., and Kitagawa, S. (2009). Soft porous crystals. Nat. Chem. 1, 695–704. doi:10.1038/nchem.444
Pubmed Abstract | Pubmed Full Text | CrossRef Full Text | Google Scholar
Ito, M., Nishihara, H., Yamamoto, K., Itoi, H., Tanaka, H., Maki, A., et al. (2013). Reversible pore size control of elastic microporous material by mechanical force. Chemistry 19, 13009–13016. doi:10.1002/chem.201301806
Pubmed Abstract | Pubmed Full Text | CrossRef Full Text | Google Scholar
Jiang, H.-L., and Xu, Q. (2011). Porous metal-organic frameworks as platforms for functional applications. Chem. Commun. 47, 3351–3370. doi:10.1039/C0CC05419D
Pubmed Abstract | Pubmed Full Text | CrossRef Full Text | Google Scholar
Kitagawa, S., Kitaura, R., and Noro, S. I. (2004). Functional porous coordination polymers. Angew. Chem. Int. Ed. 43, 2334–2375. doi:10.1002/anie.200300610
Kresge, C. T., Leonowicz, M. E., Roth, W. J., Vartuli, J. C., and Beck, J. S. (1992). Ordered mesoporous molecular sieves synthesized by a liquid-crystal template mechanism. Nature 359, 710–712. doi:10.1038/359710a0
Kyotani, T. (2006). Synthesis of various types of nano carbons using the template technique. Bull. Chem. Soc. Jpn. 79, 1322–1337. doi:10.1246/bcsj.79.1322
Matsuo, Y., Fukunaga, T., Fukutsuka, T., and Sugie, Y. (2004). Silylation of graphite oxide. Carbon N. Y. 42, 2117–2119. doi:10.1016/j.carbon.2004.03.024
Matsuo, Y., Iwasa, K., Mimura, T., and Tachibana, Y. (2014). Preparation of pillared carbon thin films from the reduction of silylated graphite oxide by UV light irradiation and their size selective electrical response to various molecules. Carbon N. Y. 75, 271–276. doi:10.1016/j.carbon.2014.04.001
Matsuo, Y., and Konishi, K. (2011). Size-dependent inclusion of organic molecules into elastic pillared carbons. Chem. Commun. (Camb) 47, 4409–4411. doi:10.1039/c0cc05552b
Pubmed Abstract | Pubmed Full Text | CrossRef Full Text | Google Scholar
Matsuo, Y., and Konishi, K. (2012). Intercalation of various organic molecules into pillared carbon. Carbon N. Y. 50, 2280–2286. doi:10.1016/j.carbon.2012.01.047
Matsuo, Y., Sakai, Y., Fukutsuka, T., and Sugie, Y. (2007). Preparation of pillared carbons by pyrolysis of silylated graphite oxide. Chem. Lett. 36, 1050–1051. doi:10.1246/cl.2007.1050
Matsuo, Y., Sakai, Y., Fukutsuka, T., and Sugie, Y. (2009a). Preparation and characterization of pillared carbons obtained by pyrolysis of graphite oxides silylated by 3-aminopropylmethyldiethoxysilane. Carbon N. Y. 47, 804–811. doi:10.1016/j.carbon.2008.11.040
Matsuo, Y., Komiya, T., and Sugie, Y. (2009b). The effect of alkyl chain length on the structure of pillared carbons prepared by the silylation of graphite oxide with alkyltrichlorosilanes. Carbon N. Y. 47, 2782–2788. doi:10.1016/j.carbon.2009.06.021
Matsuo, Y., Komiya, T., and Sugie, Y. (2012a). Preparation of microporous pillared carbons from the silylated graphite oxide prepared by a two-step method. J. Phys. Chem. Soilds 73, 1424–1427. doi:10.1016/j.jpcs.2011.10.045
Matsuo, Y., Ueda, S., Konishi, K., Marco-Lozar, J. P., Lozano-Castelló, D., and Cazorla-Amorós, D. (2012b). Pillared carbons consisting of silsesquioxane bridged graphene layers for hydrogen storage materials. Int. J. Hydrogen Energy 37, 10702–10708. doi:10.1016/j.ijhydene.2012.04.033
Matsuo, Y., Tachibana, Y., and Konishi, K. (2012c). Preparation of pillared carbon thin films and their size selective electrical response to organic vapor. Carbon N. Y. 50, 5346–5348. doi:10.1016/j.carbon.2012.07.013
Matsuo, Y., Tabata, T., Fukunaga, T., Fukutsuka, T., and Sugie, Y. (2005). Preparation and characterization of silylated graphite oxide. Carbon N. Y. 43, 2875–2882. doi:10.1016/j.carbon.2005.06.006
Rosseinski, M. J. (2004). Recent developments in metal-organic framework chemistry: design, discovery, permanent porosity and flexibility. Microporous Mesoporous Mater. 73, 15–30. doi:10.1016/j.micromeso.2003.05.001
Shimojima, A., and Kuroda, K. (2008). Controlled synthesis of nanostructured silica-based materials from designed alkoxysilanes. J. Solgel Sci. Technol. 46, 307–311. doi:10.1007/s10971-007-1662-3
Yaghi, O. M., O’Keefe, M., Ocwig, N. W., Chae, H. K., Eddaoudi, M., and Kim, J. (2003). Reticular synthesis and the design of new materials. Nature 423, 705–714. doi:10.1038/nature01650
Pubmed Abstract | Pubmed Full Text | CrossRef Full Text | Google Scholar
Yamauchi, Y., Suzuki, N., Radhakrishnan, L., and Wang, L. (2009a). Framework as an electrocatalyst for ethanol oxidation. Angew. Chem. Int. Ed. 49, 5348–5351. doi:10.1002/anie.201000863
Yamauchi, Y., Suzuki, N., Radhakrishnan, L., and Wang, L. (2009b). Breakthrough and future: nanoscale controls of compositions, morphologies, and mesochannel orientations toward advanced mesoporous materials. Chem. Rec. 9, 321–339. doi:10.1002/tcr.200900022
Yang, L., Kinoshita, S., Yamada, T., Kanda, S., Kitagawa, H., Tokunaga, M., et al. (2010). A metal-organic.
Yoo, H. D., Park, Y., Ryu, J. H., and Oh, S. M. (2011). Electrochemical activation behaviors studied with graphitic carbon electrodes of different interlayer distance. Electrochim. Acta 56, 9931–9936. doi:10.1016/j.electacta.2011.08.117
Zeng, M. H., Wang, Q.-X., Tan, Y.-X., Hu, S., Zhao, H.-X., Long, L.-S., et al. (2010). Rigid pillars and double walls in a porous metal-organic framework: single-crystal to single-crystal, controlled uptake and release of iodine and electrical conductivity. J. Am. Chem. Soc. 132, 2561–2563. doi:10.1021/ja908293n
Pubmed Abstract | Pubmed Full Text | CrossRef Full Text | Google Scholar
Keywords: pillared carbon, silylation, graphite oxide, porous properties, intercalation
Citation: Matsuo Y, Hayashida A and Konishi K (2015) Porous properties of pillared carbons prepared from the thermal reduction of graphite oxide repeatedly silylated with methyltrichlorosilanes. Front. Mater. 2:21. doi: 10.3389/fmats.2015.00021
Received: 06 January 2015; Accepted: 26 February 2015;
Published online: 01 April 2015.
Edited by:
Ashok K. Sundramoorthy, University of Wisconsin-Madison, USAReviewed by:
Thiagarajan Soundappan, Washington University in St. Louis, USASenthil Kumar S., VIT University, India
Jianyuan Zhang, University of Washington, USA
Copyright: © 2015 Matsuo, Hayashida and Konishi. This is an open-access article distributed under the terms of the Creative Commons Attribution License (CC BY). The use, distribution or reproduction in other forums is permitted, provided the original author(s) or licensor are credited and that the original publication in this journal is cited, in accordance with accepted academic practice. No use, distribution or reproduction is permitted which does not comply with these terms.
*Correspondence: Yoshiaki Matsuo, Department of Materials Science and Chemistry, Graduate School of Engineering, University of Hyogo, 2167 Shosha Himeji, Hyogo 671-2280, Japan e-mail: ymatsuo@eng.u-hyogo.ac.jp