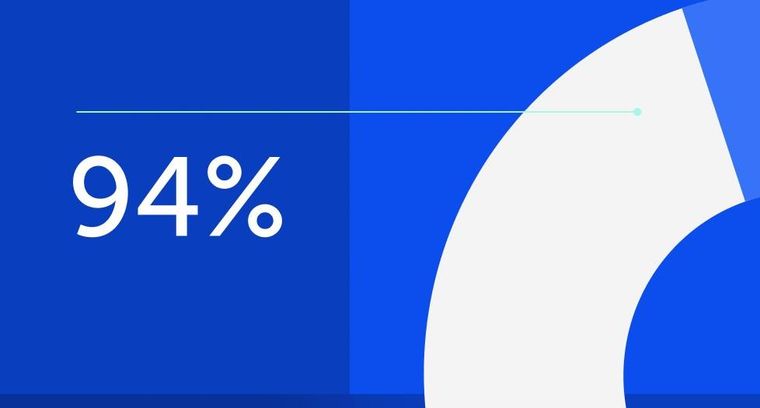
94% of researchers rate our articles as excellent or good
Learn more about the work of our research integrity team to safeguard the quality of each article we publish.
Find out more
ORIGINAL RESEARCH article
Front. Mar. Sci., 03 April 2025
Sec. Aquatic Physiology
Volume 12 - 2025 | https://doi.org/10.3389/fmars.2025.1576079
This article is part of the Research TopicThe Interplay Between Pathogen–Host–Environment on the Emergence of DiseaseView all articles
Introduction: Biofloc technology (BFT) offers a sustainable approach to aquaculture by enhancing water quality and nutrient utilization. This study investigated the effects of varying carbon-to-nitrogen ratios, achieved through different combinations of jaggery and corn flour, rice flour, or rice bran, on the growth performance, body composition, histological health, and immune response of common carp (Cyprinus carpio) fingerlings in a BFT system.
Methods: A 90-day experiment was conducted using common carp fingerlings, where different groups were fed diets supplemented with jaggery in combination with either corn flour, rice flour, or rice bran, creating varying C:N ratios. Water quality parameters, Biofloc volume, growth performance (final weight, weight gain, specific growth rate), body composition (crude protein, crude lipid), histological analysis of liver and intestine, Biofloc morphology, and immune-related gene expression were assessed.
Results: The addition of carbon sources, particularly jaggery and rice flour, significantly improved water quality by reducing total ammonia nitrogen, nitrite, and nitrate levels, and increasing Biofloc volume. The jaggery and rice flour group exhibited the highest final weight, weight gain, specific growth rate, and levels of crude protein and crude lipid. Histological analysis showed improved hepatic and intestinal health in this group. Biofloc morphology varied, with rod shapes observed in the jaggery and rice flour group and spherical peanut shapes in the jaggery and corn flour group. The jaggery and rice flour group also demonstrated elevated expression of immune-related genes.
Discussion: The combination of jaggery and rice flour provided an optimal C:N ratio, leading to enhanced water quality, improved growth performance, better body composition, healthier histological structure, and a strengthened immune response in common carp fingerlings. The unique Biofloc morphology and increased immune gene expression observed in this group suggest a synergistic effect of jaggery and rice flour in promoting a robust and efficient BFT system for common carp aquaculture.
The aquaculture sector, particularly intensive aquaculture systems, has witnessed significant growth, expanding at a rate exceeding 10% annually (Najdegerami and Tukmechi, 2023). However, the environmental implications of these systems are substantial, with effluent discharge posing a significant threat to both freshwater and marine ecosystems, compromising their ecological integrity (Bauer et al., 2006; Abiri et al., 2022). To mitigate these negative externalities and promote sustainable aquaculture practices, researchers worldwide are actively exploring alternative approaches. Recirculating aquaculture systems (RAS) represent a prominent example of such alternatives, although they are not without their own set of challenges.
Recent years have witnessed the emergence of Biofloc Technology (BFT) as a promising aquaculture practice (Xu and Pan, 2014). By meticulously manipulating the carbon-to-nitrogen (C/N) ratio through the incorporation of cost-effective carbon sources, BFT fosters microbial proliferation, leading to a reduction in water exchange requirements and the concurrent generation of valuable single-cell protein (Becerra-Dorame et al., 2011). This innovative approach leverages heterotrophic bacteria and other microorganisms to efficiently degrade fish excreta and organic matter, resulting in the synthesis of microbial biomass while simultaneously mitigating harmful nitrogenous compounds, such as nitrite (NO2-N) and ammonia (NH4-N) (De Schryver et al., 2008; Avnimelech, 2009). Numerous studies have unequivocally demonstrated the multifaceted benefits of BFT on various aspects of fish and shrimp culture. These advantages encompass enhanced growth rates (Bakhshi et al., 2018b; Kumar et al., 2017), improved water quality parameters (Ebrahimi et al., 2019), modulation of gut microbial communities (Li et al., 2018; Kim et al., 2014), fortified immune responses (Long et al., 2015), and elevated antioxidant capacities (Banuelos-Vargas et al., 2021) in common carp aquaculture.
The structural complexity of carbohydrate carbon sources significantly influences the biofilter efficiency and the associated microbial community dynamics (Avnimelech, 2006). Studies by Kumar et al. (2017) have demonstrated that complex carbohydrates, such as those derived from wheat and rice bran, exhibit superior nutritional value compared to simpler sugars like molasses or dextrin. These complex substrates promote robust microbial growth and extended nitrogen removal (as nitrite and ammonium) in BFT systems (Khanjani et al., 2017). Consequently, accelerating the decomposition of complex carbon sources and optimizing nitrogenous waste removal are key research objectives in advancing BFT technology (Romano et al., 2018).
Within the teleost family Cyprinidae, Chinese and Indian carps hold a preeminent position in global freshwater aquaculture production [FAO (Food and Agriculture Organization) (2016)]. Notably, the common carp (Cyprinus carpio) enjoys particularly extensive cultivation in Iran and elsewhere (Ødegård et al., 2010). According to a 2023 report based on FAO data, global common carp production reached 4.2 million metric tonnes in 2020, with rising market demand observed internationally (Barange, 2018; Rahman, 2015; Sándor et al., 2021). BFT has proven advantages for fish growth, water purity, and disease resistance in various aquaculture species, including tilapia (Avnimelech, 2007; Crab et al., 2009; Megahed, 2010), Labeo rohita (Verma et al., 2016), and Cyprinus carpio L. (Bakhshi et al., 2018b) Cyprinus carpio (Ebrahimi et al., 2019). However, despite its widespread study in other species, research on BFT application in Cyprinus carpio aquaculture remains limited (Wang et al., 2015a). Given Cyprinus carpio economic significance and BFT potential to address issues such as high feed costs (Bauer et al., 2012) and water quality management, further research is essential to explore the benefits of BFT for Cyprinus carpio, thereby promoting a sustainable approach to enhance productivity and reduce environmental impact in its farming (Minabi et al., 2020).
This research endeavored to ascertain the synergistic influences of utilizing multiple carbon sources within a biofloc aquaculture paradigm. The central premise posited that these combinatorial approaches would augment biofloc cultivation, thereby culminating in enhanced fish well-being and aquatic milieu quality. Concomitantly, this investigation sought to elucidate whether these synergistic combinations could amplify the overall operational efficacy of the biofloc system. To evaluate these hypotheses, a comprehensive assessment was conducted, encompassing the influence of diverse carbon source combinations on a spectrum of variables, including water quality parameters, growth trajectory, and compositional attributes of fish biomass. Furthermore, the study delved into the intricate microbial community structure of the biofloc, histopathological alterations in fish organs, and the immunological competence of common carp fingerlings cultivated within this biofloc milieu.
200 common carp fingerlings (mean weight 9.4 g ± 1.4 g) were procured from a government hatchery in Nalagarh, India. After a 30-minute saltwater bath (10% NaCl) for disinfection, the fingerlings were acclimatized in a 100 L fiberglass tank for 14 days under controlled conditions. A commercial carp diet (5% crude lipid, 30% crude protein, 6% crude fiber) was administered twice daily at 3% body weight under a light: dark ration is 12:12. To establish a BFT, system, acclimatized species was transferred into 100L treatment water tank. Total ammonia nitrogen (TAN) levels were monitored, and carbon sources (jaggery, corn flour, rice flour, or rice bran) were added following Avnimelech’s (1999) method. This approach, requiring a 20:1 carbohydrate-to-TAN ratio, promotes microbial growth and beneficial byproduct production. Once nitrate, nitrite, and TAN concentrations fell below 10, 2, and 1.5 mg/L, respectively (Pérez-Fuentes et al., 2016), the following amounts of carbon sources were added per tank i.e, 5 g of jaggery, 10 g of rice bran, 10 g of corn flour, and 10 g of rice flour. These amounts were adjusted periodically based on regular monitoring of water quality parameters to maintain the optimal nutrient balance fingerlings were stocked at a density of 20 fish per tank. Experimental treatments included a control group with daily 20% water exchange and no carbon source, and three BFT groups: jaggery + corn flour (JAG: CF+BFT), jaggery + rice flour (JAG: RF+BFT), and jaggery + rice bran (JAG: RB+BFT). All tanks were maintained at a light intensity of 600 lux and continuous aeration. The C:N ratio was managed by adding carbon sources (jaggery, rice bran, corn flour, and rice flour) to maintain a 20:1 ratio, with adjustments made weekly based on water quality monitoring. Carbon was added whenever TAN levels fell below 1.5 mg/L or nitrate/nitrite levels dropped below 10 mg/L and 2 mg/L, respectively. These adjustments ensured the optimal microbial growth and maintained water quality for the fingerlings (Figures 1A, B).
Figure 1. (A) Biofloc culture tank facilities and experimental area. (B) Schematic diagram of biofloc system.
A weekly monitoring protocol was established to evaluate the physicochemical properties of the water at 09:00 hours. A digital pH meter (systolic S091) was used to measure pH. To determine the concentrations of TAN, nitrite nitrogen (NO2-N), and nitrate nitrogen (NO3-N), 50 mL water samples were centrifuged at 6000 rpm for 7 minutes. The supernatant was then analyzed using colorimetric kits (Nice Master Test Kit, India) to quantify the parameters. Biofloc volume (BFV) was assessed weekly by filling an Imhoff cone with 1 L of experimental tank water and recording the volume of settled biofloc (mL/L) after a 30-minute settling period. Dissolved oxygen (DO) levels were measured daily with an AZ instrument 8403 portable DO meter.
Upon experimental conclusion, the growth parameters of the fingerlings were quantified utilizing the following equations:
Following a 90-day experimental period, triplicate samples were randomly collected from each tank. For each replicate, dorsal muscle tissue was harvested from three individuals between the lateral line and dorsal fin. Pooled tissue samples were subsequently subjected to desiccation to achieve constant dry weight, enabling the determination of moisture content. The dried muscle tissue was then ground into a fine powder for further chemical analysis, including the quantification of lipid, protein, and ash content. To assess the nutritional value of microbial flocs, 1 L of floc suspension was collected from each replicate. The flocs were isolated by centrifugation at 5000 rpm for 5 minutes, and the resulting pellet was dried in an oven at 100°C until reaching a constant weight. The dried floc samples were then analyzed for protein and lipid content using standard analytical methods (AOAC, 2000; Cunniff and Washington, 1997).
To evaluate the structural integrity of the hepatic and intestinal tissues, a total of twelve specimens were subjected to experimental manipulation, with each group comprising three replicates. Specimen were euthanized using a clove oil solution, with a concentration maintained between 30 and 50 mg/L, prior to necropsy. The tissues were excised following humane euthanasia and after necropsy. Specifically, after the specimens were euthanized using a clove oil solution and underwent necropsy, the liver and intestine were meticulously dissected for preservation in formalin. The preserved tissues underwent routine histological processing, including dehydration, clearing, paraffin embedding, and sectioning at a thickness of 5 mm. Histological sections were subjected to hematoxylin and eosin (H&E) staining, a conventional method employed for the morphological examination of cellular components (Bradford, 1976). For histomorphometry, ImageJ software was utilized to quantitatively analyze various histological parameters in high-power microscopic fields of the liver and intestinal sections. These measurements included the thickness of the intestinal wall, the size and density of villi (finger-like projections that enhance the absorptive surface area), as well as the presence of any abnormal histological features.
To evaluate the impact of the experimental period on the immune response of fish, renal tissue samples were harvested at its conclusion. Total RNA was isolated from individual specimens utilizing the Trizol method. The expression levels of Complement-C3, Transferrin, and Interleukin-1β (IL-1β) were quantified through quantitative real-time PCR (qPCR) analysis. Specific primers were employed in conjunction with the StepOnePlus system, while β-actin mRNA served as the internal reference gene (housekeeping gene) for data normalization.
Reverse transcription of RNA into cDNA was conducted employing the iScript cDNA Synthesis Kit. Subsequent quantitative polymerase chain reaction (qPCR) assays were performed utilizing Bio-Rad SYBR Green qPCR Master Mix, adhering to the experimental protocol outlined by Kumar et al. (2020). The relative expression levels of interleukin-1 beta (IL-1β), complement component 3 (C3), and transferrin were quantified using a standardized comparative method.
Quantitative PCR (qPCR) reactions were performed in a 20 µL volume containing 10 µL of 2X SYBR Green qPCR Master Mix, 1 µL of 10 pmol forward and reverse primers each, 1 µL of template DNA (30–60 ng), and 7 µL of PCR-grade water. Primer sequences are listed in Table 1. All samples were subjected to triplicate qPCR analysis. Relative gene expression levels were quantified using the 2-ΔΔCt method, with β-actin serving as the internal reference gene for normalization (Pfaffl et al., 2002; Livak and Schmittgen, 2001).
Utilizing JMP statistical software version 18.0, a Two-way ANOVA was performed on the entire dataset to assess the presence of significant differences among the treatment group means. Duncan’s multiple range test was subsequently implemented at a significance level of P< 0.05 to identify specific pairwise differences between treatment groups and the image analysis was performed using ImageJ Version 1.54p software.
Dissolved oxygen (DO) concentrations averaged 7.5 mg/L (range: 6-8 mg/L) and temperature remained stable at 25°C (range: 24-25°C), conditions optimal for common carp cultivation. Dissolved inorganic nitrogen species, including TAN, NO2, and NO3-, exhibited dynamic fluctuations over the experimental period as depicted in Figure 2.
Figure 2. Water physicochemical parameters of different treatments during the 90 days trial on common carp fingerling: (A) TAN (B) pH (C) DO (D) NO2-N (E) NO3-N (F) BVF. Data points represent the mean of three replicate measurements conducted weekly. Standard deviations across all measurements within a week remained within a 5% range of the mean (not graphically depicted). In the figures combination of carbon sources were evaluated: Jaggery: corn flour (JAG:CF + BFT), Jaggery: rice flour (JAG: RF + BFT), and Jaggery: rice bran (JAG: RB + BFT)
TAN concentrations in all experimental groups were maintained within the recommended range (0.28-1 mg/L) for optimal growth of common carp fingerlings (Ajamhasani et al., 2023). However, significant fluctuations in TAN levels were observed across all treatments, with the highest frequency of fluctuations occurring in treatments incorporating BFT. At the experiment’s conclusion, the JAG: RF+BFT treatment exhibited the highest TAN concentration, followed by JAG: RB+BFT, JAG: CF+BFT, and the control. Nitrite-Nitrogen (NO2-N) levels in the control group remained relatively stable throughout the experiment. In contrast, alternative treatments exhibited an initial period of NO2-N accumulation over the first 60 days, culminating in a subsequent rapid decrease in concentration.
Biofloc technology (BFT) treatments demonstrated a progressive elevation of nitrate-nitrogen (NO3-N) concentrations, with a discernible upward trajectory from the baseline measurement, culminating in maximum values within the 70–80-day interval. Furthermore, BFT systems displayed a statistically significant increase in the coefficient of variation for water quality parameters when compared to the control. The JAG: RF+BFT treatment displayed the maximum biofloc volume, followed by JAG: RB+BFT and JAG: CF+BFT. Figure 2 illustrates the trends in pH and DO. pH values varied from 7.35 to 8.65 during the investigation. After a modest preliminary reduction in all groups until 35 Days, a significant diminution was observed in ensuing periods. The JAG: CF+BF treatment exhibited the lowest final pH, followed by JAG: RF+BF and JAG: RB+BF. DO concentrations were consistently lower in JAG: RF+BF and JAG: CF+BF treatments compared to the control and JAG: RB+BFT.
A 90-day comparative study between a conventional control system and a BFT system demonstrated no significant (P > 0.05) variation in key performance indicators, including final body weight, overall productivity, weight gain, SGR, and survival rate. This was achieved despite a 25% reduction in daily feed input under BFT conditions, suggesting improved feed utilization efficiency (Table 2). However, a significant reduction in feed conversion ratio (FCR) was observed in fingerlings subjected to the JAG: RF+BFT treatment compared to other groups (P< 0.05). While no significant FCR differences were noted between JAG: CF+BFT and JAG: RB+BFT treatments (P > 0.05), the control group exhibited the highest FCR, which was significantly different from all other groups (P< 0.05). Table 2 presents the survival probabilities for various treatment cohorts, illustrating favourable survival outcomes across all experimental conditions. The JAG: CF+BFT and JAG: RB+BFT cohorts exhibit statistically superior survival probabilities relative to the untreated cohort, with corresponding p-values less than 0.05.
Table 2. Investigating the impact of varying carbon source combinations on biofloc system efficiency and subsequent growth performance in Cyprinus carpio fingerlings.
The proximate composition of fingerlings was significantly influenced by the type of carbon source employed, as detailed in Table 3. A significant augmentation in both protein and lipid concentrations was detected across all carbon source treatments relative to the control group (P< 0.05). A statistically significant augmentation of these parameters was observed in the JAG: RF+BFT treatment group compared to the control group (P< 0.05). In contrast to the control group, the JAG: CF+BFT treatment exhibited significantly lower moisture content and significantly higher ash content (P< 0.05).
Table 3. Assessing the impact of carbon source combinations on the somatic composition of Cyprinus carpio fingerlings in a BFT environment.
Concurrently, the impact of carbon sources on the biochemical profile of microbial flocs was examined (Table 4). While no significant alterations in protein content were detected among the different treatments (P > 0.05), The JAG: RF+BFT treatment, supplemented with rice flour, demonstrated a statistically significant increase in lipid accumulation within microbial flocs compared to the control group (P< 0.05).
Surface morphological characteristics of biofloc produced with diverse carbon source combinations were investigated utilizing scanning electron microscopy (SEM). Figure 3 presents field emission scanning electron microscopy (FESEM) images of biofloc from [A] Jaggery: corn flour (JAG: CF + BFT), [B] Jaggery: rice flour (JAG: RF + BFT), [C] Jaggery: rice bran (JAG: RB + BFT), and [D] a control group (Figure 3).
Figure 3. Scanning electron microscopic investigation of biofloc structure: (A) Jaggery, corn flour (JAG:CF + BFT); (B) Jaggery, rice flour (JAG: RF + BFT); (C) Jaggery, rice bran (JAG: RB + BFT) and (D) Control.
The biofloc produced with Jaggery: corn flour (JAG: CF + BFT) exhibited a distinctive peanut-shaped morphology, likely indicative of the presence of diatoms. In contrast, the biofloc from Jaggery: rice flour (JAG: RF + BFT) treatments displayed a rod-shaped structure, suggesting a different microbial composition. The biofloc formed with Jaggery: rice bran (JAG: RB + BFT) exhibited irregular circular shapes, possibly due to the diverse microbial community within. The control group, lacking any specific carbon source, did not show a discernible shape.
These findings corroborate previous research by Mahanand et al. (2013), who observed similar bacterial structural characteristics when using wheat flour as a carbon source. The observed variations in biofloc morphology highlight the influence of carbon source on the microbial community composition and the resulting structural features of the biofloc.
Histological analysis of the liver revealed a significant upregulation of protein synthesis in all fish groups, as evidenced by increased basophilic staining of hepatocytes (Figures 4E–H). This, in conjunction with well-organized hepatic architecture and abundant zymogen granules, indicated robust hepatic function. However, fish exposed to JAG: CF + BFT (Figure 4B) and JAG: RF + BFT (Figure 4C) exhibited signs of hepatic degeneration, characterized by a reduced number of active zymogen granules and mild hepatocyte damage.
Figure 4. Intestines: (A–D) and Liver: (E–H) of examined Common Carp for 90 days in BFT-based tanks with diverse carbon sources. Jaggery: corn flour (JAG:CF + BFT) (A, E), Jaggery: rice flour (JAG: RF + BFT) (B, F), and Jaggery: rice bran (JAG: RB + BFT) (C–G) and Control (D, H).
Microscopic examination of the intestines (Figures 4A–D) revealed a generally healthy foregut across all groups, with the exception of the JAG: RB + BFT group, which displayed increased lymphocyte infiltration. Fish reared in BFT systems exhibited morphological alterations in the intestinal villi. The JAG: RF + BFT group showed elongated villi, while the JAG: RF + BFT and JAG: CF + BFT groups exhibited increased villi width (Figures 5, 6).
Figure 5. The effects of combination of carbon sources in BFT system on average villi height and width in intestine of common carp fingerlings.
Figure 6. The effects of combination of carbon sources in BFT system on average goblet cell counts in intestine of common carp fingerlings.
An analysis of gene expression in Figure 7 revealed a statistically significant increase in the levels of renal immune genes, namely Complement C3, IL-1β, and Transferrin, observed in the JAG: RF + BFT group when compared to the control group (p< 0.05). Furthermore, the JAG: CF + BFT and JAG: RB + BFT groups also showed a significant increase in the expression of Complement C3, IL-1β, and Transferrin compared to the control group, but their levels were lower than those observed in the JAG: RF + BFT group (Figure 7).
Figure 7. Gene expression levels of (C3, IL-1B, and Transferrin) in Cyprinus carpio cultured under Biofloc system conditions were evaluated after a 90-day experimental period. Data are presented as mean ± SD. Significant differences (p<0.05) were observed in gene expression levels within the same tissue type.
This investigation demonstrates that the selection of combination carbon source employed in BFT significantly influences a range of developmental parameters in common carp fingerlings. These effects include water quality parameters, fish growth rates, body composition, histological tissue structure, and the expression of genes involved in immunological responses. Intensive aquaculture systems are frequently challenged by the accumulation of toxic nitrogenous compounds, primarily ammonia (NH4+) and nitrite (NO2-), within the aquatic environment (Avnimelech, 1999). Prior investigations have unequivocally demonstrated the deleterious consequences of elevated ammonia concentrations exceeding 0.1 mg L-¹ and nitrite concentrations exceeding 5 mg L-¹ on the health and well-being of cultured fish and shrimp populations (Wang et al., 2015b). The levels of ammonium and nitrite, key nitrogenous species, are highly sensitive parameters for monitoring water quality in high-stocking-density aquaculture (Tovar et al., 2000). The microbial flocs developed in this study, utilizing a diverse array of carbon substrates, demonstrated efficacy in maintaining key water quality parameters within physiologically suitable limits for the optimal growth and well-being of common carp. Notably, a two-phase pattern emerged in the accumulation and subsequent reduction of TAN, NO2-, and NO3-. During the initial 60 days, a steady increase in these nitrogenous compounds was observed across all BFT treatments, likely attributable to the nitrification process as documented in previous BFT studies (Xu et al., 2012a; Zhao et al., 2012; Long et al., 2015; Xu et al., 2016). However, a marked decline in these parameters was evident from day 60 onwards, suggesting the immobilization of nitrogenous compounds by heterotrophic bacteria, which consequently suppressed nitrification. Microbial communities are well-established as pivotal drivers of nutrient turnover within BFT systems (De Schryver and Verstraete, 2009) , with potential contributions to supplementary nutrition (Ray et al., 2012). Our results provide empirical support for this hypothesis. We observed a significant association between temporal shifts in microbial community composition and fluctuations in water quality parameters across the duration of the experiment. Notably, concurrent improvements in water quality parameters were observed alongside shifts in microbial composition. This positive association underscores the critical role of microbial community management in optimizing overall system performance (Ray et al., 2012).
Consistent with Avnimelech (2007), our results indicate that simple carbohydrates, exemplified by the combination of rice flour and jaggery (JAG: RF + BFT), exhibit superior efficacy in decomposing nitrogenous waste products compared to complex carbohydrates such as rice bran. These observations align with the findings of Ekasari et al. (2015) and reinforce the importance of carbohydrate selection in BFT systems. Previous investigations have consistently highlighted the beneficial impacts of BFT on growth performance and feed conversion ratio (FCR) across a range of aquatic species, including Oreochromis spp (Azim and Little, 2008), Macrobrachium rosenbergii (Asaduzzaman et al., 2009), Litopenaeus vannamei (Burford et al., 2004; Xu et al., 2012a, b), Labeo rohita (Mahanand et al., 2013), Clarias gariepinus (Bakar et al., 2015), and Carassius auratus (Wang et al., 2015b).
This research examined the impact of various carbon substrates on the growth performance of juvenile common carp cultured in a BFT environment. Fish cultured in BFT exhibited enhanced growth performance compared to the control group, as indicated by a decrease in FCR. The most pronounced growth promotion was observed in treatments combining rice flour and jaggery (JAG: RF + BFT), which significantly outperformed the control group. While final weight, productivity, weight gain, SGR, and survival rates were marginally elevated in BFT-reared fingerlings, these differences were not statistically significant.
The underlying mechanisms by which BFT enhances common carp growth remain largely unexplored. Previous research has highlighted the proximate and ultimate analyses of microbial flocs, which are rich in essential amino acids, lipids, minerals, and vitamins (Thompson et al., 2002; Ekasari et al., 2014). Our findings corroborate these observations, as the JAG: RF + BFT treatment led to the formation of microbial flocs with elevated protein and lipid content, which were subsequently assimilated by the fish. The observed elevation in fish protein and lipid content, despite a controlled commercial diet, can be directly attributed to the supplementary intake of nutritionally dense microbial flocs. Comparative analysis of the microbial floc composition with established dietary protein (28-32%) and lipid (5-15%) requirements for common carp (Takeuchi et al., 1979) revealed that the protein content was adequate for fingerlings. However, the lipid content was notably lower. Nevertheless, the exogenous provision of carbon sources within the biofloc technology (BFT) system enhanced the utilization of dietary protein and lipid by the fish, corroborating the observations of Crab et al. (2010) and Ekasari et al. (2015) who documented analogous improvements in shrimp nutrition. In accordance with the findings of Najdegerami et al. (2016), our investigation demonstrated that a 25% decrease in DFI significantly enhanced the growth performance of common carp cultivated within BFT systems. The hepatic and intestinal tissues, critical organs for nutrient metabolism, exhibit histological alterations that serve as reliable biomarkers of toxic conditions (Bakhshi et al., 2018a). Our findings corroborate this assertion, as we observed fewer histological changes in the control and biofloc-enriched media compared to the treatments with JAG and RF. In line with Bakhshi et al. (2018b), the absence of significant histological alterations in the liver of common carp reared in biofloc systems with zero water exchange suggests the protective effects of biofloc on hepatic tissue integrity. However, the JAG: RF + BFT group displayed minor hepatic changes characterized by cytoplasmic vacuolation, indicative of hepatotoxicity. This observation aligns and suggesting that the combination of JAG and RF in the presence of biofloc can induce some level of hepatic stress. Intestinal morphology, as assessed by goblet cell counts, villi length, and villi width, was significantly enhanced in the JAG: RF + BFT and JAG: RB + BFT groups compared to the control. These improvements suggest that biofloc supplementation can positively influence intestinal health and nutrient absorption. The upregulation of immune genes, such as interleukin-1β (IL-1β), transferrin, and C3, is often indicative of heightened immune activity (Kumar et al., 2020; Pressley et al., 2005; Hoque et al., 2020). IL-1β, a key pro-inflammatory cytokine, plays a critical role in initiating and sustaining inflammatory responses (Pressley et al., 2005). Transferrins, multifunctional proteins, are essential for iron transport, antimicrobial defense, and cellular growth and protection (Gomme et al., 2005). Serum transferrin levels are closely linked to stress and infection and serve as biomarkers for acute phase responses (Peatman et al., 2007). C3, a pivotal protein within the complement cascade, plays a crucial role in both innate and adaptive immune responses, mediating opsonization, direct lysis, immune regulation, and inflammatory processes (Roy et al., 2016; Zhu et al., 2013). Our investigation revealed a substantial increase in mRNA transcript levels for IL-1β, transferrin, and C3 in common carp subjected to the JAG: RF + BFT experimental condition. The transcriptional levels of these genes were notably elevated, with the highest expression of transferrin. These findings suggest that the BFT environment may enhance stress tolerance and immunological adaptability in common carp.
This research meticulously evaluated the influence of diverse carbon substrate compositions within a biofloc technology (BFT) system on the growth trajectories of common carp fingerlings. Utilizing jaggery combined with corn flour, rice flour, or rice bran as carbon sources within the BFT matrix demonstrably enhanced key water quality metrics, somatic growth indices, nutrient utilization efficiency, and hepatic and intestinal tissue health. A significant negative correlation was observed between increased carbon-to-nitrogen (C/N) ratios and the accumulation of deleterious nitrogenous compounds, while a positive correlation was noted with heterotrophic bacterial proliferation. Notably, the jaggery and rice flour-supplemented BFT treatment (JAG: RF + BFT) yielded the highest final body mass and cumulative weight gain in the experimental fish. Conversely, the JAG: RF + BFT and control groups exhibited optimal feed conversion ratios (FCR) and specific growth rates (SGR), respectively. Collectively, the JAG: RF + BFT regime demonstrated a superior integration of water quality parameters, growth performance, feed efficiency, and biochemical profiles in common carp. These findings provide robust evidence supporting the implementation of refined BFT systems in intensive common carp aquaculture. To elucidate the complex physiological mechanisms underlying the differential responses of common carp to various carbon substrate combinations within the BFT environment, future studies should incorporate advanced transcriptomic and proteomic analyses.
The original contributions presented in the study are included in the article/supplementary material, further inquiries can be directed to the corresponding author/s.
The animal study was approved by Chairman: Dr. Deepak Kapoor, Member secretary: Dr. Lalit Sharma, Main Nominee of CPCSEA: Dr. Munish Goyal, approved/recommended by IAEC Shoolini University, Ethical approval no: IAES/SU/24/11. The study was conducted in accordance with the local legislation and institutional requirements.
NR: Conceptualization, Data curation, Formal Analysis, Investigation, Methodology, Software, Validation, Visualization, Writing – original draft, Writing – review & editing. JJ: Conceptualization, Funding acquisition, Project administration, Supervision, Writing – original draft, Writing – review & editing. AP: Investigation, Methodology, Project administration, Resources, Supervision, Validation, Writing – original draft, Writing – review & editing. SD: Supervision, Validation, Writing – original draft, Writing – review & editing.
The author(s) declare that no financial support was received for the research and/or publication of this article.
Thanks authorities of Shoolini University of Biotechnology and Management Sciences, who provide technical support.
The authors declare that the research was conducted in the absence of any commercial or financial relationships that could be construed as a potential conflict of interest.
The author(s) declare that no Generative AI was used in the creation of this manuscript.
All claims expressed in this article are solely those of the authors and do not necessarily represent those of their affiliated organizations, or those of the publisher, the editors and the reviewers. Any product that may be evaluated in this article, or claim that may be made by its manufacturer, is not guaranteed or endorsed by the publisher.
Abiri S. A., Chitsaz H., Najdegerami E. H., Akrami R., Jalali A. S. (2022). Influence of wheat and rice bran fermentation on water quality, growth performance, and health status of common carp (Cyprinus carpio L.) juveniles in a biofloc-based system. Aquaculture 555, 738168. doi: 10.1016/j.aquaculture.2022.738168
Ajamhasani E., Akrami R., Najdegerami E. H., Chitsaz H., Shamloofar M. (2023). Different carbon sources and probiotics in biofloc based common carp (Cyprinus carpio) culture: Effects on water quality, growth performance, fish welfare and liver histopathology. J. World Aquaculture Soc. 54, 1546–1562. doi: 10.1111/jwas.12993
AOAC (2000). Official Methods of Analysis of AOAC International. 17th edition (Gaithersburg, MD, USA: Association of Analytical Communities).
Avnimelech Y. (1999). Carbon/nitrogen ratio as a control element in aquaculture systems. Aquaculture 176, 227–235. doi: 10.1016/S0044-8486(99)00085-X
Avnimelech Y. (2006). Bio-filters: the need for an new comprehensive approach. Aquac. Eng. 34, 172–178. doi: 10.1016/j.aquaeng.2005.04.001
Avnimelech Y. (2007). Feeding with microbial flocs by tilapia in minimal discharge bioflocs technology ponds. Aquaculture 264, 140–147. doi: 10.1016/j.aquaculture.2006.11.025
Avnimelech Y. (2009). Biofloc technology: A practical guide book (Baton Rouge, USA: World Aquaculture Society), 978-1-888807-16-5.
Azim M. E., Little D. C. (2008). The biofloc technology (BFT) in indoor tanks: water quality, biofloc composition, and growth and welfare of Nile tilapia (Oreochromis niloticus). Aquac. Res. 37, 1406–1412. doi: 10.1016/j.aquaculture.2008.06.036
Bakar N. S. A., Nasir N. M., Lananan F., Hamid S. H. A., Lam S. S., Jusoh A. (2015). Optimization of C/N ratios for nutrient removal in aquaculture system culturing African catfish, (Clarias gariepinus) utilizing bioflocs technology. Int. Biodeter. Biodegr. 102, 100–106. doi: 10.1016/j.ibiod.2015.04.001
Bakhshi F., Najdegerami E. H., Manaffar R., Tukmechi A., Farah K. R. (2018b). Use of different carbon sources for the biofloc system during the grow-out culture of common carp (Cyprinus carpio L.) fingerlings. Aquaculture. 484, 259–267. doi: 10.1016/j.aquaculture.2017.11.036
Bakhshi F., Najdegerami E H., Manaffar R., Tokmechi A., Rahmani Farah K., Shalizar Jalali A. (2018a). Growth performance, haematology, antioxidant status, immune response and histology of common carp (Cyprinus carpio L.) fed biofloc grown on different carbon sources. Aquaculture Res. 49, 393–403. doi: 10.1111/are.13469
Banuelos-Vargas I., de Oca G. A. R.-M., Martinez-Montano E., Perez-Jimenez A., Mendoza-Gamboa O. A., EstradaGodínez J. A., et al. (2021). Antioxidant and immune response of juvenile red tilapia (Oreochromis sp) cultured at different densities in sea water with biofloc plus probiotics. Aquaculture 544, 737112. doi: 10.1016/j.aquaculture.2021.737112
Barange M. (2018). Fishery and aquaculture statistics. FAO yearbook (Fishery and Aquaculture Statistics = FAO Annuaire. Statistiques des Peches et de l’Aquaculture = FAO Anuario. Estadisticas de Pesca y Acuicultura), I–82.
Bauer J. M., Kramer E., Mainzer A. K., Stevenson R., Grav T., Masiero J. R., et al. (2012). WISE/NEOWISE preliminary analysis and highlights of the 67p/Churyumov-Gerasimenko near nucleus environs. Astrophys. J. 758, 18. doi: 10.1088/0004-637X/758/1/18
Bauer E., Williams B. A., Smidt H., Mosenthin R., Verstegen M. W. A. (2006). Influence of dietary components on development of the microbiota in single-stomached species. Nutr. Res. Rev. 19, 63–78. doi: 10.1079/NRR2006123
Becerra-Dorame M. J., Martınez-Cordova L. R., Lopez-Elıas J. A., Martınez-Porchas M. (2011). Evaluation of autotrophic and heterotrophic microcosm-based systems on the production response of Litopenaeus vannamei intensively nursed without Artemia and with zero water exchange. Isr. J. Aquacult. Bamidgeh 63, 620–627. doi: 10.46989/001c.20597
Bradford M. M. (1976). A rapid and sensitive method for the quantitation of microgram quantities of protein utilizing the principle of protein-dye binding. Analytical Biochem. 72, 248–254. doi: 10.1016/0003-2697(76)90527-3
Burford M., Thompsona P. J., McIntoshb R. P., Baumanb R. H., Pearson D. C. (2004). The contribution of flocculated material to shrimp (Litopenaeus vannamei) nutrition in a high-intensity, zero-exchange system. Aquaculture 232, 525–537. doi: 10.1016/S0044-8486(03)00541-6
Crab R., Chielens B., Wille M., Bossier P., Verstraete W. (2010). The effect of different carbon sources on the nutritional value of bioflocs, a feed for Macrobrachium rosenbergii postlarvae. Aquacult. Res. 41, 559–567. doi: 10.1111/j.1365-2109.2009.02353.x
Crab R., Kochva M., Verstraete W., Avnimelech Y. (2009). Bioflocs technology application in over-wintering of tilapia. Aquac. Eng. 40, 105–112. doi: 10.1016/j.aquaeng.2008.12.004
Cunniff P., Washington D. (1997). Official methods of analysis of AOAC International. J. AOAC Int. 80, 127A. doi: 10.1093/jaoac/80.6.127A
De Schryver P., Crab R., Defoirdt T., Boon N., Verstraete W. (2008). The basics of bioflocs technology: the added value for aquaculture. Aquaculture 277, 125–137. doi: 10.1016/j.aquaculture.2008.02.01
De Schryver P., Verstraete W. (2009). Nitrogen removal from aquaculture pond water by heterotrophic nitrogen assimilation in lab-scale sequencing batch reactors. Biosci Biotechnol. 100, 1162–1167. doi: 10.1016/j.biortech.2008.08.043
Ebrahimi A., Akrami R., Najdegerami E. H., Ghiasvand Z., Koohsari H. (2019). Effects of different protein levels and carbon sources on water quality, antioxidant status and performance of common carp (Cyprinus carpio) juveniles raised in biofloc based system. Aquaculture 516, 734639. doi: 10.1016/j.aquaculture.2019.734639
Ekasari J., Angela D., Waluyo S. H., Bachtiar T., Surawidjaja E. H., Bossier P., et al. (2014). The size of biofloc determines the nutritional composition and the nitrogen recovery by aquaculture animals. Aquaculture 426, 105–111. doi: 10.1016/j.aquaculture.2014.01.023
Ekasari J., Zairin M., Putri D. U., Sari N. P., Surawidjaja E. H., Bossier P. (2015). Biofloc-based reproductive performance of Nile tilapia Oreochromis niloticus L. broodstock. Aquac. Res. 46 (2), 509–512. doi: 10.1111/are.12185
FAO (Food and Agriculture Organization) (2016). Cultured aquatic species information programme penaeus monodon (Fabricius 1798). Available online at: http://www.fao.org/fishery/culturedspecies/Penaeusmonodon/en.
Gomme P. T., McCann K. B., Bertolini J. (2005). Transferrin: structure, function and potential therapeutic actions. Drug Discovery Today 10, 267–273. doi: 10.1016/S1359-6446(04)03333-1
Hoque F., Pawar N., Pitale P., Dutta R., Sawant B., Gireesh-Babu P., et al. (2020). Pathogenesis and expression profile of selected immune genes to experimental edwardsiella tarda infection in iridescent shark pangasianodon hypophthalmus. Aquac Rep. 17, 100371. doi: 10.1016/j.aqrep.2020.100371
Khanjani M. H., Sajjadi M. M., Alizadeh M., Sourinejad I. (2017). Nursery performance of Pacific white shrimp (Litopenaeus vannamei Boone 1931) cultivated in a biofloc system: The effect of adding different carbon sources. Aquaculture Res. 48, 1491–1501. doi: 10.1111/are.12985
Kim Y. S., Sasaki T., Awa M., Inomata M., Honryo T., Agawa Y., et al. (2014). Effect of dietary taurine enhancement on growth and development in red sea bream Pagrus major larvae. Aquac. Res. 47, 1168–1179. doi: 10.1111/are.12573
Kumar S., Anand P. S. S., De D., Deo A. D., Ghoshal T. K., Sundaray J. K., et al. (2017). Effects of biofloc under different carbon sources and protein levels on water quality, growth performance and immune responses in black tiger shrimp Penaeus monodon (Fabricius 1978). Aquaculture Res. 48, 1168–1182. doi: 10.1111/are.12958
Kumar V., Wille M., Lourenço T. M., Bossier P. (2020). Biofloc-based enhanced survival of litopenaeus vannamei upon AHPND-causing vibrio parahaemolyticus challenge is partially mediated by reduced expression of its virulence genes. Front. Microbiol. 11. doi: 10.3389/fmicb.2020.01270
Li J., Liu G., Li C., Deng Y., Tadda M. A., Lan L., et al. (2018). Effects of different solid carbon sources on water quality, biofloc quality and gut microbiota of Nile tilapia (Oreochromis niloticus) larvae. Aquaculture 495, 919–931. doi: 10.1016/j.aquaculture.2018.06.078
Livak K. J., Schmittgen T. D. (2001). Analysis of relative gene expression data using real-time quantitative PCR and the 2-DDCT method. Methods 25, 402–408. doi: 10.1006/meth.2001.1262
Long L., Yang J., Li Y., Guan C., Wu F. (2015). Effect of biofloc technology on growth, digestive enzyme activity, hematology, and immune response of genetically improved farmed tilapia (Oreochromis niloticus). Aquaculture 448, 135–141. doi: 10.1007/s10499-012-9557-x
Mahanand S. S., Moulick S., Rao P. S. (2013). Optimum formulation of feed for rohu, Labeo rohita (Hamilton), with biofloc as a component. Aquac. Int. 21, 347–360. doi: 10.1007/s10499-012-9557-x
Megahed M. E. (2010). The effect of microbial biofloc on water quality, survival and growth of the green tiger shrimp (Penaeus Semisulcatus) fed with different crude protein levels. J. Arabian Aquacult Soc 5, 119–142.
Minabi K., Sourinejad I., Alizadeh M., Ghatrami E. R., Khanjani M. H. (2020). Effects of different carbon to nitrogen ratios in the biofloc system on water quality, growth, and body composition of common carp (Cyprinus carpio L.) fingerlings. Aquaculture Int. 28, 1883–1898. doi: 10.1007/s10499-020-00564-7
Najdegerami E. H., Bakhshi F., Lakani F. B. (2016). Effects of biofloc on growth performance, digestive enzyme activities and liver histology of common carp (Cyprinus carpio L.) fingerlings in zero-water exchange system. Fish Physiol. Biochem. 42, 457–465. doi: 10.1007/s10695-015-0151-9
Najdegerami E. H., Tukmechi A. (2023). Poly-β-hydroxybutyrate concentration, microbial enzymes activity, and nutritional value in biofloc system using different carbon sources and C/N ratios in common carp, Cyprinus carpio culture. J. World Aquaculture Soc. 54 (4), 844–860. doi: 10.1111/jwas.12922
Ødegård J., Sommer A. I., Præbel A. K. (2010). Heritability of resistance to viral nervous necrosis in Atlantic cod (Gadus morhua L.). Aquaculture 300, 59–64. doi: 10.1016/j.aquaculture.2010.01.006
Peatman E., Baoprasertkul P., Terhune J., Xu P., Nandi S., Kucuktas H., et al. (2007). Expression analysis of the acute phase response in channel catfish (Ictalurus punctatus) after infection with a gram-negative bacterium. Dev. Comp. Immunol. 31, 1183–1196. doi: 10.1016/j.dci.2007.03.003
Pérez-Fuentes J. A., Hernández-Vergara M. P., Pérez-Rostro C. I., Fogel I. (2016). C: N ratios affect nitrogen removal and production of Nile tilapia Oreochromis niloticus raised in a biofloc system under high density cultivation. Aquaculture 452, 247–251. doi: 10.1016/j.aquaculture.2015.11.010
Pfaffl M. W. (2002). Relative expression software tool (REST(C)) for group-wise comparison and statistical analysis of relative expression results in real-time PCR. Nucleic Acids Res. 30, 36e–3 36. doi: 10.1093/nar/30.9.e36
Pressley M. E., Phelan P. E., Eckhard Witten P., Mellon M. T., Kim C. H. (2005). Pathogenesis and inflammatory response to edwardsiella tarda infection in the zebrafish. Dev. Comp. Immunol. 29, 501–513. doi: 10.1016/j.dci.2004.10.007
Rahman M. M. (2015). Role of common carp (Cyprinus carpio) in aquaculture production systems. Front. Life Sci. 8, 399–410. doi: 10.1080/21553769.2015.1045629
Ray A. J., Seaborn G., Vinatea L., Browdy C. L., Leffler J. W. (2012). Effects of Biofloc reduction on microbial dynamics in minimal-exchange, superintensive shrimp, Litopenaeus vannamei, culture systems. J. World Aquacult. Soc 43, 790–801. doi: 10.1111/j.1749-7345.2012.00607.x
Romano N., Dauda A. B., Ikhsan N., Karim M., Kamarudin M. S. (2018). Fermenting rice bran as a carbon source for biofloc technology improved the water quality, growth, feeding efficiencies, and biochemical composition of African catfish Clarias gariepinus juveniles. Aquaculture Res. 49, 3691–3701. doi: 10.1111/are.13837
Roy S., Kumar V., Kumar V., Behera B. K. (2016). Acute phase proteins and their potential role as an indicator for fish health and in diagnosis of fish diseases. Protein Pept. Lett. 24, 78–89. doi: 10.2174/0929866524666161121142221
Sándor Z. J., Révész N., Varga D., Toth F., Ard o L., Gyalog G. (2021). Nutritional and economic benefits of using DDGS (distiller’ dried grains soluble) as feed ingredient in common carp semi-intensive pond culture. Aquaculture Rep. 21, 100819. doi: 10.1016/j.aqrep.2021.100819
Takeuchi T., Watanabe T., Ogino C. (1979). Optimum ratio of dietary energy to protein for carp. Bull. Jpn. Soc Sci. Fish. 45, 983–987. doi: 10.1111/j.1749-7345.2012.00607.x
Thompson F. L., Abreu P. C., Wasielesky W. (2002). Importance of biofilm for water quality and nourishment in intensive shrimp culture. Aquaculture 203, 263–278. doi: 10.1016/S0044-8486(01)00642-1
Tovar A., Moreno C., Manuel-Vez M., Garcia-Vargas M. (2000). Environmental impacts of intensive aquaculture in marine waters. Water Res. 34, 334–342. doi: 10.1016/S0043-1354(99)00102-5
Verma A. K., Rani A. B., Rathore G., Saharan N., Gora A. H. (2016). Growth, non-specific immunity and disease resistance of Labeo rohita against Aeromonas hydrophila in biofloc systems using different carbon sources. Aquaculture 457, 61–67. doi: 10.1016/j.aquaculture.2016.02.011
Wang C., Pan L., Zhang K., Xu W., Zhao D., Mei L. (2015a). Effects of different carbon sources addition on nutrition composition and extracellular enzymes activity of bioflocs, and digestive enzymes activity and growth performance of Litopenaeus vannamei in zero- exchange culture tanks. Aquac. Res. 47, 1–12. doi: 10.1111/are.12784
Wang G., Yu E., Xie J., Yu D., Li Z., Luo W., et al. (2015b). Effect of C/N ratio on water quality in zero-water exchange tanks and the biofloc supplementation in feed on the growth performance of crucian carp, Carassius auratus. Aquaculture 443, 98–104. doi: 10.1016/j.aquaculture.2015.03.015
Xu W.-J., Morris T. C., Samocha T. M. (2016). Effects of C/N ratio on biofloc development, water quality, and performance of Litopenaeus vannamei juveniles in a biofloc-based, high-density, zero-exchange, outdoor tank system. Aquaculture 453, 169–175. doi: 10.1016/j.aquaculture.2015.11.021
Xu W. J., Pan L. Q. (2014). Dietary protein level and C/N ratio manipulation in zero-exchange culture of Litopenaeus vannamei: evaluation of inorganic nitrogen control, biofloc composition and shrimp performance. Aquac. Res. 45, 1842–1851. doi: 10.1111/are.12126
Xu W. J., Pan L. Q., Sun X. H., Huang J. (2012a). Effects of bioflocs on water quality, and survival, growth and digestive enzyme activities of Litopenaeus vannamei (Boone) in zero- water exchange culture tanks. Aquac. Res. 44, 1093–1102. doi: 10.1111/j.1365-2109.2012.03115.x
Xu W. J., Pan L. Q., Zhao D. H., Huang J. (2012b). Preliminary investigation into the contribution of bioflocs on protein nutrition of Litopenaeus vannamei fed with different dietary protein levels in zero-water exchange culture tanks. Aquaculture 350- 353, 147–153. doi: 10.1016/j.aquaculture.2012.04.003
Zhao P., Huang J., Wang X. H., Song X. L., Yang C. H., Zhang X. G., et al. (2012). The application of bioflocs technology in high-intensive, zero exchange farming systems of Marsupenaeus japonicus. Aquaculture 354-355, 97–106. doi: 10.1016/j.aquaculture.2012.03.034
Keywords: Biofloc, Biofloc volume, common carp, gene expression, histopathology
Citation: Rai N, Julka JM, Panigrahi A and Das SP (2025) Synergistic carbon source utilization in Biofloc aquaculture of common carp (Cyprinus carpio): impacts on growth, health, and environmental parameters. Front. Mar. Sci. 12:1576079. doi: 10.3389/fmars.2025.1576079
Received: 13 February 2025; Accepted: 12 March 2025;
Published: 03 April 2025.
Edited by:
Basanta Kumar Das, Central Inland Fisheries Research Institute (ICAR), IndiaReviewed by:
Pande Gde Sasmita Julyantoro, Udayana University, IndonesiaCopyright © 2025 Rai, Julka, Panigrahi and Das. This is an open-access article distributed under the terms of the Creative Commons Attribution License (CC BY). The use, distribution or reproduction in other forums is permitted, provided the original author(s) and the copyright owner(s) are credited and that the original publication in this journal is cited, in accordance with accepted academic practice. No use, distribution or reproduction is permitted which does not comply with these terms.
*Correspondence: Sofia Priyadarsani Das, ZGFzLnNvZmlhQGdtYWlsLmNvbQ==
Disclaimer: All claims expressed in this article are solely those of the authors and do not necessarily represent those of their affiliated organizations, or those of the publisher, the editors and the reviewers. Any product that may be evaluated in this article or claim that may be made by its manufacturer is not guaranteed or endorsed by the publisher.
Research integrity at Frontiers
Learn more about the work of our research integrity team to safeguard the quality of each article we publish.